- 1Key Laboratory of Buffalo Genetics, Breeding and Reproduction Technology, Ministry of Agriculture and Guangxi Buffalo Research Institute, Chinese Academy of Agricultural Sciences, Nanning, China
- 2Institute of Animal and Dairy Sciences, University of Agriculture, Faisalabad, Pakistan
This study was aimed to evaluate the effect of mulberry leaf flavonoids (MLF) on oxidative stress, metabolic hormones, and milk production in Murrah buffaloes. Forty multiparous Murrah buffaloes (4 ± 1 lactations) with similar body weight (average 600 ± 50 Kg) and stage of lactation (90 ± 20 d) were randomly selected for this trial. Four treatment groups (10 buffaloes per group) with different doses of MLF included; control (0 g/d), MLF15 (15 g/d), MLF30 (30 g/d), and MLF45 (45 g/d). Buffaloes were fed with total mix ration consisting of grass (Pennisetum purpureum schum), brewery's grain and concentrate mixture for 5 weeks. Meteorological data including ambient temperature and relative humidity were recorded using the online dust monitoring system to calculate temperature-humidity index (THI). After 1 week of the adaptation, milk yield was recorded daily while physiological parameters (respiratory rate, rectal, and body surface temperature), and milk composition were measured weekly. At the end of the trial, blood samples were collected to analyze serum metabolic hormones including estradiol (E2), growth hormone (GH), prolactin (PRL), Tri-iodothyronine (T3), and Thyroxine (T4). Moreover, serum heat shock proteins (HSP), antioxidants enzymes including malondialdehyde (MDA), total antioxidant capacity (T-AOC), superoxide dismutase (SOD), catalase (CAT), and glutathione peroxidase (GSH-Px) and blood biochemical indices were also analyzed. Results revealed a decrease (P = 0.012) in serum MDA level while increasing (P < 0.01) the HSP and serum GHS-Px contents in supplemented buffaloes. Treatment showed a linear and quadratic decrease (p = 0.001) in the serum T-AOC while reducing CAT contents linearly (p = 0.012) as compared to the control. However, no effect of treatment on serum SOD content was observed. Treatment resulted a linear increase (p = 0.001) in serum GH and PRL hormones while increasing serum E2 levels linearly (P < 0.001) and quadratically (P = 0.025). Treatment increased (p = 0.038) the daily milk yield as compared to the control. However, increase (P < 0.05) in serum T3 and T4 contents, fat corrected milk (4%) and milk protein (%) was observed only in MLF45. Moreover, we observed no change in serum biochemical indices except insulin which linearly increased (p = 0.002) in MLF45. Our findings indicated that MLF at 45 g per day is an appropriate level to enhance milk performance and alleviate heat stress in buffaloes.
Introduction
Flavonoids belong to a diverse group of plant polyphenols that are widely distributed in different plant species and possess a wide range of biological and pharmacological activities. Most of the plant species used as animal fodders are rich sources of flavonoids along with other polyphenolic contents (1). Due to excellent biological properties, flavonoids are considered efficient feed supplements for livestock to enhance performance (growth and development) and quality of animal products (2). Flavonoids from plant species of genus Morus (commonly known as mulberry) which belongs to the family Moraceae are famous for their antioxidant potential. The genus Morus contains about 68 species and the majority of them are cultivated in Asia (3). China has the largest area of mulberry cultivation (626,000 ha) followed by India (with nearly 280,000 ha). Several other countries [e.g., Thailand and Brazil (ca. 35,000 ha)] still have some mulberry production but on a much smaller scale (4). Owing to its easy propagation and excellent growth characteristics, it can yield leaf biomass of about 25–30 tones/ha/year with a quite less harvesting interval of about 9 to 10 weeks (5). Mulberry leaves contain rich protein content (14–30%) with high in vivo dry matter digestibility (75–85%) coupled with a quite luring palatability due to their succulent nature (6). Traditionally, mulberry foliage has been used as alternate forage for livestock in China, mainly due to their rich nutrient profile and flavonoid contents (7). Feeding of mulberry leaves has shown to increase fiber degradation and utilization leading to enhanced milk production in ruminants (8). Moreover, mulberry leaves have shown potent antioxidant and anti-inflammatory properties owing to their rich flavonoid contents (9).
Mulberry leaf flavonoids (MLF) are of great importance due to their excellent antioxidant, biological, and pharmacological activities (10, 11). Major flavonoids in mulberry leaf include isoquercitrin, astragalin, kaempferol, quercetin, and rutin (12). Recent studies have revealed the potential of mulberry-derived flavonoids to effectively improve or sustain animal performance and health (13, 14). The most promising activities of flavonoids in addition to antioxidants; are their potential to modulate different metabolic pathways especially those linked with energy homeostasis in the body. Due to structural homology with estrogenic hormones, flavonoids exhibit similar anabolic functions through modulation of key lipid and carbohydrate metabolic pathways (15, 16). Mulberry leaf extract has shown to upregulate the activities of glycolytic enzymes while downregulating gluconeogenic enzymes through modulation of gene and transcriptional factors involved in glucose homeostasis in the liver of mice (17). Studies have also shown that hydro-ethanolic extract of mulberry leaves significantly reduced the level of lipid peroxidation and adipocyte size in the liver. Moreover, combined mulberry leaf and fruit extract significantly reduced the obesity-related inflammation and oxidative stress in the high-fat diet obesity model (18).
Flavonoids also possess a remarkable ability to enhance non-specific immunity while reducing oxidative stress by promoting the activities of superoxide dismutase (SOD) and glutathione peroxidase (GSH-Px) while lowering the MDA level (19). This activity of flavonoids is mainly attributed to their action as a reducing agent and a hydrogen donor to scavenge reactive oxygen species (ROS) efficiently by removing the peroxide and superoxide radicals (19). Supplementation of MLF significantly improved overall antioxidant capacity while reducing the oxidative stress in E. coli challenged pre-weaning calves (20).
Mulberry leaf flavonoids have been extensively studied in mice and monogastric animal models, but studies on ruminants especially dairy animals, are limited. The MLF have also shown to increase the apparent digestibility of organic matter (OM) and neutral detergent fiber (NDF), while reducing methane emission in sheep (7). We hypothesized that owing to their effective antioxidant activity, MLF can effectively reduce the oxidative stress posed by the hot and humid climate of southern China. Moreover, their homology with anabolic steroids can enhance the secretion of metabolic hormones which can subsequently affect lactogenesis (21). The alleviation of oxidative stress coupled with increased secretion of metabolic hormones can synergistically lead to desirable effects on antioxidant status, nutrient metabolism, and milk production performance of buffaloes. Therefore, this study was designed to evaluate the effect of MLF supplementation on antioxidant parameters, metabolic hormones, and milk production of buffaloes under the hot and humid climate of South China.
Materials and Methods
Materials
Extract of mulberry (Morus alba) leaves (flavonoids 5%) was purchased from Xi'an Feida Biotechnology Co. Ltd., Xi'an, China. The commercial MLF extract mainly consisted of flavones (65.0%), flavonols (20.0%), and other polyphenols (15.0%).
Geographical Location and Environmental Conditions
This study was conducted from June to July 2019 at the Guangxi Buffalo Research Institute, located in Nanning, South China (N 22° 53′22.59″N, E 108°21′51.19″E; 122 meters above sea level). We used an online dust monitoring system (Shenzhen Greenford Environmental Technology Co., Ltd.) to record weather data in real-time, mainly including air temperature (AT, °C) and relative humidity (RH, %), with an interval of 30 min, at an installation height buffalo's body. Environmental variables were recorded twice daily in the morning (at 8.00 A.M) and afternoon (at 2.30 P.M) during the study period (Figure 1). Average daily temperature and relative humidity were used to calculate Temperature Humidity Index (THI) using the following formula proposed by Thom (22);
Where AT is the air temperature and DPT is the dew point temperature of the buffalo shed.
Animals, Diets, and Experimental Design
All procedures used in this experiment were approved by the Ethics committee of the Chinese Academy of Agriculture Sciences, Guangxi Buffalo Research Institute, China. Forty multiparous Murrah buffaloes (4 ± 1 lactations) with an average body weight of 600 ± 50 Kg with the almost same stage of lactation (90 ± 20 days) were randomly selected for this trial. Three levels of MLF were compared in a complete randomized design to evaluate their effect on serum metabolites, stress proteins, antioxidant parameters, and milk production performance of Murrah buffaloes. Four treatment groups (10 buffaloes per group) with different doses of MLF included; MLF15 (15 g/d/head), MLF30 (30 g/d/head), MLF45 (40 g/d/head), and control group (0 g/d/head). Buffaloes were fed with total mix ration (TMR) consisting of grass (Pennisetum purpureum schum), brewery's grain and concentrate mixture for 5 weeks. The analysis of crude protein in TMR was performed according to AOAC procedures (23). The TMR samples were also analyzed for neutral detergent fiber (NDF) and acid detergent fiber (ADF) as described previously (24) using an ANKOM2000 Fiber Analyzer Unit (ANKOM Technology Corp., Macedon, NY, USA). Neutral detergent fiber content was analyzed with heat-stable α-amylase and sodium sulfite per sample in the neutral detergent solution. The NDF and ADF were expressed inclusive of residual ash. The gross energy (GE) of rations was determined by a bomb calorimeter (PARR Calorimeter, USA). Details of the chemical composition of the experimental diet are given in Table 1.
Animal Management and Recording of Physiological Parameters
All animals were managed under similar housing and management conditions. Buffaloes were housed in open-sided buffalo shed during milking time, where they were fed once in the morning and afternoon. For exercise, buffaloes were set free in an adjacent open area with a stocking density of 15 m2/head. Free access to water was provided to all buffaloes throughout the day. Fans were installed in the buffalo shed above the animal's height to improve airflow. Buffaloes were allowed 30 min swimming time before milking (Once in each morning and afternoon). All animals were fed with a measured quantity of feed twice daily in the morning and evening before milking for ad libitum intake. Nutrient requirement of buffalo was calculated according to the technical standards for feeding of water buffalo in China, issued by Hunan Provincial Quality and Technical Supervision Bureau (25). It is based on the bodyweight of animals and the average quantity of milk produced to meet the nutrient requirements of animals. Feed intake of each buffalo was measured individually by collecting and measuring residual feed daily for consecutive 7 days during the last week of the trial.
Weekly average body surface temperature (BST) was recorded on each Tuesday at 8:00 A.M and 2:30 P.M using an animal infrared thermometer from three different body sites (forehead, left chest, and abdomen). At the same time, rectal temperature (RT) was also recorded using a veterinary rectal thermometer (by inserting in the rectum for 15 s) while respiratory rate (RR) was recorded as times/min by observing thoracic movements using a stopwatch and a counter (for 2 min).
Milk Yield and Composition
Each buffalo was milked twice with milking machines and daily milk yield was recorded for all groups throughout the experimental period. The first week was given as an adaptation period to animals, after that milk yield for morning and evening was recorded daily for each buffalo, while milk samples for determination of milk composition were collected weekly. Milk composition (Milk total solids, protein, fat, and lactose) was analyzed immediately after milking for morning and evening separately using MilkoScanTM F120 (FOSS, Hillerød, Denmark). Fat corrected milk (FCM) at 4% was calculated by using the following equation (26);
Blood Sampling and Haemtological Analysis
Blood samples were collected on the last day of the trial before morning feeding. Blood samples were taken in 10 ml evacuated tubes from the jugular vein to analyze hematological parameters. Two blood samples were collected from each buffalo; first blood was collected in a plain vacutainer tube (10 ml plain vacuum tubes) for obtaining serum, while the second blood sample was collected in a vacutainer tube containing EDTA as the anticoagulant for hematological analysis. Later on, samples were prepared (blood serum) or analyzed (whole blood samples) in the laboratory directly after receiving them. Blood samples in plain tubes were centrifuged at 3,000 rpm for 15 min, and serum was harvested according to standard methods (27). Whole blood samples were used for further analysis of hematological profile including total protein (TP), albumin (ALB), globulins (GLOB), blood urea nitrogen (BUN), and glucose (Glu) using commercial kits according to manufacturer's instructions.
Determination of Serum Heat Shock Proteins (HSP), Hormones, and Antioxidant Enzymes
Blood samples were put on ice after collection and immediately transferred to the laboratory for separation of serum. Collected serum was stored at −20°C until further analysis. Expression of serum stress proteins (HSP70 and 90) was determined using ELISA Kits. Moreover, metabolic hormones, including Estradiol (E2), Prolactin (PRL), growth hormone (GH), Triiodothyronine (T3), and Thyroxine (T4) were analyzed using ELISA Kits provided by CUSABIO BIOTECH CO., Wuhan, (China) through ELISA assay (ELISA microplate reader) according to manufacturer's instructions. Level of serum antioxidant enzymes including, T-AOC (A015), MDA (A003-1), T-SOD (A001-1), GSH-Px(A005), and CAT (A007-1-1) was determined through spectrophotometer using the Nanjing Built-in Kits (www.njjcbio.com) according to manufacturer's instructions. Average values for each treatment group are expressed as Mean ± S.E. The coefficient of variation (inter and intra-assay) of these kits was in the same range (<10%).
Statistical Analysis
Data were subjected to the one-way analysis of variance (ANOVA) in SPSS software (SPSS, 2014). Data were analyzed as a complete randomized design using the following statistical model;
where X is the overall mean; Ti is the fixed effect of treatment (i = control, MLF15, MLF30, and MLF45); Aj is the random effect of the animal and eij is the random error term.
Treatment means were compared using Duncan's multiple range test. Moreover, polynomial contrasts (linear and quadratic) were measured to determine the dose-dependent response of MLF supplementation. Significant effects of the treatment were declared at p < 0.05.
Results
Meteorological Data
Results revealed that the average ambient temperature was 30.73°C, with a range of 27.5–35°C. Relative humidity ranged from 59 to 88 % with an average of 77%. The temperature-humidity index showed a variation from a minimum value of 78 to a maximum value of 87 with, an average value of 82 during 5 weeks of the study period (Figure 1).
Effect of Treatment on Physiological and Serum Biochemical Parameters
Results revealed no significant effect of supplementation of MLF on physiological parameters, including body surface temperate and respiratory rate (Table 2). However, significantly higher rectal temperature was observed in supplemented groups (MLF15 and 45) as compared to the control group (38.47 to 38.50 vs. 38.31). Our study revealed no effect of treatment on serum biochemical parameters including TP, ALB, GLB, BUN, and glucose in lactating buffaloes (Table 2). However, MLF45 linearly increased (p = 0.002) the insulin level as compared to the control.
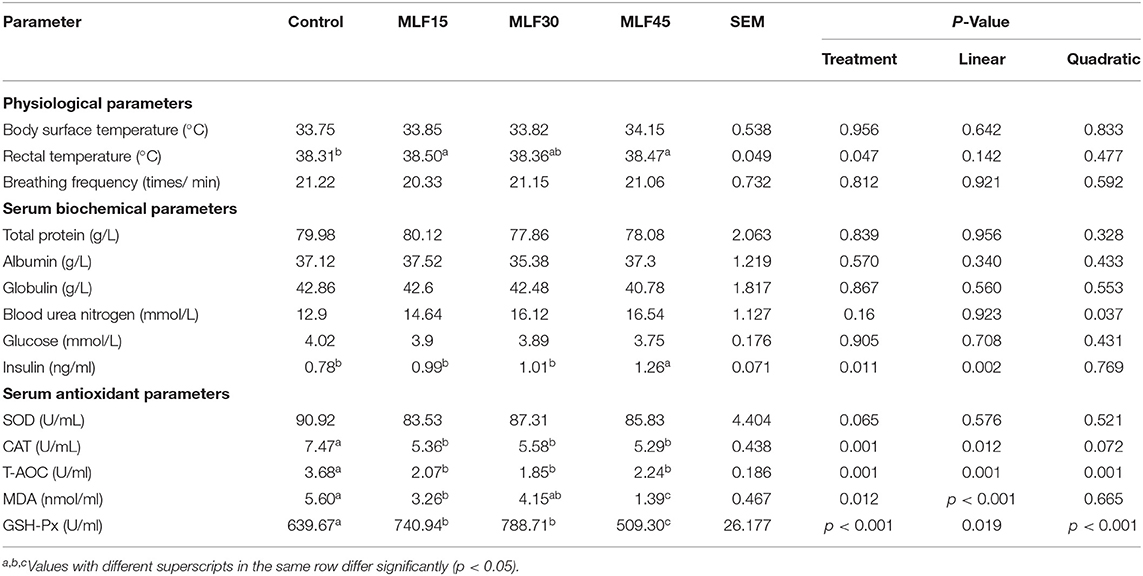
Table 2. Effect of MLF on the physiological, serum biochemical, and antioxidant parameters of buffalo.
Effect of MLF on Serum Antioxidant Parameters
Supplementation of MLF revealed no effect on serum SOD levels in lactating buffaloes as compared to the control group (Table 2). Treatment showed a linear and quadratic decrease (p = 0.001) in the serum T-AOC while reducing CAT contents linearly (p = 0.012) as compared to the control. Moreover, a linear decrease (p < 0.001) in the serum MDA levels was observed only with lower (41 %) and high (75%) levels of MLF as compared to control. The decrease in MDA levels with a medium level of MLF supplementation was not significant as compared to control. However, serum GSH-Px linearly increased (p = 0.019) with the first two levels of MLF (15 and 30 g/d) as compared to the control. But, the higher level of MLF (45 g/d) resulted in a quadratic decrease (p < 0.001) in serum GSH-Px content as compared to control and other treatment groups.
Effects of MLF on Heat Shock Proteins and Serum Metabolic Hormones
The treatment significantly affected the expression of serum heat shock proteins in buffaloes (Table 3). The level of HSP90 linearly increased (p = 0.001) with treatment as compared to the control but the higher level of MLF (45 g/d) exhibited a more pronounced effect on HSP90 levels. However, only MLF45 linearly increased (p = 0.04) the expression of HSP70 as compared to the control and other treatment groups. Analysis of metabolic hormones showed a linear increase (p < 0.001) in serum estradiol (E2) levels with MLF30 and MLF45 as compared to the other treatment groups. However, a linear increase (p < 0.001) in the serum prolactin levels was observed in response to MLF treatment. Similarly, growth hormone also showed a linear increase (p = 0.001) in response to MLF supplementation. The first two levels of MLF (15 and 30 g/d) exhibited no effect on serum T3 and T4 levels as compared to the control group. However, the higher level of MLF (45 g/d) increased the T3 (p = 0.003) and T4 (both linearly and quadratically, p < 0.05) levels as compared to other treatment groups. The MLF45 showed a more pronounced effect on T4 levels as compared to T3 (40 vs. 25% increase as compared to the control group).
Effect of MLF on Milk Yield and Composition
Results revealed a significant effect of treatment on different milk yield and composition parameters except milk lactose (Table 4). The higher level of MLF increased (p = 0.038) the daily milk yield of buffaloes as compared to the control group. A comparison amongst different levels of MLF revealed a similar increase in daily milk yield by the first two levels (MLF15 and MLF30) as compared to the control group (p < 0.05). However, the higher level of MLF (MLF45) showed a more obvious increase in milk yield as compared to low and medium levels. The MLF45 linearly (p = 0.004) and quadratically (p = 0.009) increased the FCM (4%) as compared to other treatment groups. The MLF45 also increased (p = 0.02) the milk fat (%) as compared to MLF15 but this increase together with a decrease observed in response to MLF15 and MLF30 was not significant as compared to the control. Low and medium levels of MLF showed no effect on milk protein (%) but, the high level (MLF45) increased (p = 0.024) the milk protein (%) as compared to the control. Milk total solids (%) decreased (p = 0.035) in MLF15 as compared to MLF45 and control groups.
Discussion
Physiological and Serum Biochemical Parameters
Flavonoids have shown to increase the metabolic rate by activation of brown adipose tissue, leading to thermogenesis and enhanced energy utilization. Mulberry leaf flavonoids can mediate the transcriptional signaling of different genes related to fat metabolism (28). An increase in rectal temperature in MLF15 and 45 groups indicate the effect of mulberry flavonoids on energy homeostasis in buffaloes, which is in agreement with earlier studies (29). Studies have reported that supplementation of mulberry leaf increased oxygen consumption and activity of obese mice (29). Moreover, flavonoid contents of mulberry leaf activated the brown adipose tissue (which are rich in mitochondria) through the mediation of up-regulated uncoupling protein 1 (UCP1), leading to release dietary energy as body heat (30). This heat increment may increase rectal temperature and body surface temperature in animals (29). This fact might have attributed to increased rectal temperature observed in the present study. Furthermore, activation of adipose tissues can enhance thermogenesis and energy expenditure leading to improved glucose homeostasis and insulin sensitivity (31, 32). Moreover, MLF have shown to trigger the AMPK-PGC-1α signaling pathway to improve glucose metabolism and enhance mitochondrial function to restore ATP homeostasis in skeletal muscles (33). No effect of MLF on respiratory rate and body surface temperature shows the absence of any adverse effects of MLF on the physiology of buffaloes.
No change in serum biochemical parameters was observed in the present study except insulin levels. The non-significant effect of treatment on blood metabolites is in agreement with earlier studies on beef steers fed with ensiled mulberry leaves and sun-dried mulberry pomace (34). These findings reveal that treatment with MLF showed no adverse effects on liver metabolism in buffaloes, as indicated by no change in serum metabolites. It also implies that the level of MLF used in this study is safe for lactating buffaloes as it did not show any adverse effects on downstream metabolic and physiological functions. Moreover, an increase in the serum insulin level in response to MLF45 is in agreement with earlier studies that reported enhanced insulin secretion and sensitivity in response to intra-duodenal supplementation of quercetin (a major flavonoid present in mulberry leaves) in dairy cows (35). Moreover, quercetin has also shown to increase the number of pancreatic islets, which are responsible for insulin secretion (36). In addition, quercetin enhanced the insulin release in isolated islets of Langerhans in vitro in a mice model (37). These findings clearly indicate that MLF can positively affect glucose metabolism and could be beneficial in regard to the metabolic adaption of high-producing dairy animals to early lactation. Additionally, optimum insulin levels and proper action are required to effectively alleviate heat stress and minimize oxidative stress damage (38, 39). Overall our findings revealed that MLF supplementation can increase insulin secretion which can subsequently improve the heat tolerance and milk performance of water buffaloes during heat stress.
Serum Antioxidant Parameters
Under normal environmental conditions (thermoneutral zone), a balance exists in the oxidant-antioxidant system to maintain body homeostasis. However, exposure to higher ambient temperature disrupts this delicate balance leading to oxidative stress. Heat stress exposes animals to an excessive load of ROS leading to severe oxidative stress that subsequently increases lipid peroxidation and reduces immune response (40, 41). Therefore, it is crucial to maintain an oxidant-antioxidant balance to avoid the adverse effects of oxidative stress. In dairy animals, especially in lactating buffaloes, the effect of this oxidative stress is more pronounced as milk production is also a metabolic activity that generates heat. The MDA is an end product of lipid peroxidation which is commonly used as a biomarker to indicate the degree of oxidative stress and level of free radicals (42). Under oxidative stress conditions, the defense system of a body fails to scavenge a large number of free radicals in time due to reduced activities of antioxidant enzymes (CAT, SOD, GSH-Px) under chronic heat stress (43). Therefore, levels of MDA and antioxidant enzymes in ruminants are commonly used as physiological indicators to determine the degree of oxidative and heat stress (44).
In the present study, the THI value exceeded 80 during the study period which resulted in heat stress in lactating buffaloes as revealed by higher MDA levels in the control group. However, MLF significantly reduced MDA levels in treated buffaloes revealing its potent ability to alleviate oxidative stress. Remarkable decrease in MDA levels up to 75% as compared to the control indicated the useful potential of MLF to alleviate heat-induced oxidative stress. These findings are is in agreement with earlier studies reporting inhibition of ROS by mulberry flavonoids in a dose-dependent fashion (45). Even the mulberry leaf powder or their extracts have shown antioxidant, anti-inflammatory, hypolipidemic, and neuroprotective properties (9, 46, 47). A substantial decrease in MDA levels in treated groups revealed that MLF possess practical potential to decrease lipid peroxidation, as reported previously in mice model (18, 48).
The treatment showed no effect on serum SOD content in the present study. However, it significantly decreased the levels of serum T-AOC and CAT as compared to the control. Moreover, a higher GSH-Px level in MLF15 and MLF30 but lower level in MLF45 was observed as compared to the control. Studies have reported that oxidative stress induces the expression and activity of GSH-Px, hence the higher level of GSH-Px indicates more severe heat-induced oxidative stress (49). A lower level of GSH-Px observed in buffaloes supplemented with a higher dose of MLF (45 g/d) reveals the optimum level of flavonoids required to adequately scavenge free ROS ultimately leading to reduced oxidative stress and subsequent decreased activity of GSH-Px. The decrease in the activity of serum T-AOC and CAT in buffaloes supplemented with MLF revealed a relatively lower level of oxidative stress. This is in agreement with earlier studies on pre-weaning calves supplemented with MLF (20). Moreover, treatment with flavonoids has shown to enhance antioxidant capacity, improve non-specific immunity, and alleviate oxidative stress by increasing SOD and GSH-Px activity while decreasing the MDA concentration (19). It is mainly attributed to the ability of flavonoids to act as reducing agents and hydrogen donors to neutralize ROS and remove hydrogen peroxide and superoxide ions (19). The findings of the present experiment support the earlier studies about the dual functionality of MLF to alleviate oxidative stress; (1) by directly interacting with ROS (2) by increasing the activity of antioxidant enzymes.
Heat Shock Proteins and Metabolic Hormones
Heat shock proteins are well-known for their ability to mediate heat stress and considered as cellular thermometers. Especially the 70-kDa and 90-kDa heat shock proteins (HSP70 and 90) play a key role in thermo-tolerance. These proteins function as molecular chaperons and have significant roles in cellular thermotolerance, apoptosis, immune-modulation, and heat stress (50). Under oxidative stress conditions, the cellular expression of HSP is up-regulated to minimize the accumulation of denatured or abnormal proteins. The HSP are ubiquitously essential to prevent cell damage and enhance the ability to withstand oxidative and thermal stress (51).
In the present study, MLF significantly increased the serum HSP90 content as compared to the control group. However, a higher HSP90 level was observed in MLF45 while the other two treatment doses showed the same levels. Moreover, lower and medium levels of MLF (15 and 30 g/d) showed no effect on serum HSP70 content but a high level of MLF (40 g/d) significantly increased HSP70 content as compared to the control. These findings revealed a variable effective dose of MLF required to enhance the expression of HSP in buffaloes under heat stress conditions. Earlier studies in mice model have reported that quercetin can effectively upregulate the expression of the HSP (especially HSP70) through mediating the ERK/PPARγ signaling pathways (52). Moreover, the extent of such effects induced by flavonoids on HSP was dependent on the molecular weight (family) of HSP (52). This may be attributed to the fact that we observed slightly variable effects of MLF on the expression of serum HSP70 and 90.
Dietary flavonoids not only act as potent antioxidants but also regulate various signaling pathways to resist heat-induced oxidative stress at the cellular level (53). Moreover, they also possess the ability to enhance the absorption and utilization of dietary nutrients, immune response, and the development of mammary glands as well as lactation performance in animals (54, 55). In the present study, supplementation of MLF enhanced the concentration of serum metabolic hormones including E2, GH, and PRL. This may be attributed to the fact that the molecular structure of flavonoids resembles anabolic steroid hormones (phytoestrogens), which enables them to regulate the secretion of the different endocrine hormones by mediating hypothalamus-pituitary-axis (21). Owing to structural similarities of flavonoids with natural estrogen hormone along with other steroid hormones and their antagonists (56), they possess a great affinity for endoplasm reticulum and subsequent ability to mediate gene expression like estrogens, albeit at a lower affinity (57).
In the present study, a higher dose of MLF (45 g/day) showed more pronounced effects on the secretion of E2, GH, and PRL hormones as compared to low and medium levels of supplementation. We observed an increase of 46, 72, and 50 % in serum concentration of E2, GH, and PRL, respectively, in MLF45 as compared to the control group. The lower and medium levels of MLF (MLF15 and MLF30) had no effect on serum T3 and T4 contents. However, a substantial increase in serum T3 (25%) and T4 (40%) contents was observed with a higher dose of MLF (45 g/d) in buffaloes. It reveals that the effect of MLF on endocrine physiology is dose-dependent which is in agreement with earlier studies (45, 58).
Milk Yield and Composition
Due to rich nutrient profile, flavonoid contents, and abundant availability, mulberry leaves have been considered as alternate forage for ruminants especially in China (7). Studies have shown that ensiled mulberry leaves and sun-dried mulberry pomace are also potential feed ingredients for animals (59, 60). Mulberry leaf flavonoids have shown to improve the feed intake and growth performance in ruminants. However, no study has explored the effect of MLF on milk yield and composition in dairy cattle and buffalo. Therefore, we will compare our findings with studies supplementing flavonoids from different sources. Our findings revealed a significant increase in the daily milk yield of buffaloes in response to all levels of MLF supplementation as compared to the control group. However, this increase was more pronounced in MLF45 as the other two groups did not show a significant difference between each other. Similarly, FCM (4%) and milk protein (%) was also significantly higher in MLF45 as compared to other treatment and control groups. Similar findings have been reported earlier regarding the increase in daily milk and protein yield and energy corrected milk in response to supplementation of flavonoid-rich diet containing grape seed or grape marc meal extract or green tea or Curcuma in dairy cattle (61, 62).
In the present study, the treatment showed a significant increase in milk fat and protein (%) only with a higher dose of MLF (45 g/d) as compared to the control. Increased milk protein content has been observed in an earlier study in dairy cows supplemented with quercetin (35). In addition, our findings were consistent with higher propionate and total VFA contents observed in earlier studies involving supplementation of MLF in Holstein calves (63). These desirable changes in rumen fermentation may be attributed to the favorable effect of MLF on major cellulolytic bacteria (like Ruminococcus albus) as supplementation of ensiled mulberry leaves in the diet of fattening steers showed a significant increase in R. albus (60). Such changes in gut bacteria can potentially increase cellulose degradation leading to better rumen kinetics and higher VFA yield. Moreover, diet containing ensiled mulberry leaves and mulberry fruit pomace has shown to increase the relative abundance of amylolytic bacteria (particularly S. bovis, and Ruminobacter amylophilus) which can positively influence starch degradation in the upper gut and consequently increase the glucose content of the intestine (60). It has also been suggested that mulberry leave and fruit pomace can produce more fermentable glucose in the gut and also positively influence protein utilization by microorganisms subsequently leading to better energetic efficiency in ruminants (59, 60). Moreover, flavonoids (like quercetin) have shown to decrease total protozoa and methanogens to significantly decrease in vitro methane production without adversely affecting rumen microbial fermentation (64). These findings collectively suggest that MLF can potentially modulate rumen microbiome to mediate fermentation kinetics subsequently leading to better nutrient utilization and performance in ruminants. It is quite pertinent to mention that in the present study, MLF substantially decreased the abundance of Prevotella in the rumen of buffalo (data not presented) in MLF45 as compared to MLF15 and the control. It is well-established that Prevotella has a negative correlation with DMI and milk fat content (65, 66). Therefore, a reduction in the abundance of Prevotella in MLF45 was well-associated with higher milk fat (%). Moreover, Prevotella species are more specialized for protein degradation, peptide fermentation, and their uptake in the rumen (67). Its reduction in the rumen may have increased the amount of rumen bypass protein leading to enhanced duodenal protein supply that subsequently affected milk protein contents. These findings are in agreement with earlier study reporting reduction of dietary protein degradation under in vitro conditions when ryegrass grass was supplemented with rutin (68). In addition, studies have reported that lower Prevotella abundance has been observed in high producing cows as compared to low producing dairy cows (69). It is the most likely reason for higher milk yield and protein (%) observed in MLF45 as compared to other treatment groups.
The present study revealed that MLF at 45 g per day is an appropriate dose for supplementation in buffaloes to enhance milk production performance. The dose-dependent effect of MLF observed in the present study is also consistent with earlier studies reporting the higher average daily gain in calves fed with a high level of MLF as compared to lower levels (63, 70, 71). These studies suggested that alleviation of oxidative stress by MLF can impart beneficial effects on animal health and performance as observed on the average daily gain in calves previously. The desirable effects of MLF on lactation performance observed in the present study were well-associated with levels of serum GH and PRL. The galactopoietic effects of GH are well-established as its level is positively associated with milk yield in dairy cattle (72–74). The galactopoietic effects of growth hormone are mediated via increased secretion of GH-releasing factor and insulin-like growth factor-I (IGF-I) in dairy cows (74, 75). Studies have suggested that GH stimulates milk production by partitioning nutrients from adipose tissue and muscle, increasing blood flow to the mammary gland, increasing feed intake, and reducing whole-body amino acid oxidation and urinary nitrogen loss (76). Many studies have shown that the positive effects of GH on milk yield in dairy animals are related to an increase in the proliferation and activity of mammary epithelial cells (77, 78). It may be mediated either through the direct effect of GH on the mammary gland or an indirect effect via increased secretion of IGF-1 (79, 80). Moreover, GH also modulates the ribosomal protein S6 phosphorylation leading to enhanced protein synthesis in the mammary gland ultimately increasing milk protein yield (81, 82). On the other hand, PRL also plays an important regulatory function in mammary gland development, milk secretion, and expression of milk proteins (83–85) and have shown galactopoietic effects in dairy ruminants (86). Moreover, increasing the PRL secretion by injecting a dopamine antagonist (domperidone) was found to increase milk production (87). In addition, more apoptosis was found in the mammary glands of cows when PRL secretion was inhibited (88), suggesting that PRL is a survival factor for mammary cells and important mediator for lactation persistency. Keeping in view of the above-mentioned facts, it is suggested that a more pronounced effect of a higher dose of MLF on milk yield and milk protein (%) observed in the present study is partly attributed to the higher level of GH and PRL found in this group. In addition, higher insulin levels observed in MLF45 were well-associated with higher milk yield, FCM, and milk protein in the present study. Our findings are in agreement with earlier studies as supplementation of quercetin has shown to increase milk protein (%) in dairy cows that was believed to be associated with plasma insulin concentration via the GH-IGF axis (35, 89).
Mulberry leaf flavonoids have shown to increase the nutrient digestibility, dietary metabolizable energy, and rumen fermentation in pre and post-weaning calves (70, 90). The desirable effects of MLF on lactation performance observed in the present study may be attributed to the increased secretion of metabolic hormones and modulation of the insulin/IGF-1 signaling pathway (91). Moreover, flavonoids also possess excellent antioxidant activity, which leads to the restoration of physiological homeostasis at a cellular level like maintaining insulin levels. An increase in insulin secretion and subsequent acceleration in oxidative degradation of sugars eventually leads to enhanced feed intake and positive energy balance under heat stress conditions (92). Moreover, MLF possess functional characteristics like modulation of expression and activities of several enzymes involved in lipid and carbohydrate metabolism, which can ultimately affect animal performance to a greater extent (15, 16). Many studies have also provided the convincing evidence that flavonoids possess the therapeutic potential to improve metabolic parameters possibly through modulating peroxisome proliferator-activated receptor (PPAR)-γ, which plays a crucial role in the regulation of fatty acid and glucose metabolism (93, 94). Furthermore, mulberry leaves have also shown to maintain optimum conditions for rumen fermentation to enhance nutrient digestion and utilization in beef cattle (92). Dietary supplementation of MLF has also been reported to enhance the digestibility of organic matter and NDF in sheep (7). So, all these desirable effects can synergistically contribute to the overall beneficial outcomes in terms of better lactation performance in dairy animals as observed in this study.
According to the best of our knowledge, it is the first study to reveal the excellent antioxidant potential of MLF to alleviate heat stress in lactating buffaloes managed under the tropical environment. The increase observed in daily milk yield, FCM, and milk protein content in response to MLF45 is economically important and indicates the practical feasibility of MLF supplementation in dairy production systems particularly under heat stress conditions. The outcome of the present study suggested that alleviation of oxidative stress significantly contributes to the beneficial effects on metabolic status and lactation performance under a hot and humid climate.
Conclusions
Dietary supplementation of MLF significantly decreased the oxidative stress marker (MDA) while increasing the serum heat shock proteins, GHS-Px, and insulin contents. However, the treatment decreased T-AOC and CAT while no effect on serum SOD contents was observed owing to reduced oxidative stress in buffaloes. Our study revealed the dose-dependent effect of MLF on serum metabolic hormones (E2, PRL, GH, T3, and T4), daily milk yield, FCM (4%), and milk protein (%) in Murrah buffaloes. The present study concluded that MLF at 45 g per day is the most appropriate dose for supplementation in lactating buffaloes to enhance lactation performance and alleviation of heat-induced oxidative stress during the summer season. However, studies on a larger cohort are required to provide mechanistic insights on the role of MLF on alleviation of oxidative stress, modulation of rumen microbiome and hypothalamus-pituitary axis to mediate metabolic functions and performance in lactating buffaloes.
Data Availability Statement
The original contributions presented in the study are included in the article/supplementary material, further inquiries can be directed to the corresponding author/s.
Ethics Statement
The animal study was reviewed and approved by Ethics committee of the Chinese Academy of Agriculture Sciences, Guangxi Buffalo Research Institute, China.
Author Contributions
CY: conceptualization, funding acquisition, supervision, and validation. ML and FH: data curation. FH and ZT: formal analysis. ML: investigation. ML, LP, KP, LL, and XL: methodology. XL and CY: project administration. ZT, FX, and CY: resources. FH: writing—original draft. FH, ML, and CY: writing—review & editing. All authors contributed to the article and approved the submitted version.
Funding
This study was funded by the National Key Research and Development Program of China (2016YFD0500507 and 2018YFD0501600), the National Natural Science Foundation of China (Grant No. 31560649), the Guangxi Natural Science Foundation (2018GXNSFAA281162), and The Fundamental Research Funds for the Buffalo Research Institute, CAAS (201905008).
Conflict of Interest
The authors declare that the research was conducted in the absence of any commercial or financial relationships that could be construed as a potential conflict of interest.
References
1. Besle JM, Viala D, Martin B, Pradel P, Meunier B, Berdagué J, et al. Ultraviolet-absorbing compounds in milk are related to forage polyphenols. J Dairy Sci. (2010) 93:2846–56. doi: 10.3168/jds.2009-2939
2. Olagaray KE, Bradford BJ. Plant flavonoids to improve productivity of ruminants – a review. Anim Feed Sci Technol. (2019) 251:21–36. doi: 10.1016/j.anifeedsci.2019.02.004
3. Datta RK. Mulberry cultivation and utilization in India. In: Proceeding of FAO Electronic Conference on Mulberry for Animal Production. Rome (2000). p. 45–62.
4. Sánchez MD. World distribution and utilization of mulberry and its potential for animal. In: Proceedings of the Mulberry for Animal Production: Proceedings of an Electronic Conference Carried Out Between May and August 2000. Rome (2002).
5. Sánchez MD. Mulberry, An Exceptional Forage Available Almost Worldwide! Word Animal Review 93. Rome: FAO (2000).
6. Ba NX, Giang VD, Ngoan LD. Ensiling of mulberry foliage (Morus alba) and the nutritive value of mulberry foliage silage for goats in central Vietnam. Livest Res Rural Dev. (2005) 17:23–5.
7. Chen D, Chen X, Tu Y, Wang B, Diao Q. Effects of mulberry leaf flavonoid and resveratrol on methane emission and nutrient digestion in sheep. Anim Nutr. (2015) 1:362–7. doi: 10.1016/j.aninu.2015.12.008
8. Venkatesh Kumar R, Gautam C, Shobha N, Shankar RL. Use of mulberry leaves as supplementary food in cow and goat to improve milk production. Int J Appl Res. (2015) 1:81–4.
9. Chon SU, Kim YM, Park YJ, Heo B, Park YS, Gorinstein S. Antioxidant and antiproliferative effects of methanol extracts from raw and fermented parts of mulberry plant (Morus alba L). Euro Food Res Technol. (2009) 230:231–7. doi: 10.1007/s00217-009-1165-2
10. Arabshahidelouee S, Urooj A. Antioxidant properties of various solvent extracts of mulberry (Morus indica L.) leaves. Food Chem. (2007) 102:1233–40. doi: 10.1016/j.foodchem.2006.07.013
12. Yu Y, Li H, Zhang B, Wang J, Shi X, Huang J, et al. Nutritional and functional components of mulberry leaves from different varieties: evaluation of their potential as food materials. Int J Food Prop. (2018) 21:1495–507. doi: 10.1080/10942912.2018.1489833
13. Berger LM, Wein S, Blank R, Metges CC, Wolffram S. Bioavailability of the flavonol quercetin in cows after intraruminal application of quercetin aglycone and rutin. J Dairy Sci. (2012) 95:5047–55. doi: 10.3168/jds.2012-5439
14. Stoldt A, Derno M, Das G, Weitzel JM, Wolffram S, Metges CC. Effects of rutin and buckwheat seeds on energy metabolism and methane production in dairy cows. J Dairy Sci. (2016) 99:2161–8. doi: 10.3168/jds.2015-10143
15. Kobayashi Y, Miyazawa M, Kamei A, Abe K, Kojima T. Ameliorative effects of mulberry (Morus alba L.) leaves on hyperlipidemia in rats fed a high-fat diet: induction of fatty acid oxidation, inhibition of lipogenesis, and suppression of oxidative stress. Biosci Biotechnol Biochem. (2010) 74:2385–95. doi: 10.1271/bbb.100392
16. Berger LM, Blank R, Zorn F, Wein S, Metges CC, Wolffram S. Ruminal degradation of quercetin and its influence on fermentation in ruminants. J Dairy Sci. (2015) 98:5688–98. doi: 10.3168/jds.2015-9633
17. Liu H, Wang J, Ma J, Zhang Y. Interference effect of oral administration of mulberry branch bark powder on the incidence of type II diabetes in mice induced by streptozotocin. Food Nutr Res. (2016) 60:31606. doi: 10.3402/fnr.v60.31606
18. Lim HH, Lee SO, Kim SY, Yang S, Lim Y. Anti-inflammatory and antiobesity effects of mulberry leaf and fruit extract on high fat diet-induced obesity. Exp Biol Med. (2013) 238:1160–9. doi: 10.1177/1535370213498982
19. Kahkonen MP, Hopia A, Vuorela H, Rauha J, Pihlaja K, Kujala T, et al. Antioxidant activity of plant extracts containing phenolic compounds. J Agric Food Chem. (1999) 47:3954–62. doi: 10.1021/jf990146l
20. Wang B, Yang CT, Diao QY, Tu Y. The influence of mulberry leaf flavonoids and Candida tropicalis on antioxidant function and gastrointestinal development of preweaning calves challenged with Escherichia coli O141:K99. J Dairy Sci. (2018) 101:6098–108. doi: 10.3168/jds.2017-13957
21. Miksicek RJ. Commonly occurring plant flavonoids have estrogenic activity. Mol Pharmacol. (1993) 44:37–43.
23. AOAC. Official Methods of Analysis, 16th Edn. Washington, DC: Association of Official Analytical Chemists (1997).
24. Van Soest PV, Robertson J, Lewis B. Methods for dietary fiber, neutral detergent fiber, and nonstarch polysaccharides in relation to animal nutrition. J Dairy Sci. (1991) 74:3583–97. doi: 10.3168/jds.S0022-0302(91)78551-2
25. Anonymous. Technical Regulations for Feeding Buffaloes DB43/T 325-2006. Hunan Provincial Quality and Technical Supervision Bureau. Changsha: Hunan Institute of Animal Husbandry and Veterinary Medicine (2006).
26. Gaines WL. The Energy Basis of Measuring Milk Yield in Dairy Cows. Bulletin No. 308. Urbana, IL: University of Illinois (1928).
27. Coles E. Avian hematology and blood chemistry. Veterinary Clinical Pathology, 4th Edn. Philadelphia: WB Saunders Co (1986).
28. Zheng S, Liao S, Zou Y, Qu Z, Shen W, Shi Y. Mulberry leaf polyphenols delay aging and regulate fat metabolism via the germline signaling pathway in Caenorhabditis elegans. Age. (2014) 36:9719. doi: 10.1007/s11357-014-9719-z
29. Sheng Y, Liu J, Zheng S, Liang F, Luo Y, Huang K, et al. Mulberry leaves ameliorate obesity through enhancing brown adipose tissue activity and modulating gut microbiota. Food Funct. (2019) 10:4771–81. doi: 10.1039/C9FO00883G
30. Ricquier D. Neonatal brown adipose tissue, UCPI and the novel uncoupling proteins. Biochem Soc Trans. (1998) 26:120–3. doi: 10.1042/bst0260120
31. Ravussin E, Galgani JE. The implication of brown adipose tissue for humans. Annu Rev Nutr. (2011) 31:33–47. doi: 10.1146/annurev-nutr-072610-145209
32. Chondronikola M, Volpi E, Børsheim E, Porter C, Annamalai P, Enerbäck S, et al. Brown adipose tissue improves whole-body glucose homeostasis and insulin sensitivity in humans. Diabetes. (2014) 63:4089–99. doi: 10.2337/db14-0746
33. Meng Q, Qi X, Fu Y, Chen Q, Cheng P, Yu X, et al. Flavonoids extracted from mulberry (Morus alba L.) leaf improve skeletal muscle mitochondrial function by activating AMPK in type 2 diabetes. J Ethnopharmacol. (2019) 248:112346. doi: 10.1016/j.jep.2019.112326
34. Zhou Z, Zhou B, Ren L, Meng Q. Effect of ensiled mul- berry leaves and sun-dried mulberry fruit pomace on finishing steer growth performance, blood biochemical parameters, and carcass characteristics. PLoS ONE. (2014) 9:e85406. doi: 10.1371/journal.pone.0085406
35. Gohlke A, Ingelmann CJ, Nürnberg G, Weitzel JM, Hammon HM, Görs S, et al. Influence of 4-week intraduodenal supplementation of quercetin on performance, glucose metabolism, and mRNA abundance of genes related to glucose metabolism and antioxidative status in dairy cows. J Dairy Sci. (2013) 96:6986–7000. doi: 10.3168/jds.2013-6852
36. Vessal M, Hemmati M, Vasei M. Antidiabetic effects of quercetin in streptozocin-induced diabetic rats. Comp Biochem Physiol C Toxicol Pharmacol. (2003) 135C:357–64. doi: 10.1016/S1532-0456(03)00140-6
37. Hii CS, Howell SL. Effects of flavonoids on insulin secretion and 45Ca2+ handling in rat islets of Langerhans. J Endocrinol. (1985) 107:1–8. doi: 10.1677/joe.0.1070001
38. Li G, Ali IS, Currie RW. Insulin induces myocardial protection and Hsp70 localization to plasma membranes in rat hearts. Am J Physiol Heart Circ Physiol. (2006) 291:1709–21. doi: 10.1152/ajpheart.00201.2006
39. Rhoads RP, Baumgard LH, Suagee JK, Sanders SR. Nutritional interventions to alleviate the negative consequences of heat stress. Adv Nutr. (2013) 4:267–76. doi: 10.3945/an.112.003376
40. Tanaka M, Kamiya Y, Kamiya M, Nakai Y. Effect of high environmental temperatures on ascorbic acid, sulfhydryl residue and oxidized lipid concentrations in plasma of dairy cows. Anim Sci J. (2007) 78:301–6. doi: 10.1111/j.1740-0929.2007.00439.x
41. Fandy TE, Jiemjit A, Thakar M, Rhoden P, Suarez L, Gore S, et al. Decitabine induces delayed reactive oxygen species (ROS) accumulation in leukemia cells and induces the expression of ROS generating enzymes. Clin Cancer Res. (2014) 20:1249–58. doi: 10.1158/1078-0432.CCR-13-1453
42. Karatopuk DU, Gokcimen A. Effect of tenoxicam on rat liver tissue. Turk J Gastroenterol. (2010) 21:146–52. doi: 10.4318/tjg.2010.0073
43. Aréchiga CF, Vázquez-Flores S, Ortíz O, Hernández-Cerón J, Hansen PJ. Effect of injection of β-carotene or vitamin E and selenium on fertility of lactating dairy cows. Theriogenology. (1998) 50:65–76. doi: 10.1016/S0093-691X(98)00114-9
44. Celi P. Biomarkers of oxidative stress in ruminant medicine. Immunopharmacol Immunotoxicol. (2011) 33:233–40. doi: 10.3109/08923973.2010.514917
45. Liao B, Zhu D, Thakur K, Li L, Zhang J, Wei Z. Thermal and antioxidant properties of polysaccharides sequentially extracted from mulberry leaves (Morus alba L.). Molecules. (2017) 22:2271. doi: 10.3390/molecules22122271
46. Zhang H, Tsao R. Dietary polyphenols, oxidative stress and antioxidant and anti-inflammatory effects. Curr Opin Food Sci. (2016) 8:33–42. doi: 10.1016/j.cofs.2016.02.002
47. Chen XY, Zhang T, Xin W, Mark H, Jie K, Yu D, et al. A chemical investigation of the leaves of Morus alba L. Molecules. (2018) 23:1018. doi: 10.3390/molecules23051018
48. Li Q, Liu F, Liu J, Liao S, Zou Y. Mulberry leaf polyphenols and fiber induce synergistic antiobesity and display a modulation effect on gut microbiota and metabolites. Nutrients. (2019) 11:1017. doi: 10.3390/nu11051017
49. Van der Oost R, Beyer J, Vermeulen NP. Fish bioaccumulation and biomarkers in environmental risk assessment: a review. Environ Toxicol Pharmacol. (2003) 13:57–149. doi: 10.1016/S1382-6689(02)00126-6
50. Hassan F, Nawaz A, Rehman MS, Ali MA, Dilshad SMR, Yang C. Prospects of HSP70 as a genetic marker for thermo-tolerance and immuno-modulation in animals under climate change scenario. Anim Nutr. (2019) 5:340–50. doi: 10.1016/j.aninu.2019.06.005
51. Bhat S, Kumar P, Kashyap N, Deshmukh B, Singh G. Effect of heat shock protein 70 polymorphism on thermotolerance in tharparkar cattle. Vet World. (2016) 9:113–7. doi: 10.14202/vetworld.2016.113-117
52. Oyagbemi AA, Omobowale TO, Oladavies OE, Asenuga E, Ajibade TO, Adejumobi OA, et al. Quercetin attenuates hypertension induced by sodium fluoride via reduction in oxidative stress and modulation of HSP 70/ERK/PPARγ signaling pathways. Biofactors. (2018) 44:465–79. doi: 10.1002/biof.1445
53. Mansuri ML, Parihar P, Solanki I, Parihar MS. Flavonoids in modulation of cell survival signaling pathways. Genes Nutr. (2014) 9:400. doi: 10.1007/s12263-014-0400-z
54. Arjmandi BH, Khalil DA, Hollis BW. Ipriflavone, a synthetic phytoestrogen, enhances intestinal calcium transport in vitro. Calcif Tissue Int. (2000) 67:225–9. doi: 10.1007/s002230001123
55. Zafar TA, Weaver CM, Jones K, Moore DR, Barnes S. Inulin effects on bioavailability of soy isoflavones and their calcium absorption enhancing ability. J Agric Food Chem. (2004) 52:2827–31. doi: 10.1021/jf035080f
56. Zand RSR, Jenkins DJA, Diamandis EP. Steroid hormone activity of flavonoids and related compounds. Breast Cancer Res Treat. (2000) 62:35–49. doi: 10.1023/A:1006422302173
57. Ferguson LR. Role of plant polyphenols in genomic stability. Mutat Res. (2001) 475:89–111. doi: 10.1016/S0027-5107(01)00073-2
58. Divi RL, Doerge DR. Inhibition of thyroid peroxidase by flavonoids dietary. Chem Res Toxicol. (1996) 9:16–23. doi: 10.1021/tx950076m
59. Zhou B, Meng Q, Ren L, Shi F, Wei Z, Zhou Z. Evaluation of chemical composition, in situ degradability and in vitro gas production of ensiled and sun-dried mulberry pomace. J Anim Feed Sci. (2012) 21:188–97. doi: 10.22358/jafs/66063/2012
60. Li Y, Meng QX, Zhou B, Zhou Z. Effect of ensiled mulberry leaves and sun-dried mulberry fruit pomace on the fecal bacterial community composition in finishing steers. BMC Microbiol. (2017) 17:97. doi: 10.1186/s12866-017-1011-9
61. Gessner D, Koch C, Romberg FJ, Winkler A, Dusel G, Herzog E, et al. The effect of grape seed and grape marc meal extract on milk performance and the expression of genes of endoplasmic reticulum stress and inflammation in the liver of dairy cows in early lactation. J Dairy Sci. (2015) 98:8856–68. doi: 10.3168/jds.2015-9478
62. Winkler A, Gessner DK, Koch C, Romberg FJ, Dusel G, Herzog E, et al. Effects of a plant product consisting of green tea and curcuma extract on milk production and the expression of hepatic genes involved in endoplasmic stress response and inflammation in dairy cows. Arch Anim Nutr. (2015) 69:425–41. doi: 10.1080/1745039X.2015.1093873
63. Kong L, Yang C, Dong L, Diao Q, Si B, Ma J, et al. Rumen fermentation characteristics in pre- and post-weaning calves upon feeding with mulberry leaf flavonoids and candida tropicalis individually or in combination as a supplement. Animals. (2019) 9:990. doi: 10.3390/ani9110990
64. Oskoueian E, Abdullah N, Oskoueian A. Effects of flavonoids on rumen fermentation activity, methane production, and microbial population. Biomed Res Int. (2013) 1:8. doi: 10.1155/2013/349129
65. Jami E, White BA, Mizrahi I. Potential role of the bovine rumen microbiome in modulating milk composition and feed efficiency. PLoS ONE. (2014) 9:e85423. doi: 10.1371/journal.pone.0085423
66. Jiang Y, Ogunade I, Arriola K, Qi M, Vyas D, Staples C, et al. Effects of the dose and viability of Saccharomyces cerevisiae. 2. Ruminal fermentation, performance of lactating dairy cows, and correlations between ruminal bacteria abundance and performance measures. J. Dairy Sci. (2017) 100:8102–18. doi: 10.3168/jds.2016-12371
67. Stewart C, Flint H, Bryant M. The rumen bacteria. In: The Rumen Microbial Ecosystem. Springer (1997). p. 10–72. doi: 10.1007/978-94-009-1453-7_2
68. Leiber F, Kunz C, Kreuzer M. Influence of different morphological parts of buckwheat (Fagopyrum esculentum) and its major secondary metabolite rutin on rumen fermentation in vitro. Czech J Anim Sci. (2012) 57:10–8. doi: 10.17221/5479-CJAS
69. Mu Y, Lin X, Wang Z, Hou Q, Wang Y, Hu Z. High-production dairy cattle exhibit different rumen and fecal bacterial community and rumen metabolite profile than low-production cattle. Microbiol Open. (2018) 8:e00673. doi: 10.1002/mbo3.673
70. Zhang LY, Qu PB, Tu Y, Yang CT. Effects of flavonoids from mulberry leaves and candida tropicalis on performance and nutrient digestibility in calves. Kafkas Univ Vet Fak Derg. (2017) 23:473–9. doi: 10.9775/kvfd.2016.17111
71. Yaghoubi SM, Ghorbani GR, Rahmani HR, Nikkhah A. Growth, weaning performance and blood indicators of humoral immunity in holstein calves fed supplemental flavonoids. J Anim Physiol Anim Nutr. (2008) 92:456–62. doi: 10.1111/j.1439-0396.2007.00734.x
72. Hart IC, Bines JA, Morant SV. Endocrine control of energy metabolism in the cow: correlations of hormones and metabolites in high and low yielding cows for stages of lactation. J Dairy Sci. (1979) 62:270–7. doi: 10.3168/jds.S0022-0302(79)83235-X
73. Bines JA, Hart IC. Metabolic limits to milk production, especially roles of growth hormone and insulin. J Dairy Sci. (1982) 65:1375–89. doi: 10.3168/jds.S0022-0302(82)82358-8
74. Dahl GE, Chapin LT, Allen MS, Moseley WM, Tucker HA. Comparison of somatotropin and growth hormone-releasing, factor on milk yield, serum hormones, and energy status. J Dairy Sci. (1991) 74:3421–8. doi: 10.3168/jds.S0022-0302(91)78532-9
75. Gluckman PD, Breier BH, Davis RS. Physiology of the somatotropic axis with particular reference to the ruminant. I Dairy Sci. (1987) 70:442–66. doi: 10.3168/jds.S0022-0302(87)80028-0
76. Bauman DE. Bovine somatotropin and lactation: from basic science to commercial application. Domest Animal Endocrinol. (1999) 17:101–16.
77. Berry SD, McFadden TB, Pearson RE, Akers R. A local increase in the mammary IGF-1:IGFBP-3 ratio mediates the mammogenic effects of estrogen and growth hormone. Domest Anim Endocrinol. (2001) 21:39–53. doi: 10.1016/S0739-7240(01)00101-1
78. Molento CF, Block E, Cue RI, Petitclerc D. Effects of insulin, recombinant bovine somatotropin, and their interaction on insulin-like growth factor-I secretion and milk protein production in dairy cows. J Dairy Sci. (2002) 85:738–47. doi: 10.3168/jds.S0022-0302(02)74131-3
79. Akers RM, McFadden TB, Purup S, Vestergaard M, Sejrsen K, Capuco AV. Local IGF-I axis in peripubertal ruminant mammary development. J Mammary Gland Biol Neoplasia. (2000) 5:43–51. doi: 10.1023/A:1009563115612
80. Allan GJ, Tonner E, Barber MC, Travers MT, Shand JH, Vernon RG, et al. Growth hormone, acting in part through the insulin-like growth factor axis, rescues developmental, but not metabolic, activity in the mammary gland of mice expressing a single allele of the prolactin receptor. Endocrinology. (2002) 143:4310–9. doi: 10.1210/en.2001-211191
81. Zhou Y, Akers RM, Jiang H. Growth hormone can induce expression of four major milk protein genes in transfected MAC-T cells. J Dairy Sci. (2008) 91:100–8. doi: 10.3168/jds.2007-0509
82. Hayashi AA, Nones K, Roy NC, McNabb WC, Mackenzie DS, Pacheco D, et al. Initiation and elongation steps of mRNA translation are involved in the increase in milk protein yield caused by growth hormone administration during lactation. J Dairy Sci. (2009) 92:1889–99. doi: 10.3168/jds.2008-1334
83. Horseman ND, Zhao W, Montecino-Rodriguez E, Tanaka M, Nakashima K, Engle SJ, et al. Defective mammopoiesis, but normal hematopoiesis, in mice with a targeted disruption of the prolactin gene. EMBO J. (1997) 16:6926–35. doi: 10.1093/emboj/16.23.6926
84. Dybus A. Associations of growth hormone GH and prolactin PRL genes polymorphism with milk production traits in polish black and white cattle. Anim Sci Pap Rep. (2002) 20:203–12.
85. Othman OE, Zayed FA, El Gawead AA, El-Rahman MRA. Genetic polymorphism of three genes associated with milk trait in Egyptian buffalo. J Genetic Eng Biotechnol. (2011) 9:97–102. doi: 10.1016/j.jgeb.2011.09.002
86. Lacasse P, Ollier S, Lollivier V, Boutinaud M. New insights into the importance of prolactin in dairy ruminants. J Dairy Sci. (2016) 99:864–74. doi: 10.3168/jds.2015-10035
87. Lacasse P, Ollier S. The dopamine antagonist domperidone increases prolactin concentration and enhances milk production in dairy cows. J Dairy Sci. (2015) 98:7856–64. doi: 10.3168/jds.2015-9865
88. Boutinaud M, Lollivier V, Finot L, Bruckmaier RM, Lacasse P. Mammary cell activity and turnover in dairy cows treated with the prolactin-release inhibitor quinagolide and milked once daily. J Dairy Sci. (2012) 95:177–87. doi: 10.3168/jds.2011-4461
89. McGuire MA, Dwyer DA, Harrell RJ, Bauman DE. Insulin regulates circulating insulin-like growth factors and some of their binding proteins in lactating cows. Am. J. Physiol. (1995) 269:E723–30. doi: 10.1152/ajpendo.1995.269.4.E723
90. Yang CT, Diao QY, Qu PB, Si B. Effects of Candida tropicalis and mulberry leaf flavonoids on nutrient metabolism and rumen fermentation of calves. Chinese J Anim Nutr. (2016) 28:224–34. doi: 10.3969/j.issn.1006-267x.2016.01.029
91. Ayuda-Durán B, González-Manzano S, Miranda-Vizuete A, Sánchez-Hernández E, González-Paramás AM. Exploring target genes involved in the effect of quercetin on the response to oxidative stress in Caenorhabditis elegans. Antioxidants. (2019) 8:585. doi: 10.3390/antiox8120585
92. Huyen NT, Wanapat M, Navanukraw C. Effect of Mulberry leaf pellet (MUP) supplementation on rumen fermentation and nutrient digestibility in beef cattle fed on rice straw-based diets. Anim Feed Sci Technol. (2012) 175:8–15. doi: 10.1016/j.anifeedsci.2012.03.020
93. Fang X, Gao J, Zhu D. Kaempferol and quercetin isolated from Euonymus alatus improve glucose uptake of 3T3-L1 cells without adipogenesis activity. Life Sci. (2008) 82:615–22. doi: 10.1016/j.lfs.2007.12.021
94. Chia-Chi C, Kristina M, Xie G, Arion K, Akkarach B, Angel O, et al. Quercetin is equally or more effective than resveratrol in attenuating tumor necrosis factor-{alpha}-mediated inflammation and insulin resistance in primary human adipocytes. Am J Clin Nutr. (2010) 92:1511–21. doi: 10.3945/ajcn.2010.29807
Keywords: mulberry leaf flavonoids, milk yield, antioxidant enzymes, metabolic hormones, heat shock proteins, heat stress
Citation: Li M, Hassan F, Tang Z, Peng L, Liang X, Li L, Peng K, Xie F and Yang C (2020) Mulberry Leaf Flavonoids Improve Milk Production, Antioxidant, and Metabolic Status of Water Buffaloes. Front. Vet. Sci. 7:599. doi: 10.3389/fvets.2020.00599
Received: 19 May 2020; Accepted: 27 July 2020;
Published: 04 September 2020.
Edited by:
Ahmed E. Kholif, National Research Centre, EgyptReviewed by:
Tarek Abdelfattah Morsy, National Research Centre, EgyptMetha Wanapat, Khon Kaen University, Thailand
Copyright © 2020 Li, Hassan, Tang, Peng, Liang, Li, Peng, Xie and Yang. This is an open-access article distributed under the terms of the Creative Commons Attribution License (CC BY). The use, distribution or reproduction in other forums is permitted, provided the original author(s) and the copyright owner(s) are credited and that the original publication in this journal is cited, in accordance with accepted academic practice. No use, distribution or reproduction is permitted which does not comply with these terms.
*Correspondence: Chengjian Yang, eWNqMDc0NiYjeDAwMDQwO3NpbmEuY29t
†These authors have contributed equally to this work