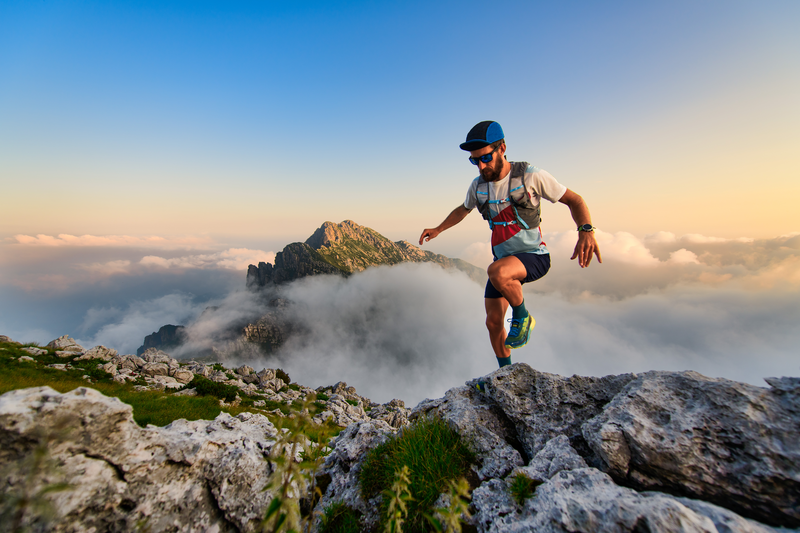
94% of researchers rate our articles as excellent or good
Learn more about the work of our research integrity team to safeguard the quality of each article we publish.
Find out more
MINI REVIEW article
Front. Vet. Sci. , 08 January 2021
Sec. Animal Nutrition and Metabolism
Volume 7 - 2020 | https://doi.org/10.3389/fvets.2020.610541
This article is part of the Research Topic Impact of Climate Change on Poultry Metabolism View all 9 articles
Increased average air temperatures and more frequent and prolonged periods of high ambient temperature (HT) associated with global warming will increasingly affect worldwide poultry production. It is thus important to understand how HT impacts poultry physiology and to identify novel approaches to facilitate improved adaptation and thereby maximize poultry growth, health and welfare. Amino acids play a role in many physiological functions, including stress responses, and their relative demand and metabolism are altered tissue-specifically during exposure to HT. For instance, HT decreases plasma citrulline (Cit) in chicks and leucine (Leu) in the embryonic brain and liver. The physiological significance of these changes in amino acids may involve protection of the body from heat stress. Thus, numerous studies have focused on evaluating the effects of dietary administration of amino acids. It was found that oral L-Cit lowered body temperature and increased thermotolerance in layer chicks. When L-Leu was injected into fertile broiler eggs to examine the cause of reduction of Leu in embryos exposed to HT, in ovo feeding of L-Leu improved thermotolerance in broiler chicks. In ovo injection of L-Leu was also found to inhibit weight loss in market-age broilers exposed to chronic HT, giving rise to the possibility of developing a novel biotechnology aimed at minimizing the economic losses to poultry producers during summer heat stress. These findings and the significance of amino acid metabolism in chicks and market-age broilers under HT are summarized and discussed in this review.
The 0.9°C rise in the world's average surface temperature since the late 19th century has been mainly caused by the increase in carbon dioxide and other human-made emissions into the atmosphere (1). This warming has mostly happened in the past 35 years, with the five warmest years on record having been since 2010 (1). The year 2020 was no exception, with a very high ambient temperature (HT) worldwide in the summer. The HT is a growing challenge for all living organisms, including chickens. In general, because they do not possess sweat glands, but instead rely on evaporative cooling (panting) as main thermoregulatory mechanism, HT is stressful to chickens and interferes with their ability to maintain a homeothermic body temperature (2). The exposure of chickens to HT can cause hyperthermia (3–5). If heat production exceeds heat dissipation capacity, HT can induce stress in chickens (6). This heat stress decreases food intake and body weight (BW) gain, ultimately hindering production and increasing mortality (7–9). Balnave and Oliva (10) reported that absorption of arginine (Arg), an essential amino acid in chickens, significantly decreased when chickens are exposed to heat stress. Several reports showed that the dietary supplementation of certain essential amino acids can mitigate the problems resulting from heat stress in birds (11–16). Increased dietary levels of certain amino acids could be useful to counteract the negative effects of heat stress in chickens. Thus, it is crucial to better understand the importance of this dietary strategy, as well as the role of the amino acids in the metabolism of heat-stressed birds.
Traditionally, it is thought that chicks are not subjected to heat stress. However, series of studies showed that chicks around 2 weeks old are susceptible to HT (35–40°C) when compared with the control thermoneutral temperature (30°C; 4, 5). Several free amino acids have been found to increase in the blood, brain, and muscle of layer chicks within a short time (15 or 30 min) following exposure to HT at 35°C (17); however, when layer chicks were exposed to prolonged HT at 35°C for 48 h, most of these amino acids decreased in the brain and plasma (18). On the basis of these findings, we noticed that citrulline (Cit), which increased in the plasma of layer chicks after short-term heat stress (15 or 30 min at 35°C) and decreased as a result of long-term heat stress (24 or 48 h at 35°C), acts hypothermically in chicks when administered to them orally (19, 20). In addition, thermal manipulation (TM) during embryogenesis was associated with reduced concentrations of leucine (Leu) in the brain and liver of broiler embryos. Notably, in ovo injection of L-Leu afforded thermotolerance in broiler chicks (21, 22). Thus, those amino acids that either increase or decrease in chicks, depending on the length of exposure to heat stress, may be used as biomarkers of heat stress.
In this review, we summarize how heat stress affects amino acid metabolism, with a particular focus on the means by which L-Cit and L-Leu afford thermotolerance. Furthermore, we describe the use of in ovo feeding of L-Leu to attenuate the BW reduction that occurs in market-age broilers under heat stress.
Animal proteins are commonly consisted of 20 amino acids. In growth-phase chicks, 11 of these [arg, histidine, isoleucine, Leu, lysine (Lys), methionine, phenylalanine, threonine, tryptophan, valine, and glycine] are essential amino acids, while the remaining are non-essential (23). Heat stress induces catabolism in organisms (24) in order to provide energy to counter the heat stress. Therefore, any variations in free amino acids that occur under heat stress (17, 18, 25, 26) can be assumed to be the result of catabolic activity. All tissue contains enzymes for amino acid catabolism and synthesis, but their expression and activity varies depending on the tissue's metabolic requirements. In catabolism, deamination/deamidation reactions occur in amino acids, which are followed either by reamination of the carbon skeleton that results to produce non-essential amino acids or by channeling the carbon skeleton into the Krebs cycle. Then, it is either oxidized, funneled toward gluconeogenesis via pyruvate carboxylase, or converted from pyruvate into acetate for the synthesis of fatty acid (27). Glycine is an integral part of the uric acid molecule, and whenever a molecule of uric acid is excreted, a molecule of glycine lost also occurred, particularly during the catabolic phase (28). Amino acids are well-documented to function not only as protein constituents but also as important physiological and behavioral regulators, and this includes the regulation of stress responses (29–34). While there have not been many investigations into the altered free amino acid concentrations found in chickens exposed to heat stress, Ito et al. (17) observed increases in several free essential and non-essential amino acids in the plasma, muscle, and brain in layer chicks following short-term heat stress (15–30 min at 35°C); however, they also found significant reductions in the levels of some other amino acids.
It is still unknown why various amino acids increase in the plasma under short-term heat stress. It is interesting that during short-term heat stress (35°C, 15 or 30 min; 17) the free amino acids have been found to be highly concentrated, in complete contrast to the situation during long-term heat stress (35°C, 24 or 48 h; 18), which was characterized by a reduction in most of the free amino acids. For instance, there was a reduction in tryptophan, Cit, and ornithine (Orn) in the plasma of layer chicks exposed to long-term heat stress (18), whereas the same amino acids increased during short-term heat stress (17). Levels of a variety of free amino acids in the chick brain and breast muscle are also affected by heat stress (17). Interestingly, however, the free amino acids found in the brain and skeletal muscle differ for the most part from those in plasma (17). This suggests that changes in free amino acid concentrations may vary by tissue and be related to tissue-specific enzymatic activity in connection with amino acid metabolism and protein turnover. The concentration of 3-methyl histidine, a marker of proteolysis (35, 36), was found to decline in response to short-term heat stress (17), which suggests that there is a reduction in protein degradation under short-term heat stress (35°C, 15 or 30 min; 17). There is always a balance in the body between protein synthesis and protein degradation, so under short-term heat stress protein synthesis may also be decreased, it may lead to increase in the free amino acid pool in the tissue.
TM during embryogenesis involves increasing the incubation temperature, leading to neonatal chicks (37) and chickens (38) acquiring thermotolerance under HT. We recently reported a significant reduction in some amino acids, including Leu, Lys, and phenylalanine, in the brain and liver of embryos subjected to TM (21). To summarize, amino acid metabolic activity can be influenced by heat stress in broiler embryos and layer chicks.
When L-Cit, L-Orn, and L-Arg were administered in the left ventricle of the brain, there was no reduction in body temperature (19); however, orally administered L-Cit, but not L-Arg or L-Orn, did decrease it under control thermoneutral temperature (19). Furthermore, oral L-Cit lowered the body temperature in layer chicks under heat exposure (20). These results indicate that L-Cit has a hypothermic role.
Nitric oxide (NO) is produced when L-Arg is converted to L-Cit by NO synthase (39) and may act as a hypothermic factor in chicks because thermoregulation has been proposed to be an important physiological function of NO (40), occurring through cutaneous dissipation (41). However, our findings suggest that NO may not be the main factor in L-Cit–dependent reduction of body temperature and thermotolerance (20). Further research is needed to find out the factor(s) involved in L-Cit–dependent reduction of body temperature. It could be concluded that the production of NO may not be a significant factor in L-Cit–dependent reduction of body temperature. As orally administered L-Cit affords thermotolerance in layer chicks, this amino acid could possibly be proposed as a novel nutritional candidate in assisting poultry to cope with heat stress.
A further finding was that there was a significant reduction in Leu in the embryonic brain and liver as a result of the TM. In ovo feeding of L-Leu led to hypothermia in broiler chicks at hatching (21). Metabolic activity was also high during embryogenesis after in ovo feeding of L-Leu. Lipid metabolism increased in broiler embryos and chicks as a result of in ovo administration of L-Leu, which might have been due to greater mitochondrial activity, as Liang et al. (42) reported that L-Leu and its metabolites [α-ketoisocaproate and β-hydroxy-β-methylbutyrate] can stimulate mitochondrial biogenesis and oxidative activities. Plasma triacylglycerol (TG), non-esterified fatty acids (NEFAs), and ketone bodies were higher in broiler chicks fed L-Leu in ovo under heat stress than in heat-exposed control chicks. One might surmise that broiler chicks would benefit from in ovo feeding of L-Leu because fat generates less heat, and dietary fats have well-known beneficial effects in hot-weather feeding programs (8).
As high levels of plasma ketone bodies were found in broiler chicks subjected to L-Leu administration in ovo, the liver might be assumed to produce and release more ketone bodies into the bloodstream (22). As a result, a higher demand for acetyl-CoA may occur in the liver, ultimately stimulating the β-oxidation of fatty acids for the production of more acetyl-CoA. Fatty acid oxidation is heavily exergonic in comparison with glucose oxidation, with a high ATP yield (43). Yahav (44) surmised that hyperthermia during heat stress in meat-producing broilers might be the result of a reduced investment in energy under such conditions. Therefore, the fact that the energy produced through lipid metabolism in male broiler chicks given L-Leu is more readily available might assist in affording thermotolerance under heat stress (Figure 1). However, we have not observed any changes in lipid metabolism in chronic heat–exposed market-age broilers subjected to in ovo L-Leu feeding (45; Figure 1). Thus, it might be predicted that lipid metabolic activity, which was greater in embryos administered L-Leu in ovo, could persist until the neonatal period.
Figure 1. Schematic overview of metabolic possibilities resulting from in ovo administration of L-Leu in embryos, young broiler chicks, and market-age broilers. The broken curved line indicates a possible lipid metabolic memory extending from the embryo to the young chick. Arrows indicate the progression (→), increase (↑), or reduction (↓) of metabolites and metabolic processes. L-Leu, L-leucine; TG, triacylglycerol; NEFA, non-esterified fatty acid; HP, heat production; O2, oxygen; CO2, carbon dioxide; GABA, γ-aminobutyric acid.
As stated above, in ovo administration of L-Leu affords thermotolerance in broiler chicks. However, it is important practically to examine whether this effect continues until market age. Han et al. (45) conducted a study where broilers (4 or 6 weeks old) injected with L-Leu in ovo were examined under acute and chronic heat stress conditions. They measured changes in body temperature and BW, as well as amino acid metabolism and plasma metabolites. After in ovo administration of L-Leu, male broilers (29 or 30 days old) were subjected to acute heat stress (30 ± 1°C for 120 min) or to chronic heat stress (over 30 ± 1°C; aged from 15 to 44 days old). Under acute heat stress, the increased body temperature was found to be suppressed in broilers receiving the in ovo administration of L-Leu, without affecting food intake, plasma TG, NEFA, ketone bodies, glucose, lactic acid, or thyroid hormones. Under chronic heat stress, in ovo administration of L-Leu increased the daily body temperature. Of note, under chronic heat stress, in ovo administration of L-Leu resulted in a higher daily BW gain in comparison with the control group. Furthermore, under acute heat stress, there was a significant increase in several essential amino acids, including Leu and isoleucine, in the liver, and a decrease in their concentration in the plasma, following in ovo administration of L-Leu. These findings indicated that in ovo administration of L-Leu in broilers up to market age produces thermotolerance under conditions of acute heat stress chiefly by altering the amino acid metabolism.
Although there is limited information available on the in ovo injection of amino acids on the performance of broilers under heat stress, dietary administration of some amino acids was found to be beneficial related to growth and meat quality under heat stress. For instance, El-Naggar et al. (46) reported that dietary supplementation of γ-aminobutyric acid (GABA) increased food intake and BW gain in broilers under heat stress. It was found that dietary glutamine improved the antioxidative state of broiler muscles under heat stress (47). Del Vesco et al. (48) showed that dietary methionine supplementation improved protein deposition in acute heat-exposed broilers. Therefore, amino acids could play important role to protect the growth of broilers under heat stress.
In birds, body temperature is considered to be a reliable indicator of thermotolerance acquisition (44). Previously, we have demonstrated that in ovo administration of L-Leu in broilers under acute heat stress produced thermotolerance by lowering body temperature and reducing the mRNA expression of heat-shock proteins (22, 49). In market-age broilers, enhanced body temperature due to acute heat stress was lowered by L-Leu, suggesting that in ovo administration of L-Leu affords thermotolerance under acute, but not chronic, heat stress until market age is reached. It could be that the reduced body temperature resulted from less heat production, from greater heat loss, or from both. Neither food intake nor plasma metabolites (TG, NEFA, and ketone bodies) were influenced by L-Leu administration following 120 min of heat exposure in market-age broilers, suggesting that food intake and lipid metabolism may not contribute much to L-Leu–induced thermotolerance (45). Thyroid hormones, in particular triiodothyronine (T3), are important in modifying the metabolic rate, which influences body-temperature regulation (44). In ovo administration of L-Leu (34.5 μmol) had no effect on T3 concentrations, even when there was a reduction in body temperature during hatching (50). Thus, as suggested by Han et al. (45), plasma thyroid hormones are not influenced by L-Leu under conditions of acute heat stress. TM during embryogenesis, which affords thermotolerance, results in a reduction in thyroid hormones at hatching and in posthatch chicks, as well as in chicks exposed to heat stress (51). L-Leu–mediated thermotolerance could be assumed to be a different mechanism to that of TM. Interestingly, in ovo administration of L-Leu in broilers resulted in an increase in body temperature under chronic heat stress. Under continuous heat stress, higher body temperature might be considered to be an adaptive strategy in the broilers treated with L-Leu, because the increased body temperature has been found to enhance sensible heat loss (44). However, the mechanisms underlying the various responses of body temperature under short-term and chronic heat stress are not clear, and as mentioned by Han et al. (45), molecular and physiological analyses are needed.
Chronic heat stress in broilers has negative effects on feed intake, BW gain, and feed-conversion ratios (52). However, in ovo administration of L-Leu has been found to attenuate the reduction in BW induced by heat stress in broilers (45). In conditions of continuous heat stress, between 31 and 44 days of age, the difference in BW between the in ovo water–administered control and the L-Leu–administered groups tends to be reduced. It is well-known that long-term feed restriction during heat waves in the summer leads to an improvement in heat resistance and thermotolerance, although significantly reducing broiler performance (53). It could be thought that there is a conflict between feed restriction and thermotolerance because feed restriction causes nutritional deficiency in broilers. However, the L-Leu–treated group showed a significantly higher BW compared with the control heat-exposed group, where at 23 days of age the average BW of the L-Leu–administered group was 8.4% higher than that of the control group (45). In ovo administration of L-Leu has been reported to enhance growth in chicken embryos (54), and in neonatal pigs, muscle protein synthesis has been found to be improved by dietary L-Leu supplementation (55, 56). Because in ovo administration of L-Leu appears to maintain a normal growth under cyclic heat stress (45), it might be assumed that in ovo administration of L-Leu accelerates protein synthesis to maintain growth in broilers under heat stress.
Acute heat stress induces catabolic activity in organisms (24). It is well-documented that the liver plays a vital role in the regulation of metabolism, including amino acid metabolism, and it regulates many physiological processes affected by heat stress (57). Many essential amino acids, including Leu, Lys, and isoleucine, were found to be significantly increased in the liver and decreased in the plasma following in ovo administration of L-Leu in comparison with control broilers. Acute heat stress alters amino acid metabolism in chicks (17) and decreases some free amino acid concentrations in the brain and plasma of chick embryos (49). During heat stress, higher amino acid concentrations in the liver might be expected, in order to match other tissues' energy need. Han et al. (45) reported higher hepatic concentrations and lower plasma concentrations of amino acids in the L-Leu–treated group than in the control group, which suggests that there were stronger heat-stress responses related to amino acid metabolism in broilers treated with L-Leu. Thus, in ovo administration of L-Leu might improve amino acid metabolism during acute heat stress. Amino acids are derived from catabolized proteins and provide carbon backbones for glucose or fatty acid production (57); thus, L-Leu administration might enhance energy metabolism in broilers, and high energy is needed to maintain thermoregulation under heat stress (44). Han et al. (45) showed that the concentration of Lys in the liver increased without any reduction in food intake after 120 min of heat stress, which might indicate that this is a form of metabolic support for various organs via the blood circulatory system, because it has been shown that the dietary Lys requirement increases during heat stress (58). In broilers, food intake was not affected, and body temperature was lowered significantly following L-Leu treatment under 120-min heat stress (45). With 180-min heat stress, food intake was reduced significantly, and Lys concentrations remained higher in the plasma and brain in L-Leu–treated broiler chicks in comparison with control chicks (49). Limesand et al. (59) reported that L-Lys oxidation was significantly increased under chronic hypoglycemia for supporting the growth of fetus. The higher concentrations of Lys in the broiler liver fed L-Leu in ovo (43) may be connected to increasing β-oxidation for a higher need of energy supply under heat stress. This suggests that, under heat stress, increased Lys might be a source of higher energy for organs, including the brain, because Lys supplementation compensated for heat stress–induced feed-intake suppression (60). Methionine has been reported to be involved in the expression of stress-related genes, and it provides cellular protection against oxidative stress (61). It has also been reported that supplementation of branched-chain amino acids (Leu, isoleucine, and valine) accelerated protein synthesis and assisted in recovery following a heat-related injury (62). Thus, the changes in amino acid metabolism related to in ovo administration of L-Leu could contribute to thermotolerance in broilers, rather than to lipid metabolism as previously predicted in broiler chicks (Figure 1; 22). Future studies are expected to clarify the mechanisms involved in greater detail. The diencephalon is the area of the brain concerned with thermoregulation (44), and oxidative damage has been found here under conditions of prolonged heat stress (18). Han et al. (45) reported a significantly higher concentration of GABA in the brain of the broilers fed L-Leu in ovo in comparison with the controls under conditions of chronic heat stress. Al Wakeel et al. (63) reported that supplementation with GABA countered the adverse effects of chronic heat stress on growth, antioxidant status, and immune function in broilers. It might be predicted that increased diencephalic GABA concentration from in ovo administration of L-Leu would reduce the negative effects caused by heat stress because GABA is a major inhibitory neurotransmitter, which plays an important role in controlling excitability (64), and it might therefore have helped to protect growth under conditions of chronic heat stress (Figure 1).
Heat stress causes changes in amino acid metabolism in chicks and market-age broilers. Research collectively points to L-Cit and L-Leu because the levels of these two amino acids were affected by heat stress, and treatment with them afforded thermotolerance in chicks and market-age broilers, respectively. Notably, in ovo administration of L-Leu supported a higher BW in market-age broilers in comparison with the control group under conditions of heat stress. Heat stress presents a major problem for poultry production both today and in the future, and our study and others (53, 65–69) can contribute to alleviating this serious global challenge.
All authors listed have made a substantial, direct and intellectual contribution to the work, and approved it for publication.
This work was supported by Grants-in-Aid for Scientific Research from the Japan Society for the Promotion of Science (grant numbers JP21380165, JP17H01503, JP17KT0077, JP15K07694, 18K19271, and JP19H03110).
The authors declare that the research was conducted in the absence of any commercial or financial relationships that could be construed as a potential conflict of interest.
1. NASA, News & Feature Releases,. Available online at: https://www.giss.nasa.gov/research/news/20170118/ (accessed January 18, 2017).
2. Marder J, Arad Z. Panting and acid-base regulation in heat stressed birds. Comp Biochem Physiol A Comp Physiol. (1989) 94:395–400. doi: 10.1016/0300-9629(89)90112-6
3. Yahav S, Hurwitz S. Induction of thermotolerance in male broiler chickens by temperature conditioning at an early age. Poult Sci. (1996) 75:402–6. doi: 10.3382/ps.0750402
4. Chowdhury VS, Tomonaga S, Nishimura S, Tabata S, Cockrem JF, Tsutsui K, et al. Hypothalamic gonadotropin-inhibitory hormone precursor mRNA is increased during depressed food intake in heat-exposed chicks. Comp Biochem Physiol A Mol Integr Physiol. (2012) 162:227–33. doi: 10.1016/j.cbpa.2012.03.009
5. Chowdhury VS, Tomonaga S, Nishimura S, Tabata S, Furuse M. Physiological and behavioral responses of young chicks to high ambient temperature. J Poult Sci. (2012) 49:212–8. doi: 10.2141/jpsa.011071
6. Soleimani AF, Kasim A, Alimon AR, Meimandipour A, Zulkifli I. Ileal endogenous amino acid flow of broiler chickens under high ambient temperature. J Anim Physiol Anim Nutr. (2010) 94:641–7. doi: 10.1111/j.1439-0396.2009.00951.x
7. Lin H, Jiao H, Buyse J, Decuypere E. Strategies for preventing heat stress in poultry. Worlds Poult Sci J. (2006) 62:71–86. doi: 10.1079/WPS200585
8. Daghir NJ. Nutrient requirements of poultry at high temperature. In: Daghir NJ, editor. Poultry Production in Hot Climates. 2nd ed. Wallingford: CABI (2008). p. 132–59. doi: 10.1079/9781845932589.0132
9. Azad MA, Kikusato M, Maekawa T, Shirakawa H, Toyomizu M. Metabolic characteristics and oxidative damage to skeletal muscle in broiler chickens exposed to chronic heat stress. Comp Biochem Physiol A Mol Integr Physiol. (2010) 155:401–6. doi: 10.1016/j.cbpa.2009.12.011
10. Balnave D, Oliva AG. The influence of sodium bicarbonate and sulfur amino acids on the performance of broilers at moderate and high temperatures. Aust J Agric Res. (1991) 42:1385–97. doi: 10.1071/AR9911385
11. Mendes AA, Watkins SE, England JA, Saleh EA, Waldroup AL, Waldroup PW. Influence of dietary lysine levels and arginine: lysine ratios on performance of broilers exposed to heat or cold stress during the period of three to six weeks of age. Poult Sci. (1997) 76:472–81. doi: 10.1093/ps/76.3.472
12. Rose SP, Uddin MS. Effect of temperature on the responses of broiler chickens to dietary lysine balance. Br Poult Sci. (1997) 38:S36–7.
13. Brake J, Balnave D, Dibner JJ. Optimum dietary arginine: lysine ratio for broiler chickens is altered during heat stress in association with changes in intestinal uptake and dietary sodium chloride. Br Poult Sci. (1998) 39:639–47. doi: 10.1080/00071669888511
14. Daghir NJ, Farran MT, Barbour GW, Bexk MM. Nutritive value of high-oil corn grown under semiarid conditions and its impact on broiler performance and carcass composition. Poult Sci. (2003) 82:267–71. doi: 10.1093/ps/82.2.267
15. Willemsen H, Swennen Q, Everaert N, Geraert PA, Mercier Y, Stinckens A, et al. Effects of dietary supplementation of methionine and its hydroxy analog DL-2-hydroxy-4-methylthiobutanoic acid on growth performance, plasma hormone levels, and the redox status of broiler chickens exposed to high temperatures. Poult Sci. (2011) 90:2311–20. doi: 10.3382/ps.2011-01353
16. Dai SF, Gao F, Xu XL, Zhang WH, Song SX, Zhou GH. Effects of dietary glutamine and gamma-aminobutyric acid on meat colour, pH, composition, and water holding characteristic in broilers under cyclic heat stress. Br Poult Sci. (2012) 53:471–81. doi: 10.1080/00071668.2012.719148
17. Ito K, Erwan E, Nagasawa M, Furuse M, Chowdhury VS. Changes in free amino acid concentrations in the blood, brain and muscle of heat exposed chicks. Br Poult Sci. (2014) 55:644–52. doi: 10.1080/00071668.2014.957653
18. Chowdhury VS, Tomonaga S, Ikegami T, Erwan E, Ito K, Cockrem JF, et al. Oxidative damage and brain concentrations of free amino acid in chicks exposed to high ambient temperature. Comp Biochem Physiol A Mol Integr Physiol. (2014) 169:70–6. doi: 10.1016/j.cbpa.2013.12.020
19. Chowdhury VS, Shigemura A, Erwan E, Ito K, Bahry MA, Tran PV, et al. Oral administration of L-citrulline, but not Larginine or L-ornithine, acts as a hypothermic agent in chicks. J Poult Sci. (2015) 52:331–5. doi: 10.2141/jpsa.0150014
20. Chowdhury VS, Han G, Bahry MA, Tran PV, Do PH, Yang H, et al. L-Citrulline acts as potential hypothermic agent to afford thermotolerance in chicks. J Therm Biol. (2017) 69:163–70. doi: 10.1016/j.jtherbio.2017.07.007
21. Han G, Yang H, Bahry MA, Tran PV, Do PH, Ikeda H, et al. L-Leucine acts as a potential agent in reducing body temperature at hatching and affords thermotolerance in broiler chicks. Comp Biochem Physiol A Mol Integr Physiol. (2017) 204:48–56. doi: 10.1016/j.cbpa.2016.10.013
22. Han G, Yang H, Bungo T, Ikeda H, Wang Y, Nguyen LTN, et al. In ovo L-leucine administration stimulates lipid metabolisms in heat-exposed male, but not female, chicks to afford thermotolerance. J Therm Biol. (2018) 71:74–82. doi: 10.1016/j.jtherbio.2017.10.020
24. Maeda E, Kimura S, Yamada M, Tashiro M, Ohashi T. Enhanced gap junction intercellular communication inhibits catabolic and pro-inflammatory responses in tenocytes against heat stress. J Cell Commun Signal. (2017) 11:369–80. doi: 10.1007/s12079-017-0397-3
25. Ito K, Bahry MA, Hui Y, Furuse M, Chowdhury VS. Acute heat stress up-regulates neuropeptide Y precursor mRNA expression and alters brain and plasma concentrations of free amino acids in chicks. Comp Biochem Physiol A Mol Integr Physiol. (2015) 187:13–9. doi: 10.1016/j.cbpa.2015.04.010
26. Eltahan HM, Bahry MA, Yang H, Han G, Nguyen LTN, Ikeda H, et al. Central NPY-Y5 sub-receptor partially functions as a mediator of NPY-induced hypothermia and affords thermotolerance in heat-exposed fasted chicks. Physiol Rep. (2017) 5:e13511. doi: 10.14814/phy2.13511
27. Harvey RA, Ferrier DR. Lippincott's Illustrated Reviews: Biochemistry. 5th ed. Philadelphia, PA: Lippincott William & Wilkins (2011) p. 261–7.
28. Maruyama K, Sunde ML, Harper AE. Conditions affecting plasma amino acid patterns in chickens fed on practical and purified diets. Poult Sci. (1976) 55:1615–26. doi: 10.3382/ps.0551615
29. Asechi M, Tomonaga S, Tachibana T, Han L, Hayamizu K, Denbow DM, et al. Intracerebroventricular injection of Lserine analogs and derivatives induces sedative and hypnotic effects under an acute stressful condition in neonatal chicks. Behav Brain Res. (2006) 170:71–7. doi: 10.1016/j.bbr.2006.02.005
30. Hamasu K, Haraguchi T, Kabuki Y, Adachi N, Tomonaga S, Sato H, et al. L-Proline is a sedative regulator of acute stress in the brain of neonatal chicks. Amino Acids. (2009) 37:377–82. doi: 10.1007/s00726-008-0164-0
31. Hamasu K, Shigemi K, Kabuki Y, Tomonaga S, Denbow DM, Furuse M. Central L-proline attenuates stress-induced dopamine and serotonin metabolism in the chick forebrain. Neurosci Lett. (2009) 460:78–81. doi: 10.1016/j.neulet.2009.05.036
32. Kurauchi I, Shigemi K, Kabuki Y, Hamasu K, Yamane H, Aoki M, et al. Central L-ornithine, but not polyamines, induces a hypnotic effect in neonatal chicks under acute stress. Nutr Neurosci. (2010) 13:17–20. doi: 10.1179/147683010X12611460763481
33. Kurata K, Shigemi K, Tomonaga S, Aoki M, Morishita K, Denbow DM, et al. L-Ornithine attenuates corticotrophin releasing factor-induced stress responses acting at GABAA receptors in neonatal chicks. Neuroscience. (2011) 172:226–31. doi: 10.1016/j.neuroscience.2010.10.076
34. Erwan E, Chowdhury VS, Nagasawa M, Goda R, Otsuka T, Yasuo S, et al. Oral administration of D-aspartate, but not Laspartate, depresses rectal temperature and alters plasma metabolites in chicks. Life Sci. (2014) 109:65–71. doi: 10.1016/j.lfs.2014.05.015
35. Young VR, Munro HN. Ntau-methylhistidine (3-methylhistidine) and muscle protein turnover: an overview. Fed Proc. (1978) 37:2291–300.
36. Nishizawa N. Development and its application of a method to estimate catabolic rate of myofibrillar proteins by measuring urinary excretion of N-methylhistidine. J Nutr Sci Vitaminol. (1983) 36:409–23.
37. Moraes V, Malheiros R, Bruggeman V, Collin A, Tona K, Van As P, et al. Effect of thermal conditioning during embryonic development on aspects of physiological responses of broilers to heat stress. J Therm Biol. (2003) 28:133–40. doi: 10.1016/S0306-4565(02)00049-9
38. Loyau T, Metayer-Coustard S, Berri C, Crochet S, Cailleau-Audouin E, Sannier M, et al. Thermal manipulation during embryogenesis has long-term effects on muscle and liver metabolism in fast-growing chickens. PLoS ONE. (2014) 9:e0105339. doi: 10.1371/journal.pone.0105339
39. Palmer RMJ, Ferrige AG, Moncada S. Nitric oxide release accounts for the biological activity of endothelium-derived relaxing factor. Nature. (1987) 237:524–6. doi: 10.1038/327524a0
40. Szabo C. Physiological and pathophysiological roles of nitric oxide in the central nervous system. Brain Res Bull. (1996) 41:131–41. doi: 10.1016/0361-9230(96)00159-1
41. Mathai ML, Hjelmqvist H, Keil R, Gerstberger R. Nitric oxide increases cutaneous and respiratory heat dissipation in conscious rabbits. Am J Physiol. (1997) 272:R1691–7. doi: 10.1152/ajpregu.1997.272.6.R1691
42. Liang C, Curry BJ, Brown PL, Zemel MB. Leucine modulates mitochondrial biogenesis and SIRT1-AMPK signaling in C2C12 myotubes. Nutr Metab. (2014) 2014:239750. doi: 10.1155/2014/239750
44. Yahav S. Regulation of body temperature: strategies and mechanisms. In: Scanes CG, editor. Sturkie's Avian Physiology. 6th ed. London: Academic Press (2015). p. 869–905. doi: 10.1016/B978-0-12-407160-5.00037-3
45. Han G, Ouchi Y, Hirota T, Haraguchi S, Miyazaki T, Arakawa T, et al. Effects of l-leucine in ovo feeding on thermotolerance, growth and amino acid metabolism under heat stress in broilers. Animal. (2020) 14:1701–9. doi: 10.1017/S1751731120000464
46. El-Naggar K, El-Kassas S, Abdo SE, Kirrella AKK, Wakeel RAA. Role of gamma-aminobutyric acid in regulating feed intake in commercial broilers reared under normal and heat stress conditions. J Therm Biol. (2019) 84:164–75. doi: 10.1016/j.jtherbio.2019.07.004
47. Hu H, Dai S, Li J, Wen A, Bai X. Glutamine improves heat stress-induced oxidative damage in the broiler thigh muscle by activating the nuclear factor erythroid 2-related 2/Kelch-like ECH-associated protein 1 signaling pathway. Poult Sci. (2020) 99:1454–61. doi: 10.1016/j.psj.2019.11.001
48. Del Vesco AP, Gasparino E, Grieser DO, Zancanela V, Voltolini DM, Khatlab AS, et al. Effects of methionine supplementation on the expression of protein deposition-related genes in acute heat stress-exposed broilers. PLoS ONE. (2015) 10:e0115821. doi: 10.1371/journal.pone.0115821
49. Han G, Yang H, Wang Y, Zha ng R, Tashiro K, Bungo T, et al. Effects of in ovo feeding of L-leucine on amino acids metabolism and heat-shock protein-70, and−90 mRNA expression in heat-exposed chicks. Poult Sci. (2019) 98:1243–53. doi: 10.3382/ps/pey444
50. Han G, Yang H, Wang Y, Haraguchi S, Miyazaki T, Bungo T, et al. L-Leucine increases the daily body temperature and affords thermotolerance in broiler chicks. Asian Austral J Anim Sci. (2019) 32:842–8. doi: 10.5713/ajas.18.0677
51. Loyau T, Bedrani L, Berri C, Métayer-Coustard S, Praud C, Coustham V, et al. Cyclic variations in incubation conditions induce adaptive responses to later heat exposure in chickens: a review. Animal. (2015) 9:76–85. doi: 10.1017/S1751731114001931
52. Nawab A, Ibtisham F, Li G, Kieser B, Wu J, Liu W, et al. Heat stress in poultry production: mitigation strategies to overcome the future challenges facing the global poultry industry. J Therm Biol. (2018) 78:131–9. doi: 10.1016/j.jtherbio.2018.08.010
53. Abu-Dieyeh ZHM. Effect of chronic heat stress and long-term feed restriction on broiler performance. Int J Poult Sci. (2006) 5:185–90. doi: 10.3923/ijps.2006.185.190
54. Kita K, Ito K, Sugahara M, Kobayashi M, Makino R, Takahashi N, et al. Effects of in OVO administration of branched-chain amino acids on embryo growth and hatching time of chickens. Poult Sci. (2015) 52:34–6. doi: 10.2141/jpsa.0130208
55. Suryawan A, Davis TA. Regulation of protein degradation pathways by amino acids and insulin in skeletal muscle of neonatal pigs. J Anim Sci Biotechnol. (2014) 5:8. doi: 10.1186/2049-1891-5-8
56. Duan Y, Li F, Liu H, Li Y, Liu Y, Kong X, et al. Nutritional and regulatory roles of leucine in muscle growth and fat reduction. Front Biosci. (2015) 20:796–813. doi: 10.2741/4338
57. Hubbard AH, Zhang X, Jastrebski S, Singh A, Schmidt C. Understanding the liver under heat stress with statistical learning: an integrated metabolomics and transcriptomics computational approach. BMC Genom. (2019) 20:502. doi: 10.1186/s12864-019-5823-x
58. Han Y, Baker DH. Effects of sex, heat stress, body weight, and genetic strain on the dietary lysine requirement of broiler chicks. Poult Sci. (1993) 72:701–8. doi: 10.3382/ps.0720701
59. Limesand SW, Rozance PJ, Brown LD, Hay WW. Effects of chronic hypoglycemia and euglycemic correction on lysine metabolism in fetal sheep. Am J Physiol Endocrinol Metab. (2009) 296:E879–7. doi: 10.1152/ajpendo.90832.2008
60. Corzo A, Moran ET, Hoehler D. Lysine needs of summer-reared male broilers from six to eight weeks of age. Poult Sci. (2003) 82:1602–7. doi: 10.1093/ps/82.10.1602
61. Luo S, Levine RL. Methionine in proteins defends against oxidative stress. FASEB J. (2009) 23:464–72. doi: 10.1096/fj.08-118414
62. Wen H, Naito K, Kinoshita Y, Kobayashi H, Honjoh K, Tashiro K, et al. Changes in transcription during recovery from heat injury in Salmonella typhimurium and effects of BCAA on recovery. Amino Acids. (2012) 42:2059–66. doi: 10.1007/s00726-011-0934-y
63. Al wakeel RA, Shukry M, Azeez AA, Mahmoud S, Saad MF. Alleviation by gamma aminobutyric acid supplementation of chronic heat stress-induced degenerative changes in jejunum in commercial broiler chickens. Stress. (2017) 6:562–72. doi: 10.1080/10253890.2017.1377177
64. McCormick DA. GABA as an inhibitory neurotransmitter in human cerebral cortex. J Neurophysiol. (1989) 62:1018–27. doi: 10.1152/jn.1989.62.5.1018
65. Yahav S, McMurtry JP. Thermotolerance acquisition in broiler chickens by temperature conditioning early in life–the effect of timing and ambient temperature. Poult Sci. (2001) 80:1662–6. doi: 10.1093/ps/80.12.1662
66. Dridi S, Decuypere E, Buyse J. Cerulenin upregulates heat shock protein-70 gene expression in chicken muscle. Poult Sci. (2013) 92:2745–53. doi: 10.3382/ps.2013-03242
67. Nanto F, Ito C, Kikusato M, Toyomizu M. Effects of wholegrain paddy rice on growth performance, oxidative stress and morphological alterations of the intestine in broiler chickens exposed to acute and chronic heat stress. J Poult Sci. (2015) 52:109–18. doi: 10.2141/jpsa.0140009
68. El-Deep MH, Ijiri D, Ebeid TA, Ohtsuka A. Effects of dietary nano-selenium supplementation on growth performance, antioxidative status, and immunity in broiler chickens under thermoneutral and high ambient temperature conditions. J Poult Sci. (2016) 53:274–83. doi: 10.2141/jpsa.0150133
Keywords: amino acids metabolism, body temperature, heat stress, growth performance, poultry
Citation: Chowdhury VS, Han G, Eltahan HM, Haraguchi S, Gilbert ER, Cline MA, Cockrem JF, Bungo T and Furuse M (2021) Potential Role of Amino Acids in the Adaptation of Chicks and Market-Age Broilers to Heat Stress. Front. Vet. Sci. 7:610541. doi: 10.3389/fvets.2020.610541
Received: 26 September 2020; Accepted: 27 November 2020;
Published: 08 January 2021.
Edited by:
Kyung-Woo Lee, Konkuk University, South KoreaReviewed by:
Marco Zampiga, University of Bologna, ItalyCopyright © 2021 Chowdhury, Han, Eltahan, Haraguchi, Gilbert, Cline, Cockrem, Bungo and Furuse. This is an open-access article distributed under the terms of the Creative Commons Attribution License (CC BY). The use, distribution or reproduction in other forums is permitted, provided the original author(s) and the copyright owner(s) are credited and that the original publication in this journal is cited, in accordance with accepted academic practice. No use, distribution or reproduction is permitted which does not comply with these terms.
*Correspondence: Vishwajit S. Chowdhury, dmMtc3VyQGFydHNjaS5reXVzaHUtdS5hYy5qcA== orcid.org/0000-0001-6709-2788
†Present address: Guofeng Han, Department of Animal Nutrition and Food Science, College of Animal Science and Technology, Nanjing Agricultural University, Nanjing, China
Hatem M. Eltahan, Agriculture Research Center, Animal Production Research Institute, Agriculture Ministry, Cairo, Egypt
Disclaimer: All claims expressed in this article are solely those of the authors and do not necessarily represent those of their affiliated organizations, or those of the publisher, the editors and the reviewers. Any product that may be evaluated in this article or claim that may be made by its manufacturer is not guaranteed or endorsed by the publisher.
Research integrity at Frontiers
Learn more about the work of our research integrity team to safeguard the quality of each article we publish.