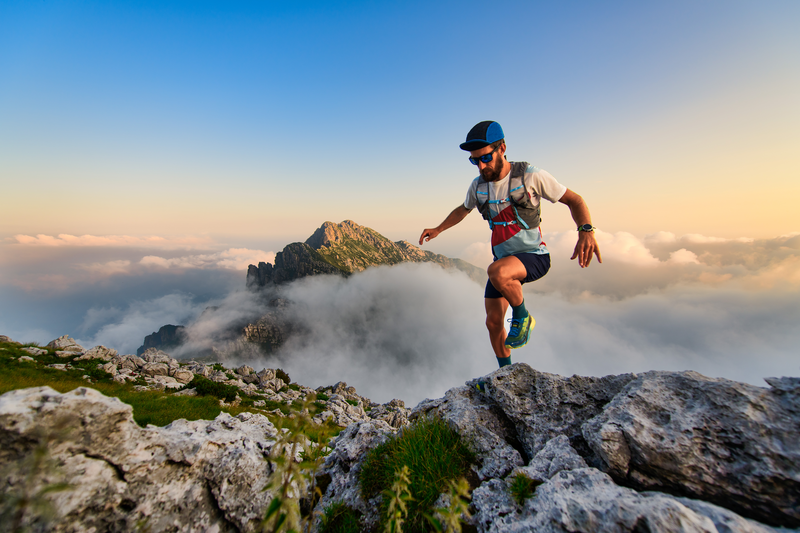
94% of researchers rate our articles as excellent or good
Learn more about the work of our research integrity team to safeguard the quality of each article we publish.
Find out more
ORIGINAL RESEARCH article
Front. Vet. Sci. , 02 December 2020
Sec. Animal Reproduction - Theriogenology
Volume 7 - 2020 | https://doi.org/10.3389/fvets.2020.577775
This article is part of the Research Topic Buffalo Health and Production View all 30 articles
In vitro embryo production and embryo transfer (ET) in buffaloes has been developed for decades. However, most studies are focused on the donor or laboratory improvements, and there is a lack of reports regarding the recipients. Therefore, our aim was to investigate factors associated to pregnancy (P/ET), pregnancy loss (PL), and calving rates in buffalo recipients. The studied factors were season, recipient parity, the synchronization protocol, the CL diameter, asynchrony between the embryo and the recipient, the day of the recipient estrous cycle, the embryo (fresh vs. vitrified), the day of embryo development, and the embryo stage. These retrospective data, from a program of in vitro produced embryos, were analyzed by logistic regression, and the odds ratio was also estimated. Two factors were related to P/ET and the calving rate: (1) progesterone associated to estradiol plus eCG protocol for fixed time ET tended to affect positively P/ET on day 30 (41.9 vs. 36.1%, respectively; P = 0.07; AOR = 1.28) and P/ET on day 60 (37.8 vs. 36.1%, respectively; P = 0.09; AOR = 1.08) compared to the Ovsynch protocol; and (2) the CL diameter (≥14.5 mm) at transfer increased P/ET on day 30 (47.4 vs. 32.5%; P < 0.01; AOR = 1.87) and on day 60 (45.3 vs. 27.7%; P < 0.01; AOR = 2.16), and also the calving rate (37.9 vs. 21.7%; P < 0.01; AOR = 2.20). PL was greater when ET was done in the nonbreeding season compared to the breeding season (PL 30–60: 12.8 vs. 0.0%, P = 0.01; AOR > 999.99; PL 60-calving: 26.8 vs. 3.6%, P = 0.03; AOR = 9.90; and PL 30-calving: 36.2 vs. 3.6%, P = 0.01; AOR = 15.30). In conclusion, the data of our study indicated that the synchronization protocol, the CL diameter, and ET during the breeding season impacted the reproductive efficiency of buffalo recipients.
Brazil is home to 0.67% of the world buffalo herd, with a population of about 1,390,066 (1). Buffaloes have advantages due to their rusticity, adaptability, and the quality of their main products, the milk, and the meat.
Several reproductive biotechnologies, such as superovulation for in vivo embryo production (SOV) or ovum pick-up (OPU) for in vitro embryo production (IVEP), have been used in order to obtain animals with better performance and high genetic merit (2). Unfortunately, the efficiency of superovulation for in vivo embryo transfer (ET) in buffaloes is low when compared to cattle (3). For this reason, the association of OPU and IVEP is currently the most competitive and suitable solution for this purpose, reducing the interval between generations (3, 4).
The strategy of embryo transfer (ET) has enabled genetic progress through maternal lineage and also contributed to the control of infectious diseases (5). Besides the use of fresh embryos, both conventional freezing and vitrification were used for cryopreservation, resulting in pregnancy and calving (6–12), although low pregnancy/embryo transfer [P/ET; 10.5–26.9% (8, 10, 11)] and calving rates [10.0–25% (8, 9, 11, 12)] were generally reported.
In cattle, the recipient plays a critical role in the success of ET programs (13, 14). Several factors are associated with P/ET and calving rates, such as the diameter of corpus luteum (CL), synchronization protocols, season (14), the synchrony between the embryo stage and the recipient, embryo quality, and recipient management (15). However, in buffaloes, only one retrospective study regarding recipients was carried out (16). In that article, asynchrony >12 h and the use of grade I embryos were the factors that enhanced P/ET; the CL diameter also tended to increase P/ET. Since then, few studies were focused on buffalo recipients and, to our knowledge, none were conducted to evaluate multiple factors influencing pregnancy and calving outcomes or pregnancy loss after ET using in vitro produced embryos.
Therefore, the aim of the present study was a retrospective analysis of a dataset obtained from an ET program in order to determine which factors can affect pregnancy, calving rates, and pregnancy loss in buffalo recipients.
The dataset was obtained from 201 ovum pick-up (OPU) sessions and 184 ETs carried out at Cenatte Embriões LTDA, located in the city of Pedro Leopoldo, Minas Gerais, Brazil (latitude: 19°37′12″ south, longitude: 44°2′38″ west).
A total of 73 crossbred Murrah buffalo females (23 nulliparous and 50 multiparous), healthy, nonpregnant, and cycling were used as oocyte donors and embryo recipients. The animals were kept under grazing of Brachiaria decumbens and B. brizantha with free access to water and mineral salt. During the autumn and winter periods, all animals were supplemented with elephant grass silage to meet the demand of each category (17).
All females were used as oocyte donors along the experimental period, and groups of 6–36 animals were submitted to 11 replicates with a minimum interval of 19 days, for 8 months, without any hormonal stimulation.
The animals were contained and submitted to epidural anesthesia, using 3 ml of 2% lidocaine (Lidovet®, Bravet). OPU procedure was performed using transvaginal ultrasound equipment (Aloka 500®, Tokyo, Japan; frequency 5 MHz) coupled to a system with an 18G disposable hypodermic needle (BD) and a 1.7-mm-diameter and 80-cm-long Teflon aspiration line (WTA, Watanabe Applied Technology). The vacuum pressure used was 60–65 mmHg (10 and 12 ml of water/minute). A warm solution (37°C) containing PBS with sodium heparin (5,000 UI/L; Parinex®, Hypolabor), 1% fetal bovine serum (FBS, Nutricell), and 0.1% antibiotic solution (amikacin) was used to collect the aspirated oocytes from follicles larger than 2 mm.
After OPU, cumulus-oocyte complexes (COCs) were searched and classified under a stereomicroscope. TCM 199 with 5% FBS, pyruvate (0.2 mM), and amikacin (83.4 mg/ mL) was used to wash the COCs collected. The criteria used for the evaluation of COC were the presence, the number of layers, and the degree of compaction of Cumulus cells, as well as the appearance of the cytoplasm in color, homogeneity, and integrity. Up to their parameters, the COCs were classified as follows: grade I (three or more layers of cells of the compact cumulus and homogeneous cytoplasm), II (one to three layers of cumulus cells covering totally or partially the oocyte and with homogeneous or heterogeneous cytoplasm), and III (one layer of cumulus cells covering the oocyte totally or partially and with cytoplasm homogeneous or heterogeneous), denuded, degenerated, expanded, and atretic (18). For in vitro embryo production (IVEP), only oocytes grades I, II, and III (viable oocytes) were used.
Viable oocytes were maintained in 1.2 ml cryovials (Corning) containing an oocyte maturation medium, inside an incubator for embryo transport (WTA) at 38.5°C with gas mixture (5.5% CO2, 5.0% O2, and 89.5% N2), until the arrival to the laboratory. The maximum transport time to the laboratory was 6 h after the beginning of OPU.
In the laboratory, groups of 10 to 12 oocytes were transferred to 50-μL drops of a maturation medium, composed of TCM199 with sodium bicarbonate supplemented with 10% FBS, pyruvate (0.2 mM), amikacin (83.4 mg/ mL), 0.5 μg of FSH (Folltropin), 5 μg of LH (Lutropin), 1 μg of 17 α-estradiol, and supplemented with 50 μM of 2-mercaptoethanol, covered with sterile mineral oil. Oocytes were incubated for 20–24 h, at 38.7°C, with 5.5% CO2 in air and saturated humidity.
One hour before the end of the maturation period, the semen from a tested bull was thawed at 35°C for 20 s and deposited on a Percoll gradient (30, 60, and 90%) for sperm selection. Centrifugation was performed at 9,000 × g for 2 min, and, subsequently, the pellet was washed twice in a total alkaline phosphatase medium (TALP), at 4,500 × g for 1 min. The inseminating dose was adjusted to 1.2 million sperm/mL. After IVM, the oocytes were washed in a TCM199 HEPES medium containing 0.3% BSA, pyruvate (0.2 mM), and amikacin (83.4 mg/mL). Then they were washed in a TALP fertilization medium with 0.5% BSA, pyruvate (0.2 mM), amikacin (83.4 mg/ mL), heparin (20 μg/ mL), and PHE solution (2 μM of penicillamine, 1 μM of hypotaurine, and 0.25 μM of epinephrine), and groups of 10–12 oocytes were co-cultured with sperm in 50 μL drops of this same medium, under the same conditions of atmosphere, humidity, and temperature used for IVM for 18–20 h.
After fertilization (D0), the presumptive zygotes were denuded, and groups of 10–12 were transferred to 100 μL drops containing a SOFaa medium with 3% FBS under mineral oil, during 5–7 days at 38.7°C, with 5.5% CO2, 5.0% O2, and 89.5% N2 in saturated humidity. Forty-eight hours after fertilization, the embryo cleavage was evaluated. There was no change of medium throughout the in vitro culture. On 5th to 7th days after fertilization (D5–D7, respectively), the blastocyst rate (blastocysts/viable oocytes) was calculated.
Embryos were morphologically evaluated and classified according to the IETS manual (19), in terms of developmental stage: early blastocyst, blastocyst, expanded blastocyst, and hatched blastocyst; and quality: grade I (excellent or good), II (fair), III (poor), or IV (degenerated).
Only grade I D5 and D6 embryos, varying from early to hatched blastocysts, were transferred. The embryos were loaded in 0.25-mL straws containing a maturation washing medium (TCM 199 HEPES, 5% FBS, pyruvate, and amikacin) and were maintained in the portable incubator until transfer to recipients.
Vitrification was performed when the number of embryos exceeded the number of recipients. Only grade I D5, D6, and D7 embryos, varying from expanded to hatched blastocysts, were vitrified on Cryotop® according to the method described by De Rosa et al. (20). Briefly, the embryos were placed in a solution containing 7.5% ethylene glycol and 7.5% dimethyl sulfoxide (DMSO), prepared in TCM 199 HEPES supplemented with 20% FBS, for 3 min and then were transferred to a second solution containing 16.5% ethylene glycol, 16.5% DMSO prepared in FBS with 0.5 M sucrose. After 25 s, the embryos were kept in a volume of <0.1 μL of vitrification solution and loaded on Cryotop®, which was immediately immersed in liquid nitrogen. The warming procedure was carried out by immersing the top of Cryotop® containing the embryos in a 0.25 M sucrose solution. After 1 min, the embryos were transferred to a 0.15 M sucrose solution for 5 min and then loaded in 0.25-mL straws for transfer to recipients.
All females previously used as oocyte donors were also used as recipients, along 24 months, totalizing 184 procedures divided in 18 replicates (fresh: n = 10; vitrified: n = 8 replicates).
All recipients were submitted to one of the two following synchronization protocols: Ovsynch (21) or estradiol plus progesterone based (22). Briefly, the Ovsynch protocol consisted of 0.5 mg of lecirelin (Gestran Plus®, Tecnopec) on a random day of estrous cycle. Seven days later, 0.5 mg of cloprostenol (Sincrosin®, MSD Animal Health) was administered. A second dose of lecirelin (0.25 mg) was injected 48 h later. The second protocol consisted in the insertion of an intravaginal progesterone device, used for 8 days (multiparous) or 16 days (nulliparous) associated to 2 mg of estradiol benzoate (Estrogin®, Biofarm) and 530 mg of cloprostenol, on a random day of estrous cycle. Nine days later, the devices were removed and 400 IU of eCG (Folligon®, MSD Animal Health) and PGF2α were administered to all recipients. Finally, after 48 h, the recipients were treated with 0.5 mg of lecirelin (Gestran Plus®, Tecnopec).
Six or seven days later, ovulation was evaluated by the presence of a CL, which was measured by ultrasound (Aloka 500®, Tokyo, Japan; frequency 5 MHz), and fresh or vitrified embryos were transferred in the uterine horn ipsilateral to CL, by a nonsurgical method. Only one embryo was transferred in each recipient. Asynchrony between embryos and recipients was ±1 day.
Pregnancies were monitored by ultrasound (Aloka 500, Tokyo, Japan; frequency 5 MHz) 30 and 60 days after the expected ovulation. At the due day, the animals were evaluated for labor stages, dystocia, and placental retention. The newborns were weighed, the sex determined, and suckling reflex and umbilicus were examined.
P/ET was calculated as the percentage of pregnant females, diagnosed on days 30 (P/ET 30) and 60 (P/ET 60), on the total number of the recipients. The calving rate was calculated as the percentage of females calving out on the total number of recipients. PL was calculated as the percentage of nonpregnant females, from days 30 to 60, day 60 to calving, and day 30 to calving, in relation to females pregnant on days 30, 60, and 30, respectively.
Data concerning in vitro embryo production were shown according to descriptive statistics, except by analysis of birth weight associated to sex of calves, which was analyzed by logistic regression, by using the GLIMMIX procedure in SAS for Windows (23). Data regarding recipients were analyzed by univariate and multivariate logistic regression, using the LOGISTIC procedure (23). All groups with fewer than 10 recipients were excluded from the analysis. For pregnancy and calving rate analysis, the following were excluded: asynchrony (−1 day, n = 7), day of embryo development (day 7, n = 7), and embryo stage (hatched blastocyst, n = 9). For pregnancy loss, the following were excluded: asynchrony (−1 day, n = 2), day of embryo development (day 7, n = 2), and embryo stage (early blastocyst, n = 5; blastocyst, n = 8; hatched blastocyst, n = 5). Adjusted odds ratio (AOR) and 95% confidence interval (CI) were generated by logistic regression. Odds ratio is a measure of association between a treatment and an outcome and represents the odds that an outcome will occur given a particular treatment compared to the odds of the outcome occurring in the absence of that treatment or another treatment (24). Results are presented as percentages and AOR. P ≤ 0.05 were considered significant, and those 0.05 ≤ P ≤ 0.10 were considered as tendency.
A total of 998 oocytes were recovered from 201 OPU sessions (5.0 ± 0.5/donor), of which 584 were viable oocytes (2.9 ± 0.3/donor). A total of 318 structures cleaved (1.6 ± 0.2/donor), resulting in a cleavage rate of 54.5%. The blastocyst rate was 44.9% producing 262 embryos (1.3 ± 0.1/donor), of which 229 were grade I, 5 grade II, and 28 grade III.
From the total embryos produced, 114 were transferred fresh resulting in P/ET 30, P/ET 60, and a calving rate of 43.0% (49/114), 41.2% (47/114), and 35.1% (40/114), respectively. Pregnancy loss between days 30 and 60 was 4.1% (2/49), between day 60 and calving was 14.9% (7/47), and between day 30 and calving was 18.4% (9/49). The mean gestation length was 310.2 ± 0.5 days. All calvings were eutocic, and the average weight of calves born was 32.6 ± 0.2 kg, being lower for females (31.4 ± 0.4 kg; n = 20) than for males (33.8 ± 0.6 kg; n = 20; P < 0.01). Concerning postpartum occurrences, two females showed retained placenta (5%), two cervix prolapse (5%), and one rejected her calf (2.5%).
For vitrified embryos, 70 were transferred and P/ET 30, P/ET 60, and the calving rate were, respectively, 37.1% (26/70), 31.4% (22/70), and 24.3% (17/70). Pregnancy loss between days 30 and 60 was 15.4% (4/26), between day 60 and calving was 22.7% (5/22), and between day 30 and calving was 34.6% (9/26). The mean gestation length was 314.5 ± 0.9 days, and one of the animals presented dystocia. The average weight of calves born was 33.8 ± 0.2 kg, being similar in females (33.6 ± 0.7 kg; n = 10) and males (34.0 ± 0.4 kg; n = 7; P = 0.33). In the postpartum period, retained placenta was not observed in any recipient, and one of the calves had suckling difficulty in the first 24 h (5.9 %).
Pregnancy and calving rates were significantly associated to the synchronization protocol and the CL diameter. Reproductive season, parity, asynchrony, day of recipient estrus, type of embryo, and day or stage of embryo development did not affect P/ET 30, P/ET 60, or the calving rate (Table 1).
Table 1. Factors associated to pregnancy (P/ET) on days 30 and 60, and calving rate in buffalo recipients.
When progesterone associated to estradiol plus the eCG-based protocol was compared to Ovsynch, there was a tendency of greater P/ET 30 (41.9 vs. 36.1%; P = 0.07; AOR = 1.28) and P/ET 60 (37.8 vs. 36.1%; P = 0.09; AOR = 1.08).
Recipients with a CL diameter larger than or equal to 14.5 mm had increased P/ET 30 (47.4 vs. 32.5%; P < 0.01; AOR = 1.87), P/ET 60 (45.3 vs. 27.7%; P < 0.01; AOR = 2.16), and a calving rate (37.9 vs. 21.7%; P < 0.01; AOR = 2.20), when compared to those with CL <14.5 mm.
Pregnancy loss (PL) was associated only to the season of the embryo transfer. No other factor influenced PL from days 30 to 60 (PL 30–60), day 60 to calving (PL 60-C), or day 30 to calving (PL 30-C; Table 2).
Table 2. Factors associated to pregnancy loss (PL) from days 30 to 60, day 60 to calving, and day 30 to calving in buffalo recipients.
PL was greater when ET was performed in the nonbreeding season compared to the breeding season (PL 30–60: 12.8 vs. 0.0%, P = 0.01; AOR > 999.99; PL 60-C: 26.8 vs. 3.6%, P = 0.03; AOR = 9.90; and PL 30-C: 36.2 vs. 3.6%, P = 0.01; AOR = 15.30).
The interest in large-scale production of buffalo embryos has been growing worldwide (3). Despite this, embryo recipients are still neglected compared to donors of oocytes and embryos, and few studies were conducted to clarify aspects related to pregnancy and even fewer to clarify aspects related to calving and pregnancy loss. To our knowledge, the present retrospective nonrandomized study is the first to achieve this purpose for buffalo recipients.
In this study, the CL diameter was the main factor that influenced pregnancy until calving. Although controversial, several studies in cattle demonstrated that P/ET was higher when recipients had larger CL, mainly due to an increase in progesterone levels (14). High levels of progesterone allow embryo elongation and increase interferon τ production by the conceptus, avoiding luteolysis in the critical period of maternal recognition of pregnancy (25). In buffaloes, few trials were conducted to evaluate these features. In a study with synchronization of ovulation protocol for fixed time artificial insemination (AI) in buffaloes, a significant and positive correlation between the diameter of dominant follicle at AI and the CL diameter 10 days later, ovulation rate, and pregnancy/AI was found, besides a negative correlation with embryo mortality (22). In another trial (26), cows categorized with a larger diameter of pre-ovulatory follicles on the day of estrus had a positive correlation with the CL diameter and progesterone concentration, and these variables were greater in pregnant than in nonpregnant females. Interestingly, in that work, nonpregnant females had CL diameters close to 15 mm (and progesterone <1 ng/ mL), corroborating Di Palo et al. [1990, revised in (27)] who reported higher fertility in buffaloes with CL ≥ 15 mm. Therefore, the data of the present study support that the CL diameter (≥14.5 mm) can be used as a parameter to select the recipients in buffaloes in order to obtain better outcomes.
Another finding associated to P/ET 30 and P/ET 60 was the synchronization protocol. The Ovsynch protocol has been used in buffaloes to synchronize ovulation for fixed time artificial insemination (21). As previously shown in buffaloes and cattle, the main disadvantage of this protocol is the response to the first GnRH, which can depend on the status of the dominant follicle in the beginning of treatment (28–30). Nevertheless, in anestrus animals, the efficiency of this protocol results in low follicular response and conception rates (21). To overcome this negative effect, treatments based on the association of progesterone and estradiol esters, like in cattle, have been also applied to buffalo reproductive management, with positive pregnancy results independent of animal cyclicity (22). Besides that, in the present study, eCG was associated to P4 plus the E2-based protocol. The beneficial effect of eCG on the maximum diameter of the dominant follicle, the ovulation rate, the CL diameter, CL development, and progesterone production is well-documented in cattle and buffaloes, culminating with higher P/ET (14, 31, 32).
Concerning the PL, our data demonstrated an association between PL and the season when the embryos were transferred. When ET occurred in the nonbreeding season (from October to April), a consistent embryo and fetal mortality was observed along all periods analyzed. A seasonal effect on late embryo mortality (between 26 and 40 days of pregnancy) was previously described by Campanile et al. (33). The authors recorded 45% embryo mortality when the buffaloes were inseminated during periods of increasing daylight. These authors explained this result with a reduced CL capacity to secrete progesterone. Moreover, Vecchio et al. (34) reported high incidence of fetal mortality (between 45 and 90 days of pregnancy) in buffaloes naturally mated in increasing daylight. Overall, these works confirmed our data and indicate that new trials should be performed in order to overcome early and late PL in buffaloes when reproductive strategies are conducted during the nonbreeding season.
Although in the present study progesterone levels were not analyzed, the factors affecting P/ET, the calving rate, and PL herein found collectively suggest that strategies that favor the increase in progesterone levels (synchronization protocols based on progesterone associated to estradiol plus eCG, selection of recipients by the CL diameter, and ET during the breeding season) can improve P/ET until calving and reduce embryo/fetal mortality (Figure 1).
Figure 1. Graphic model of main factors affecting pregnancy per embryo transfer on day 30 (P/ET30), on day 60 (P/ET60), the calving rate (CR), and pregnancy loss from day 30 to 60 (PL 30-60), from day 60 to calving (PL 60-C), and from day 30 to calving (PL 30-C) in buffalo recipients of embryos produced in vitro. (1) Effect of the synchronization protocol on P/ET (P4 vs. Ovsynch), (2) effect of the CL diameter on P/ET (≥14.5 vs. <14.5 mm), and (3) effect of season of year on pregnancy loss (breeding season vs. nonbreeding season).
Regarding the other factors analyzed, parity did not influence the outcomes of buffalo recipients in the present study, in accordance to another work (35) in which P/ET and pregnancy per treated animal did not differ between nulliparous, primiparous, or multiparous buffaloes submitted to a protocol of fixed time ET. This reinforces the optimization of different animal categories in ET programs in buffaloes.
Misra et al. (16) reported that asynchrony ≥ 12 h impaired pregnancy rates in buffalo recipients. Inversely, our data support the possibility of asynchrony between the recipient and the embryo of 1 day (0 and +1) without negative effects on the pregnancy of buffalo recipients, as observed in cattle in which ±24 h did not affect pregnancy rates (36). Associated with this outcome, the day of recipient estrous cycle and the day or stage of embryo development also did not influence our study, in accordance with trials performed in cattle (36, 37) and in buffalo (8, 16). Collectively, the absence of effect of these factors indicates a possible flexibilization in ET schedule allowing the widespread use of this technique in buffalo farms.
In the present study P/ET, the calving rate, or PL was not affected by the type of embryo (fresh vs. vitrified). In cattle, the transfer of fresh embryos is associated to better pregnancy results and lower PL than the transfer of frozen-thawed embryos (36, 37). This can be attributed to injuries caused during cryopreservation. However, in buffaloes, studies comparing these different embryos in contemporary conditions were not found. Nevertheless, our results evidence that vitrification is efficient in buffaloes and can be used to optimize ET programs in this species.
In vitro embryo production outcomes in the current study were, in general, above the average reported in the literature. In previous studies, a low number of total oocytes [1.2 ± 0.07 to 4.1 ± 0.5 (38, 39)], viable oocytes [1.2 ± 0.2 to 1.9 ± 0.3 (38, 40)], blastocyst rate [10–30% (4, 41–43)], P/ET (0–26.9%), and calving rate for fresh [12.5–25.5% (6, 9, 12)] and vitrified embryos [P/ET: 10.5–16.4%, and 10–10.9%, respectively (8, 10, 11)] were reported. These differences can be attributed to several factors, including genetics, nutrition, environment, individual variation in the number of follicles/oocytes, the criterion of oocyte selection, oocyte quality, culture media, use of antioxidants, atmospheric gas, and sire, among others. Corroborating the current study, similar PL [20–33.3% (8, 11)], gestation length [302.3–319.7 days (11, 12)], and weight of calves [27–38.8 kg (8, 10, 12)] were observed. In the present study, no occurrences of large calf syndrome, hydramnio, hydroalantoid, or umbilical cord anomalies were detected. For abnormal postpartum incidence (retained placenta, cervix prolapse, calf rejection, and difficulty of suckling), 12.5 and 5.9% for fresh and vitrified embryos, respectively, were found in the present study. However, no information regarding postpartum problems in buffalo recipients was found in the literature.
Our findings indicate that strategies applied to recipients, as synchronization protocols based on progesterone associated to estradiol plus eCG, selection of recipients by the CL diameter, and ET during breeding season, can improve P/ET and calving rates, reducing PL in buffaloes. However, further prospective studies with a larger number of recipients are necessary to confirm these findings.
The datasets generated for this study are available on request to the corresponding author.
The animal study was conducted according to all ethical care in animal use, according to law number 11,794/ 2008 from Brazilian Constitution (Arouca Law).
WS and MA conceived the study. RM, HB, WS, LG, PB, RD, BG, MR, EB, and RL conducted the experiment. JS analyzed the data. LG wrote the manuscript. PB, BG, RD, MR, and EB critically revised the manuscript. All authors approved the final version of the manuscript.
This work was supported by FAPEMIG (Fundação de Amparo à Pesquisa do Estado de Minas Gerais; Proc APQ-0591-5.04/08).
The authors declare that the research was conducted in the absence of any commercial or financial relationships that could be construed as a potential conflict of interest.
The handling editor declared a past co-authorship with the authors BG and PB.
FAPEMIG (Fundação de Amparo à Pesquisa do Estado de Minas Gerais), Cenatte Embriões LTDA, USP (Universidade de São Paulo), UNINA (Università di Napoli Federico II), and UFMG (Universidade Federal de Minas Gerais).
1. Food Agriculture Organization of United Nations. FAOSTAT. (2018). Available online at: http://www.fao.org/faostat/en/#data/QA (accessed June 01, 2020).
2. Singh B, Chauhan FMS, Singla SK, Gautam SK, Verma V. Reproductive biotechniques in buffaloes (Bubalus bubalis): status, prospects and challenges. Reprod Fertil Dev. (2009) 21:499–510. doi: 10.1071/RD08172
3. Baruselli PS, Carvalho JGS, Elliff FM, Silva JCB, Chello D, Carvalho NAT. Embryo transfer in buffalo (Bubalus bubalis). Theriogenology. (2020) 150:221–8. doi: 10.1016/j.theriogenology.2020.01.037
4. Gasparrini B. In vitro embryo production in buffalo species: state of the art. Theriogenology. (2002) 57:237–56. doi: 10.1016/S0093-691X(01)00669-0
5. Techakumphu M, Sukavong Y, Yienvisavakul V, Buntaracha B, Pharee S, Intaramongkol S, et al. The transfer of fresh and frozen embryos in an elite swamp buffalo herd. J Vet Med Sci. (2001) 63:849–52. doi: 10.1292/jvms.63.849
6. Madan ML, Chauhan MS, Singla SK, Manik RS. Pregnancies established from water buffalo (Bubalus bubalis) blastocysts derived from in vitro matured, in vitro fertilized oocytes and co-cultured with cumulus and oviductal cells. Theriogenology. (1994) 42:591–600. doi: 10.1016/0093-691X(94)90376-T
7. Galli C, Crotti G, Notari C, Turini P, Duchi R, Lazzari G. Embryo production by ovum pick up from live donors. Theriogenology. (200l) 55:1341–57. doi: 10.1016/S0093-691X(01)00486-1
8. Hufana-Duran D, Pedro PB, Venturina HV, Hufana RD, Salazarc AL, Duran PG, et al. Post-warming hatching and birth of live calves following transfer of in vitro-derived vitrified water buffalo (Bubalus bubalis) embryos. Theriogenology. (2004) 61:1429–39. doi: 10.1016/j.theriogenology.2003.08.011
9. Neglia G, Gasparrini B, Di Brienza VC, Di Palo R, Zicarelli L. First pregnancies carried to term after transfer of vitrified buffalo embryos entirely produced in vitro. Vet Res Commun. (2004) 28:233–6. doi: 10.1023/B:VERC.0000045414.65331.6a
10. Sá Filho MF, Carvalho NAT, Gimenes LU, Torres-Junior JRS, Ferreira CR, Perecin F, et al. Prenhezes de embriões bubalinos frescos e vitrificados produzidos in vitro. Acta Sci Vet. (2005) 33:431.
11. Hufana-Duran D, Pedro PB, Venturina HV, Duran PG, Cruz LC. Full-term delivery of river buffalo calves (2n=50) from in vitro-derived vitrified embryos by swamp buffalo recipients (2n=48). Livest Sci. (2007) 107:213–9. doi: 10.1016/j.livsci.2006.09.022
12. Liang X, Zhang X, Yang B, Cheng M, Huang F, Pang C, et al. Pregnancy and calving rates following transfer of in-vitro-produced river and F1 (river × swamp) buffalo (Bubalus bubalis) embryos in recipients on natural oestrus or synchronised for ovulation. Reprod Fertil Dev. (2007) 19:670–6. doi: 10.1071/RD07048
13. Bó GA, Baruselli PS, Moreno D, Cutaia L, Caccia M, Tríbulo R, et al. The control of follicular wave development for self-appointed embryo transfer programs in cattle. Theriogenology. (2002) 57:53–72. doi: 10.1016/S0093-691X(01)00657-4
14. Baruselli PS, Ferreira RM, Sá Filho MF, Nasser LFT, Rodrigues CA, Bó GA. Bovine embryo transfer recipient synchronization and management in tropical environments. Reprod Fertil Dev. (2010) 22:67–74. doi: 10.1071/RD09214
15. Hasler JF. Forty years of embryo transfer in cattle: a review focusing on the journal Theriogenology, the growth of the industry in North America, and personal reminisces. Theriogenology. (2014) 81:152–69. doi: 10.1016/j.theriogenology.2013.09.010
16. Misra AK, Rao MM, Kasiraj R, Reddy NS, Pant HC. Factors affecting pregnancy rate following nonsurgical embryo transfer in buffalo (Bubalus bubalis): a retrospective study. Theriogenology. (1999) 52:1–10. doi: 10.1016/S0093-691X(99)00105-3
17. Nutrient Requirements of Dairy Cattle: Seventh Revised Edition (2001). Available online at: https://www.nap.edu/catalog/dairymodel/ (accessed January, 2010).
18. Leibfried L, First NL. Characterization of bovine follicular oocytes and their ability to mature in vitro. J Anim Sci. (1979) 48:76–86. doi: 10.2527/jas1979.48176x
19. Stringfellow DA, Seidel SM. Manual da Sociedade Internacional de Transferência de Embriões. Illinois: International Embryo Transfer Society Inc. (1998). 180 p.
20. De Rosa A, Attanasio L, Boccia L, Vecchio D, Campanile G, Gasparrini B. Cryotop vitrification for in vitro produced bovine and buffalo (Bubalus bubalis) embryos at different stages of development. Ital J Anim Sci. (2007) 6(Suppl 2):747–50. doi: 10.4081/ijas.2007.s2.747
21. Baruselli PS, Madureira EH, Banarbe VH, Barnabe RC, Berber RCA. Evaluation of synchronization of ovulation for fixed timed insemination in buffalo (Bubalus bubalis). Braz J Vet Res Anim Sci. (2003) 40:431–42. doi: 10.1590/S1413-95962003000600007
22. Monteiro BM, Souza DC, Vasconcellos GSFM, Carvalho NAT, Barusellli PS. Effect of season on dairy buffalo reproductive performance when using P4/E2/eCG-based fixed-time artificial insemination management. Theriogenology. (2018) 119:275–81. doi: 10.1016/j.theriogenology.2018.07.004
24. Szumilas M. Explaining odds ratios [published corrections appears in J Can Acad Child Adolesc Psychiatry. (2015) 24:58]. J Can Acad Child Adolesc Psychiatry. (2010) 19:227–9.
25. Mann GE, Lamming GE. Relationship between maternal endocrine environment, early embryo development and inhibition of the luteolytic mechanism in cows. Reprod. (2001) 121:175–80. doi: 10.1530/reprod/121.1.175
26. Pandey AK, Ghuman SPS, Dhaliwal GS, Honparkhe M, Phogat JB, Kumar S. Effects of preovulatory follicle size on estradiol concentrations, corpus luteum diameter, progesterone concentrations and subsequente pregnancy rate in buffalo cows (Bubalus bubalis). Theriogenology. (2018) 107:57–62. doi: 10.1016/j.theriogenology.2017.10.048
27. Campanile G, Baruselli PS, Neglia G, Vecchio D, Gasparrini B, Gimenes LU, et al. Ovarian function in the buffalo and implications for embryo development and assisted reproduction. Anim Reprod Sci. (2010) 121:1–11. doi: 10.1016/j.anireprosci.2010.03.012
28. Vasconcelos JLM, Silcox RW, Rosa GJM, Pursley JR, Wiltbank MC. Synchronization rate, size of the ovulatory follicle, and pregnancy rate after synchronization of ovulation beginning on different days of the estrous cycle in lactating dairy cows. Theriogenology. (1999) 52:1067–78. doi: 10.1016/S0093-691X(99)00195-8
29. Bisinotto RS, Santos JEP. The use of endocrine treatments to improve pregnancy rates in cattle. Reprod Fertil Dev. (2012) 24:258–66. doi: 10.1071/RD11916
30. Neglia G, Gasparrini B, Salzano A, Vecchio D, De Carlo E, Cimmino R, et al. Relationship between the ovarian follicular response at the start of an Ovsynch-TAI program and pregnancy outcome in the Mediterranean river buffalo. Theriogenology. (2016) 86:2328–33. doi: 10.1016/j.theriogenology.2016.07.027
31. Carvalho NAT, Soares JG, Porto Filho RM, Gimenes LU, Souza DC, Nichi M, et al. Equine chorionic gonadotropin improves the efficacy of a timed artificial insemination protocol in buffalo during the nonbreeding season. Theriogenology. (2013) 79:423–8. doi: 10.1016/j.theriogenology.2012.10.013
32. Rigoglio NN, Fátima LA, Hanassaka JY, Pinto GL, Machado ASD, Gimenes LU, et al. Equine chorionic gonadotropin alters luteal cells morphologic features related to progesterone synthesis. Theriogenology. (2013) 79:673–9. doi: 10.1016/j.theriogenology.2012.11.023
33. Campanile G, Neglia G, Gasparrini B, Galiero G, Prandi A, Di Palo R, et al. Embryonic mortality in buffaloes synchronized and mated by AI during the seasonal decline in reproductive function. Theriogenology. (2005) 63:2334–40. doi: 10.1016/j.theriogenology.2004.10.012
34. Vecchio D, Di Palo R, Zicarelli L, Grassi C, Cammarano A, D'Occhio MJ, et al. Embryonic mortality in buffalo naturally mated. Ital J Anim Sci. (2007) 6:677–9. doi: 10.4081/ijas.2007.s2.677
35. Soares JG, Carvalho NAT, Souza DC, Basso AC, Vieira AN, Untura RM, et al. Effect of animal category on FTET efficiency in buffaloes. Anim Reprod. (2015) 12:628.
36. Hasler JF. Factors affecting frozen and fresh embryo transfer pregnancy rates in cattle. Theriogenology. (2001) 56:1401–15. doi: 10.1016/S0093-691X(01)00643-4
37. Vieira LM, Rodrigues CA, Mendanha MF, Sá Filho MF, Sales JNS, Souza AH, et al. Donor category and seasonal climate associated with embryo production and survival in multiple ovulation and embryo transfer programs in Holstein cattle. Theriogenology. (2014) 82:204–12. doi: 10.1016/j.theriogenology.2014.03.018
38. Manjunatha BM, Ravindra JP, Gupta PSP, Devaraj M, Nandi S. Oocyte recovery by ovum pick up and embryo production in river buffaloes (Bubalus bubalis). Reprod Dom Anim. (2008) 43:477–80. doi: 10.1111/j.1439-0531.2007.00939.x
39. Sá Filho MF, Carvalho NAT, Gimenes LU, Torres-Júnior JR, Nasser LFT, Tonhati H, et al. Effect of recombinant bovine somatotropin (BST) on follicular population and on in vitro buffalo embryo production. Anim Reprod Sci. (2009) 113:51–9. doi: 10.1016/j.anireprosci.2008.06.008
40. Gupta V, Manik RS, Chauhan MS, Singla SK, Akshey YS, Palta P. Repeated ultrasound-guided transvaginal oocyte retrieval from cyclic Murrah buffaloes (Bubalus bubalis): oocyte recovery and quality. Anim Reprod Sci. (2006) 91:89–96. doi: 10.1016/j.anireprosci.2005.01.023
41. Nandi S, Raghu HM, Ravindranatha BM, Chauhan MS. Production of buffalo (Bubalus bubalis) embryos in vitro: premises and promises. Reprod Dom Anim. (2002) 37:65–74. doi: 10.1046/j.1439-0531.2002.00340.x
42. Suresh KP, Nandi S, Mondal S. Factors affecting laboratory production of buffalo embryos: a meta-analysis. Theriogenology. (2009) 72:978–85. doi: 10.1016/j.theriogenology.2009.06.017
43. Gimenes LU, Ferraz ML, Fantinato-Neto P, Chiaratti MR, Mesquita LG, Sá Filho MF, et al. The interval between the emergence of pharmacologically synchronized ovarian follicular waves and ovum pickup does not significantly affect in vitro embryo production in Bos indicus, Bos taurus, and Bubalus bubalis. Theriogenology. (2015) 83:385–93. doi: 10.1016/j.theriogenology.2014.09.030
Keywords: embryo transfer, OPU, IVEP, fresh embryos, vitrification, embryo/fetal mortality
Citation: Saliba WP, Gimenes LU, Drumond RM, Bayão HXS, Di Palo R, Gasparrini B, Rubessa M, Baruselli PS, Sales JNS, Bastianetto E, Leite RC and Alvim MTT (2020) “Which Factors Affect Pregnancy Until Calving and Pregnancy Loss in Buffalo Recipients of in vitro Produced Embryos?” Front. Vet. Sci. 7:577775. doi: 10.3389/fvets.2020.577775
Received: 29 June 2020; Accepted: 10 October 2020;
Published: 02 December 2020.
Edited by:
Domenico Vecchio, Istituto Zooprofilattico Sperimentale del Mezzogiorno, ItalyReviewed by:
Bouchra El Amiri, Institut National de la Recherche Agronomique de Settat, MoroccoCopyright © 2020 Saliba, Gimenes, Drumond, Bayão, Di Palo, Gasparrini, Rubessa, Baruselli, Sales, Bastianetto, Leite and Alvim. This is an open-access article distributed under the terms of the Creative Commons Attribution License (CC BY). The use, distribution or reproduction in other forums is permitted, provided the original author(s) and the copyright owner(s) are credited and that the original publication in this journal is cited, in accordance with accepted academic practice. No use, distribution or reproduction is permitted which does not comply with these terms.
*Correspondence: Lindsay Unno Gimenes, bGluZHNheS5naW1lbmVzQHVuZXNwLmJy
Disclaimer: All claims expressed in this article are solely those of the authors and do not necessarily represent those of their affiliated organizations, or those of the publisher, the editors and the reviewers. Any product that may be evaluated in this article or claim that may be made by its manufacturer is not guaranteed or endorsed by the publisher.
Research integrity at Frontiers
Learn more about the work of our research integrity team to safeguard the quality of each article we publish.