- 1Kyushu Research Station, National Institute of Animal Health, NARO, Kagoshima, Japan
- 2Viral Disease and Epidemiology Research Division, National Institute of Animal Health, NARO, Tsukuba, Japan
Epizootic congenital abnormalities caused by Akabane, Aino, and Chuzan viruses have damaged the reproduction of domestic ruminants in East Asia for many years. In the past, large outbreaks of febrile illness related to bovine ephemeral fever and Ibaraki viruses severely affected the cattle industry in that region. In recent years, vaccines against these viruses have reduced the occurrence of diseases, although the viruses are still circulating and have occasionally caused sporadic and small-scaled epidemics. Over a long-term monitoring period, many arboviruses other than the above-mentioned viruses have been isolated from cattle and Culicoides biting midges in Japan. Several novel arboviruses that may infect ruminants (e.g., mosquito- and tick-borne arboviruses) were recently reported in mainland China based on extensive surveillance. It is noteworthy that some are suspected of being associated with cattle diseases. Malformed calves exposed to an intrauterine infection with orthobunyaviruses (e.g., Peaton and Shamonda viruses) have been observed. Epizootic hemorrhagic disease virus serotype 6 caused a sudden outbreak of hemorrhagic disease in cattle in Japan. Unfortunately, the pathogenicity of many other viruses in ruminants has been uncertain, although these viruses potentially affect livestock production. As global transportation grows, the risk of an accidental incursion of arboviruses is likely to increase in previously non-endemic areas. Global warming will also certainly affect the distribution and active period of vectors, and thus the range of virus spreads will expand to higher-latitude regions. To prevent anticipated damages to the livestock industry, the monitoring system for arboviral circulation and incursion should be strengthened; moreover, the sharing of information and preventive strategies will be essential in East Asia.
Introduction
Arthropod-borne (arbo-) viruses are transmitted by hematophagous arthropods. The arboviruses include various taxonomic groups, such as Rhabdoviridae, Nairoviridae, Peribunyaviridae, Phenuiviridae, Orthomyxoviridae, Flaviviridae, Togaviridae, Reoviridae, and Asfarviridae (1, 2). They cause a broad spectrum of illness ranging from asymptomatic to severe and fatal disease in vertebrate hosts. Because some of the arboviruses have affected human and domestic animal health, the efforts to prevent and control the diseases caused by arboviruses continue, but novel arboviruses have continuously emerged and have sometimes been involved in human and animal diseases. The battle thus seems to have no end.
Bluetongue virus (BTV; the genus Orbivirus, the family Reoviridae) is probably the most well-known arbovirus affecting livestock ruminant productions in the world (3). The virus causes severe illness in ruminants and its presence can act as a barrier for international trades of animals and animal products. Since end of the last century, the global range of BTV has remarkably changed (4). The incursion of BTV serotype 8 into northern Europe caused large economic damage to the livestock industry. Another important arbovirus, epizootic hemorrhagic disease virus (EHDV; the genus Orbivirus), also has been regarded as a threat to European counties in recent years, because its outbreaks has been reported in the countries bordering the region (3, 5). The ruminant diseases caused by both viruses have been listed as notifiable terrestrial animal diseases by World Organization for Animal Health (OIE) and well-investigated through the world. In addition, recent appearance of an emerging arbovirus, Schmallenberg virus (SBV), in northern Europe (6) has caught the world's attention (7). However, many other arboviruses, which are not listed by OIE, also have been involved in ruminant illness and their epizootic might have severely impacted on the livestock industry in each region. Unfortunately, the situation of arbovirus infections of ruminant livestock remains uncertain in many parts of the world.
East Asia, i.e., the eastern subregion of Asia, has the largest human population in the world, and the livestock industry is an important component of the lives of the region's populations. The estimated global distribution data for livestock animals indicated that East Asia is one of the regions with the highest population density of domestic ruminants in the world (8). Significant concern about the health and safety of livestock for consumption has thus been expressed. Unfortunately, along with other important diseases, a variety of arbovirus infections has probably damaged the production of domestic ruminants (Tables 1, 2), although there is insufficient statistical data at this time to draw any conclusion.
Akabane disease and bovine ephemeral fever (BEF) are still problematic in East Asia, despite the widespread use of vaccines against them. Outbreaks of other endemic arbovirus infections such as Aino virus infection and Ibaraki disease have occasionally been reported. In addition, recent progress in the research tools for virus hunting have enhanced the detection of the circulation of arboviruses that were previously unrecognized in East Asia (Table 1). Notably, some of the arboviruses are suspected of being associated with ruminant diseases such as epizootic congenital abnormalities, febrile illness, and neurological disorders (Table 3). Many reports about arboviruses infecting domestic ruminants have been provided from mainland China, Japan, the Republic of Korea, and Taiwan, which are part of East Asia. This review provides a compilation of the available knowledge about the arboviruses that are associated with domestic ruminants in the East Asia region at present. Gaps in the research that remain to be addressed and future research directions are also discussed.
History of Endemic and Emerging Arboviruses Affecting Domestic Ruminants
Bovine Ephemeral Fever
BEF is characterized by sudden fever, anorexia, depression, and ananastasia, and it can be regarded as the first recognized arbovirus infection in domestic ruminants in East Asia. Due to the cessation of lactation in dairy cattle and the loss of condition in beef cattle, BEF has had considerable economic impact (9). The etiological virus is bovine ephemeral fever virus (BEFV; species Bovine fever ephemerovirus, genus Ephemerovirus, family Rhabdoviridae). The virus was widely distributed through Africa, the Middle East, Asia, and Australia. Phylogenetic analysis based on the surface glycoprotein (G) gene demonstrated that BEFV isolates are grouped into four lineages with different geographical origins: Africa, the Middle East, Asia, and Australia (9, 10). Recently, several BEFV isolates clustered into Asian lineage have been appeared in Turkey and Iran, indicating its expansion to the westward (11, 12). Outside of East Asia, large BEF epizootics have been reported in Israel, Turkey, and Australia (11, 13, 14).
Old records indicated that epidemics of BEF occurred in the central and western parts of Japan in the late nineteenth century (15). Between 1949 and 1951, ~700,000 cases and 10,000 deaths were reported in the same region (16). The disease was initially called “bovine epizootic fever,” but the etiological virus was later identified as BEFV based on the serological relationship between the Japanese isolate and BEFVs isolated in Australia and South Africa (17). Large- and medium-scale outbreaks of BEF repeatedly occurred in Japan until 1989 (18, 19). Since then, the epidemics had been limited to the southwestern islands of Japan, which are close to Taiwan (20, 21). However, in 2015 a reoccurrence of BEF was reported in the southern end of Kyushu, which is one of the main islands of Japan (22).
From 1955 to 2011, BEF epidemics were often recorded in mainland China (23). China's nationwide sero-surveillance in cattle between 2012 and 2014 demonstrated that the range of BEFV covered most of the area of mainland China (24). Epidemics of BEF have also occurred in Korea, and some of them were probably linked to those in Japan (19). Meteorological analysis demonstrated that a low-jet stream blew from mainland China, along the Korean Peninsula, to western Japan at the peak of BEF epidemic in China and Korea in 1988 and 1991 (19, 25, 26). The stream probably brought the infected vectors and contributed to the BEF expansion to Japan. The seroprevalence of BEFV without clinical cases was demonstrated in Korea between 2009 and 2012 (27). Several intervals of BEF epidemic have been documented in Taiwan between 1967 and 2014 (28).
Although the mortality rate of BEF is known to be <1% (9), a high case-fatality rate due to a novel genogroup of BEFV was observed in several recent outbreaks in mainland China and Taiwan (23, 29). This novel genogroup of BEFV was first isolated in mainland China in 2011 and was then detected in Taiwan and Japan 2 and 4 years later, respectively (22, 23, 30). These reports indicate that the virus rapidly spread in East Asia. The impact of BEF caused by the novel genogroup on dairy and beef production should thus be evaluated.
At laboratory diagnosis, the virus neutralization test (VNT) is generally used to detect rising titers of neutralization antibody in paired acute and convalescent sera from affected cattle which show the typical clinical signs of BEF (14). For serological detection of BEFV, an indirect-ELISA with the recombinant protein of BEFV G gene was also developed (31). In recent years, conventional RT-PCRs targeting the G gene was available to detect BEFV in the diseased cattle (32–34). Real-time RT-PCR and reverse-transcription, loop-mediated isothermal amplification (LAMP) assays were also established for rapid and sensitive detection of BEFV gene (32, 35, 36).
Vaccination is now the current gold standard to prevent BEF. Commercial attenuated and killed vaccines have been developed and widely used in Japan, Korea, China and Taiwan (23, 27, 33, 37–39). Repetitive outbreaks of BEF in East Asia indicate that continuous vaccination program is essential to provide sufficient immunity against BEFV among naïve population and minimize economical loss caused by BEF. Antigenic association between the vaccine strains and recent field isolates of BEF should be assessed to guarantee the vaccine efficacy.
Ibaraki Disease
During a large epidemic of an acute febrile disease in 1959 and 1960 in Japan, deglutitive difficulty based in the esophageal and laryngopharyngeal musculature was observed among affected cattle (40). A total of 43,793 cases and 4,298 deaths were reported during the epidemic. That febrile disease was at one time called “bluetongue-like disease” because its clinical signs resembled those caused by BTV. The etiological agent was isolated from naturally and experimentally infected cattle and was designated as Ibaraki virus (IBAV), which denotes the location from which it was isolated (40, 41). The clinical signs of IBAV were exhibited by the experimentally infected cattle. IBAV was later identified as a strain of EHDV serotype 2 (EHDV-2) based on a virus neutralization test (42). Smaller outbreaks were reported in southern Japan in 1982 and 1987 (43–45), but no case report was known between 1988 and 2012. A resurgence of IBAV was observed in southern Japan in 2013, although only a few cattle showed the typical symptoms (46).
An occurrence of Ibaraki disease was recognized in Korea in 1982, when the epidemic was observed in southern Japan (47). In Taiwan, IBAV was initially obtained in 1990 from the cerebellum of a calf with non-suppurative encephalitis, and an occurrence of Ibaraki disease was observed at several farms in the same year (48). IBAV was also isolated from the affected cattle and from asymptomatic cattle from the same cowsheds where the affected cattle were raised. The seroprevalence (25–85%) of IBAV in dairy cattle was revealed in Taiwan between 1987 and 1990. There is no available information about Ibaraki disease outside of the above-mentioned regions.
At laboratory diagnosis, detection of neutralization antibody to IBAV and virus isolation from diseased cattle are usually tested (41, 43–46). IBAV-specific RT-PCR based on segment 2 is available to detect the virus from infected cattle (46). A real-time RT-PCR assay for typing of EHDV would be able to detect the presence of IBAV in diagnostic samples (49). Currently, commercial attenuated and killed vaccines to IBAV are prepared in Japan (50, 51).
Akabane Disease
A large outbreak of abortions, premature births, still births, and congenital malformations in calves was reported in Japan between 1972 and 1975 (52). The number of affected calves reached ~42,000 during the outbreak. The malformed calves had congenital lesions characterized by arthrogryposis-hydranencephaly (A-H) syndrome (53, 54). A serological survey of affected calves demonstrated that Akabane virus (AKAV; species Akabane orthobunyavirus, genus Orthobunyavirus, family Peribunyaviridae) was a causative agent of the disease (54). The virus is widely distributed in Africa, the Middle East, Asia and Australia (55) and severe outbreaks of Akabane disease have sometimes occurred in Japan, Australia, and Israel (52, 56–59).
Although AKAV was first isolated in 1959 from mosquitoes (60), its relationship with ruminant disease had been unknown until the outbreak. The etiology of AKAV in bovine and caprine fetuses was demonstrated by experimental infections of pregnant cows and goats (61, 62). Stillbirths and congenital malformations of neonate lambs caused by AKAV were observed in northern Japan between late 1985 and early 1986 (63). The isolation of AKAV from Culicoides biting midges, cattle, and a piglet has also been successful (64–67). In 1984, AKAV was isolated from a postnatally infected calf with encephalitis during a small outbreak in Japan; it was a suspected causative agent (68).
Outbreaks of encephalomyelitis caused by postnatal AKAV infection occurred in 2006 and 2011 with 180 and 165 deaths, respectively (67, 69). The AKAVs isolated in Japan since 1959 are clustered into two genogroups (I and II) (67, 70). Genogroup I AKAVs have frequently been detected and/or isolated from affected cattle with encephalomyelitis (67, 69). These isolates probably have high neurotropic and/or neurovirulence and cause the neurological disorders in cattle. Recent monitoring in Japan revealed that the frequent incursion of genogroup I AKAVs increased the number of postnatal encephalitis cases (65). There have been repeated epidemics of Akabane disease including both in utero and postnatal infections in Japan (52, 67).
The first recognition of Akabane disease in Korea was in 1979 (71). Several AKAV isolates were then obtained from aborted fetuses, affected cattle with neurological disorders, and sentinel cattle between 1993 and 2010 (72–75). Serological surveillance of domestic ruminants revealed that frequent incursions of AKAV had occurred, and the virus occasionally spread widely (27, 76–78). Ruminants affected by AKAV have been reported since the first recognition (52, 79). In 2010, a large outbreak of epizootic encephalomyelitis in postnatally infected cattle also occurred in the southern part of Korea (74). The causative AKAV isolates were classified into genogroup I (75). Before that outbreak, several sporadic cases of bovine encephalomyelitis were reported in 2000 and 2001 (80).
In 1992, AKAV was isolated in Taiwan from affected calves with non-suppurative encephalitis and healthy calves (81). The 307 sera collected from 341 affected calves with encephalitis in 1989 and 1990 contained the specific antibodies to AKAV (82). A high seroprevalence (94.3%) of AKAV was also observed in 1994 (82). Since 1998, AKAV has been isolated from mosquitoes, bamboo rats (Rhizomys pruinosus), and a goat in the southern part of mainland China (83–87). Large-scale serological surveillance demonstrated that AKAV infection was relatively common in cattle and sheep in most of mainland China (83). However, no detailed description of AKAV outbreaks in mainland China can be obtained from published references, and the impacts of AKAV on the Chinese livestock industries remain unknown.
Diagnosis of Akabane disease is usually conducted by VNT for detection of specific antibodies to AKAV in fetal fluids or precolostrum sera of malformed newborns (55). ELISAs were also established for serological tests to detect antibodies to AKAV (88, 89) and the commercial ELISA kits are often used for the diagnosis and serological surveillance (90–92). The virus may be isolated from aborted fetuses (93). Because the neutralization antibodies to AKAV probably clear the virus and virus infected cells before the delivery of affected neonates, virus isolation is not successful from them. The virus gene is also unable to be detected from most of the affected calves. However, conventional and real-time RT-PCR assays can provide a sensitive detection of the AKAV-specific gene in central nervous system of the postnatally infected cattle with encephalomyelitis (69, 74, 94). Attenuated and inactivated vaccines against AKAV for breeding cows have been developed (95–97) and commercially provided in Japan and Korea.
Aino Virus Infection
Aino virus (AINOV; species Aino orthobunyavirus, genus Orthobunyavirus) was first isolated from mosquitoes in Japan in 1964 (98). Culicoides biting midges are currently regarded as incriminate vectors for AINOV (65). During the outbreak of bovine congenital abnormalities caused by AKAV in Japan in 1972–1975, the precolostral sera of several affected calves with A-H syndrome contained antibodies to AINOV, but not to AKAV (53). Since then, cases suspected of being associated with AINOV have been sporadically reported in the western part of Japan (99–102). AINOV was also obtained from an aborted bovine fetus with brain lesions (103, 104). These findings indicated that AINOV was involved in abnormal deliveries in the region. Later, teratogenicity characterized by arthrogryposis, hydranencephaly, and cerebellar hypoplasia was demonstrated in neonate calves intrauterinely injected with AINOV (105). Several outbreaks (from 17 to 700 cases) of Aino virus infection in Japan were reported between 1995 and 2006 (52). All known Japanese AINOVs likely belong to a single genogroup, but genetically distant from Australian AINOVs (106–108).
A few cases of Aino virus infection were also reported in Korea, and the virus was isolated from a healthy cow in 1999 (52). The seroprevalence of AINOV was demonstrated in cattle ( ≤ 33.2%) and goats (13.3%) in Korea (27, 77, 78). Although there has been no report of Aino virus infection in China, 10 of 50 AKAV-positive cattle sera collected during the nationwide surveillance of AKAV in mainland China neutralized AINOV (83). A partial genome of AINOV was detected from Culicoides biting midges and mosquitoes collected at dairy farms in Taiwan between 2012 and 2015 (109).
In Australia, AINOV was also isolated (110, 111) and its association with congenital abnormalities was reported (112). Shuni virus, which is closely related with AINOV and distributed in Africa and the Middle East, caused abortions and congenital malformations in sheep, goat, and cattle (113), and neurological disorders in cattle (114).
VNT for precolostral sera of the affected neonates is the most common diagnostic test at present. Commercial inactivated vaccines for breeding cattle are available in Japan and Korea (51, 115).
Chuzan Disease
From the winter of 1985 to the spring of 1986, an epizootic of congenital abnormalities of calves with hydranencephaly-cerebellar hypoplasia (HCH) syndrome occurred in southern Japan (116). Unlike Akabane disease, the affected calves did not show arthrogryposis. Approximately 2,400 affected calves were reported during the epizootic. In the autumn of 1985, a virus classified as a member of the Orbivirus genus was isolated from Culicoides biting midges and bovine blood samples collected in the epizootic region; it was tentatively designated as Chuzan virus (CHUV) (117). The etiological role of CHUV in the epizootic was suggested by serological tests for the affected calves (116). In addition, a newborn calf with HCH syndrome was delivered from a dam experimentally infected with CHUV, and the disease caused by CHUV was named Chuzan disease (118). It was later reported that CHUV is serologically cross-reacted with Kasba virus (KASV), which was isolated in India in 1957 and is a serotype of the species Palyam virus (119). Recent genetical characterization of Palyam virus group also demonstrated the closed relationship between CHUV, KASV, and Vellore virus, which was isolated in India in 1956 (120, 121). After the large 1985–1986 outbreak in southern Japan, the disease has sporadically occurred there.
The isolation of the virus and its seroprevalence (91%) among dairy cattle in Taiwan in 1993 were reported (122). In Korea, the prevalence of CHUV has been recognized since 1993, but only a few sporadic cases of affected calves have been reported in recent years (27, 52, 77). Two isolates of CHUV were obtained from sentinel cattle in the southern part of mainland China in 2012 (123, 124). CHUV was also detected from yaks in the Qinghai-Tibetan Plateau in 2016–2017, although no affected case was reported (125). The impact of CHUV in mainland China remains unclear.
Presence of antibody to CHUV in precolostral serum from abnormal calves indicates its association with lesions in central nervous system (126). For serological diagnosis, VNT is appropriate. Inactivated vaccines against CHUV for breeding cows was developed and are commercially available in Japan and Korea (51, 115, 127).
Bluetongue
Bluetongue is an infectious hemorrhagic disease in domestic ruminants and is caused by BTV, which belongs to the genus Orbivirus and consists of at least 27 serotypes (128). Culicoides biting midges play a principal role in its transmission. The virus is widely distributed through the world. The circulation of a variety of BTV serotypes has been detected in East Asia. In 1974, bovine sera shown to be BTV-positive by a complement-fixation (CF) test were obtained from cattle in southern Japan (129). Thereafter, at least eight BTV serotypes have been found in Japan based on virus isolation and serological surveillance (130–132). In 1994 and 2001, a total of 23 and 45 sheep, respectively, on the same farm in central Japan developed typical symptoms of bluetongue (131). Twenty-three cattle also developed bluetongue in the same region in 1994.
Suspected bluetongue cases in sheep were first reported in the southern part of mainland China in 1974, and the virus was isolated in 1979 during an outbreak of bluetongue-like disease (133, 134). The prevalence of 12 BTV serotypes in mainland China was determined based on virus isolation (135). A probable novel serotype of BTV spread in the northwestern part of China in 2014 (136). Extensive research including risk analyses of BTV spread were conducted in areas with large sheep populations (137–139).
The first BTV isolation in Taiwan was in 2003 (140). The circulation of two BTV serotypes in Taiwan has been identified to date (141). A retrospective study revealed that antibodies to BTV have been present in goat sera in Taiwan since 1989. However, no clinical cases of bluetongue have been observed. In Korea, BTV-1 was isolated from a bovine blood sample collected at an abattoir in 2012 (142), and thereafter, the circulation of BTV was confirmed in goats, deer, and dairy cattle by serological surveillance (143–145). Seven BTV serotypes have been detected in Korea to date. Recently, BTV-3 was also obtained from a blood sample of cattle reared in Korea (146).
The genomic analysis of BTV isolates in East Asia demonstrated that most of them cluster within the eastern BTV topotype from Asia and Australia (132, 135, 146–150). However, a part of segments of Chinese BTV-7 isolate probably originated from those of African BTV isolates which cluster within the western BTV topotype (151). The finding suggests that genetic reassortment events between different topotypes have occurred in regions neighboring to East Asia.
Various serological and molecular assays have been developed and used for laboratory diagnosis (152). Competitive ELISA, VNT, and conventional/real-time RT-PCR assays have been apricated for BTV surveillances in mainland China and Korea (137–139, 141, 143–145). The agar gel immunodiffusion (AGID) test for detection of antibodies to BTV also had been used (131). However, cross-reactions between IBAV and BTV may result in false-positive to BTV-AGID (153). There is no available information for applicable BTV vaccines in East Asia.
Other Orthobunyaviruses
Around the year 2000, the circulation of three orthobunyaviruses (genus Orthobunyavirus) which were new in East Asia was identified. In 1999, Peaton virus (PEAV; species Peaton orthobunyavirus), which was initially isolated in Australia in 1976 (154), was obtained from sentinel cattle and Culicoides biting midges in Japan (155). A stocked virus isolated in 1987 was also identified as PEAV (107). Australian researchers reported that the experimental infection of a pregnant ewe with PEAV induced congenital defects in its lamb (156). Precolostral sera obtained from 31 malformed calves between 1996 and 2016 were positive for PEAV, but negative for known teratogenic viruses (157). These findings suggested that, like AKAV and AINOV, PEAV causes congenital malformations in calves and lambs. Antibodies to PEAV were detected in cattle in mainland China during large-scale sero-surveillance for AKAV (83). The seroprevalence (1.1%) of PEAV among cattle was also demonstrated in Korea during 2013–2015 surveillance (78). In Taiwan, a partial sequence of PEAV was obtained from Culicoides biting midges (109). The PEAV circulation was also observed in Israel (158). During the epizootic, PEAV specific gene was detected from a calf with hydranencephaly (159).
Sathuperi virus (SATV; species Schmallenberg orthobunyavirus) was isolated from mosquitoes in India in 1957, and its prevalence in Nigeria in the 1960s was identified (160, 161). The virus has been isolated from sentinel cattle and Culicoides biting midges since 1999, and an association between SATV and congenital abnormalities in calves was suggested because precolostral sera from several calves with congenital malformations neutralized SATV (162, 163). The seroprevalence (4.9%) of SATV in Korea was also reported (78).
Shamonda virus (SHAV; species Schmallenberg orthobunyavirus) was initially isolated in Nigeria in 1960s (161), and since then there had been no report of it for a long while. In 2002, SHAV suddenly emerged in southern Japan, which is geographically far from Africa (164). Precolostral sera from several malformed calves delivered after the SHAV spread in the epizootic region contained neutralization antibodies to SHAV (164–166). Between the winter of 2015 and the spring of 2016, over 20 malformed calves exposed to intrauterine infection with SHAV were reported in southern Japan (166, 167). Antibodies to SHAV (5.6%) were also detected in Korea, but there is no information regarding its association with abnormal deliveries (78).
The pathogenicity of these orthobunyaviruses is poorly understood, because no experimental infections to cattle successfully developed clinical signs that are observed in the clinical cases in the field. The reported cases that were probably affected by PEAV, SATV, or SHAV were highly sporadic after their epizootic in Japan. The congenital lesions in the brains of calves infected with PEAV or SHAV were milder than those of calves infected with AKAV or AINOV (157, 166). It is probable that the viruses currently circulating in East Asia have relatively low pathogenicity. However, like other segmented viruses, a genetic shift of orthobunyaviruses with three segmented genomes potentially strengthens their virulence to ruminant hosts (168).
It is of interest that the “S” and “L” RNA segments of SBV have a high degree of sequence identity with that of SHAV, but the sequence of the “M” RNA segment of SBV was genetically close to that of SATV (163, 169). In vitro coinfection assays in mammalian and insect cells easily generate reassortants between SATV and SHAV (170). The genetic reassortment might have also occurred among other orthobunyaviruses isolated from ruminants in East Asia and Australia in their evolutional history (70, 107). Although the impacts of PEAV, SATV, and SHAV remain unclear, these viruses should be listed among the teratogenic viruses in ruminants, and their circulation and the suspected cases associated with them should be monitored in East Asia.
Orbiviruses Other Than IBAV, CHUV, and BTV
In 1997, over 1,000 cases of abortion and stillbirths in cattle occurred in southern Japan. A potential etiological agent was isolated from aborted fetuses, sentinel cattle, and Culicoides biting midges and was identified as a strain of EHDV (65, 171, 172). The EHDV strain was initially regarded as a variant of IBAV, because the virus was cross-reacted with IBAV by a virus neutralization test, and the sequence of its segment 2 was reported to show a degree of similarity to that of IBAV (172, 173). Later research demonstrated that this virus belongs to the serotype 7 group of EHDV, based on phylogenetic studies of segment 2 sequences encoding the outermost capsid protein (46, 174). Ibaraki disease-like symptoms (e.g., swallowing difficulty) in cattle were also reported during the epizootic. In 2013, EHDV-7 was also isolated in the southern part of mainland China from sentinel cattle, but its pathogenicity remains unknown (175).
In 2015, an outbreak of febrile illness resembling Ibaraki disease in cattle occurred in a limited part of mainland Japan (176). The EHDV serotype 6 (EHDV-6) gene was detected in all affected cattle by serotype-specific RT-PCR. Although EHDV serotype 1 has been detected and isolated in Japan (46, 174, 177), its association with clinical cases in ruminants has not been demonstrated. A strain of EHDV that was isolated in the southwestern islands of Japan was serologically and genetically distinct from the known EHDV serotypes and was tentatively assigned to the new serotype 10 (174).
D'Aguilar virus (DAGV), which is a member of the species Palyam virus, was first isolated in Australia in 1968 (110). The analysis of stocked viruses revealed that the incursion of DAGV occurred in Japan's southwestern islands in 1987 at the least (178). DAGV spread in southern Japan in the autumn of 2001, and affected calves with Chuzan disease-like symptoms were observed during the following winter and spring. During the epizootic period, no CHUV was isolated from cattle in the affected region. Precolostral sera of these calves clearly neutralized DAGV, suggesting its association with congenital malformations. The virus has been repeatedly isolated in the southern part of mainland Japan and Japan's southwestern Islands (179, 180).
Bunyip Creek virus (BCV), which is another serotype of species Palyam virus, was isolated from cattle and Culicoides biting midges in Japan in 2008 and 2009 (179, 180). There is no evidence that BCV was associated with ruminant disease in Japan or in Australia, where BCV was initially isolated (181).
Yunnan orbivirus (YUOV) was first isolated from mosquitoes collected in the southern part of mainland China in 1997, and it was identified as a member of a new orbivirus species, Yunnan orbivirus, in 2005 (182). A second serotype of Yunnan orbivirus (YOUV-2), which is also called Middle Point virus, was obtained from healthy cattle in Australia in 1998 (183, 184). In addition, another orbivirus related with YOUV was isolated from a blood sample of cattle in the southern part of mainland China in 2015 and was designated as Guangxi orbivirus (185). Interestingly, YOUV was also isolated from cattle, sheep, a donkey, and a dog in Peru in 1997 (186). The infected animals showed neurological signs. Although no evidence of an association between YOUV and animal disease in East Asia has been described, this finding suggests its potential ability to cause neurological disease in domestic ruminants.
Zoonotic Arboviruses
Mosquito-Borne Arboviruses
Several zoonotic arboviruses of importance to human health have been detected from domestic ruminants in East Asia (Table 4). Some of these arboviruses cause clinical signs in both infected humans and ruminants, but the others produce asymptomatic and negligible mild disease in ruminants.
Japanese encephalitis virus (JEV; species Japanese encephalitis virus, genus Flavivirus, family Flaviviridae) caused severe encephalitis not only in humans but also in horses and cattle (187). The JEV infection resulted in abortion and stillbirth in sows and aspermia and disruptions of spermatogenesis in boars (187). Although JEV has spread across a wide area in Japan (188), only a few affected cases of cattle have been sporadically recorded in Japan (189–193).
Outbreaks of West Nile virus (WNV; species West Nile virus, genus Flavivirus) infection have occurred across the Mediterranean region, Eastern Europe, Africa, Asia, and North America (194). Human cases of WNV infection were also reported in western China in 2011, and WNV was simultaneously isolated from mosquitoes in the affected area (195). The WNV gene was detected from domestic pigeons in Korea in 2014 (196). It was recently demonstrated that sporadic cases of severe lymphoplasmacytic meningoencephalitis were associated with WNV in California (197). However, ruminants are not considered hosts severely affected by WNV, and no ruminant illness associated with WNV has been recorded in East Asia.
Banna virus (BAV), which belongs to the species Banna virus of the genus Seadornavirus within the family Reoviridae, was first isolated in mainland China in 1987 from a patient with meningoencephalitis (198, 199). Since then, BAV has been obtained from pigs, cattle, ticks, mosquitoes, and Culicoides biting midges over a wide range of mainland China (200, 201). The virus is thought to cause encephalitis in humans, and many cases associated with it might have been misdiagnosed as Japanese encephalitis (200). At present, there is no evidence of a relationship between BAV and ruminant illness.
Batai virus (BATV), which belongs to the species Batai orthobunyavirus of the genus Orthobunyavirus, has been isolated in Japan and mainland China since 1994 (202–205). BATV is widely distributed in Asia and Europe, and its infection has been observed in cattle, sheep, and goats (202, 206, 207). BATV was reported to cause a mild flu-like illness in humans and mild illness among sheep and goats. At present, no affected case associated with BATV has been reported in East Asia. Ngari virus, which is a possible reassortant between Bunyamwera virus and BATV, has been implicated in human hemorrhagic fever in Africa (202, 208, 209). In the future, BATV may obtain a higher virulence by genetic reassortment with other related orthobunyaviruses. The potential risk of BATV to public health in East Asia should be considered.
Tick-Borne Arboviruses
Four medically important tick-borne arboviruses that infect domestic ruminants are prevalent in East Asia (Table 4). Crimean-Congo hemorrhagic fever virus (CCHFV; species Crimean-Congo hemorrhagic fever orthonairovirus, genus Orthonairovirus, family Nairoviridae) was involved in human clinical cases in the northwestern part of mainland China (210). Although infected domestic ruminants play a crucial role as reservoirs of CCHFV in an endemic cycle of transmission, they do not develop any symptomatic illness after CCHFV infection (211).
Tick-borne encephalitis virus (TBEV; species Tick-borne encephalitis virus, genus Flavivirus, family Flaviviridae) is widely distributed in mainland China, Japan, and Korea (212). This virus sometimes causes severe encephalitis in humans. In Europe, TBEV infection in humans following the consumption of unpasteurized milk and cheese from domestic ruminants has been reported (213). However, the role of domestic ruminants in TBEV endemics in East Asia remains unknown. Another zoonotic tick-borne flavivirus, Kyasanur Forest disease virus (KFDV), was isolated from a febrile patient in the southern part of mainland China in 1989 (214). Although domestic ruminants may be infected with KFDV (215), their role in the viral transmission remains unclear.
Severe fever with thrombocytopenia syndrome virus (SFTSV; species Huaiyangshan banyangvirus, genus Banyangvirus, family Phenuiviridae) was recognized in mainland China, Japan, and Korea (216–218). Although this tick-borne virus has probably been circulating in East Asia for many years, its association with the severe illness in humans was not recognized until 2011. The seroprevalence of SFTSV in domestic ruminants in mainland China, Japan, and Korea was reported (219–222), and epidemiological studies suggested that farmers in rural areas are at risk of SFTSV infection (223). The continuous monitoring for SFTSV in domestic ruminants is therefore necessary to assess the ruminants' contribution to SFTSV endemics in the affected regions.
Compared to mosquito/Culicoides-borne viruses, tick-borne viruses are not likely to expand their range within a short period of time. However, it was suggested that migratory birds play an important role as long-distance transporters for ticks infected with arboviruses (224, 225). The role of birds in any sudden incursion and occurrence of tick-borne virus infections in a region that was previously free of the infections should thus be considered.
Other Arboviruses
Several arboviruses with unknown impacts have been confirmed to infect domestic ruminants. The infected ruminants usually do not develop apparent clinical signs, and thus these viruses have been neglected for many years. Nevertheless, the recent progress in genetic analyses can characterize their properties and assign their taxonomy.
Getah virus (GETV; species Getah virus, genus Alphavirus, family Togaviridae) is an etiological agent of fever, rash, and leg edema in horses (226) and of fetal death and reproduction disorders in pigs (227, 228). The prevalence of GETV has been confirmed in mainland China, Japan, Korea, and Taiwan (229, 230). Outbreaks of GETV infection have occurred among Japanese racehorses (231, 232). It is known that GETV also infects cattle, although there is no evidence of illness in the infected cattle (226, 233, 234). However, cattle may contribute to a GETV endemic as a reservoir.
Fukuoka virus (FUKV) is assigned to the species Fukuoka ledantevirus of the genus Ledantevirus within the family Rhabdoviridae and was initially isolated from Culicoides punctatus and the mosquito Culex tritaeniorhynchus in southern Japan in 1982 (235). The virus was first regarded as a member of the ephemeral fever serogroup but was subsequently classified as a member of the Kern Canyon serogroup viruses (236, 237). FUKV was later isolated from sentinel cattle in a different region in Japan and, simultaneously, the seroprevalence of FUKV in cattle was demonstrated (238). Although cattle were identified as a susceptible host of FUKV, the pathogenicity of this virus in cattle remains uncertain. Full-genome analysis of ledanteviruses demonstrated that FUKV has a closed relationship (79.5% nucleotide identity) with Barur virus which has been isolated from ticks, fleas, mosquitoes and a rodent in India and Africa (239). Nishimuro virus from a wild boar in Japan (240) is also genetically similar to FUKV. Further studies are needed to determine their vertebrate hosts and arthropod vectors.
Sathuvachari virus (SVIV) is a member of the genus Orbivirus and was initially isolated in 1963 from starlings (Brahminy myna) collected in southern India (241). The virus was also isolated from an unidentified source collected in Vietnam over 50 years ago (242). The genetic characterization of SVIV suggested its close relationship with other mosquito-borne orbiviruses (241). An unassigned virus isolated from bovine blood collected in Japan's southwestern islands in 2005 was recently identified as SVIV (243). No illness in the infected cattle was reported when their blood samples were collected, but the finding demonstrated that cattle are a susceptible host of SVIV and indicated that the virus has a wider host range than previously thought.
An ephemerovirus tentatively called KSB-1/P/03 was isolated from sentinel cattle in southern Japan in 2003 (179). The phylogenetic analysis based on the L protein of rhabdoviruses revealed that this virus is clustered with other ephemeroviruses. The infected cattle did not demonstrate any clinical signs. Other than BEFV, no ephemerovirus had been isolated in East Asia. Further genetic analyses should be conducted to determine the taxonomic assignment of KSB-1/P/03.
A virus isolated from mosquitoes in Tibet, China in 2009 was identified as a novel species of the genus Orbivirus (244). Designated as Tibet Orbivirus (TIBOV), the virus has been obtained from mosquitoes and Culicoides biting midges in other regions of mainland China (86, 245, 246). Phylogenetic analysis of inner core (T2) protein of known orbiviruses demonstrated that TIBOV is clearly categorized into the Culicoides-borne group with BTV, EHDV, and PALV (244, 245). The amino acid sequence diversity among outermost capsid proteins of TIBOV isolates indicated that the species consists of at least two serotypes (246). The neutralization antibodies to TIBOV were detected in cattle reared in the southern border region of mainland China (246).
A novel segmented RNA virus which is genetically related to flaviviruses was detected in ticks collected in mainland China in 2010 and was designated as Jingmen tick virus (JMTV) (247). Together with insect viruses that have a similar genome composition, JMTV is tentatively referred as the Jingmenvirus group (248). Cattle sera collected in mainland China were reported to contain antibodies against JMTV (247). The genome of JMTV was also detected in a part of the seropositive samples by RT-PCR screening. JMTV was recently identified as a potential human pathogen, because patients infected with the virus showed clinical manifestations such as fever, headache, and myalgia (249).
Arthropod Vectors of Important Ruminant Arboviruses
The identification of the vectors of arboviruses is essential to understand the epidemiology of the arboviruses and to develop control measures against them. In this section, we focus on the potential vectors of arboviruses that affect domestic ruminants in East Asia. Many orbiviruses (e.g., BTV and EHDV) and orthobunyaviruses (e.g., AKAV and SBV) that are pathogenic to domestic ruminants were reported to be transmitted by Culicoides biting midges (250, 251). Long-term monitoring for virus detection in Culicoides biting midges in southern Japan demonstrated that various arboviruses such as AKAV, AINOV, SATV, CHUV, DAGV, BCV, and EHDV were preferentially isolated from C. oxystoma (Table 5) (65, 179).
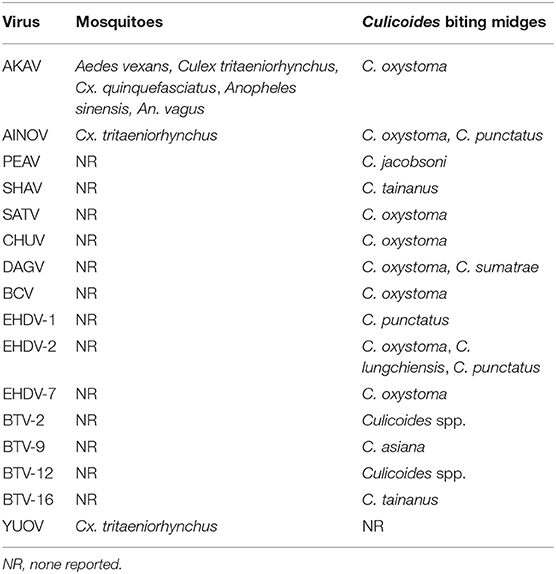
Table 5. Isolation sources of hematophagous invertebrates for arboviruses affecting domestic ruminants in East Asia.
Although Culicoides biting midges are regarded as incriminate vectors of AKAV, the virus has been obtained from three major mosquito genera (Aedes, Culex, and Anopheles) in mainland China and Japan (Table 5) (60, 84, 86). A small portion of mosquitoes that feed on infected cattle during viremia may be infected with AKAV, as observed in the experimental infection of Culex mosquitoes with SBV (252).
Culicoides species such as C. imicola and C. sonorensis, which play the principal role for ruminant arbovirus transmission in other regions, are not distributed in East Asia (253). The distribution of C. brevitarsis, which is a incriminate vector of ruminant arboviruses in Australia (254) was described in southern Japan and Taiwan (255–257). However, molecular analyses using mitochondrial and nuclear DNA indicated that the specimens collected in southern Japan were distinct from the C. brevitarsis specimens collected in Australia, and thus a new species was recorded: Culicoides asiana (258, 259). The isolation of BTV from field-collected C. asiana was successful (260), and an experimental infection indicated that this species is susceptible to AKAV (261).
These findings suggested the vector capacity of C. asiana as with C. brevitarsis. The above-cited studies also suggested that C. brevitarsis and C. asiana were co-distributed throughout Southeast Asia and its neighboring regions, including Hainan Island, which is in a subtropical zone in China (258, 259). The role of these two species in arbovirus transmission should be further evaluated in their distribution ranges.
A laboratory rearing system of Culicoides biting midges was successfully established for limited species distributed in North America and Europe (251, 253). This limitation hampers the progress in the assessments of the vector capacity of various other Culicoides species. Instead, field-collected midges have often been used for checking the oral susceptibility and vector competence of ruminant arboviruses (262–266). Unfortunately, the vector competence of Culicoides biting midges distributed in East Asia is poorly understood. The viral isolations from pools of field-collected midges which were identified at the species level have helped estimate vector species (65, 179).
The oral susceptibility of several Japanese Culicoides species for AKAV was demonstrated (261). As the virus isolation from field-collected midges suggested, C. oxystoma was susceptible to AKAV, and the viral replication and dissemination were demonstrated in the infected midges. Interestingly, C. tainanus and C. punctatus, which have not been considered AKAV-competent vectors, were also orally infected with AKAV. During the AKAV endemic in northern Japan in 2010, C. tainanus and C. punctatus were dominant/subdominant species at cowsheds in the endemic region, but C. oxysoma has been never collected there (267). These species, which are common in East Asia (268–271) probably contributed to the AKAV transmission during that period.
BEFV has been isolated from several mosquito and Culicoides species in Africa, Australia, and Central Asia (9), but there has been no record of the isolation of BEFV from invertebrate vectors in East Asia so far (28). A laboratory experimental infection of Culicoides biting midges indicated that, at the least, they are not efficient vectors of BEFV (272). The vector search will be essential to understand the epidemiology of BEFV in East Asia.
In light of the BTV and SBV endemic experiences in northern Europe, it appears that the Culicoides species that are indigenous in northern temperate regions have the potential to transmit ruminant arboviruses (273, 274). It is necessary to assess the vector capacity of Culicoides species which are distributed in the higher-latitude regions in East Asia. It is also important to determine their distribution range and seasonality to gain a greater understanding of the potential risks of arbovirus spreads, even in previous arbovirus-free regions.
Research Gaps and Future Directions
Vaccinations are probably the most effective preventive measures for arbovirus infections in domestic ruminants. The numbers of animals affected by AKAV, AINOV, CHUV, IBAV, and BEFV were dramatically reduced in Japan after the spread of vaccinations (52). However, limited effectiveness of the current vaccination strategy for BEFV was suggested in Taiwan and Israel (33, 275). It might be difficult to confer long-lasting effective immunity in vaccinated and infected cattle (276, 277), and this probably caused the repetition of BEF occurrence in the above-mentioned regions. It remains difficult to prevent the encephalomyelitis caused by postnatal AKAV infection (67). Vaccines for AKAV are generally administered to breeding cows but not other cattle such as fattening cattle and bulls. There is not yet enough preparation for the pathogens that have recently emerged, such as PEAV, SATV, SHAV, and YUOV, which are suspected to cause ruminant illnesses. Probable loss and future threat caused by these viruses also remain uncertain. Depending on the situation, the development of preventive measures for these arboviruses that have newly emerged in East Asia would be necessary.
The genogroup shifts of AKAV in Japan clearly reflected the change in the types of disease (67). The newly emerged clade of BEFV seems to be more virulent than those of previously endemic strains (28). In addition, the reassortment of segmented RNA viruses (such as orthobunyaviruses and orbiviruses) may increase the viral fitness in vertebrate hosts and/or invertebrate vectors and enhance the virulence in vertebrate hosts and/or the transmission efficacy by invertebrate vectors. Therefore, continuous monitoring of the virulence and pathogenicity of endemic arboviruses should be conducted in East Asia.
The recent emergence of “new” arboviruses in East Asia has attracted interest in their origins and incursion routes. In addition, genogroup shifts of endemic arboviruses in the region have probably been induced by the introductions of viruses from a different genetic pool in other regions. Extensive surveillance in Australia obtained many arboviruses, and some of them are also endemic or were recently emerged in East Asia (278). However, phylogenetic studies of orthobunyaviruses and BEFV indicated that they evolved independently in separate gene pools with different geographical origins (22, 108, 279). In contrast, BEFV, BTV, BANV, and JEV strains, which were isolated in Southeast Asia, often have closed genetic relationships with those isolated in East Asia (280–283).
The circulation of other ruminant arboviruses such as AKAV, AINOV, PEAV, and BATV has also been demonstrated in Southeast Asian countries by serological surveillance and virus isolations (284–287). Unfortunately, there are not enough reports to determine the current status of these arboviruses in Southeast Asia. Further virological surveillance and genetic characterizations of isolated viruses are essential to elucidate the epidemiological linkage of ruminant arboviruses between East Asia and Southeast Asia.
The long-range dispersal of vector insects by winds is considered a principal cause of the introduction of arboviruses to geographically distant locations, including previously arbovirus-free areas (288). Historically, windborne introductions of Culicoides-borne animal diseases from distant endemic areas have been estimated based on meteorological data (289, 290). Plausible dispersal events from eastern Indonesia, Timor-Leste, and southern Papua New Guinea explored by using an atmospheric dispersion model helped elucidate the incursion of exotic Culicoides species and BTVs into northern Australia (291). Atmospheric dispersion models would be useful to assess the potential sources of arbovirus incursions from outside of East Asia and their spread within the region.
As a pioneering study, a backward-trajectory analysis of the BEF epidemic in 2012 in Japan's southwestern islands showed that the estimated potential sources of infected vectors were Southeast Asian countries such as Vietnam and Philippine (21). Reassessments of historical outbreaks of ruminant arbovirus infections with an atmospheric dispersal model could also help determine their incursion sources and spread patterns.
With the exception of BEFV, which has a higher incident rate in cattle (9), almost all of the arboviruses generally result in a low morbidity, asymptomatic/mild illnesses or no apparent acute phase in domestic ruminants, and thus they silently circulate in the endemic regions. Active surveillance such as sentinel and vector surveillance systems are therefore needed for the efficient detection of the arboviruses' circulation in the field prior to disease outbreaks (292). The recent increase in the number of emerging arboviruses in East Asia indicates that other undetected arboviruses may have circulated. The expansion of global transportation may also enhance the risk of arbovirus introductions from geographically distant areas. Global warming will certainly affect the distribution and active period of vectors, and thus the ranges of virus spread will expand to higher-latitude regions.
Although sensitive detection systems are essential to identify the incursion and circulation of arboviruses, the current serological and genetic techniques cannot cover all arboviruses, especially if they are novel. Next-generation sequencing (NGS) technologies will support the detection and characterization of such viruses (293). The findings revealed by NGS will contribute to the development of diagnostic/detection systems suitable for the arboviruses and to the progress of etiological studies.
Clearly, knowledge gaps remain regarding the transmission cycles of arboviruses and vector ecology in East Asia. To the best of our knowledge, there is no detailed distribution map of vectors in the East Asia region. The vector capacity for arboviruses of veterinary importance has been evaluated for only a limited number of species. Further studies of the systematics, biology, and vector competence of mosquitoes, Culicoides biting midges, and ticks should be conducted. The knowledge obtained from vector studies will contribute to increased accuracy of epidemiological analyses based on landscape and meteorological data. Risk assessments based on such analyses will enable the development of better surveillance and preventive measures for arbovirus infections which threaten the production of ruminant livestock, as suggested in earlier investigations (294, 295).
Finally, the construction of a network in East Asia and its neighboring countries would be essential to promote research on arbovirus infections of importance to livestock. The sharing of epidemiological information, diagnostic tools, and control/preventive strategies are highly important because neighboring countries are likely to experience the same problems due to arboviruses. Language barriers may present a challenge to information exchanging and sharing. Many scientific reports and public records from East Asian countries have been written in national languages such as Chinese, Japanese, and Korean, but a limited portion of them might have been translated in English. Unfortunately, the status of arbovirus infections in domestic ruminants in Mongolia and North Korea remains unclear. Regional cooperation would therefore be a crucial key to clarify the current status of arbovirus infections and minimize their impacts on the livestock industries in East Asia.
Author Contributions
TY executed a first draft. YH and KM helped draft the manuscript. All authors contributed equally to the final draft and editing of the manuscript.
Funding
This study was conducted under the research project on Development of the Management Technologies for the Risk of Introduction of Livestock Infectious Diseases and Their Wildlife-borne Spreads in Japan funded by the Ministry of Agriculture, Forestry and Fisheries of Japan (FY2018-2022).
Conflict of Interest
The authors declare that the research was conducted in the absence of any commercial or financial relationships that could be construed as a potential conflict of interest.
References
1. Arrigo NC, Weaver SC, Calisher CH. The taxonomy of arboviruses. In: Vasilakis N, Gubler DJ, editors. Arboviruses. Norfolk: Caister Academic Press (2016). p. 9–29. doi: 10.21775/9781910190210.02
2. Abudurexiti A, Adkins S, Alioto D, Alkhovsky SV, Avšič-Županc T, Ballinger MJ, et al. Taxonomy of the order Bunyavirales: update 2019. Arch Virol. (2019) 164:1949–65. doi: 10.1007/s00705-019-04253-6
3. Maclachlan NJ, Zientara S, Wilson WC, Richt JA, Savini G. Bluetongue and epizootic hemorrhagic disease viruses: recent developments with these globally re-emerging arboviral infections of ruminants. Curr Opin Virol. (2019) 34:6–62. doi: 10.1016/j.coviro.2018.12.005
4. Wilson AJ, Mellor PS. Bluetongue in europe: past, present and future. Philos Trans R Soc Lond B Biol Sci. (2009) 364:2669–81. doi: 10.1098/rstb.2009.0091
5. Savini G, Afonso A, Mellor P, Aradaib I, Yadin H, Sanaa M, et al. Epizootic heamorragic disease. Res Vet Sci. (2011) 91:1–17. doi: 10.1016/j.rvsc.2011.05.004
6. Hoffmann B, Scheuch M, Höper D, Jungblut R, Holsteg M, Schirrmeier H, et al. Novel orthobunyavirus in cattle, Europe, 2011. Emerg Infect Dis. (2012) 18:469–72. doi: 10.3201/eid1803.111905
7. Endalew AD, Faburay B, Wilson WC, Richt JA. Schmallenberg disease-a newly emerged Culicoides-borne viral disease of ruminants. Viruses. (2019) 11:1065. doi: 10.3390/v11111065
8. Gilbert M, Nicolas G, Cinardi G, Van Boeckel TP, Vanwambeke, SO„, et al. Global distribution data for cattle, buffaloes, horses, sheep, goats, pigs, chickens and ducks in 2010. Sci Data. (2018) 5:180227. doi: 10.1038/sdata.2018.227
9. Walker PJ, Klement E. Epidemiology and control of bovine ephemeral fever. Vet Res. (2015) 46:124. doi: 10.1186/s13567-015-0262-4
10. Dacheux L, Dommergues L, Chouanibou Y, Doméon L, Schuler C, Bonas S, et al. Co-circulation and characterization of novel African arboviruses (genus Ephemerovirus) in cattle, Mayotte island, Indian Ocean, 2017. Transbound Emerg Dis. (2019) 66:2601–4. doi: 10.1111/tbed.13323
11. Oguzoglu TÇ, Ertürk A, Çizmeci SG, Koç BT, Akça Y. A report on bovine ephemeral fever virus in Turkey: antigenic variations of different strains of BEFV in the 1985 and 2012 outbreaks using partial glycoprotein gene sequences. Transbound Emerg Dis. (2015) 62:e66–70. doi: 10.1111/tbed.12187
12. Almasi S, Bakhshesh M. Antigenic variation of bovine ephemeral fever viruses isolated in Iran, 2012-2013. Virus Genes. (2019) 55:654–9. doi: 10.1007/s11262-019-01688-6
13. Aziz-Boaron O, Klausner Z, Hasoksuz M, Shenkar J, Gafni O, Gelman B, et al. Circulation of bovine ephemeral fever in the Middle East-strong evidence for transmission by winds and animal transport. Vet Microbiol. (2012) 158:300–7. doi: 10.1016/j.vetmic.2012.03.003
14. Walker PJ. Bovine ephemeral fever in Australia and the world. Curr. Top. Microbiol Immunol. (2005) 292:57–80. doi: 10.1007/3-540-27485-5_4
15. Sato K. A history of bovine influenza in Japan. Jpn J Vet Hist. (2016) 53:14–23. doi: 10.3201/eid2208.160362
17. Inaba Y, Sato K, Tanaka Y, Ito H, Omori T. Serological identification of bovine epizootic fever virus as ephemeral fever virus. Jpn J Microbiol. (1969) 13:388–9. doi: 10.1111/j.1348-0421.1969.tb00484.x
18. Omori T. Ibaraki disease: a bovine epizootic disease resembling bluetongue. Nat Anim Health Q. (1970) 10:45–55.
19. Shirakawa H, Ishibashi K, Ogawa T. A comparison of the epidemiology of bovine ephemeral fever in South Korea and south-western Japan. Aust Vet J. (1994) 71:50–2. doi: 10.1111/j.1751-0813.1994.tb06153.x
20. Aizawa M, Takayoshi K, Kokuba T, Kato T, Yanase T, Yamakawa M, et al. Molecular epidemiological analysis of bovine ephemeral fever virus isolated in Okinawa Prefecture. J Jpn Vet Med Assoc. (2008) 61:363–6. doi: 10.12935/jvma1951.61.363
21. Hayama Y, Moriguchi S, Yanase T, Suzuki M, Niwa T, Ikemiyagi K, et al. Epidemiological analysis of bovine ephemeral fever in 2012-2013 in the subtropical islands of Japan. BMC Vet Res. (2016) 12:47. doi: 10.1186/s12917-016-0673-0
22. Hirashima Y, Nojiri M, Ohtsuka Y, Kato T, Shirafuji H, Kurazono M, et al. Resurgence of bovine ephemeral fever in mainland Japan in 2015 after a 23-year absence. J Vet Med Sci. (2017) 79:904–11. doi: 10.1292/jvms.16-0345
23. Zheng F, Qiu C. Phylogenetic relationships of the glycoprotein gene of bovine ephemeral fever virus isolated from mainland China, Taiwan, Japan, Turkey, Israel and Australia. Virol J. (2012) 9:268. doi: 10.1186/1743-422X-9-268
24. Li Z, Zheng F, Gao S, Wang S, Wang J, Liu Z, et al. Large-scale serological survey of bovine ephemeral fever in China. Vet Microbiol. (2015) 176:155–60. doi: 10.1016/j.vetmic.2014.12.008
25. Ogawa T. Epidemiological investigation of bovine ephemeral fever outbreaks in Kyushu Island in Japan during the fall of 1988. Prev Vet Med. (1992) 14:69–76. doi: 10.1016/0167-5877(92)90085-T
26. Ogawa T, Satoh C, Yamane I, Inoue T. Epidemiology of bovine ephemeral fever in China. Bull Natl Inst Anim Health. (1992) 100:29–35.
27. Kim YH, Oem JK, Lee EY, Lee KK, Kim SH, Lee MH, et al. Seroprevalence of five arboviruses in sentinel cattle as part of nationwide surveillance in South Korea, 2009-2012. J Vet Med Sci. (2015) 77:247–50. doi: 10.1292/jvms.14-0163
28. Lee F. Bovine ephemeral fever in Asia: recent status and research gaps. Viruses. (2019) 11:412. doi: 10.3390/v11050412
29. Yang SJ, Wu L. Tainan cattle farms hit by bovine ephemeral fever. Focus Taiwan (2014). Available Online at: http://m.focustaiwan.tw/news/aall/201404150029.aspx
30. Ting LJ, Lee MS, Lin YL, Cheng MC, Lee F. Invasion of exotic bovine ephemeral fever virus into Taiwan in 2013-2014. Vet Microbiol. (2016) 182:15–17. doi: 10.1016/j.vetmic.2015.10.025
31. Zheng FY, Lin GZ, Qiu CQ, Zhou JZ, Cao XA, Gong„, et al. Serological detection of bovine ephemeral fever virus using an indirect ELISA based on antigenic site G1 expressed in Pichia pastoris. Vet J. (2010) 185:211–5. doi: 10.1016/j.tvjl.2009.06.002
32. Zheng F, Lin G, Zhou J, Wang G, Cao X, Gong X, et al. A reverse-transcription, loop-mediated isothermal amplification assay for detection of bovine ephemeral fever virus in the blood of infected cattle. J Virol Methods. (2011) 171:306–9. doi: 10.1016/j.jviromet.2010.10.028
33. Ting LJ, Lee MS, Lee SH, Tsai HJ, Lee F. Relationships of bovine ephemeral fever epizootics to population immunity and virus variation. Vet Microbiol. (2014) 173:241–8. doi: 10.1016/j.vetmic.2014.07.021
34. Niwa T, Shirafuji H, Ikemiyagi K, Nitta Y, Suzuki M, Kato T, et al. Occurrence of bovine ephemeral fever in Okinawa Prefecture, Japan, in 2012 and development of a reverse-transcription polymerase chain reaction assay to detect bovine ephemeral fever virus gene. J Vet Med Sci. (2015) 77:455–60. doi: 10.1292/jvms.14-0492
35. Finlaison DS, Read AJ, Zhang J, Paskin R, Kirkland PD. Application of a real-time polymerase chain reaction assay to the diagnosis of bovine ephemeral fever during an outbreak in New South Wales and northern Victoria in 2009-10. Aust Vet J. (2014) 92:24–7. doi: 10.1111/avj.12139
36. Gao S, Du J, Tian Z, Niu Q, Huang D, Wang J, et al. A SYBR green I-based quantitative RT-PCR assay for bovine ephemeral fever virus and its utility for evaluating viral kinetics in cattle. J Vet Diagn Invest. (2019) 32:44–50. doi: 10.1177/1040638719895460
37. Inaba Y, Kurogi H, Sato K, Goto Y, Omori T, Matumoto M. Formalin-inactivated, aluminum phosphate gel-adsorbed vaccine of bovine ephemeral fever virus. Arch Gesamte Virusforsch. (1973) 42:42–53. doi: 10.1007/bf01250506
38. Inaba Y, Kurogi H, Takahashi A, Sato K, Omori T. Vaccination of cattle against bovine ephemeral fever with live attenuated virus followed by killed virus. Arch Gesamte Virusforsch. (1974) 44:121–32. doi: 10.1007/bf01250220
39. Wang FI, Hsu AM, Huang KJ. Bovine ephemeral fever in Taiwan. J Vet Diagn Invest. (2001) 13:462–7. doi: 10.1177/104063870101300602
40. Omori T, Inaba Y, Morimoto T, Tanaka Y, Ishitani R. Ibaraki virus, an agent of epizootic disease of cattle resembling bluetongue. I. Epidemiologic, clinical and pathologic observations and experimental transmission to calves. Jpn J Microbiol. (1969) 13:139–57. doi: 10.1111/j.1348-0421.1969.tb00447.x
41. Omori T, Inaba Y, Morimoto T, Tanaka Y, Kono M. Ibaraki virus, an agent of epizootic disease of cattle resembling bluetongue. II. Isolation of the virus in bovine cell culture. Jpn J Microbiol. (1969) 13:159–68. doi: 10.1111/j.1348-0421.1969.tb00448.x
42. Campbell CH, Barber TL, Jochim MM. Antigenic relationship of Ibaraki, bluetongue, and epizootic hemorrhagic disease viruses. Vet Microbiol. (1978) 3:15–22. doi: 10.1016/0378-1135(78)90011-1
43. Uchikoshi N, Enaga N, Shimohira H, Aoki Y, Minamikawa F, Tokunaga M, et al. An outbreak of Ibaraki disease in Kyushu, Japan in 1982. J Jpn Vet Med Assoc. (1983) 36:648–52. doi: 10.12935/jvma1951.36.648
44. Kitano Y, Yamashita S, Fukuyama T. Pathological observations on cattle that died of Ibaraki disease. J Jpn Vet Med Assoc. (1988) 41:884–8. doi: 10.12935/jvma1951.41.884
45. Iwasaki S, Goto Y, Miura Y, Nagatomo M, Kono Y. An outbreak of Ibaraki disease in Miyazaki Prefecture. J Jpn Vet Med Assoc. (1990) 43:244–8. doi: 10.12935/jvma1951.43.244
46. Hirashima Y, Kato T, Yamakawa M, Shirafuji H, Okano R, Yanase T. Reemergence of Ibaraki disease in southern Japan in 2013. J Vet Med Sci. (2015) 77:1253–9. doi: 10.1292/jvms.15-0039
47. Bak UB, Choi HI, Lee CW, Oh HS, Rhee YO, Cheong CK, et al. An outbreak of Ibaraki disease in Korea. Korean J Vet Res. (1983) 23:81–9.
48. Liao YK, Lu YS, Huang ST, Lee SH. The etiological and epidemiological studies on the Ibaraki disease in Taiwan. Exp Rep Taiwan Anim Health Res Inst. (1996) 32:49–57.
49. Maan NS, Maan S, Potgieter AC, Wright IM, Belaganahalli M, Mertens PPC. Development of real-time RT-PCR assays for detection and typing of epizootic haemorrhagic disease virus. Transbound Emerg Dis. (2017) 64:1120–32. doi: 10.1111/tbed.12477
50. Hanaki T. Attenuated vaccine to Ibaraki disease. J Jpn Vet Med Assoc. (1980) 33:451–3. doi: 10.12935/jvma1951.33.451
51. Sekiguchi H, Kozasa T, Makie H. Current status of animal vaccines in Japan. J Jpn Vet Med Assoc. (2010) 63:234–41. http://nichiju.lin.gr.jp/mag/06304/a4.pdf
52. Forman S, Hungerford N, Yamakawa M, Yanase T, Tsai HJ, Joo YS, et al. Climate change impacts and risks for animal health in Asia. Rev Sci Tech. (2008) 27:581–97. doi: 10.20506/rst.27.2.1814
53. Miura Y, Hayashi S, Ishihara T, Inaba Y, Omori T. Neutralizing antibody against Akabane virus in precolostral sera from calves with congenital arthrogryposis-hydranencephaly syndrome. Arch Gesamte Virusforsch. (1974) 46:377–80. doi: 10.1007/bf01240082
54. Kurogi H, Inaba Y, Goto Y, Miura Y, Takahashi H. Serologic evidence for etiologic role of Akabane virus in epizootic abortion-arthrogryposis-hydranencephaly in cattle in Japan, 1972-1974. Arch Virol. (1975) 47:71–83. doi: 10.1007/bf01315594
55. Kirkland PD. Akabane virus infection. Rev Sci Tech. (2015) 34:403–10. doi: 10.20506/rst.34.2.2366
56. Haughey KG, Hartley WJ, Della-Porta AJ, Murray MD. Akabane disease in sheep. Aust Vet J. (1988) 65:136–40. doi: 10.1111/j.1751-0813.1988.tb14440.x
57. Kirkland PD, Barry RD, Harper PA, Zelski RZ. The development of Akabane virus-induced congenital abnormalities in cattle. Vet Rec. (1988) 122:582–6. doi: 10.1136/vr.122.24.582
58. Jagoe S, Kirkland PD, Harper PA. An outbreak of Akabane virus-induced abnormalities in calves after agistment in an endemic region. Aust Vet J. (1993) 70:56–8. doi: 10.1111/j.1751-0813.1993.tb15139.x
59. Brenner J, Rotenberg D, Jaakobi S, Stram Y, Guini-Rubinstein M, Menasherov S, et al. What can Akabane disease teach us about other arboviral diseases. Vet Ital. (2016) 52:353–62. doi: 10.12834/VetIt.547.2587.2
60. Oya A, Okuno T, Ogata T, Kobayashi I, Matsuyama T. Akabane, a new arbor virus isolated in Japan. Jpn J Med Sci Biol. (1961) 14:101–8. doi: 10.7883/yoken1952.14.101
61. Kurogi H, Inaba Y, Takahashi E, Sato K, Satoda K. Congenital abnormalities in newborn calves after inoculation of pregnant cows with Akabane virus. Infect Immun. (1977) 17:338–43.
62. Kurogi H, Inaba Y, Takahashi E, Sato K, Goto Y. Experimental infection of pregnant goats with Akabane virus. Natl Inst Anim Health Q. (1977) 17:1–9.
63. Nakamura K, Morita J, Hanamatsu K, Tomabechi M, Sasaki M, Saito S, et al. A collective outbreak of Akabane disease on sheep farms. J Jpn Vet Med Assoc. (1987) 40:513–8. doi: 10.12935/jvma1951.40.513
64. Kurogi H, Akiba K, Inaba Y, Matumoto M. Isolation of Akabane virus from the biting midge Culicoides oxystoma in Japan. Vet Microbiol. (1987) 15:243–8. doi: 10.1016/0378-1135(87)90078-2
65. Yanase T, Kato T, Kubo T, Yoshida K, Ohashi S, Yamakawa M, et al. Isolation of bovine arboviruses from Culicoides biting midges (Diptera: Ceratopogonidae) in southern Japan: 1985–2002. J Med Entomol. (2005) 42:63–7. doi: 10.1093/jmedent/42.1.63
66. Yamakawa M, Yanase T, Kato T, Tsuda T. Chronological and geographical variations in the small RNA segment of the teratogenic Akabane virus. Virus Res. (2006) 121:84–92. doi: 10.1016/j.virusres.2006.04.007
67. Yanase T, Kato T, Hayama Y, Akiyama M, Itoh N, Horiuchi S, et al. Transition of Akabane virus genogroups and its association with changes in the nature of disease in Japan. Transbound Emerg Dis. (2018) 65:e434–43. doi: 10.1111/tbed.12778
68. Miyazato S, Miura Y, Hase M, Kubo M, Goto Y, Kono Y. Encephalitis of cattle caused by Iriki isolate, a new strain belonging to Akabane virus. Nihon Juigaku Zasshi. (1989) 51:128–36. doi: 10.1292/jvms1939.51.128
69. Kono R, Hirata M, Kaji M, Goto Y, Ikeda S, Yanase T, et al. Bovine epizootic encephalomyelitis caused by Akabane virus in southern Japan. BMC Vet Res. (2008) 4:20. doi: 10.1186/1746-6148-4-20
70. Kobayashi T, Yanase T, Yamakawa M, Kato T, Yoshida K, Tsuda T. Genetic diversity and reassortments among Akabane virus field isolates. Virus Res. (2007) 130:162–71. doi: 10.1016/j.virusres.2007.06.007
71. Bak UB, Lim CH, Cheong CK, Hwang WS, Cho MR. Outbreaks of Akabane disease of cattle in Korea. Korean. J Vet Res. (1980) 20:65–78.
72. Cho JJ, Lee CG, Park BK, Chang CH, Chung CW, Cho IS, et al. Isolation of Akabane virus and its molecular diagnosis by reverse transcription polymerase chain reaction. Korean J Vet Res. (2000) 40:42–8. Available online at: http://kjvr.org/upload/06902772.pdf
73. Yang DK, Kim YH, Kim BH, Kweon CH, Yoon SS, Song JY, et al. Characterization of Akabane virus (KV0505) from cattle in Korea. Korean J Vet Res. (2008) 48:61–6. Available online at: http://kjvr.org/upload/06903826.pdf
74. Oem JK, Lee KH, Kim HR, Bae YC, Chung JY, Lee OS, et al. Bovine epizootic encephalomyelitis caused by Akabane virus infection in Korea. J Comp Pathol. (2012) 147:101–5. doi: 10.1016/j.jcpa.2012.01.013
75. Oem JK, Yoon HJ, Kim HR, Roh IS, Lee KH, Lee OS, et al. Genetic and pathogenic characterization of Akabane viruses isolated from cattle with encephalomyelitis in Korea. Vet Microbiol. (2012) 158:259. doi: 10.1016/j.vetmic.2012.02.017
76. Lim SI, Kweon CH, Tark DS, Kim SH, Yang DK. Sero-survey on Aino, Akabane, Chuzan, bovine ephemeral fever and Japanese encephalitis virus of cattle and swine in Korea. J Vet Sci. (2007) 8:45–9. doi: 10.4142/jvs.2008.9.4.381
77. Yang DK, Hwang IJ, Kim BH, Kweon CH, Lee KW, Kang MI, et al. Serosurveillance of viral diseases in Korean native goats (Capra hircus). J Vet Med Sci. (2008) 70:977–9. doi: 10.1292/jvms.70.977
78. Jun K, Yanaka T, Lee KK, Lee JB. Seroprevalence of bovine arboviruses belonging to genus Orthobunyavirus in South Korea. J Vet Med Sci. (2018) 80:1619–23. doi: 10.1292/jvms.17-0542
79. Yang DK, Kim SY, Kim HH, Kang MS, Nah JJ, Choi SS, et al. The follow up study after massive outbreak of Akabane and bovine ephemeral fever viruses in Korea. Korean J Vet Serv. (2013) 36:151–5. doi: 10.7853/kjvs.2013.36.3.151
80. Lee JK, Kim JH, Park BK, Yoo HS, Lee BC, Kim DY. Akabane viral encephalitis in calves in South Korea. Vet Rec. (2007) 161:236–8. doi: 10.1136/vr.161.7.236
81. Liao YK, Lu YS, Goto Y, Inaba Y. The isolation of Akabane virus (Iriki strain) from calves in Taiwan. J Basic Microbiol. (1996) 36:33–9. doi: 10.1002/jobm.3620360108
82. Liao YK, Chang CY, Lu YS. The serological investigation of Akabane disease in Taiwan. J Chin Soc Vet Sci. (1996) 22:121–8.
83. Wang J, Blasdell KR, Yin H, Walker PJ. A large-scale serological survey of Akabane virus infection in cattle, yak, sheep and goats in China. Vet Microbiol. (2017) 207:7–12. doi: 10.1016/j.vetmic.2017.05.014
84. Feng Y, He B, Fu S, Yang W, Zhang Y, Tu C, et al. Isolation and identification of the Akabane virus from mosquitoes in Yunnan Province, China. Bing Du Xue Bao. (2015) 31:51–7.
85. Tang HB, Chen F, Rao G, Bai A, Jiang J, Du Y, et al. Characterization of Akabane virus from domestic bamboo rat, Southern China. Vet Microbiol. (2017) 207:280. doi: 10.1016/j.vetmic.2017.06.018
86. Cao Y, Fu S, Song S, Cai L, Zhang H, Gao L, et al. Isolation and genome phylogenetic analysis of arthropod-borne viruses, including Akabane virus, from mosquitoes collected in Hunan Province, China. Vector Borne Zoonotic Dis. (2019) 19:62–72. doi: 10.1089/vbz.2018.2267
87. Tang HB, Ren P, Qin S, Lin J, Bai A, Qin S, et al. Isolation, genetic analysis of the first Akabane virus from goat in China. J Vet Med Sci. (2019) 81:1445–9. doi: 10.1292/jvms.18-0602
88. Tsuda T, Yoshida K, Yanase T, Ohashi S, Yamakawa M. Competitive enzyme-linked immunosorbent assay for the detection of the antibodies specific to Akabane virus. J Vet Diagn Invest. (2004) 16:571–6. doi: 10.1177/104063870401600613
89. Lunt RA, White JR, Newberry KM, Pourquier P, Comtet L. Development of a commercial ELISA for the detection of serum antibody to Akabane virus. In: Poster Presented at the 2007 WAVLD Meeting. Melbourne (2007).
90. Kittelberger R, McFadden AM, Kirkland PD, Hannah MJ, Orr D, Bueno R, et al. Evaluation of two commercial enzyme-linked immunosorbent assay kits for the detection of serum antibodies against Akabane virus in cattle. J Vet Diagn Invest. (2013) 25:645–8. doi: 10.1177/1040638713500496
91. Oem JK, Kim YH, Kim SH, Lee MH, Lee KK. Serological characteristics of affected cattle during an outbreak of bovine enzootic encephalomyelitis caused by Akabane virus. Trop Anim Health Prod. (2014) 46:261–3. doi: 10.1007/s11250-013-0468-2
92. Li X, Jing H, Liu X, Wang Q, Qiu S, Liu D, et al. Comparative evaluation of two commercial ELISA kits for detection of antibodies against Akabane virus in cattle serum. BMC Vet Res. (2019) 15:408. doi: 10.1186/s12917-019-2156-6
93. Kurogi H, Inaba Y, Takahashi E, Sato K, Omori T, Miura Y, et al. Epizootic congenital arthrogryposis-hydranencephaly syndrome in cattle: isolation of Akabane virus from affected fetuses. Arch Virol. (1976) 51:67–74. doi: 10.1007/bf01317835
94. Shirafuji H, Yazaki R, Shuto Y, Yanase T, Kato T, Ishikura Y, et al. Broad-range detection of arboviruses belonging to Simbu serogroup lineage 1 and specific detection of Akabane, Aino and Peaton viruses by newly developed multiple TaqMan assays. J Virol Methods. (2015) 225:9–15. doi: 10.1016/j.jviromet.2015.08.021
95. Kurogi H, Inaba Y, Takahashi E, Sato K, Goto Y, Satoda K, et al. Development of inactivated vaccine for Akabane disease. Natl Inst Anim Health Q. (1978) 18:97–108.
96. Kurogi H, Inaba Y, Takahashi E, Sato K, Akashi H, Satoda K, et al. An attenuated strain of Akabane virus: a candidate for live virus vaccine. Natl Inst Anim Health Q. (1979) 19:12–22.
97. Kim YH, Kweon CH, Tark DS, Lim SI, Yang DK, Hyun BH, et al. Development of inactivated trivalent vaccine for the teratogenic Aino, Akabane and Chuzan viruses. Biologicals. (2011) 39:152–7. doi: 10.1016/j.biologicals.2011.02.004
98. Takahashi K, Oya A, Okazda T, Matsuo R, Kuma M. Aino virus, a new member of Simbu group of arbovirus from mosquitoes in Japan. Jpn J Med Sci Biol. (1968) 21:95–101. doi: 10.7883/yoken1952.21.95
99. Egashira T, Itimaru H, Yoshinaga N, Enaga N, Utikoshi N, Minamikawa R. Abnormal birth of cattle suggesting an infection of Aino virus. J Jpn Vet Med Assoc. (1989) 42:94–6. doi: 10.12935/jvma1951.42.94
100. Fukutomi T, Ouchi M, Okuda K, Maruno F, Tabayashi K. An abnormal calf birth suggestive of an infection of Aino virus in Okayama Prefecture. J Jpn Vet Med Assoc. (1991) 44:17–9. doi: 10.12935/jvma1951.44.17
101. Kitano Y, Yamashita S, Furukawa M, Makinoda K. Congenital abnormality of calves suggesting Aino virus infection in Kagoshima Prefecture. J Jpn Vet Med Assoc. (1993) 46:469–71. doi: 10.12935/jvma1951.46.469
102. Ishibashi K, Tomishita Y, Shirakawa H, Takaya M, Watanabe A. Congenital scoliosis of calves suspected of Aino virus infection. J Jpn Vet Med Assoc. (1994) 47:87–90. doi: 10.12935/jvma1951.47.87
103. Noda Y, Uchinuno Y, Shirakawa H, Nagasue S, Nagano N, Ohe R, et al. Aino virus antigen in brain lesions of a naturally aborted bovine fetus. Vet Pathol. (1998) 35:409–11. doi: 10.1177/030098589803500511
104. Uchinuno Y, Noda Y, Ishibashi K, Nagasue S, Shirakawa H, Nagano M, et al. Isolation of Aino virus from an aborted bovine fetus. J Vet Med Sci. (1998) 60:1139–40. doi: 10.1292/jvms.60.1139
105. Tsuda T, Yoshida K, Ohashi S, Yanase T, Sueyoshi M, Kamimura S, et al. Arthrogryposis, hydranencephaly and cerebellar hypoplasia syndrome in neonatal calves resulting from intrauterine infection with Aino virus. Vet Res. (2004) 35:531–8. doi: 10.1051/vetres:2004029
106. Yamakawa M, Yanase T, Kato T, Tsuda T. Molecular epidemiological analyses of the teratogenic Aino virus based on the sequences of a small RNA segment. Vet Microbiol. (2008) 129:40–7. doi: 10.1016/j.vetmic.2007.11.001
107. Yanase T, Aizawa M, Kato T, Yamakawa M, Shirafuji H, Tsuda T. Genetic characterization of Aino and Peaton virus field isolates reveals a genetic reassortment between these viruses in nature. Virus Res. (2010) 153:1–7. doi: 10.1016/j.virusres.2010.06.020
108. Wang J, Firth C, Amos-Ritchie R, Davis SS, Yin H, Holmes EC, et al. Evolutionary history of Simbu serogroup orthobunyaviruses in the Australian episystem. Virology. (2019) 535:32–44. doi: 10.1016/j.virol.2019.06.013
109. Tzeng HY, Wu HH, Ting LJ, Chang NT, Chou YC, Tu WC. Monitoring Taiwanese bovine arboviruses and non-arboviruses using a vector-based approach. Med Vet Entomol. (2019) 33:195–202. doi: 10.1111/mve.12345
110. Doherty RL, Carley JG, Standfast HA, Dyce AL, Snowdon WA. Virus strains isolated from arthropods during an epizootic of bovine ephemeral fever in Queensland. Aust Vet J. (1972) 48:81–6. doi: 10.1111/j.1751-0813.1972.tb02220.x
111. Miura Y, Inaba Y, Goto Y, Takahashi E, Kurogi H, Hayashi S, et al. Serological comparison of Aino and Samford viruses in Simbu group of bunyaviruses. Microbiol Immunol. (1978) 22:651–4. doi: 10.1111/j.1348-0421.1978.tb00417.x
112. Coverdale OR, Cybinski DH, St George TD. Congenital abnormalities in calves associated with Akabane virus and Aino virus. Aust Vet J. (1978) 54:151–2. doi: 10.1111/j.1751-0813.1978.tb05538.x
113. Golender N, Brenner J, Valdman M, Khinich Y, Bumbarov V, Panshin A, et al. Malformations caused by Shuni virus in ruminants, Israel, 2014-2015. Emerg Infect Dis. (2015) 21:2267–8. doi: 10.3201/eid2112.150804
114. Golender N, Bumbarov V, Assis I, Beer M, Khinich Y, Koren O, et al. Shuni virus in Israel: neurological disease and fatalities in cattle. Transbound Emerg Dis. (2019) 66:1126–31. doi: 10.1111/tbed.13167
115. Shin YK, Oem JK, Yoon S, Hyun BH, Cho IS, Yoon S, et al. Monitoring of five bovine arboviral diseases transmitted by arthropod vectors in Korea. J Bacteriol Virol. (2009) 39:353–62. doi: 10.4167/jbv.2009.39.4.353
116. Goto Y, Miura Y, Kono Y. Serologic evidence for the etiologic role of Chuzan virus in an epizootic of congenital abnormalities with hydranencephaly-cerebellar hypoplasia syndrome of calves in Japan. Am J Vet Res. (1988) 49:2026–9.
117. Miura Y, Goto Y, Kubo M, Kono Y. Isolation of Chuzan virus, a new member of the Palyam subgroup of the genus Orbivirus, from cattle and Culicoides oxystoma in Japan. Am J Vet Res. (1988) 49:2022–5.
118. Miura Y, Kubo M, Goto Y, Kono Y. Hydranencephaly-cerebellar hypoplasia in a newborn calf after infection of its dam with Chuzan virus. Nihon Juigaku Zasshi. (1990) 52:689–94. doi: 10.1292/jvms1939.52.689
119. Jusa ER, Inaba Y, Kadoi K, Kurogi H, Fonseca E, Shope RE. Identification of Kagoshima and Chuzan viruses of Japan as Kasba Virus, an orbivirus of the Palyam serogroup. Aust Vet J. (1994) 71:57. doi: 10.1111/j.1751-0813.1994.tb06155.x
120. Yamakawa M, Kubo M, Furuuchi S. Molecular analysis of the genome of Chuzan virus, a member of the Palyam serogroup viruses, and its phylogenetic relationships to other orbiviruses. J Gen Virol. (1999) 80:937–41. doi: 10.1099/0022-1317-80-4-937
121. Ebersohn K, Coetzee P, Snyman LP, Swanepoel R, Venter EH. Phylogenetic characterization of the Palyam serogroup orbiviruses. Viruses. (2019) 11:pii: E446. doi: 10.3390/v11050446
122. Liao YK, Lu YS, Hwang C, Chang CE. The study on the epidemiology of Akabane virus and Chuzan virus infection in cattle in Taiwan. Exp Rep TPRIAH. (1994) 30:47–53.
123. Yang H, Xiao L, Meng J, Xiong H, Gao L, Liao D, et al. Complete genome sequence of a Chuzan virus strain isolated for the first time in mainland China. Arch Virol. (2016) 161:1073–7. doi: 10.1007/s00705-015-2734-2
124. Wang F, Lin J, Chang J, Cao Y, Qin S, Wu J, et al. Isolation, complete genome sequencing, and phylogenetic analysis of the first Chuzan virus in China. Virus Genes. (2016) 52:138–41. doi: 10.1007/s11262-015-1282-x
125. Wang M, Wang Y, Baloch AR, Pan Y, Tian L, Xu F, et al. Chuzan virus in yaks, Qinghai-Tibetan Plateau, China. Emerg Infect Dis. (2018) 24:2371–3. doi: 10.3201/eid2412.171414
126. Miura Y, Kubo M, Goto Y, Kono Y. Chuzan disease as congenital hydranencephaly-cerebellar hypoplasia syndrome in calves. JARQ. (1991) 25:55–60. https://dl.ndl.go.jp/info:ndljp/pid/9286080
127. Goto Y, Miura Y, Kono Y. A preliminary study for development of an inactivated Chuzan virus vaccine. Nihon Juigaku Zasshi. (1988) 50:673–8. doi: 10.1292/jvms1939.50.673
128. Belbis G, Zientara S, Bréard E, Sailleau C, Caignard G, Vitour D, et al. Bluetongue virus: from BTV-1 to BTV-27. Adv Virus Res. (2017) 99:161–97. doi: 10.1016/bs.aivir.2017.08.003
129. Miura Y, Inaba Y, Hayashi S, Takahashi E, Matumoto M. A survey of antibodies to arthropod-borne viruses in Japanese cattle. Vet Microbiol. (1980) 5:277–82. doi: 10.1016/0378-1135(80)90026-7
130. Miura Y, Inaba Y, Tsuda T, Tokuhisa S, Sato K, Akashi H. Seroepizootiological survey on bluetongue virus infection in cattle in Japan. Natl Inst Anim Health Q. (1982) 22:154–8.
131. Goto Y, Yamaguchi O, Kubo M. Epidemiological observations on bluetongue in sheep and cattle in Japan. Vet Ital. (2004) 40:78–82. Available Online at: http://www.izs.it/vet_italiana/2004/40_3/17.pdf
132. Shirafuji H, Yanase T, Kato T, Yamakawa M. Genetic and phylogenetic characterization of genome segments 2 and 6 of bluetongue virus isolates in Japan from 1985 to 2008. J Gen Virol. (2012) 93:1465–73. doi: 10.1099/vir.0.040717-0
133. Zhang N, Li Z, Zhang K, Hu Y, Li G, Peng K, et al. Bluetongue history, serology and virus isolation in China. In: Proceedings of the First Southeast Asia and Pacific Regional Bluetongue Symposium. 1995 Aug. 22–24. Kunming (1996). p. 43–50.
134. Zhang N, MacLachlan NJ, Bonneau KR, Zhu J, Li Z, Zhang K, et al. Identification of seven serotypes of bluetongue virus from the people's Republic of China. Vet Rec. (1999) 145:427–9. doi: 10.1136/vr.145.15.427
135. Qin S, Yang H, Zhang Y, Li Z, Lin J, Gao L, et al. Full genome sequence of the first bluetongue virus serotype 21 (BTV-21) isolated from China: evidence for genetic reassortment between BTV-21 and bluetongue virus serotype 16 (BTV-16). Arch Virol. (2018) 163:1379–82. doi: 10.1007/s00705-018-3718-9
136. Sun EC, Huang LP, Xu QY, Wang HX, Xue XM, Lu P, et al. Emergence of a novel bluetongue virus serotype, China 2014. Transbound Emerg Dis. (2016) 63:585–9. doi: 10.1111/tbed.12560
137. Ma JG, Zhang XX, Zheng WB, Xu YT, Zhu XQ, Hu GX, et al. Seroprevalence and risk factors of bluetongue virus infection in Tibetan sheep and yaks in Tibetan Plateau, China. Biomed Res Int. (2017) 2017:5139703. doi: 10.1155/2017/5139703
138. Ma J, Gao X, Liu B, Xiao J, Chen H, Wang H. Spatial patterns and risk factors of bluetongue virus infection in Inner Mongolia, China. Vector Borne Zoonotic Dis. (2019) 19:525–32. doi: 10.1089/vbz.2018.2361
139. Ma J, Gao X, Liu B, Chen H, Xiao J, Wang H. Epidemiology and spatial distribution of bluetongue virus in Xinjiang, China. Peer J. (2019) 7:e6514. doi: 10.7717/peerj.6514
140. Ting LJ, Lee MS, Huang TS, Huang CC, Kuo ST, Lee F, et al. Identification of bluetongue virus in goats in Taiwan. Vet Rec. (2005) 156:52. doi: 10.1136/vr.156.2.52
141. Lee F, Ting LJ, Jong MH, Chang WM, Wang FI. Subclinical bluetongue virus infection in domestic ruminants in Taiwan. Vet Microbiol. (2010) 142:225–231. doi: 10.1016/j.vetmic.2009.10.005
142. Seo HJ, Park JY, Cho YS, Cho IS, Yeh JY. First report of bluetongue virus isolation in the Republic of Korea and analysis of the complete coding sequence of the segment 2 gene. Virus Genes. (2015) 50:156–9. doi: 10.1007/s11262-014-1140-2
143. Yeh JY, Kim JG, Choi J, Kim JK, Kim KW. Bluetongue virus antibodies in domestic goats: a countrywide and retrospective study in the Republic of Korea. Vector Borne Zoonotic Dis. (2018) 18:323–30. doi: 10.1089/vbz.2017.2181
144. Hwang JM, Kim JG, Yeh JY. Evidence of bluetongue virus circulation in farmed and free-ranging cervids from the Republic of Korea: a retrospective cross-sectional study. Vet Microbiol. (2019) 230:145–9. doi: 10.1016/j.vetmic.2019.02.009
145. Hwang JM, Kim JG, Yeh JY. Serological evidence of bluetongue virus infection and serotype distribution in dairy cattle in South Korea. BMC Vet Res. (2019) 15:255. doi: 10.1186/s12917-019-2000-z
146. Kim HJ. Complete genome sequence analysis of the bluetongue virus serotype 3 in South Korea. In: Abstract Retrieved From Abstracts in 11th World Congress on Virology and Infectious Diseases. 2018 May 17–18. Tokyo (2018). doi: 10.4172/2161-0517-C1-024
147. Lee F, Ting LJ, Lee MS, Chang WM, Wang FI. Genetic analysis of two Taiwanese bluetongue viruses. Vet Microbiol. (2011) 148:140–9. doi: 10.1016/j.vetmic.2010.08.016
148. Boyle DB, Bulach DM, Amos-Ritchie R, Adams MM, Walker PJ, Weir R. Genomic sequences of Australian bluetongue virus prototype serotypes reveal global relationships and possible routes of entry into Australia. J Virol. (2012) 86:6724–31. doi: 10.1128/JVI.00182-12
149. Zhu J, Yang H, Li H, Xiao L, Wang J, Li N, et al. Full-genome sequence of bluetongue virus serotype 1 (BTV-1) strain Y863, the first BTV-1 isolate of eastern origin found in China. Genome Announc. (2013) 1:e00403-13. doi: 10.1128/genomeA.00403-13
150. Liao D, Yang H, Xiao L, Zhu J, Wang J, Li Z. Complete genome sequence of a bluetongue virus serotype 15 strain isolated from China in 1996. Genome Announc. (2018) 6:e00557-18. doi: 10.1128/genomeA.00557-18
151. Yang H, Lv M, Sun M, Lin L, Kou M, Gao L, et al. Complete genome sequence of the first bluetongue virus serotype 7 isolate from China: evidence for entry of African-lineage strains and reassortment between the introduced and native strains. Arch Virol. (2016) 161:223–7. doi: 10.1007/s00705-015-2624-7
152. Maclachlan NJ, Mayo CE, Daniels PW, Savini G, Zientara S, Gibbs EP. Bluetongue. Rev Sci Tech. (2015) 34:329–40. doi: 10.20506/rst.34.2.2360
153. Shimizu S, Toyota I, Arishima T, Goto Y. Frequency of serological cross-reactions between Ibaraki and bluetongue viruses using the agar gel immunodiffusion test. Vet Ital. (2004) 40:583–6. http://www.izs.it/vet_italiana/2004/40_4/583.pdf
154. St George TD, Standfast HA, Cybinski DH, Filippich C, Carley JG. Peaton virus: a new Simbu group arbovirus isolated from cattle and Culicoides brevitarsis in Australia. Aust J Biol Sci. (1980) 33:235–43. doi: 10.1071/bi9800235
155. Matsumori Y, Inai K, Yanase T, Ohashi S, Kato T, Yoshida K, et al. Serological and genetic characterization of newly isolated Peaton virus in Japan. Arch Virol. (2002) 147:401–10. doi: 10.1007/s705-002-8328-4
156. Parsonson IM, McPhee DA. Bunyavirus pathogenesis. Adv Virus Res. (1985) 30:279–316. doi: 10.1016/S0065-3527(08)60453-4
157. Matsumori Y, Aizawa M, Sakai Y, Inoue D, Kodani M, Tsuha O, et al. Congenital abnormalities in calves associated with Peaton virus infection in Japan. J Vet Diagn Invest. (2018) 30:855–61. doi: 10.1177/1040638718796269
158. Brenner J, Yanase T, Kato T, Yaakobi S, Khinich E, Paz R, et al. Serological evidence suggests that several Simbu serogroup viruses circulated in Israel. Vet Ital. (2019) 55:81–9. doi: 10.12834/VetIt.1397.7622.2
159. Behar A, Leibovich BB, Edery N, Yanase T, Brenner J. First genomic detection of Peaton virus in a calf with hydranencephaly in Israel. Vet Med Sci. (2019) 5:87–92. doi: 10.1002/vms3.129
160. Dandawate CN, Pajagopalan PK, Pavri KM, Work TH. Virus isolations from mosquitoes collected in North Arcot district, Madras state, and Chittoor district, Andhra Pradesh between November 1955 and October 1957. Indian J Med Res. (1969) 57:1420–6.
161. Causey OR, Kemp GE, Causey CE, Lee VH. Isolations of Simbu-group viruses in Ibadan, Nigeria 1964-69, including the new types Sango, Shamonda, Sabo and Shuni. Ann Trop Med Parasitol. (1972) 66:357–62. doi: 10.1080/00034983.1972.11686835
162. Yanase T, Fukutomi T, Yoshida K, Kato T, Ohashi S, Yamakawa M, et al. The emergence in Japan of Sathuperi virus, a tropical Simbu serogroup virus of the genus Orthobunyavirus. Arch Virol. (2004) 149:1007–13. doi: 10.1007/s00705-003-0266-7
163. Yanase T, Kato T, Aizawa M, Shuto Y, Shirafuji H, Yamakawa M, et al. Genetic reassortment between Sathuperi and Shamonda viruses of the genus Orthobunyavirus in nature: implications for their genetic relationship to Schmallenberg virus. Arch Virol. (2012) 157:1611–16. doi: 10.1007/s00705-012-1341-8
164. Yanase T, Maeda K, Kato T, Nyuta S, Kamata H, Yamakawa M, et al. The resurgence of Shamonda virus, an African Simbu group virus of the genus Orthobunyavirus, in Japan. Arch Virol. (2005) 150:361–9. doi: 10.1007/s00705-004-0419-3
165. Hirashima Y, Sakaguchi Z, Okada1 D, Fujioka M, Kitahara S, Iwamoto J, et al. Retrospective survey of Shamonda virus infection in sentinel and breeding cattle and in malformed calves in Kagoshima Prefecture. J Jpn Vet Med Assoc. (2017) 70:729–34. doi: 10.12935/jvma.70.729
166. Hirashima Y, Kitahara S, Kato T, Shirafuji H, Tanaka S, Yanase T. Congenital malformations of calves infected with Shamonda virus, southern Japan. Emerg Infect Dis. (2017) 23:993–6. doi: 10.3201/eid2306.161946
167. Fukuda M, Horiuchi S, Maruta T, Setoyama H, Onitsuka Y, Yanase T. Malformed calves possibly associated with Shamonda virus in Miyazaki Prefecture. J Vet Med. (2018) 71:813–9.
168. Briese T, Calisher CH, Higgs S. Viruses of the family Bunyaviridae: are all available isolates reassortants? Virology. (2013) 446:207–16. doi: 10.1016/j.virol.2013.07.030
169. Goller KV, Höper D, Schirrmeier H, Mettenleiter TC, Beer M. Schmallenberg virus as possible ancestor of Shamonda virus. Emerg Infect Dis. (2012) 18:1644–6. doi: 10.3201/eid1810.120835
170. Coupeau D, Bayrou C, Baillieux P, Marichal A, Lenaerts AC, Caty C, et al. Host-dependence of in vitro reassortment dynamics among the Sathuperi and Shamonda Simbuviruses. Emerg Microbes Infect. (2019) 8:381–95. doi: 10.1080/22221751.2019.1586410
171. Watanabe Y, Makiuchi H, Imafuji T, Yamasaki K, Onitsuka T, Ohashi S. An outbreak of Ibaraki disease in Kagoshima Prefecture in 1997 and properties of the virus isolated from bovine aborted and still birth fetus. J Jpn Vet Med Assoc. (2000) 53:302–6. doi: 10.12935/jvma1951.53.302
172. Ohashi S, Yoshida K, Watanabe Y, Tsuda T. Identification and PCR-restriction fragment length polymorphism analysis of a variant of the Ibaraki virus from naturally infected cattle and aborted fetuses in Japan. J Clin Microbiol. (1999) 37:3800–3. doi: 10.1128/JCM.37.12.3800-3803.1999
173. Ohashi S, Yoshida K, Yanase T, Tsuda T. Analysis of intratypic variation evident in an Ibaraki virus strain and its epizootic hemorrhagic disease virus serogroup. J Clin Microbiol. (2002) 40:3684–8. doi: 10.1128/JCM.40.10.3684-3688.2002
174. Shirafuji H, Kato T, Yamakawa M, Tanaka T, Minemori Y, Yanase T. Characterization of genome segments 2, 3 and 6 of epizootic hemorrhagic disease virus strains isolated in Japan in 1985-2013: Identification of their serotypes and geographical genetic types. Infect Genet Evol. (2017) 53:38–46. doi: 10.1016/j.meegid.2017.05.010
175. Qi Y, Wang F, Chang J, Zhang Y, Zhu J, Li H, et al. Identification and complete-genome phylogenetic analysis of an epizootic hemorrhagic disease virus serotype 7 strain isolated in China. Arch Virol. (2019) 164:3121–6. doi: 10.1007/s00705-019-04412-9
176. Kamomae Y, Kamomae M, Ohta Y, Nabe M, Kagawa Y, Ogura Y, et al. Epizootic hemorrhagic disease virus serotype 6 infection in cattle, Japan, 2015. Emerg Infect Dis. (2018) 24:902–5. doi: 10.3201/eid2405.171859
177. Miura Y, Miyazato S, Kubo M, Goto Y, Kono Y. Kawanabe virus, an isolate from a calf in Japan: a new virus belonging to the New Jersey serotype of the epizootic hemorrhagic disease serogroup of genus Orbivirus. Nihon Juigaku Zasshi. (1988) 50:942–5. doi: 10.1292/jvms1939.50.942
178. Ohashi S, Matsumori Y, Yanase T, Yamakawa M, Kato T, Tsuda T. Evidence of an antigenic shift among Palyam serogroup orbiviruses. J Clin Microbiol. (2004) 42:4610–14. doi: 10.1128/JCM.42.10.4610-4614.2004
179. Kato T, Shirafuji H, Tanaka S, Sato M, Yamakawa M, Tsuda T, et al. Bovine arboviruses in Culicoides biting midges and sentinel cattle in southern Japan from 2003 to 2013. Transbound Emerg Dis. (2016) 63:e160–e172. doi: 10.1111/tbed.12324
180. Kato T, Yanase T, Suzuki M, Katagiri Y, Ikemiyagi K, Takayoshi K, et al. Monitoring for bovine arboviruses in the most southwestern islands in Japan between 1994 and 2014. BMC Vet Res. (2016) 12:125. doi: 10.1186/s12917-016-0747-z
181. Cybinski DH, St George TD. Preliminary characterization of D'Aguilar virus and three Palyam group viruses new to Australia. Aust J Biol Sci. (1982) 35:343–51. doi: 10.1071/BI9820343
182. Attoui H, Mohd Jaafar F, Belhouchet M, Aldrovandi N, Tao S, Chen B, et al. Yunnan orbivirus, a new orbivirus species isolated from Culex tritaeniorhynchus mosquitoes in China. J Gen Virol. (2005) 86:3409–17. doi: 10.1099/vir.0.81258-0
183. Cowled C, Melville L, Weir R, Walsh S, Hyatt A, Van Driel R, et al. Genetic and epidemiological characterization of middle point orbivirus, a novel virus isolated from sentinel cattle in northern Australia. J Gen Virol. (2007) 88:3413–22. doi: 10.1099/vir.0.83231-0
184. Attoui H, Mohd Jaafar F. Zoonotic and emerging orbivirus infections. Rev Sci Tech. (2015) 34:353–61. doi: 10.20506/rst.34.2.2362
185. Yang H, Li Z, Zhang Y, Gao L, Xie J, Liao D, et al. Isolation and genomic characterization of a novel orbivirus strain from the blood of cattle. Bing Du Xue Bao. (2019) 34:75–84.
186. Attoui H, Mendez-Lopez MR, Rao S, Hurtado-Alendes A, Lizaraso-Caparo F, Jaafar FM, et al. Peruvian horse sickness virus and Yunnan orbivirus, isolated from vertebrates and mosquitoes in Peru and Australia. Virology. (2009) 394:298–310. doi: 10.1016/j.virol.2009.08.032
187. Morita K, Nabeshima T, Buerano CC. Japanese encephalitis. Rev Sci Tech. (2015) 34:441–52. doi: 10.20506/rst.34.2.2370
188. Arai S, Matsunaga Y, Takasaki T, Tanaka-Taya K, Taniguchi K, Okabe N, et al. Japanese encephalitis: surveillance and elimination effort in Japan from 1982 to 2004. Jpn J Infect Dis. (2008) 61:333–8. https://www.ncbi.nlm.nih.gov/pubmed/18806337
189. Yamamoto S, Tsubara H, Yoshida T, Harada K. First observation on case of Japanese B encephalitis of cattle. Bull Natl Inst Anim Hlth. (1949) 22:197–203.
190. Shimizu T, Mochizuki H, Sugawa Y, Okazaki K, Matumoto M. Studies on Japanese encephalitis of cattle. 1. Bovine encephalitis caused by natural infection with Japanese encephalitis virus. Natl Inst Anim Hlth Q. (1951) 23:111–8.
191. Katayama K, Ikeda A, Saika K, Ishizaka M, Onodera M, Ito T. A case of Japanese encephalitis in a black Japanese heifer. J Jpn Vet Med Assoc. (2000) 53:293–6. doi: 10.12935/jvma1951.53.293
192. Katayama T, Saito S, Horiuchi S, Maruta T, Kato T, Yanase T, Yamakawa M., et al. Nonsuppurative encephalomyelitis in a calf in Japan and isolation of Japanese encephalitis virus genotype 1 from the affected calf. J Clin Microbiol. (2013) 51:3448–53. doi: 10.1128/JCM.00737-13
193. Kako N, Suzuki S, Sugie N, Kato T, Yanase T, Yamakawa M, et al. Japanese encephalitis in a 114-monthold cow: pathological investigation of the affected cow and genetic characterization of Japanese encephalitis virus isolate. BMC Vet Res. (2014) 10:63. doi: 10.1186/1746-6148-10-63
194. McVey DS, Wilson WC, Gay CG. West Nile virus. Rev Sci Tech. (2015) 34:431–9. doi: 10.20506/rst.34.2.2369
195. Lu Z, Fu SH, Cao L, Tang CJ, Zhang S, Li ZX, et al. Human infection with West Nile virus, Xinjiang, China, 2011. Emerg Infect Dis. (2014) 20:1421–3. doi: 10.3201/eid2008.131433
196. Kim CY, Oh H, Song J, Hur M, Suh JH, Jheong WH, et al. First detection of West Nile virus in domestic pigeon in Korea. J Vet Sci. (2016) 17:587–9. doi: 10.4142/jvs.2016.17.4.587
197. Rimoldi G, Mete A, Adaska JM, Anderson ML, Symmes KP, Diab S. West Nile virus infection in sheep. Vet Pathol. (2017) 54:155–8. doi: 10.1177/0300985816653796
198. Xu P, Wang Y, Zuo J, Lin J, Xu P. New orbiviruses isolated from patients with unknown fever and encephalitis in Yunnan Province. Chin J Virol. (1990) 6:27–33.
199. Attoui H, Billoir F, Biagini P, de Micco P, de Lamballerie X. Complete sequence determination and genetic analysis of Banna virus and Kadipiro virus: proposal for assignment to a new genus (Seadornavirus) within the family Reoviridae. J Gen Virol. (2000) 81:1507–15. doi: 10.1099/0022-1317-81-6-1507
200. Liu H, Li MH, Zhai YG, Meng WS, Sun XH, Cao YX, et al. Banna virus, China, 1987-2007. Emerg Infect Dis. (2010) 16:514–7. doi: 10.3201/eid1603.091160
201. Song S, Li Y, Fu S, Lei W, Guo X, Feng Y, et al. Genome sequencing and phylogenetic analysis of Banna virus (genus Seadornavirus, family Reoviridae) isolated from Culicoides. Sci China Life Sci. (2017) 60:1372–82. doi: 10.1007/s11427-017-9190-6
202. Yanase T, Kato T, Yamakawa M, Takayoshi K, Nakamura K, Kokuba T, et al. Genetic characterization of Batai virus indicates a genomic reassortment between orthobunyaviruses in nature. Arch Virol. (2006) 151:2253–60. doi: 10.1007/s00705-006-0808-x
203. Fu SH, Sun XH, Wang HY, Cao YX, Wang HQ, Liu WB, et al. Molecular biological identification of Batai virus isolated in China. Zhonghua Shi Yan He Lin Chuang Bing Du Xue Za Zhi. (2005) 19:331–4.
204. Liu H, Shao XQ, Hu B, Zhao JJ, Zhang L, Zhang HL, et al. Isolation and complete nucleotide sequence of a Batai virus strain in Inner Mongolia, China. Virol J. (2014) 11:138. doi: 10.1186/1743-422X-11-138
205. Zhang L, Zhang Q, Wang J, An N, Cao Y, Fu G, et al. Characterization of Batai virus isolated from a domestic muscovy duck (Cairina moschate). Virus Genes. (2017) 53:121–5. doi: 10.1007/s11262-016-1400-4
206. Hubálek Z. Mosquito-borne viruses in Europe. Parasitol Res. (2008) 103(Suppl. 1):29–43. doi: 10.1007/s00436-008-1064-7
207. Ziegler U, Groschup MH, Wysocki P, Press F, Gehrmann B, Fast C, et al. Seroprevalance of Batai virus in ruminants from East Germany. Vet Microbiol. (2018) 227:97–102. doi: 10.1016/j.vetmic.2018.10.029
208. Gerrard SR, Li L, Barrett AD, Nichol ST. Ngari virus is a Bunyamwera virus reassortant that can be associated with large outbreaks of hemorrhagic fever in Africa. J Virol. (2004) 78:8922–26. doi: 10.1128/JVI.78.16.8922-8926.2004
209. Briese T, Bird B, Kapoor V, Nichol ST, Lipkin WI. Batai and Ngari viruses: M segment reassortment and association with severe febrile disease outbreaks in East Africa. J Virol. (2006) 80:5627–30. doi: 10.1128/JVI.02448-05
210. Liang G, Li X, Gao X, Fu S, Wang H, Li M, Lu Z, et al. Arboviruses and their related infections in China: a comprehensive field and laboratory investigation over the last 3 decades. Rev Med Virol. (2018) 28:e1959. doi: 10.1002/rmv.1959
211. Spengler JR, Bergeron É, Rollin PE. Seroepidemiological studies of Crimean-Congo hemorrhagic fever virus in domestic and wild animals. PLoS Negl Trop Dis. (2016) 10:e0004210. doi: 10.1371/journal.pntd.0004210
212. Yoshii K, Song JY, Park SB, Yang J, Schmitt HJ. Tick-borne encephalitis in Japan, Republic of Korea and China. Emerg Microbes Infect. (2017) 6:e82. doi: 10.1038/emi.2017.69
213. Paulsen KM, Stuen S, das Neves CG, Suhel F, Gurung D, Soleng A, et al. Tick-borne encephalitis virus in cows and unpasteurized cow milk from Norway. Zoonoses Public Health. (2019) 66:216–22. doi: 10.1111/zph.12554
214. Wang J, Zhang H, Fu S, Wang H, Ni D, Nasci R, et al. Isolation of Kyasanur Forest disease virus from febrile patient, Yunnan, China. Emerg Infect Dis. (2009) 15:326–8. doi: 10.3201/eid1502.080979
215. Boshell J. Kyasanur Forest disease: ecologic considerations. Am J Trop Med Hyg. (1969) 18:67–80. doi: 10.4269/ajtmh.1969.18.67
216. Yu XJ, Liang MF, Zhang SY, Liu Y, Li JD, Sun YL, et al. Fever with thrombocytopenia associated with a novel bunyavirus in China. N Engl J Med. (2011) 364:1523–32. doi: 10.1056/NEJMoa1010095
217. Kim KH, Yi J, Kim G, Choi SJ, Jun KI, Kim NH, et al. Severe fever with thrombocytopenia syndrome, South Korea, 2012. Emerg Infect Dis. (2013) 19:1892–4. doi: 10.3201/eid1911.130792
218. Takahashi T, Maeda K, Suzuki T, Ishido A, Shigeoka T, Tominaga T, et al. The first identification and retrospective study of severe fever with thrombocytopenia syndrome in Japan. J Infect Dis. (2014) 209:816–27. doi: 10.1093/infdis/jit603
219. Li Z, Hu J, Bao C, Li P, Qi X, Qin Y., et al. Seroprevalence of antibodies against SFTS virus infection in farmers and animals, Jiangsu, China. J Clin Virol. (2014) 60:185–9. doi: 10.1016/j.jcv.2014.03.020
220. Tian H, Yu P, Chowell G, Li S, Wei J, Tian H, et al. Severe fever with thrombocytopenia syndrome virus in humans, domesticated animals, ticks, and mosquitoes, Shaanxi Province, China. Am J Trop Med Hyg. (2017) 96:1346–9. doi: 10.4269/ajtmh.16-0333
221. Tabara K, Fujita H, Hirata A, Hayasaka D. Investigation of severe fever with thrombocytopenia syndrome virus antibody among domestic bovines transported to slaughterhouse in Shimane Prefecture, Japan. Jpn J Infect Dis. (2016) 69:445–7. doi: 10.7883/yoken.JJID.2015.624
222. Yu KM, Yu MA, Park SJ, Kim YI, Robles NJ, Kwon HI, et al. Seroprevalence and genetic characterization of severe fever with thrombocytopenia syndrome virus in domestic goats in South Korea. Ticks Tick Borne Dis. (2018) 9:1202–6. doi: 10.1016/j.ttbdis.2018.05.001
223. Robles NJC, Han HJ, Park SJ, Choi YK. Epidemiology of severe fever and thrombocytopenia syndrome virus infection and the need for therapeutics for the prevention. Clin Exp Vaccine Res. (2018) 7:43–50. doi: 10.7774/cevr.2018.7.1.43
224. Lindeborg M, Barboutis C, Ehrenborg C, Fransson T, Jaenson TG, Lindgren PE, et al. Migratory birds, ticks, and Crimean-Congo hemorrhagic fever virus. Emerg Infect Dis. (2012) 18:2095–7. doi: 10.3201/eid1812.120718
225. Yun Y, Heo ST, Kim G, Hewson R, Kim H, Park D, et al. Phylogenetic analysis of severe fever with thrombocytopenia syndrome virus in South Korea and migratory bird routes between China, South Korea, and Japan. Am J Trop Med Hyg. (2015) 93:468–74. doi: 10.4269/ajtmh.15-0047
226. Fukunaga Y, Kumanomido T, Kamada M. Getah virus as an equine pathogen. Vet Clin North Am Equine Pract. (2000) 16:605–17. doi: 10.1016/S0749-0739(17)30099-8
227. Yago K, Hagiwara S, Kawamura H, Narita M. A fatal case in newborn piglets with Getah virus infection: isolation of the virus. Jpn J Vet Sci. (1987) 49:989–94. doi: 10.1292/jvms1939.49.989
228. Izumida A, Takuma H, Inagaki S, Kubota M, Hirahara T, Kodama K, et al. Experimental infection of Getah virus in swine. Jpn J Vet Sci. (1988) 50:679–84. doi: 10.1292/jvms1939.50.679
229. Li YY, Liu H, Fu SH, Li XL, Guo XF, Li MH, et al. From discovery to spread: the evolution and phylogeny of Getah virus. Infect Genet Evol. (2017) 55:48–55. doi: 10.1016/j.meegid.2017.08.016
230. Chang CY, Huang CC, Huang TS, Deng MC, Jong MH, Wang FI. Isolation and characterization of a Sagiyama virus from domestic pigs. J Vet Diagn Invest. (2006) 18:156–61. doi: 10.1177/104063870601800203
231. Nemoto M, Bannai H, Tsujimura K, Kobayashi M, Kikuchi T, Yamanaka T, et al. Getah virus infection among racehorses, Japan, 2014. Emerg Infect Dis. (2015) 21:883–5. doi: 10.3201/eid2105.141975
232. Bannai H, Ochi A, Nemoto M, Tsujimura K, Yamanaka T, Kondo T. A 2015 outbreak of Getah virus infection occurring among Japanese racehorses sequentially to an outbreak in 2014 at the same site. BMC Vet Res. (2016) 12:98. doi: 10.1186/s12917-016-0741-5
233. Li Y, Fu S, Guo X, Li X, Li M, Wang L, et al. Serological survey of Getah virus in domestic animals in Yunnan Province, China. Vector Borne Zoonotic Dis. (2019) 19:59–61. doi: 10.1089/vbz.2018.2273
234. Lu G, Ou J, Ji J, Ren Z, Hu X, Wang C, et al. Emergence of Getah virus infection in horse with fever in China, 2018. Front Microbiol. (2019) 10:1416. doi: 10.3389/fmicb.2019.01416
235. Kaneko N, Inaba Y, Akashi H, Miura Y, Shorthose J, Kurashige K. Isolation of a new bovine ephemeral fever group virus. Aust Vet J. (1985) 62:388–9. doi: 10.1111/j.1751-0813.1985.tb14220.x
236. Kaneko N, Inaba Y, Akashi H, Kurashige K. Further studies on FUK-11 virus-withdrawal of a member of bovine ephemeral fever group viruses. Aust Vet J. (1987) 64:289. doi: 10.1111/j.1751-0813.1987.tb15968.x
237. Calisher CH, Karabatsos N, Zeller H, Digoutte JP, Tesh RB, Shope RE, et al. Antigenic relationships among rhabdoviruses from vertebrates and hematophagous arthropods. Intervirology. (1989) 30:241–57. doi: 10.1159/000150100
238. Noda M, Inaba Y, Banjo M, Kubo M. Isolation of Fukuoka virus, a member of the Kern Canyon serogroup viruses of the family Rhabdoviridae, from cattle. Vet Microbiol. (1992) 32:267–71. doi: 10.1016/0378-1135(92)90149-N
239. Blasdell KR, Guzman H, Widen SG, Firth C, Wood TG, Holmes EC, et al. Ledantevirus: a proposed new genus in the Rhabdoviridae has a strong ecological association with bats. Am J Trop Med Hyg. (2015) 92:405–10. doi: 10.4269/ajtmh.14-0606
240. Sakai K, Hagiwara K, Omatsu T, Hamasaki C, Kuwata R, Shimoda H, et al. Isolation and characterization of a novel Rhabdovirus from a wild boar (Sus scrofa) in Japan. Vet Microbiol. (2015) 179:197–203. doi: 10.1016/j.vetmic.2015.05.013
241. Kapoor A, Tesh RB, Duraisamy R, Popov VL, Travassos da Rosa AP, Lipkin WI. A novel mosquito-borne orbivirus species found in South-east Asia. J Gen Virol. (2013) 94:1051–7. doi: 10.1099/vir.0.046748-0
242. Sadeghi M, Popov V, Guzman H, Phan TG, Vasilakis N, Tesh R, et al. Genomes of viral isolates derived from different mosquito species. Virus Res. (2017) 242:49–57. doi: 10.1016/j.virusres.2017.08.012
243. Kato T, Aizawa M, Takayoshi K, Yanase T, Tanaka S, Shirafuji H, et al. Full genome sequence of a Sathuvachari virus strain isolated in the southwestern-most archipelago of Japan. Virus Genes. (2018) 54:729–32. doi: 10.1007/s11262-018-1592-x
244. Li M, Zheng Y, Zhao G, Fu S, Wang D, Wang Z, et al. Tibet orbivirus, a novel orbivirus species isolated from Anopheles maculatus mosquitoes in Tibet, China. PLoS ONE. (2014) 9:e88738. doi: 10.1371/journal.pone.0088738
245. Lei W, Guo X, Fu S, Feng Y, Nie K, Song J, et al. Isolation of Tibet orbivirus, TIBOV, from Culicoides collected in Yunnan, China. PLoS ONE. (2015) 10:e0136257. doi: 10.1371/journal.pone.0136257
246. Wang J, Li H, He Y, Zhou Y, Xin A, Liao D, et al. Isolation of Tibet orbivirus from Culicoides and associated infections in livestock in Yunnan, China. Virol J. (2017) 14:105. doi: 10.1186/s12985-017-0774-9
247. Qin XC, Shi M, Tian JH, Lin XD, Gao DY, He JR, et al. A tick-borne segmented RNA virus contains genome segments derived from unsegmented viral ancestors. Proc Natl Acad Sci USA. (2014) 111:6744–9. doi: 10.1073/pnas.1324194111
248. Shi M, Lin XD, Vasilakis N, Tian JH, Li CX, Chen LJ, et al. Divergent viruses discovered in arthropods and vertebrates revise the evolutionary history of the Flaviviridae and related viruses. J Virol. (2015) 90:659–9. doi: 10.1128/JVI.02036-15
249. Jia N, Liu HB, Ni XB, Bell-Sakyi L, Zheng YC, Song JL, et al. Emergence of human infection with Jingmen tick virus in China: a retrospective study. EBioMedicine. (2019) 43:317–24. doi: 10.1016/j.ebiom.2019.04.004
250. Carpenter S, Veronesi E, Mullens B, Venter G. Vector competence of Culicoides for arboviruses: three major periods of research, their influence on current studies and future directions. Rev Sci Tech. (2015) 34:97–112. doi: 10.20506/rst.34.1.2347
251. Sick F, Beer M, Kampen H, Wernike K. Culicoides biting midges-underestimated vectors for arboviruses of public health and veterinary importance. Viruses. (2019) 11:E376. doi: 10.3390/v11040376
252. Manley R, Harrup LE, Veronesi E, Stubbins F, Stoner J, Gubbins S, et al. Testing of UK populations of Culex pipiens L. for Schmallenberg virus vector competence and their colonization. PLoS ONE. (2015) 10:e0134453. doi: 10.1371/journal.pone.0134453
253. Purse BV, Carpenter S, Venter GJ, Bellis G, Mullens BA. Bionomics of temperate and tropical Culicoides midges: knowledge gaps and consequences for transmission of Culicoides-borne viruses. Annu Rev Entomol. (2015) 60:373–92. doi: 10.1146/annurev-ento-010814-020614
254. Muller MJ. Veterinary arbovirus vectors in Australia –a retrospective. Vet Microbiol. (1995) 46:101–6. doi: 10.1016/0378-1135(95)00076-M
255. Wirth WW, Hubert A. The Culicoides of Southeast Asia (Diptera: Ceratopogonidae). Mem Am Entomol Inst. (1989) 44:1–508.
256. Henna M, Shinjo K, Kokuba T. Identification of biting midges (Culicoides) and their distribution in Okinawa. Bull Okinawa Prefect Inst Anim Health. (1991) 27:43–9.
257. Yanase T, Hirata M, Matsumori Y, Matsumura M, Kato T, Shirafuji H, et al. Detection of Culicoides brevitarsis activity in Kyushu. J Vet Med Sci. (2011) 73:1649–52. doi: 10.1292/jvms.11-0231
258. Bellis G, Dyce A, Gopurenko D, Yanase T, Carros C, Labushagne K, et al. Revision of the Culicoides (Avaritia) Imicola complex Khamala & Kettle (Diptera: Ceratopogonidae) from the Australian region. Zootxa. (2014) 3768:401–27. doi: 10.11646/zootaxa.3768.4.1
259. Gopurenko D, Bellis GA, Yanase T, Wardhana AH, Thepparat A, Wang J, et al. Integrative taxonomy to investigate species boundaries within Culicoides (Diptera: Ceratopogonidae): a case study using subgenus Avaritia from Australasia and Eastern Asia. Vet Ital. (2015) 51:345–78. doi: 10.12834/VetIt.515.2463.2
260. Yanase T, Kato T, Katagiri Y, Aizawa M, Nakamura K, Kokuba T, et al. Isolation and characterization of bluetongue virus from Culicoides brevitarsis (Diptera: Ceratopogonidae) in Okinawa. Med Entomol Zool. (2010) 61:85–91. doi: 10.7601/mez.61.85
261. Yanase T, Kato T, Hayama Y, Shirafuji H, Yamakawa M, Tanaka S. Oral susceptibility of Japanese Culicoides (Diptera: Ceratopogonidae) species to Akabane virus. J Med Entomol. (2019) 56:533–9. doi: 10.1093/jme/tjy201
262. Bellis GA, Gibson DS, Polkinghorne IG, Johnson SJ, Flanagan M. Infection of Culicoides brevitarsis and C. wadai (Diptera: Ceratopogonidae) with four Australian serotypes of bluetongue virus. J Med Entomol. (1994) 31:382–7. doi: 10.1093/jmedent/31.3.382
263. Carpenter S, Lunt HL, Arav D, Venter GJ, Mellor PS. Oral susceptibility to bluetongue virus of Culicoides (Diptera: Ceratopogonidae) from the United Kingdom. J Med Entomol. (2006) 43:73–8. doi: 10.1093/jmedent/43.1.73
264. Venter GJ. Culicoides spp. (Diptera: Ceratopogonidae) as vectors of bluetongue virus in South Africa - a review. Vet Ital. (2015) 51:325–33. doi: 10.12834/VetIt.505.2436.2
265. Pagès N, Talavera S, Verdún M, Pujol N, Valle M, Bensaid A, et al. Schmallenberg virus detection in Culicoides biting midges in Spain: first laboratory evidence for highly efficient infection of Culicoides of the Obsoletus complex and Culicoides imicola. Transbound Emerg Dis. (2018) 65:e1–6. doi: 10.1111/tbed.12653
266. Barber J, Harrup LE, Silk R, Veronesi E, Gubbins S, Bachanek-Bankowska K, et al. Blood-feeding, susceptibility to infection with Schmallenberg virus and phylogenetics of Culicoides (Diptera: Ceratopogonidae) from the United Kingdom. Parasit Vectors. (2018) 11:116. doi: 10.1186/s13071-018-2650-x
267. Yanase T, Hayama Y, Shirafuji H, Tsutsui T, Terada Y. Surveillance of Culicoides biting midges in northern Honshu, Japan, during the period of Akabane virus spread. J Vet Med Sci. (2019) 81:1496–503. doi: 10.1292/jvms.19-0303
268. McDonald JL, Lu LC. Female Culicoides of Taiwan with descriptions of new species (Diptera, Ceratopogonidae). J Med Entomol. (1972) 9:396–417. doi: 10.1093/jmedent/9.5.396
269. Oem JK, Chung JY, Kwon MS, Kim TK, Lee TU, Bae YC. Abundance of biting midge species (Diptera: Ceratopogonidae, Culicoides spp.) on cattle farms in Korea. J Vet Sci. (2013) 14:91–4. doi: 10.4142/jvs.2013.14.1.91
270. Chang QQ, Jiang XH, Liu GP, Li XF, Hou XH. A species checklist of the subgenus Culicoides (Avaritia) in China, with a description of a new species (Diptera, Ceratopogonidae). Zookeys. (2017) 4:117–35. doi: 10.3897/zookeys.706.13535
271. Liu Y, Tao H, Yu Y, Yue L, Xia W, Zheng W, et al. Molecular differentiation and species composition of genus Culicoides biting midges (Diptera, Ceratopogonidae) in different habitats in southern China. Vet Parasitol. (2018) 254:49–57. doi: 10.1016/j.vetpar.2018.02.035
272. Venter GJ, Hamblin C, Paweska JT. Determination of the oral susceptibility of South African livestock-associated biting midges, Culicoides species, to bovine ephemeral fever virus. Med Vet Entomol. (2003) 17:133–7. doi: 10.1046/j.1365-2915.2003.00414.x
273. Carpenter S, Wilson A, Mellor PS. Culicoides and the emergence of bluetongue virus in northern Europe. Trends Microbiol. (2009) 17:172–8. doi: 10.1016/j.tim.2009.01.001
274. Balenghien T, Pagès N, Goffredo M, Carpenter S, Augot D, Jacquier E, et al. The emergence of Schmallenberg virus across Culicoides communities and ecosystems in Europe. Prev Vet Med. (2014) 116:360–9. doi: 10.1016/j.prevetmed.2014.03.007
275. Aziz-Boaron O, Gleser D, Yadin H, Gelman B, Kedmi M, Galon N, et al. The protective effectiveness of an inactivated bovine ephemeral fever virus vaccine. Vet Microbiol. (2014) 173:1–8. doi: 10.1016/j.vetmic.2014.06.021
276. Aziz-Boaron O, Leibovitz K, Gelman B, Kedmi M, Klement E. Safety, immunogenicity and duration of immunity elicited by an inactivated bovine ephemeral fever vaccine. PLoS ONE. (2013) 8:e82217. doi: 10.1371/journal.pone.0082217
277. Zheng FY, Chen QW, Li Z, Gong XW, Wang JD, Yin H. Experimental infection with bovine ephemeral fever virus and analysis of its antibody response cattle. Res Vet Sci. (2016) 104:146–51. doi: 10.1016/j.rvsc.2015.12.018
278. Gyawali N, Bradbury RS, Aaskov JG, Taylor-Robinson AW. Neglected Australian arboviruses: quam gravis? Microbes Infect. (2017) 19:388–401. doi: 10.1016/j.micinf.2017.05.002
279. Trinidad L, Blasdell KR, Joubert DA, Davis SS, Melville L, Kirkland PD, et al. Evolution of bovine ephemeral fever virus in the Australian episystem. J Virol. (2014) 88:1525–35. doi: 10.1128/JVI.02797-13
280. Xia H, Liu H, Zhao L, Atoni E, Wang Y, Yuan Z. First isolation and characterization of a group C Banna virus (BAV) from Anopheles sinensis mosquitoes in Hubei, China. Viruses. (2018) 10:555. doi: 10.3390/v10100555
281. Chaisirirat T, Sangthong P, Arunvipas P, Petcharat N, Thangthamniyom N, Chumsing W, et al. Molecular characterization of bovine ephemeral fever virus in Thailand between 2013 and 2017. Vet Microbiol. (2019) 227:1–7. doi: 10.1016/j.vetmic.2018.10.013
282. Duan YL, Miao HS, Liao F, Kou ML, Li ZH, Wang Z, et al. The serologic investigation and viral isolation of bluetongue virus in Shangri-La in Southwest China. Transbound Emerg Dis. (2019) 66:2353–61. doi: 10.1111/tbed.13292
283. Schuh AJ, Ward MJ, Brown AJ, Barrett AD. Phylogeography of Japanese encephalitis virus: genotype is associated with climate. PLoS Negl Trop Dis. (2013) 7:e2411. doi: 10.1371/journal.pntd.0002411
284. Miura Y, Inaba Y, Tsuda T, Tokuhisa S, Sato K, Akashi H, et al. A survey of antibodies to arthropod-borne viruses in Indonesian cattle. Nihon Juigaku Zasshi. (1982) 44:857–63. doi: 10.1292/jvms1939.44.857
285. Daniels PW, Sendow I, Soleha E, Sukarsih, Hunt NT, Bahri S. Australian-Indonesian collaboration in veterinary arbovirology-a review. Vet Microbiol. (1995) 46:151–174. doi: 10.1016/0378-1135(95)00080-T
286. Bryant JE, Crabtree MB, Nam VS, Yen NT, Duc HM, Miller BR. Isolation of arboviruses from mosquitoes collected in northern Vietnam. Am J Trop Med Hyg. (2005) 73:470–3. doi: 10.4269/ajtmh.2005.73.470
287. Purnomo Edi S, Ibrahim A, Sukoco R, Bunali L, Taguchi M, Kato T, et al. Molecular characterization of an Akabane virus isolate from West Java, Indonesia. J Vet Med Sci. (2017) 79:774–9. doi: 10.1292/jvms.17-0009
288. Elbers AR, Koenraadt CJ, Meiswinkel R. Mosquitoes and Culicoides biting midges: vector range and the influence of climate change. Rev Sci Tech. (2015) 34:123–37. doi: 10.20506/rst.34.1.2349
289. Burgin LE, Gloster J, Sanders C, Mellor PS, Gubbins S, Carpenter S. Investigating incursions of bluetongue virus using a model of long-distance Culicoides biting midge dispersal. Transbound Emerg Dis. (2013) 60:263–72. doi: 10.1111/j.1865-1682.2012.01345.x
290. Durr PA, Graham K, van Klinken RD. Sellers' revisited: a big data reassessment of historical outbreaks of bluetongue and African horse sickness due to the long-distance wind dispersion of Culicoides midges. Front Vet Sci. (2017) 4:98. doi: 10.3389/fvets.2017.00098
291. Eagles D, Melville L, Weir R, Davis S, Bellis G, Zalucki MP. Long-distance aerial dispersal modelling of Culicoides biting midges: case studies of incursions into Australia. BMC Vet Res. (2014) 10:135. doi: 10.1186/1746-6148-10-135
292. Thompson PN, Etter E. Epidemiological surveillance methods for vector-borne diseases. Rev Sci Tech. (2015) 34:235–47. doi: 10.20506/rst.34.1.2356
293. Vasilakis N, Tesh RB, Popov VL, Widen SG, Wood TG, Forrester NL. Exploiting the legacy of the arbovirus hunters. Viruses. (2019) 11:471. doi: 10.3390/v11050471
294. Hayama Y, Moriguchi S, Yanase T, Ishikura Y, Abe S, Higashi T, et al. Spatial epidemiological analysis of bovine encephalomyelitis outbreaks caused by Akabane virus infection in western Japan in 2011. Trop Anim Health Prod. (2016) 48:843–7. doi: 10.1007/s11250-016-1014-9
Keywords: arthropod-borne virus, cattle, Culicoides, mosquito, orbivirus, orthobunyavirus, rhabdovirus
Citation: Yanase T, Murota K and Hayama Y (2020) Endemic and Emerging Arboviruses in Domestic Ruminants in East Asia. Front. Vet. Sci. 7:168. doi: 10.3389/fvets.2020.00168
Received: 05 October 2019; Accepted: 10 March 2020;
Published: 07 April 2020.
Edited by:
William C. Wilson, United States Department of Agriculture, United StatesReviewed by:
Diego G. Diel, Cornell University, United StatesSushila Maan, Lala Lajpat Rai University of Veterinary and Animal Sciences, India
Copyright © 2020 Yanase, Murota and Hayama. This is an open-access article distributed under the terms of the Creative Commons Attribution License (CC BY). The use, distribution or reproduction in other forums is permitted, provided the original author(s) and the copyright owner(s) are credited and that the original publication in this journal is cited, in accordance with accepted academic practice. No use, distribution or reproduction is permitted which does not comply with these terms.
*Correspondence: Tohru Yanase, dHlhbmFzZSYjeDAwMDQwO2FmZnJjLmdvLmpw
†ORCID: Tohru Yanase orcid.org/0000-0003-0838-9156