- 1Veterinary Sciences, Botswana University of Agriculture and Natural Resources, Gaborone, Botswana
- 2Department of Veterinary Services, Ministry of Agriculture, Gaborone, Botswana
- 3Paul G. Allen School for Global Animal Health, Washington State University, Pullman, WA, United States
- 4UMR Animal, Health, Territories, Risks and Ecosystems (ASTRE), CIRAD-INRA- Uni. Montpellier, Campus International de Baillarguet, Montpellier, France
- 5CIRAD, UMR ASTRE, RP-PCP, Harare, Zimbabwe
Ticks and tick borne diseases (TBDs) undermine livestock production with considerable economic losses to livestock producers in endemic areas worldwide. Despite the impact of ticks and TBDs in livestock production, there is a paucity of information on ticks and diseases they transmit in Botswana. To address this gap, a cross-sectional study was conducted to determine (i) the seroprevalence of selected tick borne (TB) pathogens and (ii) the diversity and abundance of ixodid ticks among 301 cattle foraging around two protected areas in northern Botswana, differing by the presence or absence of a physical barrier (fence) separating wildlife and livestock. Competitive inhibition enzyme linked immuno-sorbent assay (cELISA) was used to test for Anaplasma spp. infection and Indirect Fluorescence Antibody Test (IFAT) was used to test for Theileria parva, Babesia bovis, and B. bigemina. Ticks were identified morphologically at either genus or species level. Seroprevalence of cattle was found to be 90% for Anaplasma spp., followed by 38.6% for Babesia spp. and 2.4% for T. parva. Except for Babesia spp., comparisons of the seroprevalence of the selected haemoparasites between the two wildlife-livestock interface areas were not significantly different. The overall prevalence of ticks was found to be 73.4% with Amblyomma variegatum being the most abundant (53.1%) followed by Rhipicephalus evertsi evertsi (31.7%) and R. (B.) decoloratus (7.7%). Except for Babesia spp., comparisons of the seroprevalence of the selected haemoparasites between the two study areas were not significantly different while comparisons of the burden of tick infestation between the study sites revealed significant difference for A. variegatum and R. evertsi evertsi with both tick infestations higher where there is no barrier. Our work provided baseline data on TBD pathogens and tick infestation in cattle populations exposed to different levels of contact with adjacent buffalo populations. The presence of a veterinary fence did not significantly influence the seroprevalence of the selected TBD pathogens (except for Babesia spp.) but seemed to reduce tick burdens in cattle. Findings from this study can be used for guiding future epidemiological study designs to improve our understanding of ticks and TBDs dynamics in northern Botswana.
Introduction
Ticks (Acari: Ixodidae) and tick borne haemoparasites including anaplasmosis, babesiosis, ehrlichiosis (cowdriosis), and theileriosis limit livestock production in endemic areas worldwide including sub-Saharan Africa (1, 2). Ticks and TBDs affect nearly 80% of the world's cattle population, with estimated annual global costs ranging from US$ 14-19 billion (3). Limited information exists on the impact of TBDs in the national economies of sub Saharan countries. In Tanzania, for example, the annual losses due to TBDs in the livestock sector were estimated at US$ 364 million. An estimated 70% of these losses were theileriosis, the remaining 30% being represented by anaplasmosis, babesiosis, and cowdriosis (4). In South Africa, annual losses due to TBDs are estimated to range between R70 and R550 million (5). Taken together, this may lead to increased poverty and impact negatively on food security. The level of interaction between wild and domestic animals relies on host behavior, which is influenced by environmental conditions and land use (protected areas or communal land, veterinary fences and infrastructure (6, 7). The coexistence of wildlife, livestock and humans at different wildlife-livestock interfaces, may facilitate pathogen transmission affecting animal, wildlife and public health (8). Kock (9) reported that wildlife can contribute to livestock TBDs by serving as both sources and maintenance hosts for pathogens causing diseases. The African buffalo (Syncerus caffer), for example, is the natural reservoir host of Theileria parva which causes East Coast fever (ECF) and Corridor Disease (10). Other TB pathogens of veterinary importance such as Anaplasma marginale, Ehrlichia ruminantium, B. bigemina, and B. bovis causing Anaplasmosis, heartwater, and babesiosis) have also been reported in African buffalo (11, 12). In Botswana, a recent study by Eygelaar et al. (2), revealed the presence Theileria spp. and Anaplasma spp. in buffalo in two protected areas in northern Botswana. The buffalo does not show clinical disease following infection with TBDs but as a reservoir host, it can transmit TBDs pathogens to cattle when both species share infected ticks during their interaction resulting in decreased animal production (2, 8). On the other hand, it has been reported that domestic livestock play a role in facilitating the spread of tick borne haemoparasites among the wild population (13). Exploring the prevalence of tick borne pathogens and tick infestation in cattle at the edge of protected areas in Southern Africa including Botswana, where interactions between domestic and wild ruminants are common and widespread is necessary not only for herd health but also for wildlife conservation and public health. In the case of zoonosis, the health of rural people with limited access to health services can suffer from the spill over of pathogens from domestic and wildlife populations (7). Little is known on TBDs pathogens in cattle at the wildlife-livestock interface in northern Botswana. Therefore, this study aimed to determine the seroprevalence of selected TBDs pathogens (Anaplasma spp., B. bigemina, B. bovis, and T. parva) and to document diversity, abundance, and spatial distribution of ixodid tick species circulating in cattle at two different wildlife-livestock interface areas in northern Botswana.
Materials and Methods
Study Sites
The study was conducted between April and August 2015 in northern Botswana, Maun west (MW) located in Maun district, and Chobe west (CW) located in Kasane district. Both districts encompass iconic protected areas such as the Okavango Delta (OD) in Maun district and Chobe National Park (CNP) in Kasane which are the largest wildlife areas and tourism attractions of the country and part of the Kavango-Zambezi Trans-frontier Conservation Area (KAZA TFCA). IN CW, the Chobe river forms the border between Botswana and Namibia and there is no veterinary fence (no fence interface) separating wildlife from livestock populations, while in MW there is a fence surrounding the edge of the OD (fenced interface) (Figure 1). In Southern Africa, veterinary cordon fences are prone to be damaged by elephants and floods leading to buffalo incursions into livestock areas or visa-visa (14, 15). Chobe river and the Okavango delta are the main sources of water for livestock and wildlife in the study sites providing abundant water throughout the year. As in other areas of Botswana MW and CW are characterized by three seasons; namely: the wet season (November–March), the cool dry season (April–July), and the hot dry season (August–October). This seasonal pattern influences the movement and contact of livestock and wildlife with the peak interaction during the dry season as compared to the wet season. Vegetation is predominantly deciduous dry woodland and scattered grassland (2). The total cattle census in northern Botswana is estimated at 210,000 heads, with 3,500 in MW and 4,530 in CW and (Department of Veterinary Services, Botswana, unpublished Records, 2015). In both study areas, cattle are raised under traditional (communal) system characterized by shared grazing.
Using a global positioning system (GPS) device (Garmin eTrex® Legend C), geographical coordinates of each sampled localities (crush pens) were taken. In MW, the crushes were selected within 10 km from the veterinary cordon fence while in CW, within 10 km from Chobe river (Figure 1). In both areas, cattle of different age groups (calves and adults) based on dentition, both sexes (male and females), and breeds comprising of indigenous Tswana (B. indicus) and other breeds (produced by crossing of Tswana breed with imported breeds), presented to the crush pens were selected for sampling in this study. Sampling in this study was done in winter and in two parts: seroprevalence in April (dry cool season) and tick collection in August (hot dry season). The same sample size was implemented for seroprevalence and tick sampling but in different animals.
Tick Sampling and Identification
A total of 301 cattle were sampled. Ticks were detached by manual (hand) removal holding the tick at the basis of the capitulum and gently removing it by exerting a horizontal pull to the body surface so as not to lose the mouthpart. During sampling, an effort was made to collect from different body parts (head, ears, neck, dewlap, legs, perineum, belly, udder, scrotum, and the base of the tail) in order to obtain a representative idea of all the species present using tick collection method described by Londt et al. (16). Specimens were preserved in 70% ethanol. The sample vials were labeled indicating owner's name, crush pen, date of collection and animal information (age, sex, and breed), and month of collection. Ticks were identified and categorized to genus and species levels using a stereoscope (80-fold magnification) and following morphological descriptions made by Walker et al. (17). The geographic coordinates of each locality was recorded and used for the production of maps of the distribution of the tick species in Arcgis 10.4.
Sampling and Sample Size Determination
The prevalence of antibodies of Anaplasma spp., Babesia spp., and Theileria parva in cattle in Botswana, was unknown therefore prevalence from previous studies in neighboring countries were used to determine sample size. Thus, the expected prevalence rate of 26% for A. marginale (18) in South Africa using cELISA with specificity of 99.5%, sensitivity of 98% (19), a 95% level of confidence and a 10% tolerable error was used to predict prevalence of antibodies of tick borne pathogens. A software Free calc® Version 2—Survey tool box described by Humphry et al. (20) was used to calculate the sample size. The sample size to detect antibodies against anaplasmosis was estimated at a minimum of 296 cattle. However, a total of 301 cattle were sampled. The sample size was distributed proportionally to the crushes according to the available census data from the veterinary services. Within every herd, a systematic random sampling was applied.
Serological Testing
At each epidemiological unit represented by a crush, the cattle to be sampled were systematically randomly selected with sampling interval of 10 animals. Cattle were manually restrained and about 4 ml of blood was obtained by venepuncture of the jugular or coccygeal veins of each animal using plain vacutainer tubes. The blood was then centrifuged and sera harvested into cryovials, labeled and stored frozen at −20°C until the samples were tested.
cELISA Test for Anaplasma spp.
Antibodies against Anaplasma spp. in serum were detected by MSP-5 competitive enzyme linked immunosorbent assay (cELISA) using commercially available Anaplasma Antibody Test Kit, cELISA (VMRD Inc., Pullman, WA, USA) [sensitivity (se) 95%; specificity (sp) 98%]. The test was performed according to the manufacturer's instruction at Botswana University of Agriculture and Natural Resources (BUAN), Botswana. Briefly, 96-well flat-bottom plates coated with recombinant MSP- 5 were incubated with the sample sera for 1 h at room temperature (21–25°C) and washed 2 times with a wash solution. Then, antibody peroxidase conjugate was added to each well and incubated for an additional 20 min at room temperature. After incubation, plates were washed four times and o-phenylenediamine dihydrochloride was added to the plates and incubated for an additional 20 min. A stop solution was added before the plate was read. An ELISA reader (Multiskan FC, Thermo Scientific, and Waltham, USA) was used to measure the optical density at 620 nm wavelength. A cut off of 30% inhibition was used to differentiate between positive and negative samples. Serum samples with ≥30% inhibition were considered positive while samples with <30% inhibition were considered negative. The percent inhibition (% I) was calculated using formula: 100 – [(Test sample optical density/Mean negative control sera optical density) × 100]
IFAT for T. parva, B. bovis, and B. bigemina
The sampling process allowed obtaining sera from 301 cattle. Unfortunately, six samples were lost making 295 serum samples available for testing. As the samples were coming from FMD zones, prior to testing, sera was subjected to heat treatment at 60°C during 30 min. This procedure was reported not to affect the test performance. The schizont antigen IFAT (se of 96% and sp of 95%) was carried out according to the method previously described by Burridge and Kimber and Goddeeris et al. (21–23) with slight changes at Agricultural Research council Onderstpoort Veterinary Research Council Institute (ARC-OVI), Republic of South Africa. Phosphate-buffered saline solution (PBS) was used to make 2-fold dilutions of the test and control sera of 1/80, 1/160 in 96 well micro-liter plates. Diluted test serum was incubated at 37°C for 60 min with the antigen fixed on the glass slides. Unbound antibodies are removed by washing them off. Bound antibodies are revealed by incubating the antigen-antibody complexes (60 min) with anti-species (e.g., anti-bovine) Immunoglobulin G (IgG) antibodies conjugated to a fluorescence compound—fluorescein isothiocyanate (FITC). Unbound FITC-conjugate is washed away leaving only the FITC-conjugate bound to test serum antibody-antigen complex. The fluorescing complexes (for a positive reaction) are read with a fluorescence microscope at 50x and 100x objectives. No fluorescing complexes are observed in a negative reaction and dull non-specific fluorescence may be observed. A titer of ≥1:80 were considered as positive reactions (Olivier Matthee, personal communication).
Statistical Analysis
Data from serological tests was entered into Microsoft excel spread sheet, 2007. Seroprevalence was determined by expressing positive sera as a percentage of the total number of sera tested while the prevalence of ticks was determined by dividing the number of animals infested by the sample size expressed as a percentage. Risk factors potentially affecting seroprevalence and tick infestation such as area, age, sex and breed were considered in this analysis. Specifically, the chi-square test calculation was used to determine the association of the risk factors and selected tick borne pathogen seroprevalence and tick infestation using SAS (24). P < 0.05 were considered statistically significant.
Results
Tick Infestation and Distribution
The overall prevalence of ticks on cattle, without taxonomic and area differentiation was 73.4% (221/301) CI 95% [68.1–78.1]. A total of 2,522 ixodid ticks (1,415 males and 1,107 females) were collected from cattle at the two study sites. Three tick genera Amblyomma, Rhipicephalus (including Boophilus sub-genus), and Hyalomma and seven species were identified: A. variegatum, R. evertsi-evertsi, R. (B.) decoloratus, R. appendiculatus, H. marginatum rufipes, H. truncatum, and R. sanguineous. Amblyomma, was the most abundant and widely distributed genus in all study sites 53.1% (1,338/2,522) CI 95% [51.1–55.0] followed by Rhipicephalus, 40.8% (1,030/2,522) CI 95% [38.9–42.7] and Hyalomma, 6.1% (154/2,522) CI 95% [5.2–7.1]. A. variegatum was the most prevalent and widely distributed species (53.1%) followed by R. evertsi evertsi (31.7%); R. (B.) decoloratus (7.7%); H. marginatum rufipes (4%); H. truncatum (2.1%) R. appendiculatus (1%), respectively (Table 1, Figure 2).
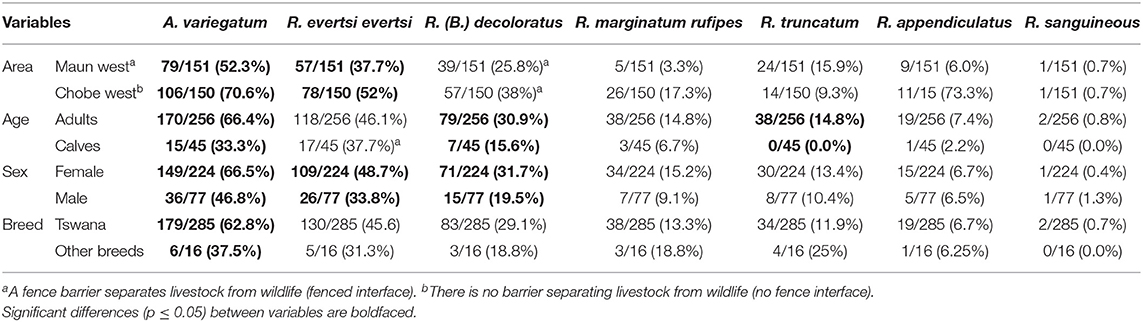
Table 1. A comparison of ixodid tick species prevalence (%) per area, age, sex, and breed at the wildlife-livestock interface areas in northern Botswana (Maun west and Chobe west).
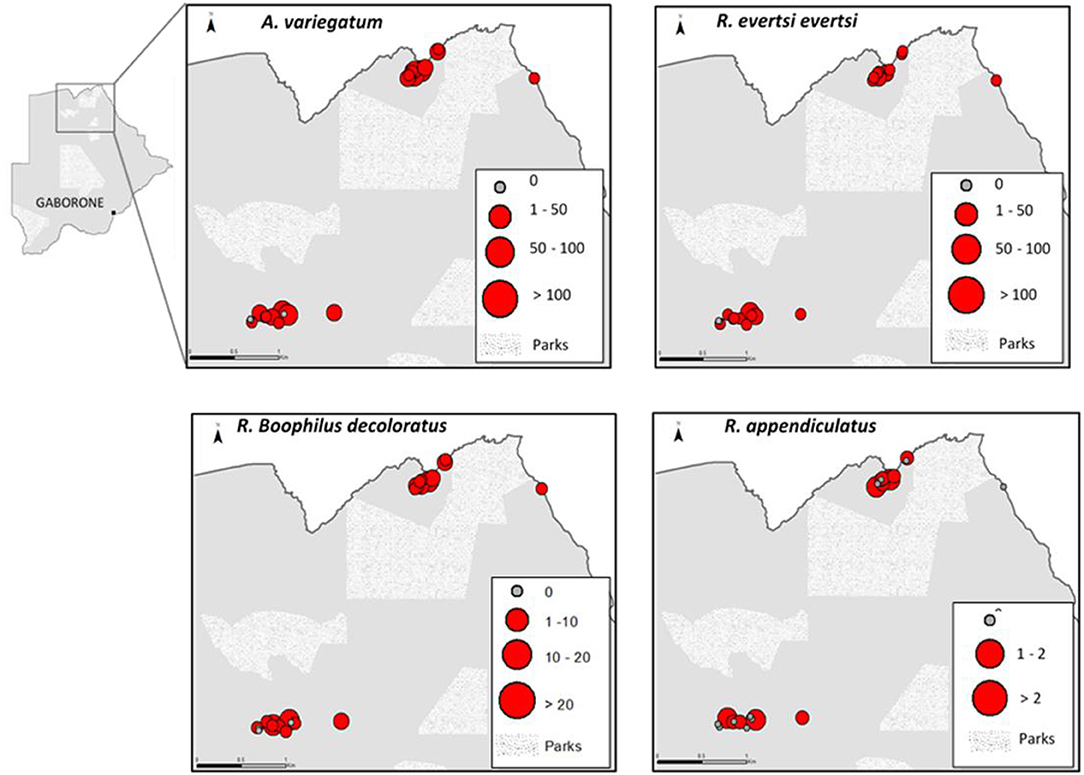
Figure 2. Spatial distribution of tick species at the wildlife-livestock interface in northern Botswana (Maun west and Chobe west).
A Comparison Between Areas
The comparison of A. variegatum and R. evertsi evertsi between study zones indicated that the infestation with these tick species were significantly higher (p = 0.001) and (p = 0.01), respectively in Chobe west where there is no fence between livestock and wildlife compared to Maun west where a fence is present (Table 1).
Influence of Age, Sex, and Breed
There was a significant difference (p ≤ 0.05) between age of cattle that were infested with A. variegatum, R. (B.) decoloratus, and H. truncatum with adults having a higher infestation than calves. There was also significant difference (p ≤ 0.05) between sex of the animals that were infested with A. variegatum, R. evertsi evertsi, and R (B.) decoloratus with females being more prone to infestation than males. Except for A. variegatum, which was more prevalent in Tswana compared to other breeds, there was no significant difference in infestation by ticks between breeds (Table 1).
Seroprevalence of Selected TBD Pathogens in Cattle in Northern Botswana
The seroprevalence of selected TBDs in Maun west (fenced interface) and Chobe west (unfenced interface), was determined using serological techniques. Seroprevalence of cattle in the two areas combined was found to be 90, 95% CI [86.1–92.9] for Anaplasma spp. being the highest, followed by Babesia spp. at 38.6, 95% CI [33.27–44.31] and the lowest was T. parva only at 2.4, 95% CI [1.15–4.82]. The test for Babesia spp. delineated the organisms into Babesia bovis and Babesia bigemina. B. bigemina had a higher prevalence (29.5%) compared to B. bovis (9.2%). Observed seroprevalence for the TBDs is given in (Table 2).
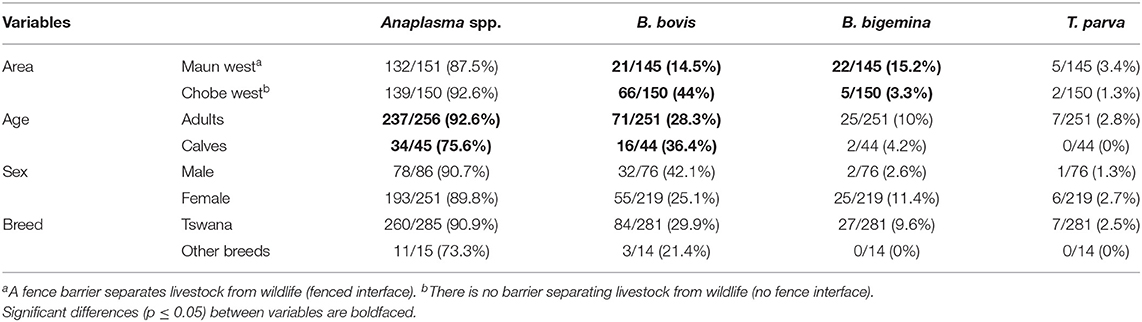
Table 2. A comparison of selected TBD pathogens seroprevalence (%) per area, age, sex, and breed at wildlife-livestock interface areas in northern Botswana (Maun west and Chobe west).
A Comparison Between Areas
Comparisons were made to determine effect by area and type of interface on seroprevalence. There was no significant difference in seroprevalence of Anaplasma spp. antibodies between Maun west and Chobe west (Table 2). Interestingly, seroprevalence of B. bovis in Maun west was significantly higher than in Chobe west (p = 0.0004) while Babesia bigemina was significantly higher in Chobe west compared to Maun west (p < 0.00001). Seroprevalence of T. parva in Maun west was more than twice that in Chobe west but not significantly different (Table 2). Mixed infections were found in this study. The results indicate that a total of 125 cattle out of 295 cattle had mixed infections. Cattle in Chobe west had a higher number of mixed infections compared to those in Maun west. The highest mixed infection consisted of B. bigemina and Anaplasma spp. at 27.8, 95% CI [23.0–33.2] followed by B. bovis and Anaplasma spp. at 8.5, 95% CI [5.81–12.2], B. bigemina and B. bovis at 2.5%, B. bigemina and T. parva at 0.7, 95% CI [0.19–2.44] B. bovis and T. parva at 0.7, 95% CI [0.19–2.44], respectively. Only one (1) animal in Maun west was infected by all the pathogens.
A Comparison by Age and Sex
There was a significant difference between the ages of animals that harbored A. marginale. The infection was significantly higher in adults compared to calves (p = 0.0004). There was a significant association of the sex of animals with seroprevalence of B. bovis. The infection was significantly higher in females compared to males (p = 0.0004). Breed of cattle did not appear to predispose animals to the selected TBD pathogens although there was a tendency for Anaplasma infection to be slightly higher in indigenous Tswana cattle compared to other breeds (Table 2).
Discussion
As it is the case for many sub-Saharan countries, there is a paucity of information on the occurrence and prevalence of ticks and TBDs of cattle in Botswana. The present survey was undertaken to determine the seroprevalence of selected TBDs pathogens, the abundance, diversity, and distribution of ticks infesting cattle at the wildlife-livestock interface in northern Botswana. Although, cross-sectional studies are limited at determining a cause-effect relationship, the findings of this study provide baseline data on ticks and TBDs pathogens that can inform the development of herd health management plan and guide future epidemiological studies. This study identified the presence of seven species of ticks: (A. variegatum, R. evertsi-evertsi, R. (B.) decoloratus, R. appendiculatus, H. rufipes, H. truncatum, and R. sanguineus). Tick species identified in our study (except for R. simus and R. zambeziensis) is in agreement with previous surveys implemented several decades ago in Botswana (25–27). Similarly, the distribution and composition of the identified tick species are in accordance with previous work (27) except for R. sanguineous. The presence of some tick species like R. appendiculatus, R. (B.) decoloratus, or R. evertsi evertsi in our study, were suspected based on findings of a previous study in buffalo populations from our study area (2). Similarly, the occurrence of outbreaks of T. parva in the area was suspected but never confirmed by clinical diagnosis. Overall, tick infestation was relatively high (73.4%). The observed tick burden among cattle in the study areas may be attributed to communal grazing practice that exposes animals to tick infested areas. This high tick infestation may increase the risk of occurance to tick borne pathogens. Strategic tick control during peak periods (summer) is necessary to allow ticks to naturally sustain endemic stability of TBDs through continuous challenge.
Amblyomma variegatum, R. evertsi evertsi, R. (B.) decoloratus were the most abundant and widely distributed tick species indicating high potential for transmission of heartwater, anaplasmosis, and babesiosis. A. variegatum was the most common tick in this study. This tick species is widely distributed through West, Central, East, and southern Africa including Zambia, north eastern Botswana, the Caprivi Strip of Namibia, northern part of Zimbabwe and central and northern Mozambique (17, 27). The observed prevalence of A. variegatum found in the current study is in agreement with Tesgera et al. (28), in Ethiopia who reported A. variegatum as the predominant tick species at a much higher prevalence (64%) than those found in our study. A. variegatum is an important vector of Ehrlichia ruminantium, the organism that causes heartwater in cattle (29). Moreover, this tick species is also incriminated in the epidemiology of Dermatophilus congolensis responsible for cutaneous streptothricosis that damages hides and skins of cattle (30). Owing to the prevalence of A. variegatum in this study, it would be relevant to explore the epidemiology of heartwater in the study sites.
Riphicephalus evertsi evertsi was the second most abundant and widely distributed species. This was not surprising considering that R. evertsi evertsi tolerates a wide range of climatic conditions (31) and has a widespread distribution, being the commonly found ixodid tick on livestock throughout most part of Africa (5) and in Botswana (27). Thus, it was not surprising that it was recorded in each sampled crush. Findings of the observed tick species is of great veterinary importance as these ticks are vectors of anaplasmosis (R. evertsi evertsi) and babesiosis (Boophilus spp.) found in this survey. Despite its low density, R. appendiculatus is the vector for the protozoan T. parva, the causative pathogen of East Coast Fever, Corridor Disease and Zimbabwean Theileriosis (17).
Tick species identified in this study such as A. variegatum and H. rufipes marginatum not only are of veterinary importance, but are vectors of zoonotic diseases such as Q fever, tick-bite fever, and Crimean–Congo haemorrhagic fever, respectively. The abundance of these tick species, may suggest that humans in the sampled sites are exposed to these diseases when bitten by infected ticks. Therefore, surveillance of TBDs in humans in the study areas is recommended.
Overall, we found higher tick infestations in the unfenced interface area compared to the fenced one, except for the case of R. truncatum. However, those variations of reported tick burdens were only significantly higher (p < 0.05) for A. variegatum and R. evertsi evertsi. This result may suggest an association of increased tick burden in cattle with a wildlife-livestock interface where there is no physical separation. However, in addition to the presence of physical barriers our results could have also been confounded by many other factors such as the complex interaction of several environmental and climatic conditions (annual rainfall, atmospheric temperature, and relative humidity), vegetation type, and host availability (32–34). Additionally, some tick species are ecological flexible and may easily adapt to changing climate and new habitats (35). The influence of these variables was not evaluated and may have influenced tick infestation and disease transmission. Moreover, climate change can change the geographical distribution of ticks and thus, influencing their occurrence, and pathogen transmission. Climatic variability is known to influence the tick habitat and the prevalence and incidence of haemoparasites (36). Seasonality also impacts tick infestation of livestock. Tick infestation in cattle is higher during the rainy season than the dry season since ticks have more successful reproduction cycles in wet and warm seasons than in dry cold periods (17, 37). One of the limitations of this study is that sampling was carried out in the winter season and this may have influenced tick population dynamics and infestation. Sampling in other seasons would have given a more accurate picture of the tick diversity in the study areas. Tick control methods may also influence the prevalence and endemic stability of TBDs in an area. In our study, information about acaricide treatment was not collected. However, we acknowledge that this could be another plausible reason for differences in the tick infestations between the study sites. This is an important limitation of our study and we recommend that future assessments of TBD's of this kind consider reporting and quantifying information on acaricide treatments and including these aspects in the interpretation of the results.
Small ruminants and wildlife are host to a diversity of tick species infesting cattle (38). Moreover, a study by Espinaze et al. (13), showed that small ruminants and dogs were important domestic animals for network connectivity with wildlife. This may facilitate cross-infestation with ticks and transmission of ticks and TB pathogens between wildlife and livestock at the interface areas (39). Therefore, we also recommend the inclusion of small stock other domestic animals, buffalo, and other wildlife animals in future investigations on the epidemiology and control of ticks and TB pathogens in future studies.
In this study, results of serological tests demonstrate the exposure of cattle to different vector borne pathogens. Considering that test performances of cELISA for Anaplasma spp. and IFAT for Babesia spp. and Theileria parva were quite high, results are fairly comparable. Antibodies to Anaplasma spp. was detected at a high frequency (90%) of the samples tested with cELISA. This finding is not surprising considering that Anaplasma has a wide vector range and can also be mechanically transmitted by biting flies (40, 41). In the south east region of Botswana, with comparatively less wildlife, Ramabu et al. (42) reported a similarly high prevalence of 91% Anaplasma spp. infection in beef and dairy cattle. This indicates that Anaplasma is widespread in the country and high prevalence cannot be associated with interaction of wildlife with cattle (41). Previous studies in eastern and southern African areas by Latif (43) and Dreyer et al. (44) have all indicated that Anaplasma marginale is widely distributed with prevalence rates ranging from 32.1 to 100%. Similarly, high prevalences of Anaplasma antibodies were reported in several studies in South Africa using ELISA (45, 46) with prevalences of 87, 98.2%, respectively. In contrast to our findings, lower seroprevalence of 32.1–40.0% were reported in several countries in East Africa such as Kenya (43, 47), Tanzania (48) and a much lower prevalence of 15% at wildlife-livestock interface areas in Uganda (49).
Babesia begimina was the main Babesia species found in the present study. The higher prevalence of B. bigemina compared to B. bovis may be explained by (i) wider vector range of B. bigemina (50), (ii) a higher concentration of the B. bigemina parasite in the capillary and veins than the B. bovis parasite which is evenly distributed in the whole blood vasculature (51, 52) (iii) tick infection rates are usually higher for B. bigemina (0.23%) than in B. bovis (0.04%) (53), with a consequent slower rate of transmission of the latter to cattle. Taken together, this would also suggest that, in an area where both species are present, endemic stability would be more likely to establish for B. bigemina compared to B. bovis (54). It is inconclusive whether physical separation between wildlife and cattle affected Babesia infection in cattle owing to the findings of the current study where B. bigemina infection was higher in Chobe west and B. bovis was higher in Maun west.
Theileria parva has been described in African buffalo in several locations in the region (55–59), including northern Botswana (2). The presence of T. parva in the buffalo population suggests that buffalo may serve as a reservoir hosts for either mechanical or biological transmission to cattle particularly where there is shared pasture and water at the wildlife-livestock interface. Thus, it was hypothesized that the prevalence of T. parva would be higher in Chobe west with a higher level of interaction between cattle and buffalo as described in South Africa (40). However, our findings do not support this hypothesis. The sample size was too small to warrant such a conclusion particularly given the low observed seroprevalence of 2.4%. The low seroprevalence could be due to a low infection challenge from ticks or that there are few ticks which could act as competent transmitters of the organism in the area. Furthermore, T. parva being transmitted transtadially; it is possible that infection was lost during the transmission process (60). Other possible explanations for the low seroprevalence may be that since the disease is fatal in cattle, it is difficult to find animals with antibodies that survived the infection. We recommend that confirmatory polymerase chain reaction (PCR) be carried out on seropositive samples similar to tests done on samples from buffalo by Eygelaar et al. (2).
The high Anaplasma infection prevalence suggests a situation of endemic stability of the pathogen in the cattle population. Clinical disease outbreaks in a population in which a pathogen has reached endemic stability are rare and clinical anaplasmosis is uncommon in both cattle populations investigated in the current study. B. Bigemina, B. bovis, and T. parva occur at prevalence level inconsistent with endemic stability and thus, animals in the population are susceptible to development of clinical disease. Cattle in Maun west and Chobe west are therefore, at risk of bovine babesiosis and theileriosis. It is worth-noting that although, endemic stability mitigates disease outbreaks, it is not reliable. Moreover, using seroprevalence alone does not give a clear indication of endemic stability status of TBDs in an area. Future studies with more data are necessary to make a comprehensive analysis of endemic stability of TBDs in the study sites. Additionally, it will be important to determine which strain of Anaplasma is endemic in the study sites and if possible, to detect tick borne pathogens determined in this survey by molecular methods directly in tick samples.
Overall, tick diversity and abundance observed in this study correlated with the seroprevalence of the selected tick borne pathogens.
Conclusion
The results of this study provide the evidence of the important tick borne diseases pathogens and vectors responsible for their transmission in two important wildlife-livestock interface areas in northern Botswana. Our results suggest that irrespective of the type of interface (fenced or not fenced), ticks and TBD pathogens (Anaplasma spp., B. bovis, B. bigemina, and T. parva) are circulating in the two study areas with Anaplasma spp. being the most prevalent and A. variegatum and R. evertsi evertsi and R. (B.) decolaratus being the principal tick species infesting cattle in the study areas. Proper control measures against ticks and TBDs are necessary to improve cattle productivity and the livelihood of farmers in Maun west and Chobe west. Further epidemiological studies are needed to determine seasonal patterns of tick burdens and TBDs circulation in cattle and determine the economic impact of TBDs transmitted by the identified pathogens. Also to further clarify the relationship between livestock farming and wildlife conservation at the wildlife-livestock interface.
Data Availability Statement
The datasets generated for this study are available on request to the corresponding author.
Ethics Statement
Ethical review and approval was not required for the animal study because the sampling of cattle did not require any ethical approval. However, the project was discussed, approved by Department of Veterinary Services (Ministry of Agriculture), and undertaken with their direct collaboration in the field activities. Import permit was obtained from DVS for the movement of blood samples outside the foot and mouth disease infected area to targeted laboratories. Written informed consent was obtained from the owners for the participation of their animals in this study.
Author Contributions
OR, SR, FJ, and LG designed the study. SR contributed cELISA reagents, testing, and analysis of test results. OR collected samples, identified ticks, and conducted serological test using cELISA. OR and FJ analyzed the data. LG assisted with production of maps. OR and SR prepared the first version of the manuscript. FJ and LG corrected and improved subsequent versions. All authors read and approved the final manuscript.
Disclaimer
The contents of this publication are the sole responsibility of the authors and can in no way be taken to reflect the views of the European Union.
Conflict of Interest
The authors declare that the research was conducted in the absence of any commercial or financial relationships that could be construed as a potential conflict of interest.
Acknowledgments
The authors wish to express their appreciation to the personnel from the Veterinary Services of Botswana for facilitating staff, logistics, for this work. We are equally grateful to the farmers in Maun west and Chobe west for permission to collect samples from their animals and their assistance during sampling. This work was implemented under the framework of the GeosAf project (rp-pcp.org/_projects/) implemented by the ACP Group of States. This publication has been produced with the assistance of the European Union. We also thank the FSPI CAZCOM—Improve the Capacities of Zimbabwe for the Control of animal and zoonotic diseases—project to support this study. This work was also conducted within the framework of the Research Platform ≪Production and Conservation in Partnership≫ (www.rp-pcp.org).
References
1. Kocan KM, de la Fuente J, Blouin EF, Coetzee JF, Ewing SA. The natural history of A. marginale. Vet Parasitol. (2010) 167:95–107. doi: 10.1016/j.vetpar.2009.09.012
2. Eygelaar D, Jori F, Mokopasetso M, Sibeko KP, Collins NE, Vorster I, et al. Tick-borne haemoparasites in African buffalo (Syncerus caffer) from two wildlife areas in Northern Botswana. Parasit Vectors. (2015) 8:26. doi: 10.1186/s13071-014-0627-y
3. Kopp N, Diaz D, Amacker M, Odongo DO, Beier K, Nitsch C, et al. Identification of a synthetic peptide inducing cross-reactive antibodies binding to Rhipicephalus (Boophilus) decoloratus, Rhipicephalus (Boophilus) microplus, Hyalomma anatolicum anatolicum and Rhipicephalus appendiculatus BM86 homologues. Vaccine. (2010) 28:261–9. doi: 10.1016/j.vaccine.2009.09.085
4. Kivaria FM. Estimated direct economic costs associated with tick-borne diseases on cattle in Tanzania. Trop Anim Health Prod. (2006) 38:291–9. doi: 10.1007/s11250-006-4181-2
5. Spickett AM, Heyne IH, Williams R. Survey of the livestock ticks of the North West province, South Africa. Onderstepoort J Vet Res. (2011) 78:1–12. doi: 10.4102/ojvr.v78i1.305
6. Naidoo R, Du Preez P, Stuart-Hill G, Jago M, Wegmann M. Home on the range: factors explaining partial migration of African Buffalo in a tropical environment. PLoS ONE. (2012) 7:e36527. doi: 10.1371/journal.pone.0036527
7. Caron A, Miguel E, Gomo C. Relationship between burden of infection in ungulate populations and wildlife/livestock interfaces. Epidemiol Infec. (2013) 141:1522–35. doi: 10.1017/S0950268813000204
8. Bengis R, Kock R, Fischer J. Infectious animal diseases: the wildlife/livestock interface. Rev Sci Tech. (2002) 21:53–66. doi: 10.20506/rst.21.1.1322
9. Kock R. What is this infamous, wildlife/livestock interface? A review of current knowledge. In: Osofsky S, Cleaveland S, Karesh WB, editors. Conservation and Development Interventions at the Wildlife/Livestock Interface: Implications for Wildlife, Livestock and Human Health. Cambridge: IUCN.ike (2005). p. 1–13.
10. Norval RAI, Perry BD, Young AS. The Epidemiology of Theileriosis in Africa. London: Academic Press (1992).
11. Andrew HR, Norval RAI. The carrier status of sheep, cattle and African buffalo recovered from heartwater. Vet Pathol. (1989) 34:261–6. doi: 10.1016/0304-4017(89)90056-3
12. Iseki H, Alhassan A, Ohta N, Thekisoe OMM, Yokoyama N, Inoue N, et al. Development of a multiplex loop-mediated isothermal amplification (mLAMP) method for the simultaneous detection of bovine Babesia parasites. J Microbiol Methods. (2007) 71:281–7. doi: 10.1016/j.mimet.2007.09.019
13. Espinaze MPA, Hellard E, Horak IG, Cumming GS. Domestic mammals facilitate tick-borne pathogen transmission networks in South African wildlife. Biol Conserv. (2018) 221:228–36. doi: 10.1016/j.biocon.2018.03.017
14. Jori F, Brahmbhatt D, Fosgate G, Thompson PN, Budke C, Ward MP, et al. A questionnaire-based evaluation of the veterinary cordon fence separating wildlife and livestock along the Kruger National Park, South Africa. Prev Vet Med. (2011) 100:210–20. doi: 10.1016/j.prevetmed.2011.03.015
15. Jori F, Etter E. Transmission of foot and mouth disease at the wildlife/livestock interface of the Kruger National Park, South Africa: can the risk be mitigated? Prev Vet Med. (2016) 126:19–29. doi: 10.1016/j.prevetmed.2016.01.016
16. Londt JGH, Horak IG, de Villiers IL. Parasites of domestic and wild animals in South Africa. XIII The seasonal incidence of adult ticks (Acarina: Ixodidae) on cattle in the northern Transvaal Onderstepoort. J Vet Res. (1979) 46:31–9.
17. Walker AR, Bouattour A, Camicas JL, Estrada-Peña A, Horak IG, Latif AA, et al. Ticks of Domestic Animals in Africa: A Guide to Identification of Species. Biosciences Reports. Atalanta Houten, Netherlands (2003).
18. Marufu MC. Prevalence of ticks and tick-borne diseases in cattle on communal rangelands in the highland areas of the Eastern Cape Province (MSc thesis). University of Fort Hare, Alice, TX, United States (2010).
19. Molloy JB, Bowles PM, Knowles DP, McElwain TF, Bock RE, Kingston TG, et al. Comparison of a competitive inhibition ELISA and the card agglutination test for detection of antibodies to Anaplasma marginale and Anaplasma centrale in cattle. Aust Vet J. (1999) 77:245–9. doi: 10.1111/j.1751-0813.1999.tb11712.x
20. Humphry RW, Cameroon A, Gunn GJ. A practical approach to calculate sample size for herd prevalence surveys. Prev Vet Med. (2004) 69:1–6. doi: 10.1016/j.prevetmed.2004.07.003
21. Burridge MJ, Kimber CD. The indirect fluorescent antibody test for experimental East Coast fever (Theileria parva infection of cattle): evaluation of a cell culture schizont antigen. Res Vet Sci. (1972) 13:451–5. doi: 10.1016/S0034-5288(18)33992-4
22. Goddeeris BM, Katende JM, Irvin AD, Chumo RS. Indirect fluorescent antibody test for experimental and epizootiological studies on East coast fever (Theileria parva infection in cattle). Evaluation of a cell culture schizont antigen fixed and stored in suspension. Res Vet Sci. (1982) 33:360–5. doi: 10.1016/S0034-5288(18)32316-6
23. Morzaria SP, Brocklesby DW, Harradine DL. Evaluation of the indirect fluorescent antibody test for Babesia major and Theileria mutans in Britain. Vet Rec. (1977) 100:484–7. doi: 10.1136/vr.100.23.484
24. SAS. Statistical Analysis System Institute Inc. Users Guide, Version 9. Carry, NC, United States (2003).
25. Musuka GN, Mafika M, Molathwa E, Nuru H. New distribution records of Amblyomma hebraeum in the sandveld region of Botswana. J S Afr Vet Assoc. (2001) 72:3. doi: 10.4102/jsava.v72i1.600
26. Paine GD. Ticks (Acari: Ixodoidea) in Botswana. Bull Entomol Res. (1982) 72:1–16.doi: 10.1017/S0007485300050239
27. Walker JB, Mehlitz D, Jones GE. In Notes on the Ticks of Botswana. GTZ. 27, 33, 39–43, 55, 65 (1978).
28. Tesgera T, Regassa F, Giro B, Mohammed A. Study on prevalence and identification of ixodid ticks in cattle in Gursum district, East Hararghe Zone of Oromia Regional State, Ethiopia. J Parasitol Vector Biol. (2017) 9:27–33. doi: 10.5897/JPVB2016.0270
29. Tessema T, Gashaw A. Prevalence of ticks on local and crossbred cattle in and around Asella town, southeast Ethiopia. Ethiop Vet J. (2010) 14:79–89. doi: 10.4314/evj.v14i2.63886
30. Ikpeze OO. Dermatophilus infection in Nigeria: a mini-review. Bio Res. (2004) 2:37–41. doi: 10.4314/br.v2i2.28558
31. Walker JB, Keirans JE, Horak IG. The Genus Rhipicephalus (Acari, Ixodidae). A Guide to the Brown Ticks of the World. Cambridge: Cambridge University Press (2000). doi: 10.1023/A:1005237804239
32. Cumming GS. Comparing climate and vegetation as limiting factors for species ranges of african ticks. Ecology. (2002) 83:255–68. doi: 10.1890/0012-9658(2002)083[0255:CCAVAL]2.0.CO;2
33. Horak IG, Nyangiwe N, DeMatos C, Neves L. Species composition and geographic distribution of ticks infesting cattle, goats and dogs in a temperate and in a subtropical region of south-east Africa. Onderstepoort J Vet Res. (2009) 76:263–76. doi: 10.4102/ojvr.v76i3.28
34. Yawa M, Nyangiwe N, Muchenje V, Marufa MC. Ecological preferences and seasonal dynamics of ticks (Acari: Ixodidae) on and off bovine hosts in the Eastern Cape Province, South Africa. Exp Appl Acarol. (2018) 74:317–28. doi: 10.1007/s10493-018-0234-2
35. Estrada-Pena A. Climate, niche, ticks, and models: what they are and how we should interpret them. Parasitol Res. (2008) 103:87–95. doi: 10.1007/s00436-008-1056-7
36. Leta S, De Clercq EM, Madder M. High-resolution predictive mapping for Rhipicephalus appendiculatus (Acari: Ixodidae) in the Horn of Africa. Exp Appl Acarol. (2013) 60:531–42. doi: 10.1007/s10493-013-9670-1
37. Geeta P, Daya S, Amit KJ, Vikrant S, Santosh KV. Prevalence and seasonal variation in ixodid ticks on buffaloes of Mathura District, Uttar Pradesh, India. J Parasit Dis. (2013) 34:1–7. doi: 10.1007/s12639-012-0154-8
38. Nyangiwe N, Horak IG. Goats as alternative hosts of cattle ticks. Onderstepoort J Vet Res. (2007) 74:1–7. doi: 10.4102/ojvr.v74i1.133
39. Fyumagwa RD, Mdaki ML, Soombe C, Nyaki A. African buffalo is an important resrvoir of Anaplasma bovis in Ngorongoro Crater Tanzania. Res opin anim vet sci. (2013) 3:105–10.
40. Potgieter FT, Stoltsz WH. Bovine anaplasmosis. In: Coetzer JAW, Tustin RC, editors. Infectious Diseases of Livestock. 2nd ed. Vol. 1. Cape Town: Oxford University Press (2004) 594–616.
41. Aubry P, Geale DW. A review of bovine anaplasmosis. Transbound Emerg Dis. (2011) 58:1–30. doi: 10.1111/j.1865-1682.2010.01173.x
42. Ramabu SS, Kgwatalala PM, Nsoso SJ, Gasebonwe S. Anaplasma infection prevalence in beef and dairy cattle in the south east region of Botswana. Vet Parasitol. (2018) 12:4–8. doi: 10.1016/j.vprsr.2017.12.003
43. Latif AA. An epidemiological study of tick-borne diseases and their effect on productivity of Zebu cattle under traditional management on Rusinga Island, Western Kenya. Prev Vet Med. (1995) 22:169–81. doi: 10.1016/0167-5877(94)00408-B
44. Dreyer K, Fourie LJ, Kok DJ. Epidemiology of tick-borne diseases of cattle in Botshabelo and Thaba Nchu in the Free State Province. Onderstepoort J Vet Res. (1998) 65:288–9.
45. Mbati PA, Hlatshwayo M, Mtshali MS, Mogaswane KR, Waal TD, Dipeolu OO. Ticks and tick-borne diseases of livestock belonging to resource-poor farmers in eastern Free State of South Africa. Exp Appl Acarol. (2002) 28:217–24. doi: 10.1023/A:1025306701803
46. Ndou RV, Diphahe TP, Dzoma BM, Motsei LE. The seroprevalence and endemic stability of Anaplasmosis in cattle around Mafikeng in the North West Province, South Africa. Vet Res. (2010) 3:1–3. doi: 10.3923/vr.2010.1.3
47. Okuthe OS, Buyu GE. Prevalence and incidence of tick-borne diseases in smallholder farming systems in the Western-Kenya highlands. Vet Parasitol. (2006) 142:307–12. doi: 10.1016/j.vetpar.2006.05.016
48. Swai ES, French NP, Beauchamp G, Fitzpatrick JL, Bryant M J, Kambarage D, Ogden NH, et al. A longitudinal study of sero-conversion to tick-borne pathogens in smallholder dairy youngstock in Tanzania. Vet Parasitol. (2005) 131:129–37. doi: 10.1016/j.vetpar.2005.04.02
49. Kabuusu RM, Alexander R, Kabuusu AM, Muwanga SN, Atimnedi P, Macpherson C. Effects of wildlife-livestock interface on the prevalence of intra-ertythrocytic haemoparasites in cattle. Open J Vet Med. (2013) 3:315–8. doi: 10.4236/ojvm.2013.38051
50. Walker AR, Bouattour A, Camicas JL, Estrada-Peña A, Horak IG, Latif AA, et al. Ticks of domestic animals in Africa; a guide to identification of species. In: International Consortium of Tick Borne Diseases (ICTTD- 2). (Edinburgh) (2013). p. 221.
51. Rahman WA, Lye YP, Chandrawathani P. The seroprevalence of Bovine Babesiosis in Malaysia. Trop Biomed. (2010) 27:301−7.
52. Lemma F, Girma A, Demam D. Prevalence of Bovine babesiosis in and around Jimma Town South Western Ethiopia. Adv Biol Res. (2016) 10:37–42. doi: 10.5829/idosi.apg.2017.96.100
53. Mahoney DF, Mirre GB. Bovine babesias: estimation of infection rates in the tick vector Boophilus microplus (Canestrini). Ann Trop Med Parasit. (1971) 65:309–17. doi: 10.1080/00034983.1971.11686759
54. Bock R, Jackson L, de Vos A, Jorgensen W. Babesiosis of cattle. Parasitology. (2004) 129:247–69. doi: 10.1017/S0031182004005190
55. Oura CAL, Bishop RP, Wampande EM, Lubega GW, Taita A. Application of a reverse line blot assay to the study of haemoparasites in cattle in Uganda. Int J Parasitol. (2004) 34:603–13. doi: 10.1016/j.ijpara.2003.12.012
56. Pienaar R, Potgieter FT, Latif AA, Thekisoe OMM, Mans BJ. Mixed Theileria infections in free-ranging buffalo herds: implications for diagnosing Theileria parva infections in Cape buffalo (Syncerus caffer). Parasitology. (2011) 138:884–95. doi: 10.1017/S0031182011000503
57. Chaise ME, Sebeko KP, Collins NE, Potgieter FT, Oosthuizen MC. Identification of Theileria parva and Theileria species (buffalo) 18 S Rrna gene sequence variants in the African Buffalo (Syncerus caffer) in southern Africa. Vet Parasitol. (2011) 182:150–62. doi: 10.1016/j.vetpar.2011.05.041
58. Debeila EM. Occurrence of Anaplasma and Ehrlichia species in African Buffalo (Syncerus caffer) in Kruger National Park and Hluhluwe-iMfolozi Park in South Africa (MSc thesis). (2012). University of Pretoria, Pretoria, South Africa.
59. Oura CAL, Tait A, Asiimwe B, Lubega GW, Weir W. Haemoparasite prevalence and Theileria parva strain in Cape buffalo (Syncerus caffer) in Uganda. Vet Parasitol. (2011) 175:212–9. doi: 10.1016/j.vetpar.2010.10.032
Keywords: tick borne diseases, Anaplasma spp., Babesia spp., T. parva, seroprevalence, ticks, veterinary fence, Botswana
Citation: Raboloko OO, Ramabu SS, Guerrini L and Jori F (2020) Seroprevalence of Selected Tick Borne Pathogens and Diversity and Abundance of Ixodid Ticks (Acari: Ixodidae) at the Wildlife-Livestock Interface in Northern Botswana. Front. Vet. Sci. 7:187. doi: 10.3389/fvets.2020.00187
Received: 27 January 2020; Accepted: 23 March 2020;
Published: 05 May 2020.
Edited by:
Francisco Ruiz-Fons, Spanish National Research Council, SpainReviewed by:
A. Sonia Olmeda, Complutense University of Madrid, SpainFrancois Frederick Maree, Agricultural Research Council of South Africaf (ARC-SA), South Africa
Copyright © 2020 Raboloko, Ramabu, Guerrini and Jori. This is an open-access article distributed under the terms of the Creative Commons Attribution License (CC BY). The use, distribution or reproduction in other forums is permitted, provided the original author(s) and the copyright owner(s) are credited and that the original publication in this journal is cited, in accordance with accepted academic practice. No use, distribution or reproduction is permitted which does not comply with these terms.
*Correspondence: Obuile O. Raboloko, b29yYWJvbG9rb0BnbWFpbC5jb20=