- 1College of Life Sciences, Yantai University, Yantai, China
- 2College of Food Engineering, Ludong University, Yantai, China
- 3Department of Internal Medicine, University of Texas Southwestern Medical Center, Dallas, TX, United States
- 4College of Veterinary Medicine, China Agricultural University, Beijing, China
Copper overload is an established cause of nephrotoxicity, but the precise molecular mechanism remains unknown. Our study aimed to investigate the molecular mechanism of copper sulfate (CuSO4)-induced nephrotoxicity and the protective effect of the natural compound quercetin using a mouse model. Mice were orally administered CuSO4 only (200 mg/kg per day), or co-administered CuSO4 (200 mg/kg per day) plus quercetin (25, 50, or 100 mg/kg per day), or quercetin only (100 mg/kg per day), or vehicle for 28 days. The blood and kidneys were collected for the examination of serum biomarkers, oxidative stress biomarkers, changes in histopathology and gene and protein expression. Our results show that quercetin supplementation attenuates CuSO4-induced renal dysfunction and tubular necrosis in a dose-dependent manner. Quercetin supplementation at 50 and 100 mg/kg significantly attenuated CuSO4-induced oxidative damage. Quercetin supplementation also inhibited the activities of caspases-9 and−3, and the expression of p53 and Bax mRNAs. Furthermore, quercetin supplementation markedly activated the expression of Nrf2 and HO-1 mRNAs, but inhibited the expression of NF-κB, IL-1β, IL-6, and TNF-α mRNAs. In conclusion, our results revealed that quercetin supplementation could inhibit CuSO4-induced nephrotoxicity in mice via the inhibition of mitochondrial apoptotic and NF-κB pathways and the activation of Nrf2/HO-1 pathway. Our study highlights quercetin as a potential candidate in treating copper overload-induced nephrotoxicity.
Introduction
Copper (Cu) is a transition metal that functions as an essential trace element in the process of metabolism, growth, and development in human and animals (1). Cu plays a critical role in the activities of numbers of metalloenzymes, including dopamine beta-mono-oxygenase, cytochrome-c oxidase, and Cu, Zn-superoxide dismutase (1). In some countries, copper sulfate (CuSO4) has been widely used as a feed additive in animal production (2). However, copper overload could cause the tissue or organ damage in human and other mammals, affecting the liver, kidney, brain, lung, intestine, heart, and testis (3–10). Over the past decades, Cu overload was suggested to be associated with the development of Wilson's disease and other neurodegenerative diseases, which has raised concerns worldwide (7, 11).
The kidney is susceptible to copper toxicity due to its important role in the physiology of filtration. Cu overload results in compromised kidney function through renal phosphaturia, diminished glomerular filtration, proteinuria, and amino aciduria (12). It has been previously reported that oral administration of CuSO4 at the dose of 100 mg/kg per day for 30 days can cause marked renal damage in rats (4). Earlier studies also reported that the molecular mechanism of Cu nephrotoxicity involved oxidative stress, apoptosis and autophagy, which were characterized by various signaling pathways, including mammalian target of rapamycin (mTOR) pathway, nuclear factor kappa B (NF-κB) pathway, p53 pathway, and the endoplasmic reticulum (ER) stress pathway (13–15). In in vitro models, CuSO4-induced cell death is associated with increases in the levels of senescence, apoptosis, autophagy, mitochondrial damage, and excessive production of reactive oxygen species (ROS) (16). Recently, Alharbi and colleagues found that turmeric could protect CuSO4-induced nephrotoxicity in rats by inhibiting oxidative stress and apoptosis (14). However, to date, we still have limited therapeutic options to treat nephrotic tissue damage caused by Cu overload or poisoning. The development and discovery of novel drugs that treat or prevent Cu overload-induced nephrotoxicity remains an unmet need.
Quercetin is a natural compound whose chemical formula is 3′, 3′, 4′, 5′, 7′-pentahydroxyflavone (Figure 1). Quercetin has many biological functions including anti-oxidant, anti-inflammatory, and immune-regulatory activities (17–19). Quercetin supplementation had been shown to attenuate D-Galactose-induced renal damage in rats and aflatoxin B1-induced neurotoxicity in mice through the inhibition of oxidative stress (20, 21). A recent study also showed that quercetin supplementation could inhibit lipopolysaccharide (LPS)-induced inflammation in a proximal tubular cell line human kidney 2 (HK-2) cells which derived from normal human kidney (22). Yet, whether quercetin may have a protective role in Cu overload-induced nephrotoxicity remains unknown. Therefore, in the present study, we investigated the potential protective effect of quercetin supplementation on CuSO4 exposure-induced nephrotoxicity in mice and the underlying molecular mechanisms.
Materials and Methods
Reagents and Chemicals
Quercetin (purity ≥98%) was bought from Aladdin (Shanghai, China). Copper sulfate (CuSO4•5H2O) was bought from Sinopharm (Shanghai, China). Carboxmethyl cellulose (CMC-Na) was obtained from Sigma-Aldrich (St. Louis, MO, USA). The standard diagnostic kits for blood urea nitrogen (BUN) and creatinine (CRE) were bought from Shanghai Kehua Bio-engineering Co., Ltd. (Shanghai, China). Phenylmethylsulfonyl fluoride (PMSF), aprotinin, pepstatin A, and leupeptin were purchased from AMRESCO Inc. (Ohio, USA). All chemicals were greater than or equal to analytical grade.
Animals and treatments
All animal studies were approved by the Institutional Animal Care and Use Committee (IACUC) of Ludong University, Yantai, China (LDU-IRB20290503). Male C57BL/6 mice (8 weeks, 20–22 g) were purchased from Vital River Animal Technology, Co., Ltd. (Beijing, China). All mice had a 1 week acclimation period before experimentation. All mice were given the sufficient chow and water and housed under a light-dark period of 12 h, and a relative humidity of 50 ± 10%, and a temperature of 23 ± 2°C.
Sixty mice were split equally into the following 6 groups of 10 mice per group.
(i) control group;
(ii) quercetin 100 mg/kg/day only (QUE100) group;
(iii) CuSO4 200 mg/kg/day only group;
(iv) CuSO4 200 mg/kg/day plus quercetin 25 mg/kg/day (QUE25 + CuSO4) group;
(v) CuSO4 200 mg/kg/day plus quercetin 50 mg/kg/day (QUE50 + CuSO4) group
(vi) CuSO4 200 mg/kg/day plus quercetin 100 mg/kg/day (QUE100 + CuSO4) group
Control group mice were administered orally with equal volume of 0.5% CMC-Na (vehicle). Mice in all CuSO4 groups were administered orally with aqueous solution of CuSO4 at the dose of 200 mg/kg per day, in line with a previously published study (23). The previous studies reported that oral supplementation of quercetin in the range of 25–100 mg/kg/day for 1–4 weeks could protect against cadmium or gentamicin -induced renal toxicity in rats (24, 25). Therefore, the dose of quercetin at 25, 50, and 100 were used in the present study. Quercetin was dissolved in 0.5% CMC-Na and administered orally 2 h before CuSO4 administration. All sets of mice were treated with the respective chemicals for 4 weeks (e.g., 28 days). 24 h after the final dose, mice were humanely euthanized using sodium pentobarbital (80 mg/kg; intraperitoneal injection). Blood samples and kidney tissues were collected for histopathological, biochemical, and gene and protein expression examination, respectively.
Measurements of Serum Markers Creatinine (CRE) and Blood Urea Nitrogen (BUN)
Blood samples were collected in 1.5 mL-tubes and centrifuged for 15 min at 3,000 × g, then the serum was isolated. The levels of BUN and CRE were determined by using Hitachi 7080 automated chemical analyzer (Hitachi High-Technologies Corporation, Tokyo, Japan).
Histopathology Examination
Parts of kidneys were selected randomly from four mice. Tissues were fixed in 10% neutral-buffered formalin. After 48 h, kidney samples were used to prepare histological sections and hematoxylin-eosin (H&E) staining were performed. The histopathological scoring was done by a blind scorer using a semi-quantitative score (SQS) corresponding to the degree of renal tubular damage, following a previously published method (26).
Ultrastructural Observation of Mitochondrial Morphometry in Kidney Tissues of Mice
Cortical sections of the murine kidneys were isolated and 1 mm cubes were cut for the ultrastructure analysis. In brief, samples fixed in 2.5% glutaraldehyde (buffered at pH – 7.2) were stored at 4°C overnight. After fixation, the samples were treated with 2% OsO4 dissolved in cacodylate buffer (0.1 M, pH 7.4, 4°C) for 2 h, then processed to dehydrate, embed, and slice. Finally, the ultrathin sections (70–80 nm) were stained with solutions of lead citrate and uranyl acetate. The images were taken by using a JSM25610LV transmission electron microscope (TEM) at a voltage of 100 kV.
Measurements of Oxidative Stress Biomarkers
An amount of 50–100 mg of renal tissue was homogenized in 1 mL of homogenization buffer (0.1 mM Na-EDTA, 0.01 M Tris-HCl, 0.9% saline, 10 mM sucrose; pH 7.4) at 4°C. The supernatant was collected after centrifugation at 13,000 × g for 15 min at 4°C. The levels of glutathione (GSH), nitric oxide (NO) and malonaldehyde (MDA) and the activity of catalase (CAT), superoxide dismutase (SOD), and inducible nitric oxide synthase (iNOS) were determined by employing commercial MDA, GSH, NO SOD, CAT and iNOS assay kits (Nanjing Jiancheng, China). Total protein concentrations for all experiments were determined by using BCA assay kit (Beyotime,Haimen, China).
Measurement of caspases-3,−9 activities
Caspases-3 and−9 activities in nephrotic tissue were examined by using the commercial caspase-3 and caspase-9 Assay kit (Beyotime, Haimen, China), respectively. Protocols were followed to the manufacturer's instructions.
Measurement of TNF-α, Interleukin-1β (IL-1β), and Interleukin-6 (IL6) Levels in Renal Tissue
After treatment, the levels of IL-1β, TNF-α, and IL-6 in the renal tissues were measured by using IL-1β, TNF-α, and IL-6 enzyme-linked immunosorbent assay kits (R&D Systems, Minnesota, USA), as per manufacturer instructions.
Western Blot
An amount of 10–20 mg of renal tissue was homogenized in 500 μL lysis buffer (0.1 M Tris-HCl, 2% SDS, 10% glycerol, 4°C; pH 7.4) with the mixture of protease inhibitor (1 mM PMSF plus the mixture of 1 μg/mL pepstatin A, 1 μg/mL leupeptin, and 1 μg/mL aprotinin). The supernatants were collected after centrifugation at 14,000 × g for 15 min at 4°C. Total protein levels were determined using a BCA assay kit. The primary antibodies used were against Bax (1:1,000), cleaved-caspase-3 (1:500), and GAPDH (1:5,000) (Cell Signaling Technologies, USA). The protein expressions were quantified using Image J software and normalized to the corresponding GAPDH bands.
Quantitative Real-Time (qRT)-PCR
An amount of 30–50 mg of renal tissue was used to extract total RNA via the TRIZOL method (Life Technologies, Grand Island, NY, USA), as per manufacturer instructions. The integrity of collected RNA was checked by tabulating the ratio of the OD taken at 260 and 280 nm. Then, 1 microgram of total RNA from each tissue sample was used to reverse transcribe into cDNA using the Prime Script kit (Takara biotech, China). Quantitative real-time (qRT)-PCR reactions were carried out on the AB7500 instrument (Applied Biosystems, USA) and the expression of Nrf2, NF-κB, HO-1, IL-6, IL-1β, and TNF-α mRNA in the kidney tissue samples were measured and normalized to β-actin. The primer sequences used are listed as forward (top) and reverse (bottom) in Table 1.
Statistics
All data are analyzed and represented as mean ± S.E.M. unless specified otherwise. Statistical analyses were performed on the SPSS V18.0 platform (SPSS Inc., IL, USA). A one-way ANOVA with Tukey's multiple comparison test was used when variance was homogenous; otherwise, Dunnett's T3 test was employed. A P-value lesser than 0.05 was set as statistical significance.
Results
Quercetin Supplementation Attenuated CuSO4-induced Kidney Dysfunction and Tubular Necrosis
Compared to the control group, serum BUN and CRE levels increased to 9.5 mmol/L and 91.2 μmol/L in the CuSO4 only group (both P < 0.01), respectively (Figure 2). Quercetin supplementation at the doses of 50 and 100 mg/kg/day significantly attenuated the CuSO4 exposure-induced increase in BUN and CRE levels (all P < 0.05 or 0.01). In the QUE50 + CuSO4 and QUE100 + CuSO4 groups, the serum BUN level decreased to 7.7 and 6.8 mmol/L, respectively (Figure 2A); the serum CRE level decreased to 72.1 and 56.6 μmol/L, respectively (Figure 2B).
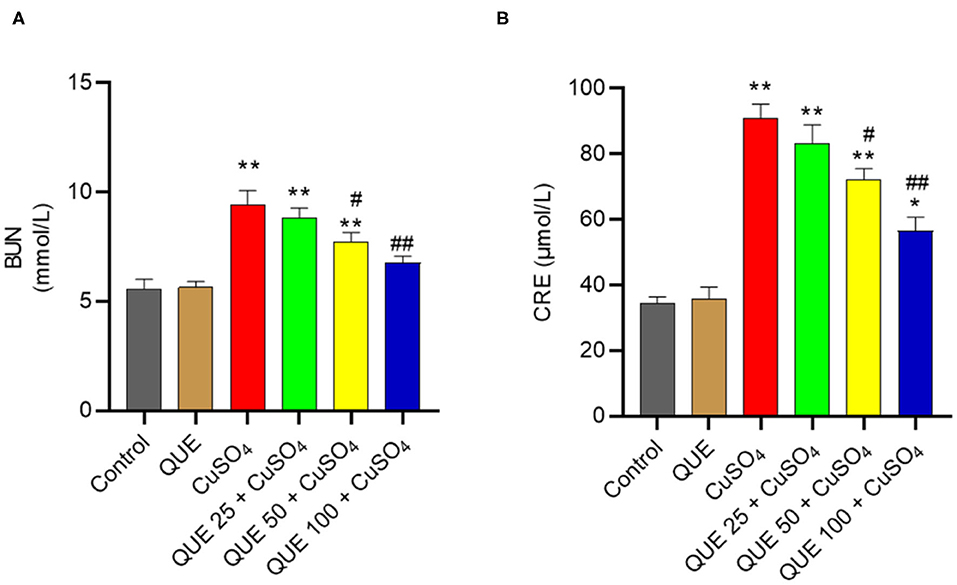
Figure 2. Effects of quercetin supplementation on the serum blood urea nitrogen (BUN) (A) and creatinine (CRE) (B). Data are reported as mean ± S.E.M (n = 10). *P < 0.05, or **P < 0.01, compared to the untreated control group, respectively; #P < 0.05, or ##P < 0.01 compared to CUSO4 alone group, respectively. QUE, quercetin.
Quercetin supplementation also attenuated CuSO4-induced histopathological damages (Figure 3). Compared to the control group, marked tubular degeneration, tubular dilation, and necrosis were detected in the CuSO4 only groups, but these pathological changes were significantly lesser in the QUE50 + CuSO4 and QUE100 + CuSO4 groups (P < 0.05 or 0.01) (Figure 3A). In our scoring model, the SQS values decreased considerably from 3.6 to 2.4 and 1.5 in the QUE50 + CuSO4 and QUE100 + CuSO4 groups, respectively, compared to the CuSO4 alone group (Figure 3B). Quercetin supplementation at 25 mg/kg/day had no discernable effect on the biochemical parameters (i.e., BUN and CRE) and histopathological damages caused by CuSO4 exposure. There was no significant change in the levels of serum BUN and CRE or histopathological changes in the QUE100 alone group, compared to control group (Figures 2, 3).
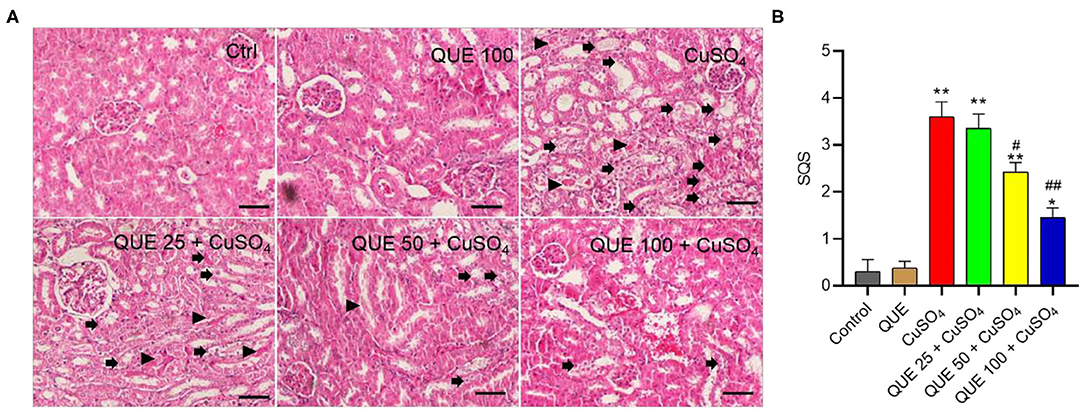
Figure 3. Representative histological sections of mouse kidney and the semi-quantitative scores (SQSs). (A) Observed histopathological changes of kidneys from control, QUE100 alone, CuSO4 alone, CuSO4 + QUE25, CuSO4 + QUE50, and CuSO4 + QUE100 groups. Black arrows indicate necrosis, tubular dilation and tubular degeneration; arrowheads indicate urinary cast formation. (B) SQSs are presented as the average ± S.E.M (n = 4). *P < 0.05, or **P < 0.01, compared to the untreated control group, respectively; #P < 0.05, or ##P < 0.01, compared to the CuSO4 alone group, respectively. Hematoxylin-eosin staining. Bar = 50 μm. Ctrl, control; QUE, quercetin.
Quercetin Supplementation Attenuated CuSO4-induced Oxidative Stress in the Kidney Tissues
CuSO4 exposure markedly induced oxidative stress in the kidney tissues of mice (Figure 4). CuSO4 exposure significantly increased in the levels of MDA, iNOS and NO to 3.6 nmol/mg protein, 0.84 U/mg protein and 10.6 μmol/g protein (all P < 0.01), respectively. It also significantly decreased the activities of SOD and CAT and the level of GSH to 107.7 U/mg protein, 68.1 U/mg protein, and 12.1 μmol/g protein (all P < 0.01), respectively. Quercetin supplementation at the doses of 50 and 100 mg/kg/day significantly downregulated the levels of MDA (to 2.5 and 2.3 nmol/mg protein, respectively; Figure 4A), the activities of iNOS (to 0.65 and 0.56 U/mg protein, respectively; Figure 4E) and the levels NO (to 7.9 and 6.4 μmol/g protein, respectively; Figure 4F). Compared to the CuSO4 alone group, quercetin supplementation at the doses of 50 and 100 mg/kg/day also significantly upregulated the activities of SOD (to 121.5 and 128.9 U/mg protein, respectively; Figure 4B), CAT (to 87.3 and 94.4 U/mg protein, respectively; Figure 4C), and GSH levels (to 15.6 and 16.3 μmol/g protein, respectively; Figure 4D) (all P < 0.05 or 0.01). Quercetin supplementation at 25 mg/kg/day did not significantly attenuate CuSO4-induced oxidative stress. In the quercetin alone group, the levels of MDA, NO, GSH and the activities of SOD, CAT, and iNOS showed no significant alterations, compared to that in the kidneys of untreated mice (Figure 4).
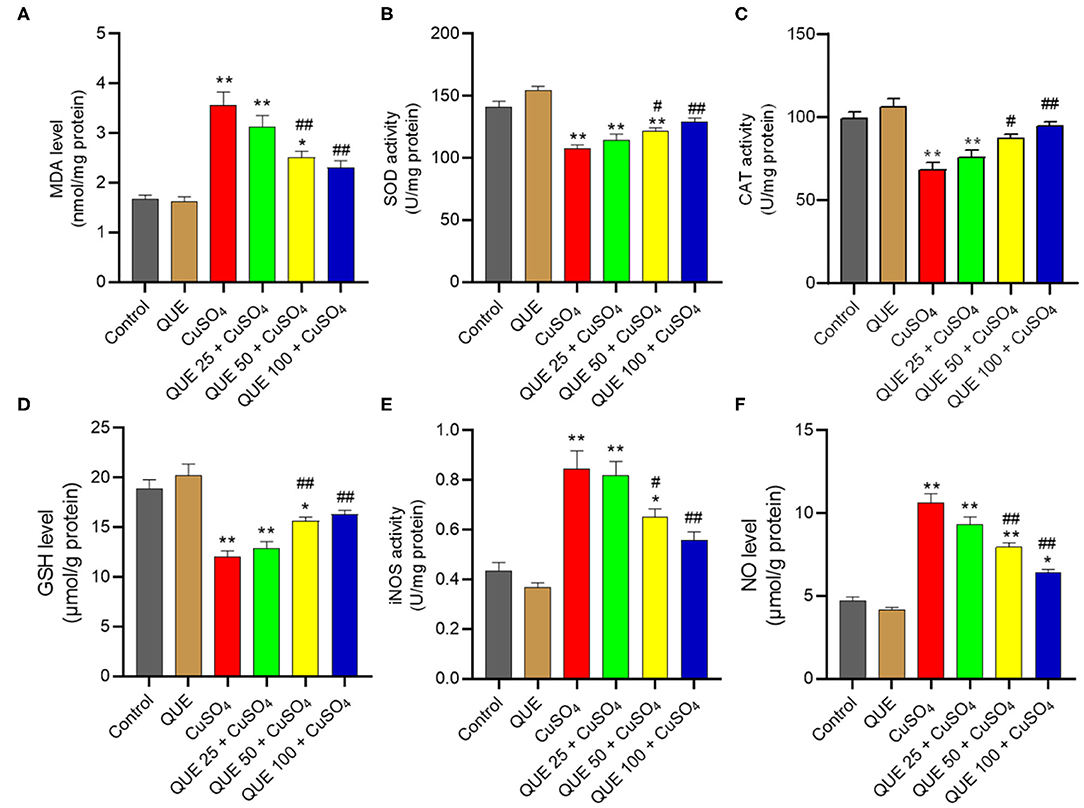
Figure 4. Quercetin supplementation attenuates CuSO4-induced oxidative stress in the mouse's kidney tissue. (A) malondialdehyde (MDA) levels; (B) the activity of superoxide dismutase (SOD); (C) the activity of catalase (CAT); (D) Glutathione (GSH) levels; (E) the activities of inducible nitric oxide synthase (iNOS); (F) nitric oxide (NO) levels. Data are reported as mean ± S.E.M (n = 10). *P < 0.05, or **P < 0.01, compared to the untreated control group, respectively; #P < 0.05, or ##P < 0.01, compared to the CuSO4 alone group, respectively. QUE, quercetin.
Quercetin Supplementation Attenuated CuSO4-induced Expressions in the Levels of IL-1β, TNF-α, and IL-6 Proteins
Compared to the control group (Figure 5A), quercetin treatment alone did not significantly alter mitochondrial morphology (Figure 5B). CuSO4 exposure led to marked pathological changes in mitochondrial morphology, characterized by the appearance of swollen and ruptured mitochondria, and the disappearance of cristae (Figure 5C). These pathological changes were significantly diminished in the QUE50 + CuSO4 and QUE100 + CuSO4 groups, but not in the QUE25 + CuSO4 group (Figures 5E,F).
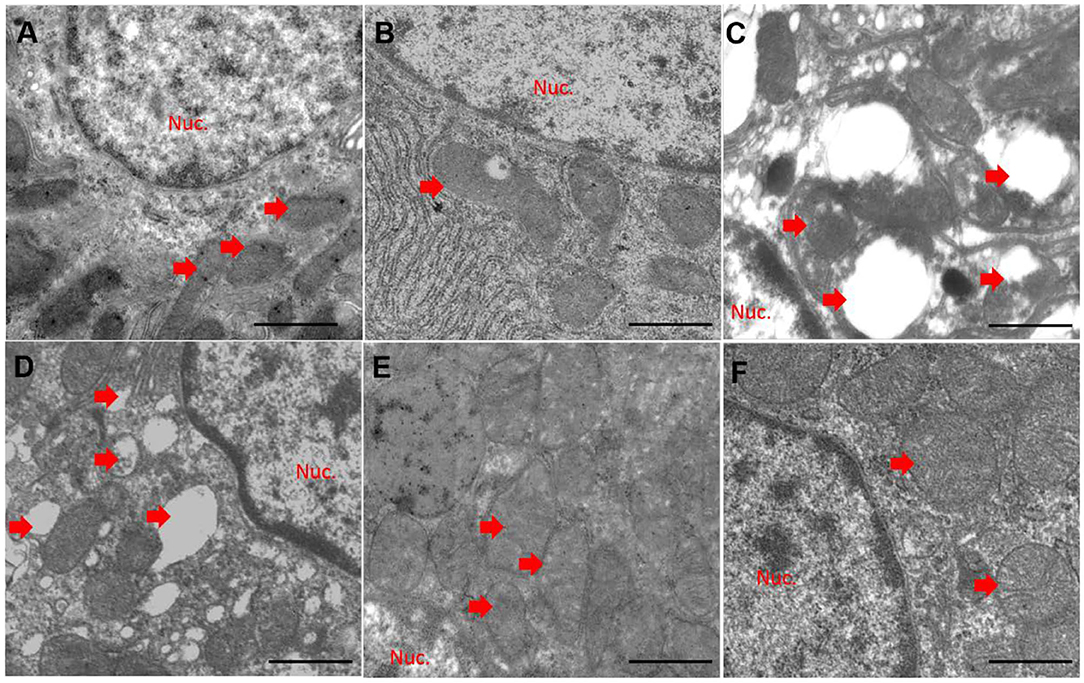
Figure 5. Quercetin supplementation attenuates CuSO4-induced mitochondrial damage in the kidney tissues of mice (n = 3). The representative mitochondrial ultrastructure in the control (A) CuSO4 alone (B) quercetin (QUE100) alone (C) QUE25 + CuSO4 (D) QUE50 + CuSO4 (E) and QUE100 + CuSO4 (F) groups. Red arrows indicated mitochondria. Nuc, nuclear. Bar = 1μm.
Quercetin Supplementation Attenuated CuSO4-induced the Activation of Mitochondrial Apoptosis Pathway in the Kidney Tissues of Mice
When seen against the vehicle control group, CuSO4 exposure significantly increased the activity of caspases-9 and−3 (both P < 0.01). Compared to the CuSO4 alone group, the kidney tissues of mice in the QUE50 + CuSO4 and QUE100 + CuSO4 groups show the activities of caspase-9 fall from 2.9-fold to 2.1-fold and 1.6-fold, respectively and the activities of caspase-3 fall from 3.5-fold to 1.9-fold and 1.7-fold (all P < 0.05 or 0.01), respectively (Figures 6A,B). Quercetin supplementation also decreased CuSO4 exposure-induced increases in the expression of Bax and p53 transcripts. In the QUE50 + CuSO4 and QUE100 + CuSO4 groups, the expression of Bax mRNA decreased from 4.4-fold to 2.8-fold and 2.1-fold, respectively (Figure 6C); the p53 mRNA decreased to from 2.8-fold to 1.8-fold and 1.5-fold, respectively (Figure 6D) (all P < 0.05 or 0.01). Furthermore, compared to CuSO4 alone group, marked attenuation in the levels of Bax and cleaved caspase-3 were detected in the QUE50 + CuSO4 and QUE100 + CuSO4 groups (all P < 0.05 or 0.01) (Figures 6E–G). These apoptotic markers showed no significant effect in the QUE25 + CuSO4 group. Compared to the control, quercetin treatment at 100 mg/kg per day did not significantly affect the activities of caspases-9 and−3, the expression of p53 and Bax mRNAs, and Bax and cleaved caspase-3 proteins (Figures 6A–G).
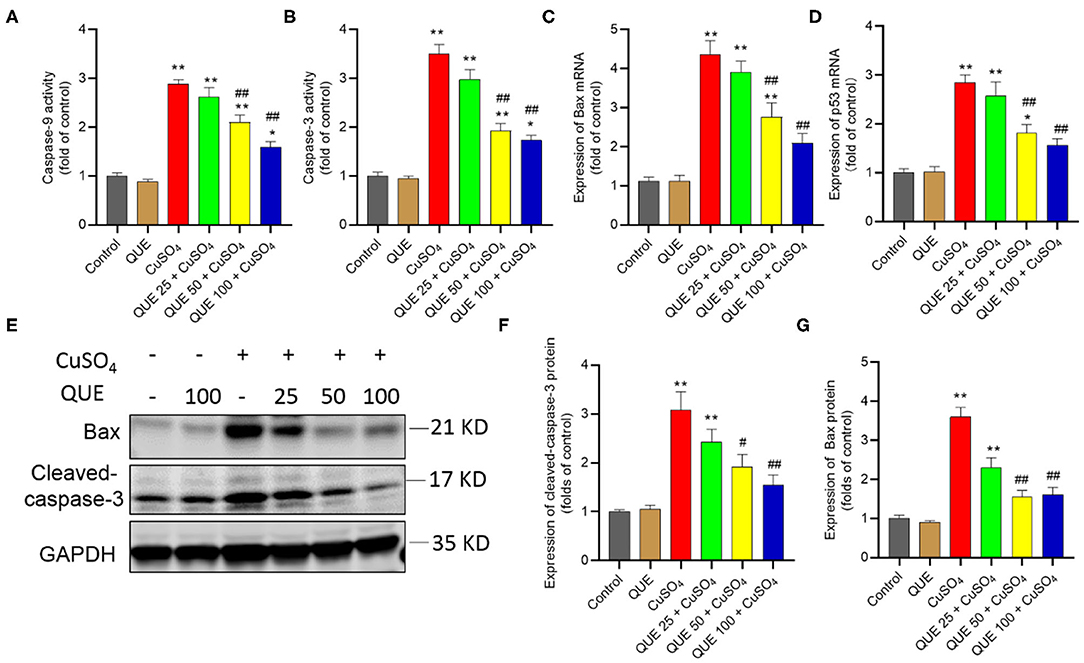
Figure 6. Quercetin supplementation attenuates CuSO4-induced the activities of caspases-9,−3, the expression of Bax and p53 mRNAs, and the expression of cleaved caspase-3 and Bax proteins in the mouse's kidney tissue. (A,B) the activities of caspases-9 and−3, respectively; (C,D) the expression of Bax and p53 mRNAs, respectively; (E–G) Representative expression of cleaved caspase-3 and Bax proteins by western blot (E) and quantification of cleaved-capase-3 (F) and Bax (G). Data are reported as mean ± S.E.M [n = 8 in (A–D) and n = 6 in (F–G)]. *P < 0.05, or **P < 0.01, compared to the untreated control group, respectively; #P < 0.05, or ##P < 0.01, the CuSO4 alone group, respectively. QUE, quercetin.
Quercetin Supplementation Attenuated CuSO4-induced Upregulation in the Levels of IL-1β, TNF-α, and IL-6 Proteins
As shown in Figure 7, CuSO4 exposure significantly induced an inflammatory response in the renal tissue of mice, quantified by an upregulation in the levels of TNF-α, IL-6, and IL-1β compared in the control group. Quercetin supplementation, especially at 50 and 100 mg/kg per day (i.e., in the QUE50 + CuSO4 and QUE100 + CuSO4 groups), markedly attenuated all of these CuSO4 exposure-induced expression of inflammatory biomarkers (P < 0.05 or 0.01) (Figure 7). A slight decrease in the levels of TNF-α, IL-6, and IL-1β were detected in the QUE25 + CuSO4 group, compared to CuSO4 alone group, but it was not significant. The levels of TNF-α, IL-1β, and IL-6 did not appreciably change in the quercetin alone group, compared to that in the control group (Figure 7).
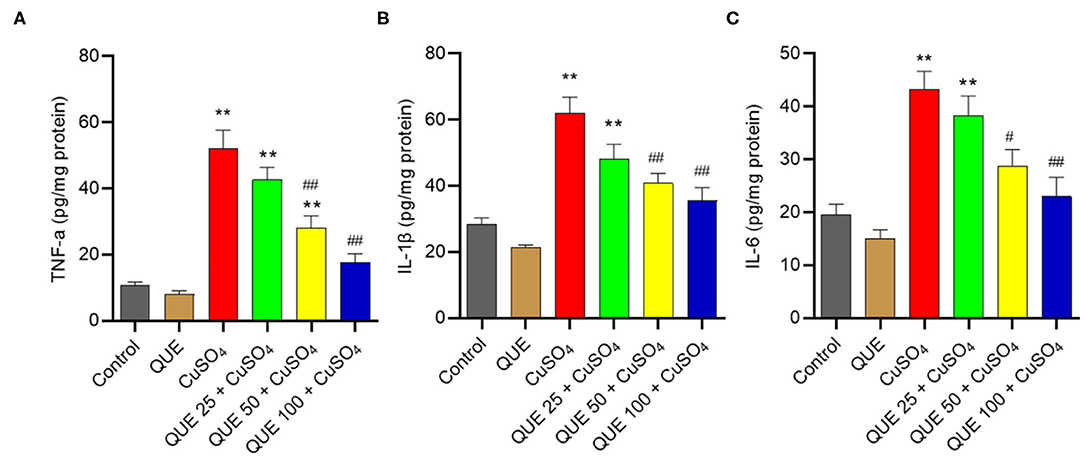
Figure 7. Quercetin supplementation attenuates CuSO4-induced the expression of TNF-α, IL-1β, and IL-6 proteins in the kidney tissues of mice. The levels of TNF-α (A) IL-1β (B) and IL-6 (C) proteins in the kidney tissues of mice were measured by using the ELISA method. Data are reported as mean ± S.E.M (n = 7). **P < 0.01, compared to the control group, respectively; #P < 0.05, or ##P < 0.01, the CuSO4 alone group, respectively. QUE, quercetin.
Quercetin Supplementation Regulated the Gene Expressions in Nrf2 and NF-κB Pathways
CuSO4 exposure significantly up-regulated the expression of Nrf2, HO-1, NF-κB, IL-1β, TNF-α, and IL-6 mRNAs, compared to that in the kidneys of untreated control group. Quercetin supplementation, especially at 100 mg/kg/day (i.e., QUE100 + CuSO4 group), significantly reduced the expression of NF-κB, IL-1β, TNF-α, and IL-6 mRNAs (Figures 8A–D), but increased the expression of Nrf2 and HO-1 mRNAs (all P < 0.05 or 0.01) (Figures 8E,F). In the QUE25 + CuSO4 group, the mRNA levels of TNF-α, decreased significantly, but not Nrf2, HO-1, NF-κB, IL-1β, or IL-6, were decreased, compared to those in the CuSO4 alone group. Quercetin treatment at 100 mg/kg/day alone mildly increased the expression of Nrf2 and HO-1 mRNAs and did not significantly changed the expression of NF-κB, IL-1β, TNF-α, and IL-6 mRNAs, compared to that in the untreated mice.
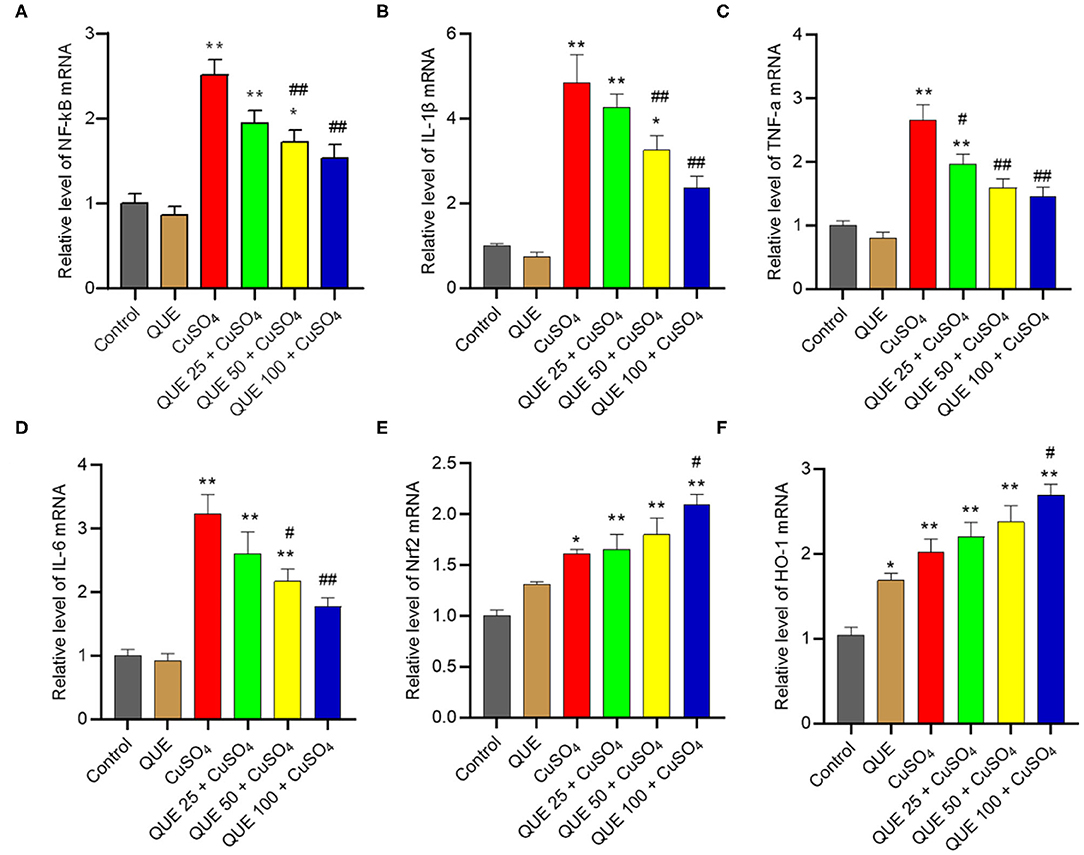
Figure 8. Effect of quercetin supplementation on the expression of NF-κB (A), IL-1β (B), TNF-α (C), IL-6 (D), Nrf2 (E), HO-1 (F) mRNAs in the kidney tissues of mice. Data are reported as mean ± S.E.M (n = 7). *P < 0.05, or **P < 0.01, compared to the control group, respectively; #P < 0.05, or ##P < 0.01, compared to the CuSO4 alone group, respectively. QUE, quercetin.
Discussion
Copper (Cu) can enter into cells though the copper transporter 1 (27, 28). Excessive Cu accumulation in multiple organs (e.g., brain, kidney, heart, liver, and reproductive organs) due to both acute and chronic uptake causes potential toxic effects, including nephrotoxicity, hepatotoxicity, neurotoxicity and reproductive toxicity (4, 10, 11, 29–31). In line with the previous studies (4), our current study showed that CuSO4 exposure at the dose of 200 mg/kg/day for 28 days caused marked nephrotoxicity in mice, observed through the upregulation of serum BUN and CRE levels, as well as histopathological damage (Figures 2, 3). Quercetin is a polyphenolic flavonoid compound found in various vegetables and fruits (32) and it has many documented pharmacologically relevant activities (19, 33, 34). In the present study, our data showed that co-administration of quercetin (at the doses ranging from 25–100 mg/kg/day) significantly attenuated CuSO4 exposure-induced increases of BUN and CRE and histopathological damages in a dose-dependent manner, indicating the nephroprotective effect of quercetin (Figures 2, 3).
The previous studies had demonstrated that oxidative stress might play a critical role in CuSO4-induced nephrotoxicity in vitro in kidney cell lines (i.e., HEK293 cells) and in vivo in a mouse/rat nephrotoxicity model (10, 23). In line with these observations, lower levels of GSH and marked decreases of SOD and CAT activities were detected in the kidney tissues of mice exposed with CuSO4 (Figure 3). Moreover, CuSO4 exposure significantly increased the level of MDA, a lipid peroxidation marker, and nitrative stress-related iNOS activities and NO levels in the kidney tissues of the CuSO4-treated mice (Figure 4). The potent anti-oxidant activity of quercetin is related with its –OH groups on the side phenyl ring (35). Pretreatment with quercetin has been shown to increase the levels of endogenous antioxidant enzymes, including Mn-SOD, Cu/Zn SOD, GSH peroxidase and CAT in hippocampal CA1 neurons of gerbils affected by ischemic injury (36). Similarly, a previous study showed that quercetin supplementation at 40 mg/kg/day for 4 weeks markedly reduced cadmium (Cd)-induced nephrotoxicity in rats by up-regulating the anti-oxidant enzymes activities (e.g., SOD and CAT, glutathione peroxidase) and the levels of vitamin C and vitamin E in the kidneys (24). In a rat model, oral supplementation of quercetin at 50 mg/kg/day for 7 days inhibited gentamicin-induced nephrotoxicity via the inhibition of lipid peroxidation and oxidative stress (25). In another study, quercetin supplementation at 50 mg/kg/day in drinking water for 75 days markedly reduced lead-induced renal oxidative damage in rats (37). In our study, supplementation with quercetin, especially at 50 and 100 mg/kg/day, significantly reduced CuSO4 exposure-induced adverse oxidative/nitrative changes (Figure 4). Taken together, our results add to the growing body of evidence suggesting that quercetin supplementation could protect against nephrotoxicity in mice by inhibiting oxidative stress and nitrative stress.
Copper ions exist as Cu2+ (oxidized) or Cu1+ (reduced) forms in biological systems (38). This process is homeostatic under normal physiological conditions. Cu overdose could disturb this balance and induce the production of excessive ROS, which leads to lipid, protein and DNA damage (39). Previous studies demonstrated that CuSO4 or copper chloride (CuCl2) exposure can induce the production of excessive ROS in HEK293 cells, mouse liver cells or neuronal cells (23, 40, 41). Mitochondria are not only the major producer of cellular ROS but also a target (42). It has been demonstrated that CuSO4 exposure can cause mitochondrial dysfunction in neuroblastoma SH-SY5Y cells, and human hepatoma (HepG2) cells (43, 44). In the present study, CuSO4 exposure caused visible mitochondrial damage, characterized by swollen and ruptured mitochondria and disappearance of cristae. These pathological changes were reduced by quercetin supplementation in a dose-dependent manner (Figure 5). Caspase-9 and Bax are two important biomarkers of the mitochondrial apoptotic pathway (45). Caspase-3 and p53 activation are key biomarkers of apoptosis (39). p53 has the ability to directly or indirectly activate Bax (46). In the present study, CuSO4 exposure significantly upregulated the activities of capases-9 and−3, and the expression of p53, Bax and cleaved-caspase-3 (Figure 6). A previous study also showed that quercetin supplementation significantly reduced the expression of cleaved caspase-3 and apoptosis in the kidney tissues and improved cisplatin nephrotoxicity in mice (47). Recently, Pakrashi et al. (48) also showed the protective effect of quercetin on ROS induced mitochondrial dysfunction in a rotenone-induced apoptotic model. Our study shows that quercetin supplementation decreased the expression of these pro-apoptotic genes and markedly suppressed these adverse indications (Figure 6). The results collectively indicate that CuSO4-exposure induced renal toxicity involved the activation of mitochondrial apoptotic pathway and inhibition of this pathway partially contributed to the nephroprotective effect of quercetin.
Nrf2 is a transcription factor that regulates the expression of anti-oxidant enzymes, including CAT, SOD, and HO-1(49). The Nrf2 pathway is important in the process of cytoprotective adaptive responses to xenobiotic exposure (50). Nrf2 is highly active in tissues or cells susceptible to oxidative stress from exposure to drugs or toxins (50–52). Under normal conditions, the Nrf2 activity is suppressed, as it is sequestered in the cytoplasm by binding to Kelch-like ECH-associated protein 1 (Keap1) (50). It has been reported that CuSO4 exposure can induce the expression of Nrf2 and downstream gene HO-1 in Hela, HEC-1A, HEK293, and A549 cells (16). In the present study, CuSO4 significantly increased the expression of Nrf2 and HO-1 expression in the kidney tissues (Figures 7E,F). Quercetin can directly interact with the binding site of Nrf2 in Keap1 protein and activate the transcriptional activity of Nrf2 (53). Consistently, quercetin supplementation per se further upregulated the expressions of Nrf2 and HO-1 and further promoted their expressions in the kidneys exposed with CuSO4 at 200 mg/kg per day for 28 days. Taken together, the enhanced activation of Nrf2/HO-1 pathway may contribute to the nephroprotective effect of quercetin.
Inflammation plays a critical role in the progression of CuSO4 exposure-induced toxic effects (54–56). In the present study, our results showed that CuSO4 exposure significantly upregulated the expression of IL-1β, TNF-α, and IL-6 proteins and mRNAs in the kidney tissues. Quercetin supplementation significantly inhibited the expression of IL-1β, IL-6, and TNF-αproteins and mRNAs. It thus appears that quercetin supplementation could reduce CuSO4 exposure-induced inflammatory response in the kidney tissues (Figures 7, 8). NF-κB is a master transcriptional mediator that plays a critical role in the cell responding to a diverse set of inflammatory stimuli (49). NF-κB is known to mediate the expression of more than 500 genes, including IL-6, IL-1β, TNF-α, and iNOS (57). Sanchez-Gonzalez and colleagues demonstrated that quercetin supplementation inhibited cisplatin induced renal damage in rats by inhibiting the expression of NF-κB and iNOS protein (47). A study by Liu et. al. demonstrated that quercetin supplementation protected against lead-induced nephrotoxicity in the rat kidney through the inhibition of inflammatory response by downregulating the mitogen-activated protein kinase (MAPKs) and NF-κB pathways. In the present study, our results also showed that CuSO4 exposure significantly upregulated the expression of NF-κB and iNOS activities and NO levels, which were inhibited significantly by quercetin supplementation (Figures 4, 8). Thus, these observations indicate that the inhibitory effect of quercetin on the inflammation caused by CuSO4 was attributed partially to the inhibition of NF-κB/iNOS/NO pathway. In addition, accumulation of ROS could activate NF-κB pathway (58). It is not clear from our study whether the production of ROS caused by CuSO4 exposure contributed to the activation of NF-κB. That would warrant further investigation.
A growing body of evidence indicates that high levels of free copper has a targeted effect on oxidation of hemoglobin, as well as direct damage to the cell membrane, which may contribute to its nephrotoxicity (59). Several studies have reported that quercetin supplementation could inhibit the oxidation of hemoglobin via the Fenton pathway (60) or alleviate the toxic effects of bisphenol A in human red blood cells. It is not clear that whether quercetin supplementation can affect Cu-caused hemolysis effect (61). This area still needs further investigation.
Conclusions
This study revealed that CuSO4 exposure could trigger the activation of p53 pathway, mitochondrial pathway, and oxidative stress and NF-κB mediated-inflammatory response in the kidney tissues, which subsequently lead to significant nephrotoxicity in mice (Figure 9). Quercetin supplementation could effectively attenuate CuSO4-exposure induced nephrotoxicity by reducing oxidative stress, apoptosis and inflammatory responses. Its protective effects involve the inhibition of mitochondrial apoptotic and NF-κB pathways and the activation of Nrf2/HO-1 pathway. Our findings highlight that quercetin may be a promising therapeutic agent against Cu exposure- induced nephrotoxicity.
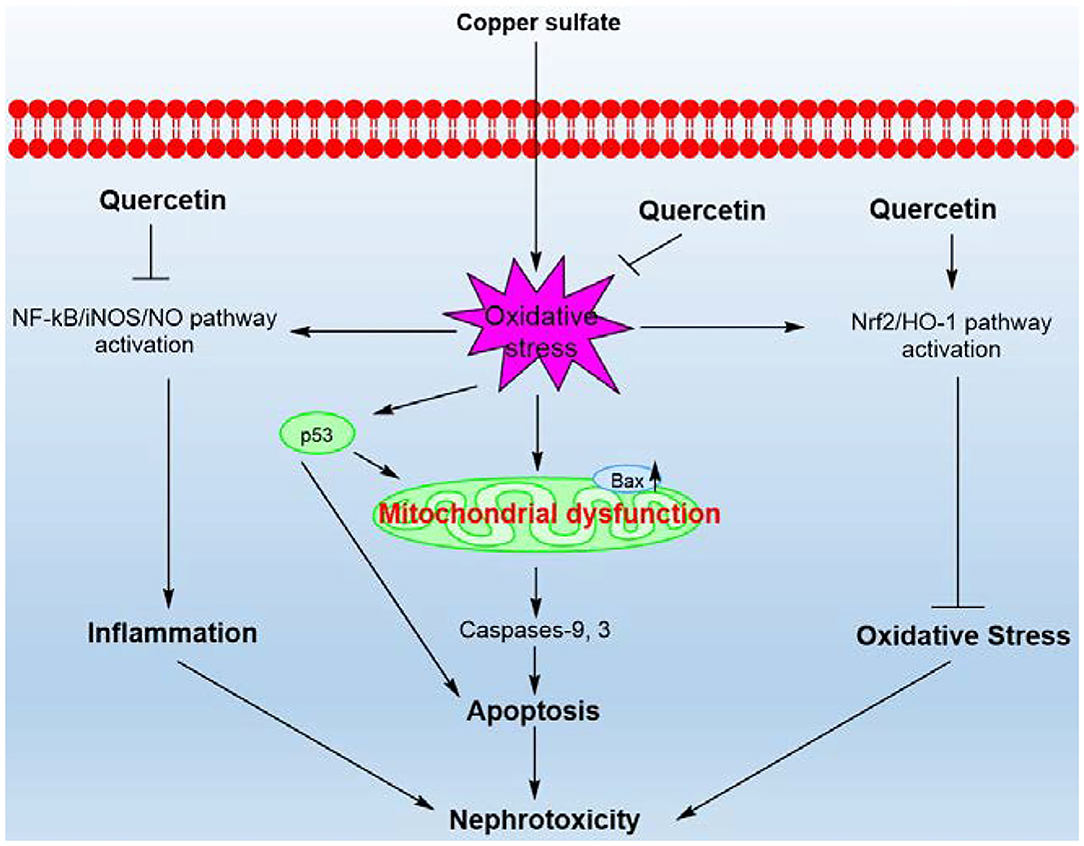
Figure 9. A proposed working model highlighting the protective effects of quercetin on CuSO4-exposure induced nephrotoxicity. CuSO4 exposure triggers oxidative stress, mitochondrial apoptotic pathway, Nrf2/HO-1 pathway and NF-κB/iNOS/NO pathway in the mouse's kidney tissue. CuSO4 exposure also increased the expression of p53, which may contribute to the activation of Bax and exacerbate mitochondrial dysfunction. Quercetin supplementation could inhibit oxidative stress, NF-κB/iNOS/NO and mitochondrial apoptotic pathways and activate the Nrf2/HO-1 pathway, thereby ameliorating CuSO4-induced nephrotoxicity in mice.
Data Availability Statement
The raw data supporting the conclusions of this article will be made available by the authors, without undue reservation.
Ethics Statement
The animal study was reviewed and approved by The Institutional Animal Care and Use Committee at the Ludong University.
Author Contributions
XP and CD: conceptualization, methodology, and original manuscript draft. CD: software. XP, MZ, and CD: formal analysis. XP and MZ: investigation. XP: data curating, acquisition of funding, and project management. XP, SD, MZ, and CD: draft review. All authors have viewed the manuscript and agree to its publication. All authors contributed to the article and approved the submitted version.
Funding
This research was funded by the National Natural Science Foundation of China (No. 32072141), the Natural Science Foundation of Shandong Province (No. ZR2020MC214), the key R&D project in Shandong Province (No. 2019GSF108011), and the key R&D project in Yantai (No. 2018XSCC047).
Conflict of Interest
The authors declare that the research was conducted in the absence of any commercial or financial relationships that could be construed as a potential conflict of interest.
References
1. Kim BE, Nevitt T, Thiele DJ. Mechanisms for copper acquisition, distribution and regulation. Nat Chem Bio. (2008) 4:176–85. doi: 10.1038/nchembio.72
2. Xiong W, Ding X, Zhang Y, Sun Y. Ecotoxicological effects of a veterinary food additive, copper sulphate, on antioxidant enzymes and mRNA expression in earthworms. Environ Toxicol Pharmacol. (2014) 37:134–40. doi: 10.1016/j.etap.2013.11.014
3. Viquez OM, Valentine HL, Amarnath K, Milatovic D, Valentine WM. Copper accumulation and lipid oxidation precede inflammation and myelin lesions in N,N-diethyldithiocarbamate peripheral myelinopathy. Toxicol Appl Pharmacol. (2008) 229:77–85. doi: 10.1016/j.taap.2008.01.005
4. Kumar V, Kalita J, Bora HK, Misra UK. Relationship of antioxidant and oxidative stress markers in different organs following copper toxicity in a rat model. Toxicol Appl Pharmacol. (2016) 293:37–43. doi: 10.1016/j.taap.2016.01.007
5. Lan AP, Chen J, Chai ZF, Hu Y. The neurotoxicity of iron, copper and cobalt in Parkinson's disease through ROS-mediated mechanisms. Biometals. (2016) 29:665–78. doi: 10.1007/s10534-016-9942-4
6. Zhang ZW, Zhi G, Qiao N, Kang ZL, Chen ZL, Hu LM, et al. Copper-induced spermatozoa head malformation is related to oxidative damage to testes in CD-1 mice. Biol Trace Elem Res. (2016) 173:427–32. doi: 10.1007/s12011-016-0675-6
7. Bulcke F, Dringen R, Scheiber IF. Neurotoxicity of copper. Adv Neurobiol. (2017) 18:313–43. doi: 10.1007/978-3-319-60189-2_16
8. Wang Y, Zhao H, Liu J, Shao Y, Li J, Luo L, et al. Copper and arsenic-induced oxidative stress and immune imbalance are associated with activation of heat shock proteins in chicken intestines. Int Immunopharmacol. (2018) 60:64–75. doi: 10.1016/j.intimp.2018.04.038
9. Wu H, Guo H, Liu H, Cui H, Fang J, Zuo Z, et al. Copper sulfate-induced endoplasmic reticulum stress promotes hepatic apoptosis by activating CHOP, JNK and caspase-12 signaling pathways. Ecotoxicol Environ Saf . (2020) 191:110236. doi: 10.1016/j.ecoenv.2020.110236
10. Kumar V, Kalita J, Bora HK, Misra UK. Temporal kinetics of organ damage in copper toxicity: a histopathological correlation in rat model. Regul Toxicol Pharmacol. (2016) 81:372–80. doi: 10.1016/j.yrtph.2016.09.025
11. Smolinski L, Litwin T, Redzia-Ogrodnik B, Dziezyc K, Kurkowska-Jastrzebska I, Czlonkowska A. Brain volume is related to neurological impairment and to copper overload in Wilson's disease. Neurol Sci. (2019) 40:2089–95. doi: 10.1007/s10072-019-03942-z
12. Fuentealba IC, Haywood S, Foster J. Cellular mechanisms of toxicity and tolerance in the copper-loaded rat. III Ultrastructural changes and copper localization in the kidney. Br J Exp Pathol. (1989) 70:543–56.
13. Tchounwou PB, Newsome C, Williams J, Glass K. Copper-induced cytotoxicity and transcriptional activation of stress genes in human liver carcinoma (HepG(2)) cells. Met Ions Biol Med. (2008) 10:285–90.
14. Alharbi B, Fadda L, Ali HM. Evaluation of the renoprotective effect of nano turmeric against toxic dose of copper sulfate: Role of vascular cell adhesion molecule-1, kidney injury molecule-1, and signal transducer and activator of transcription 3 protein expressions. J Biochem Mol Toxicol. (2019) 33:e22243. doi: 10.1002/jbt.22243
15. Zhao H, Wang Y, Fei D, Guo M, Yang X, Mu M, et al. Destruction of redox and mitochondrial dynamics co-contributes to programmed cell death in chicken kidney under arsenite or/and copper (II) exposure. Ecotoxicol Environ Saf . (2019) 179:167–74. doi: 10.1016/j.ecoenv.2019.04.062
16. Chen SY, Liu ST, Lin WR, Lin CK, Huang SM. The mechanisms underlying the cytotoxic effects of copper via differentiated embryonic chondrocyte gene 1. Int J Mol Sci. (2019) 20:5225. doi: 10.3390/ijms20205225
17. Hertog MG, Hollman PC. Potential health effects of the dietary flavonol quercetin. Eur J Clin Nutr. (1996) 50:63–71.
18. Boots AW, Haenen GR, Bast A. Health effects of quercetin: from antioxidant to nutraceutical. Eur J Pharmacol. (2008) 585:325–37. doi: 10.1016/j.ejphar.2008.03.008
19. Batiha GE, Beshbishy AM, Ikram M, Mulla ZS, El-Hack MEA, Taha AE, et al. The pharmacological activity, biochemical properties, and pharmacokinetics of the major natural polyphenolic flavonoid: quercetin. Foods. (2020) 9:374. doi: 10.3390/foods9030374
20. El-Far AH, Lebda MA, Noreldin AE, Atta MS, Elewa YHA, Elfeky M, et al. Quercetin attenuates pancreatic and renal d-galactose-induced aging-related oxidative alterations in rats. Int J Mol Sci. (2020) 21:4348. doi: 10.3390/ijms21124348
21. Gugliandolo E, Peritore AF, D'Amico R, Licata P, Crupi R. Evaluation of neuroprotective effects of quercetin against aflatoxin B1-intoxicated mice. Animals. (2020) 10:898. doi: 10.3390/ani10050898
22. Guo S, Sun J, Zhuang Y. Quercetin alleviates lipopolysaccharide-induced inflammatory responses by up-regulation miR-124 in human renal tubular epithelial cell line HK-2. Biofactors. (2020) 46:402–10. doi: 10.1002/biof.1596
23. Dai C, Liu Q, Daowen L, Sharma G, Xiong J, Xiao X. Molecular insights of copper sulfate exposure-induced nephrotoxicity: involvement of oxidative and endoplasmic reticulum stress pathways. Biomolecules. (2020) 10:E1010. doi: 10.3390/biom10071010
24. Renugadevi J, Prabu SM. Quercetin protects against oxidative stress-related renal dysfunction by cadmium in rats. Exp Toxicol Pathol. (2010) 62:471–81. doi: 10.1016/j.etp.2009.06.006
25. Abdel-Raheem IT Abdel-Ghany AA Mohamed GA. Protective effect of quercetin against gentamicin-induced nephrotoxicity in rats. Biol Pharm Bull. (2009) 32:61–7. doi: 10.1248/bpb.32.61
26. Dai C, Li J, Tang S, Li J, Xiao X. Colistin-induced nephrotoxicity in mice involves the mitochondrial, death receptor, and endoplasmic reticulum pathways. Antimicrob Agents Chemother. (2014) 58:4075–85. doi: 10.1128/AAC.00070-14
27. Prohaska JR. Role of copper transporters in copper homeostasis. Am J Clin Nutr. (2008) 88:826–9. doi: 10.1093/ajcn/88.3.826S
28. Kaplan JH, Maryon EB. How mammalian cells acquire copper: an essential but potentially toxic metal. Biophys J. (2016) 110:7–13. doi: 10.1016/j.bpj.2015.11.025
29. Oldenquist G, Salem M. Parenteral copper sulfate poisoning causing acute renal failure. Nephrol Dial Transplant. (1999) 14:441–3. doi: 10.1093/ndt/14.2.441
30. Bidewell CA, Drew JR, Payne JH, Sayers AR, Higgins RJ, Livesey CT. Case study of copper poisoning in a British dairy herd. Vet Rec. (2012) 170:464. doi: 10.1136/vr.100267
31. Kumar J, Sathua KB, Flora SJS. Chronic copper exposure elicit neurotoxic responses in rat brain: assessment of 8-hydroxy-2-deoxyguanosine activity, oxidative stress and neurobehavioral parameters. Cell Mol Biol. (2019) 65:27–35. doi: 10.14715/cmb/2019.65.1.5
32. Anand David AV, Arulmoli R, Parasuraman S. Overviews of biological importance of quercetin: a bioactive flavonoid. Pharmacogn Rev. (2016) 10:84–9. doi: 10.4103/0973-7847.194044
33. Dabeek WM, Marra MV. Dietary quercetin and kaempferol: bioavailability and potential cardiovascular-related bioactivity in humans. Nutrients. (2019) 11:2288. doi: 10.3390/nu11102288
34. Dicarlo M, Teti G, Verna G, Liso M, Cavalcanti E, Sila A, et al. Quercetin exposure suppresses the inflammatory pathway in intestinal organoids from winnie mice. Int J Mol Sci. (2019) 20:5771. doi: 10.3390/ijms20225771
35. Xu D, Hu MJ, Wang YQ, Cui YL. Antioxidant activities of quercetin and its complexes for medicinal application. Molecules. (2019) 24:1123. doi: 10.3390/molecules24061123
36. Chen BH, Park JH, Ahn JH, Cho JH, Kim IH, Lee JC, et al. Pretreated quercetin protects gerbil hippocampal CA1 pyramidal neurons from transient cerebral ischemic injury by increasing the expression of antioxidant enzymes. Neural Regen Res. (2017) 12:220–7. doi: 10.4103/1673-5374.200805
37. Liu CM, Sun YZ, Sun JM, Ma JQ, Cheng C. Protective role of quercetin against lead-induced inflammatory response in rat kidney through the ROS-mediated MAPKs and NF-kappaB pathway. Biochim Biophys Acta. (2012) 1820:1693–703. doi: 10.1016/j.bbagen.2012.06.011
38. Puchkova LV, Babich PS, Zatulovskaia YA, Ilyechova EY, Di Sole F. Copper metabolism of newborns is adapted to milk ceruloplasmin as a nutritive source of copper: overview of the current data. Nutrients. (2018) 10:1591. doi: 10.3390/nu10111591
39. Dai CS, Zhang DX, Li JC, Li J. Effect of colistin exposure on calcium homeostasis and mitochondria functions in chick cortex neurons. Toxicol Mech Methods. (2013) 23:281–8. doi: 10.3109/15376516.2012.754533
40. Park KA, Yun N, Oh YJ. Anamorsin attenuates cupric chloride-induced dopaminergic neuronal cell death. Biochem Biophys Res Commun. (2019) 520:99–106. doi: 10.1016/j.bbrc.2019.09.089
41. Liu H, Guo H, Jian Z, Cui H, Fang J, Zuo Z, et al. Copper induces oxidative stress and apoptosis in the mouse liver. Oxid Med Cell Longev. (2020) 2020:1359164. doi: 10.1155/2020/1359164
42. Dai C, Xiao X, Sun F, Zhang Y, Hoyer D, Shen J, et al. T-2 toxin neurotoxicity: role of oxidative stress and mitochondrial dysfunction. Arch Toxicol. (2019) 93:3041–56. doi: 10.1007/s00204-019-02577-5
43. Adeyemi JA, Machado ART, Ogunjimi AT, Alberici LC, Antunes LMG, Barbosa F Jr. Cytotoxicity, mutagenicity, oxidative stress and mitochondrial impairment in human hepatoma (HepG2) cells exposed to copper oxide, copper-iron oxide and carbon nanoparticles. Ecotoxicol Environ Saf . (2020) 189:109982. doi: 10.1016/j.ecoenv.2019.109982
44. Goto N, Hara H, Kondo M, Yasuda N, Kamiya T, Okuda K, et al. Hydrogen sulfide increases copper-dependent neurotoxicity via intracellular copper accumulation. Metallomics. (2020) 12:868–75. doi: 10.1039/D0MT00015A
45. Dai CS, Tang SS, Velkov T, Xiao XL. Colistin-induced apoptosis of neuroblastoma-2a cells involves the generation of reactive oxygen species, mitochondrial dysfunction, and autophagy. Mol Neurobiol. (2016) 53:4685–700. doi: 10.1007/s12035-015-9396-7
46. Geng Y, Walls KC, Ghosh AP, Akhtar RS, Klocke BJ, Roth KA. Cytoplasmic p53 and activated bax regulate p53-dependent, transcription-independent neural precursor cell apoptosis. J Histochem Cytochem. (2010) 58:265–75. doi: 10.1369/jhc.2009.954024
47. Sanchez-Gonzalez PD, Lopez-Hernandez FJ, Perez-Barriocanal F, Morales AI, Lopez-Novoa JM. Quercetin reduces cisplatin nephrotoxicity in rats without compromising its anti-tumour activity. Nephrol Dial Transplant. (2011) 26:3484–95. doi: 10.1093/ndt/gfr195
48. Pakrashi T, Taylor JE, Nelson A, Archer DF, Jacot T. The effect of levonorgestrel on fibrinolytic factors in human endometrial endothelial cells. Reprod Sci. (2016) 23:1536–41. doi: 10.1177/1933719116645193
49. Dai C, Ciccotosto GD, Cappai R, Tang S, Li D, Xie S, et al. Curcumin attenuates colistin-induced neurotoxicity in N2a cells via anti-inflammatory activity, suppression of oxidative stress, and apoptosis. Mol Neurobiol. (2018) 55:421–34. doi: 10.1007/s12035-016-0276-6
50. Zoja C, Benigni A, Remuzzi G. The Nrf2 pathway in the progression of renal disease. Nephrol Dial Transplant. (2014) 29:19–24. doi: 10.1093/ndt/gft224
51. Wu J, Liu XH, Fan JJ, Chen WF, Wang J, Zeng YJ, et al. Bardoxolone methyl (BARD) ameliorates aristolochic acid (AA)-induced acute kidney injury through Nrf2 pathway. Toxicology. (2014) 318:22–31. doi: 10.1016/j.tox.2014.01.008
52. Peng XY, Dai CS, Liu QW, Li JK, Qiu JR. Curcumin attenuates on carbon tetrachloride-induced acute liver injury in mice via modulation of the Nrf2/HO-1 and TGF-1/Smad3 pathway. Molecules. (2018) 23:215. doi: 10.3390/molecules23010215
53. Ji LL, Sheng YC, Zheng ZY, Shi L, Wang ZT. The involvement of p62-Keap1-Nrf2 antioxidative signaling pathway and JNK in the protection of natural flavonoid quercetin against hepatotoxicity. Free Rad Biol Med. (2015) 85:12–23. doi: 10.1016/j.freeradbiomed.2015.03.035
54. Pereira TCB, Campos MM, Bogo MR. Copper toxicology, oxidative stress and inflammation using zebrafish as experimental model. J Appl Toxicol. (2016) 36:876–85. doi: 10.1002/jat.3303
55. Liu J, Wang Y, Zhao H, Mu M, Guo M, Nie X, et al. Arsenic (III) or/and copper (II) exposure induce immunotoxicity through trigger oxidative stress, inflammation and immune imbalance in the bursa of chicken. Ecotoxicol Environ Saf . (2020) 190:110127. doi: 10.1016/j.ecoenv.2019.110127
56. Nguyen TH Le HD Kim TNT The HP Nguyen TM Cornet V . Anti-inflammatory and antioxidantproperties of the ethanol extract of clerodendrum Cyrtophyllum turcz in copper sulfate-induced inflammation in zebrafish. Antioxidants. (2020) 9:192. doi: 10.3390/antiox9030192
57. Karin M, Yamamoto Y, Wang QM. The IKK NF-kappa B system: a treasure trove for drug development. Nat Rev Drug Discov. (2004) 3:17–26. doi: 10.1038/nrd1279
58. Morgan MJ, Liu ZG. Crosstalk of reactive oxygen species and NF-kappaB signaling. Cell Res. (2011) 21:103–15. doi: 10.1038/cr.2010.178
59. Bozynski CC, Evans TJ, Kim DY, Johnson GC, Hughes-Hanks JM, Mitchell WJ, et al. Copper toxicosis with hemolysis and hemoglobinuric nephrosis in three adult Boer goats. J Vet Diagn Invest. (2009) 21:395–400. doi: 10.1177/104063870902100319
60. Boadi WY, Johnson D. Effects of low doses of quercetin and genistein on oxidation and carbonylation in hemoglobin and myoglobin. J Diet Suppl. (2014) 11:272–87. doi: 10.3109/19390211.2014.937046
Keywords: copper, oxidative stress, inflammation, kidney, Nrf2 pathway, NF-κB pathway
Citation: Peng X, Dai C, Zhang M and Das Gupta S (2021) Molecular Mechanisms Underlying Protective Role of Quercetin on Copper Sulfate-Induced Nephrotoxicity in Mice. Front. Vet. Sci. 7:586033. doi: 10.3389/fvets.2020.586033
Received: 22 July 2020; Accepted: 19 October 2020;
Published: 08 January 2021.
Edited by:
M. Ramiro Pastorinho, University of Evora, PortugalReviewed by:
Alberto Mantovani, National Institute of Health (ISS), ItalyBenito Soto-Blanco, Federal University of Minas Gerais, Brazil
Maria De Lourdes Pereira, University of Aveiro, Portugal
Copyright © 2021 Peng, Dai, Zhang and Das Gupta. This is an open-access article distributed under the terms of the Creative Commons Attribution License (CC BY). The use, distribution or reproduction in other forums is permitted, provided the original author(s) and the copyright owner(s) are credited and that the original publication in this journal is cited, in accordance with accepted academic practice. No use, distribution or reproduction is permitted which does not comply with these terms.
*Correspondence: Xinyan Peng, cGVuZ3hpbnlhbjIwMDZAbGR1LmVkdS5jbg==