- 1Department of Animal Production, Faculty of Agriculture, Kafrelsheikh University, Kafr El Sheikh, Egypt
- 2Department of Poultry Production, Faculty of Agriculture, Kafrelsheikh University, Kafr El Sheikh, Egypt
- 3Department of Aquatic Animals Diseases and Management, Faculty of Veterinary Medicine, Benha University, Benha, Egypt
- 4Biochemistry Department, Faculty of Veterinary Medicine, Matrouh University, Matrouh, Egypt
- 5Department of Nutrition and Veterinary Clinical Nutrition, Faculty of Veterinary Medicine, Damanhour University, Damanhour, Egypt
- 6Department of Zoology, College of Science, King Saud University, Riyadh, Saudi Arabia
The aim of the present study was to examine the effects of dietary inclusion of fermented poultry by-product meal (FPBM) on growth performance, digestive enzymes activity, innate immunity, and antioxidant capacity in Nile tilapia (Oreochromis niloticus). A basal diet containing fish meal and soybean meal was considered as a control (Con), and four other diets were produced by inclusion of 10, 20, 30, or 40% FPBM (FPBM10, FPBM20, FPBM30, and FPBM40 diets). The experiment was done in triplicates (20 fish per replicate) and the fish were fed the test diets to visual satiety twice daily for 8 weeks. The groups of fish fed the FPBM10 and FPBM20 diets showed significantly (P < 0.05) higher weight gain and specific growth rate, and lower feed conversion ratio than those fed the Con and FPBM40 diets. Moreover, inclusion of 40% FPBM led to significant reduction of feed intake compared to the other treatments. FPBM at all the tested levels improved intestinal protease activity and lipase activity was enhanced at 10–30% inclusion levels. Furthermore, the FPBM10 and FPBM20 groups revealed significantly higher amylase activity than the other treatments. The FPBM10 group exhibited significantly higher phagocytic activity than the control group and phagocytic index was enhanced by dietary inclusion of 10–30% FPBM. However, inclusion of over 30% FPBM led to significant reduction of lysozyme, phagocytic, and bactericidal activities compared to the control group. Further, FPBM10 and FPBM20 diets increased the serum IgM levels, while NBT was significantly increased by feeding FPBM10 diet compared with FPBM30 and FPBM40 groups (P < 0.05). The group fed the FPBM30 diet showed significantly higher glutathione peroxidase activity than the control group. According to the analysis of the data by the polynomial regression, the inclusion of FPBM at 11.17–25.14% can be applied effectively in the diets of tilapia for better growth performance and health condition.
Introduction
The global supply of fish for human consumption has outpaced population growth in the past five decades (1). This has raised attention to increasing the development of the aquaculture industry leading to advanced production technologies and culture systems worldwide (2). Therefore, the industry would soon run out of sufficient quantities of fish oil and fishmeal (FM) (3, 4). As a result of increasing demand, limited supply, and a dramatic increase in FM price, suitable alternative protein sources for fish feed have recently been intensively studied (5). Any reduction in feed costs with a preserved health status of fish is bound to have a direct positive effect on the profitability of aquaculture.
The use of protein sources of animal origin as FM replacement in fish feed is a growing trend in the aquaculture industry (6). In this context, among commercially available animal protein alternatives, poultry by product meal (PBM) has been one of the best nutritional value and amino acid balances (lipids, 12–15% and proteins, 58–65%), except for the low level of lysine and methionine (7, 8). It is widely available at competitive prices and is therefore one of the main dietary protein alternatives to FM in the feed of cultured species (9–11). Potential problems in PBM feeding exist due to the existence of fibers and high levels of lipids, which can cause high oxidation, malnutrition, and lower palatability (12, 13). To reduce the costs of the feed and environmental pollution, PBM can be treated with suitable microorganisms with beneficial effects (14, 15). Recently, the fermentation strategy has been applied to balance the nutritional value of animal protein sources (16). The microorganisms can be active under anaerobic conditions in order to dissimilate the ingredients organic contents (5). By this way the long chain amino acids and fatty acids can be shortened to be more available for absorption in the gastrointestinal tract of fish (17–19). It has been reported that the animal proteins (e.g., poultry feather meal, fish meal, and animal protein blend) fermented with various microorganisms such as fungus (Aspergillus sp.), bacteria (Lactobacillus sp.), and yeast (Saccharomyces cerevisiae) shown to have an enhanced nutritional status in terms of higher protein and lower fiber fractions in comparison to the respective untreated ones (5, 16, 20).
Though fermented animal proteins showed better performance than the respective untreated ones, still their inclusion level by substituting FM is not yet at the satisfied level. Samaddar et al. (16) concluded that there was no negative effect on growth of Labeo rohita when dietary FM was substituted up to 75% using a fermented animal protein blend. Mondal (20) was also able to substitute 75% FM with fermented PBM (FPBM) in the diet of Indian major carp (Catla catla). To date, no data available about using FPBM on the growth performance and health condition of Nile tilapia (Oreochromis niloticus).
Based on records of fish growth, palatability, digestive enzymes activity, apparent digestibility coefficients of nutrients, nutrient retention, blood biochemistry, and histopathologic aspects (21–23), FPBM appears to attribute benefits on fish quality and nutrients retention efficiency without indications of negative physiological impacts for the fish in short term studies. As a principal species, Nile tilapia is cultured widely as the second major candidate for aquaculture (24). Though the productivity of tilapia is rapidly increasing, profitability of the tilapia production is being decreased due to the progressive increase in feed cost (25, 26). Thus, the present study was conducted to evaluate the effects of dietary inclusion levels of FPBM on growth performance, digestive enzymes activity, and immunity of Nile tilapia.
Materials and Methods
The experimental procedure was approved by the Institutional Animal Care and Use Committee in Kafrelsheikh University (Kafrelsheikh, Egypt).
Fermentation and Diet Preparation
Fine powder of PBM was kindly provided by Elsodor company (Al-Sadat city, Egypt). PBM was adjusted to a moisture content of 60–65% using de-ionized water in a 1 L glass container with six sets of replications for each. They were autoclaved at 121°C (105 kPa) (20 min) then cooled to room temperature. Cane molasses was collected from the local market and used as a source of fermentable carbohydrates for S. cerevisiae activation. To the sterilized ingredients, actively growing culture (2.27 × 1010 CFU per g) of S. cerevisiae (Shanghai Gosun Biotechnologies CO. LTD, China) was inoculated and thoroughly mixed using a sterile glass rod manually. All the inoculated samples were incubated at 30 ± 2°C for 4 days to carry out the fermentation process. Prior to this, the fermentation conditions were optimized for moisture (50, 55, 60, 65, and 70%), temperature (28, 30, 32, and 36°C), inoculums rate (1, 2.5, 5, 7.5, and 10%), pH (5.5, 6, 6.5, 7, 7.5), and incubation period (1, 2, 3, 4, and 5 days). The optimized conditions of 60–65% moisture, 28–30°C temperature, 5% inoculum rate, 6.5–7 pH, and 4-day incubation period were applied in the present investigation. All the fermentation samples were mixed with a sterile glass rod two times every day (7.00 a.m. and 6.30 p.m.) for proper homogenization. After the fourth day, all the materials were transferred to a little higher temperature of 35°C to stop the fermentation process and allow to dry at the same temperature until the moisture content of fermented samples become <10%. The proximate and essential amino acid composition of FPBM is given in Table 2.
Five isonitrogenous (30.8% crude protein) and isolipidic (6.5% crude lipid) diets were formulated as shown in Table 1. The experimental diets were formulated to contain 0, 10, 20, 30, and 40% FPBM (Con, FPBM10, FPBM20, FPBM30, and FPBM40 diets) and were further balanced for crude protein, using FM, soybean meal, and other plant protein mixes (Table 1). Methionine, lysine, threonine, and tryptophan were added to obtain an equivalent indispensable amino acid profile. All ingredients were completely mixed, then added to produce a stiff dough and pelleted in a tabletop pelletizer with a 1–2 mm die, then the pellets were air dried. The diets obtained were stored at −20°C until they were used.
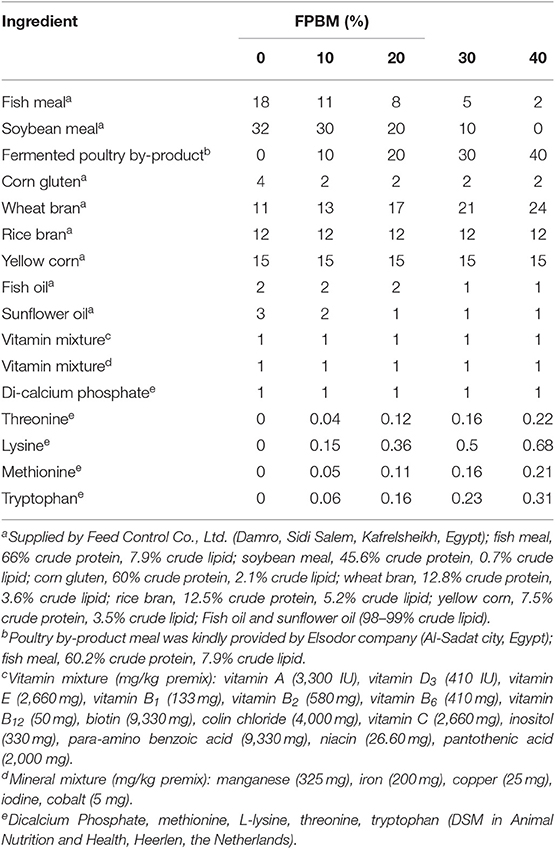
Table 1. Formulation of the experimental diets (%) used to fed Nile tilapia with varied levels of FPBM for 60 days.
Fish and Experimental Protocol
Fingerlings of Nile tilapia were obtained from a local farm (Kafrelsheikh, Egypt), and transported to Animal Production Department, Faculty of Agriculture, Kafrelsheikh University (Kafrelsheikh, Egypt). Fish was acclimatized for 2 weeks then distributed (10.6 ± 0.3 g) into 15 glass aquaria (60 L) at stocking density of 20 fish per aquarium. All the experimental aquaria were fixed with continuous aeration through single air-stone. The feeding rate was 3% of fish weight and the respective test diets were provided twice daily (8 and 15:30) for 60 days (28). After feeding time, the uneaten feed, if any were removed and 50% of water was replaced with freshly dechlorinated water. The water quality parameters including temperature, dissolved oxygen, pH, and total ammonia were carefully monitored and maintained at 24.2 ± 1.6°C, 66.32 ± 0.3 mg/L, 7.05 ± 0.6, and 0.32 ± 0.01 mg/L, respectively.
Sample Collection
All fish were fasted 24 h before the sampling. Before sampling, all fish were anesthetized with tricaine methane sulphonate (MS-222) at 25 mg/L, after which they were counted and weighed for growth and biometric indexes. Fish were measured individually for the final body weight and length. Blood samples were collected from the caudal vein of Nile tilapia (3 fish/aquarium) and pooled together. A portion of the blood was put into EDTA coated vials for whole blood and another part in non-coated vials for serum collection. Serum samples were separated by centrifugation of the coagulated blood at 3,000 rpm for 15 min and stored at −20°C for further analysis.
The whole intestine was then sampled from nine fish/group in an ice bath for the analysis of digestive enzymes. The collected intestine samples were pooled and immediately homogenized with cold PBS (pH 7.5; 1 g per 10 mL) and centrifuged at 8,000 ppm for 5 min at 4°C and the supernatants were kept at −80°C until assayed.
Chemical Analysis
AOAC (29) standard method was used to confirm the nutritional profile of each diet, PBM, and FPBM (Table 2). Amino Acid Analyzer (Biochrom 30) used for identifying the amino acid profile (EAA) by following the protocol of the manufacturer.
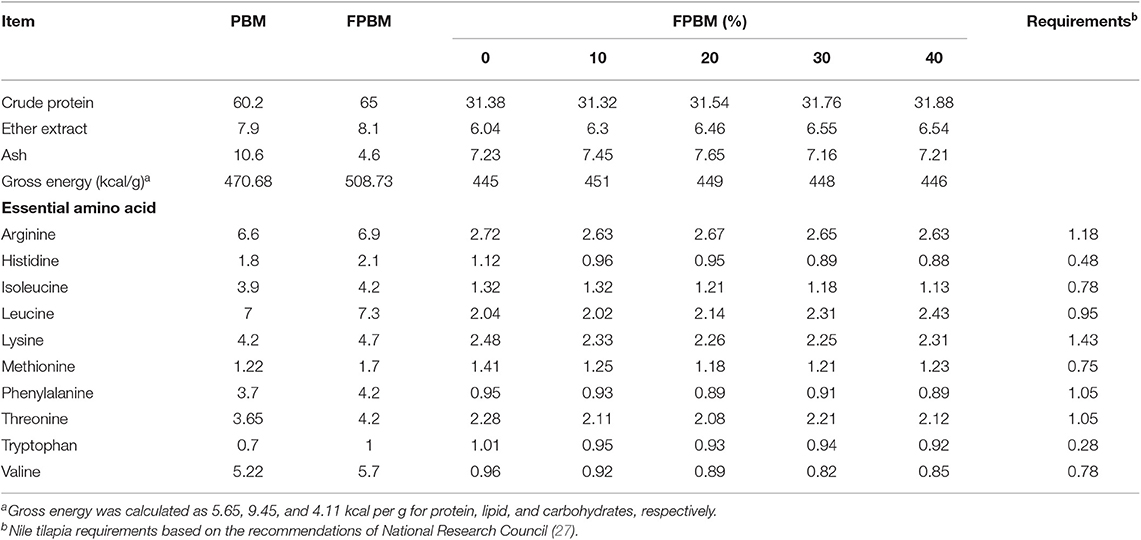
Table 2. Proximate composition (%, dry matter basis) of PBM, FPBM, and the experimental diets (%) used to fed Nile tilapia with varied levels of FPBM for 60 days.
Growth Performance Calculations
All fish per tank were weighed and counted separately during the final sampling.
Digestive Enzymes Activity
The total protein content was measured by Lowry et al. (30) method, in which BSA was used as a standard. According to Anson (31), Folin and Ciocalteus Phenol Reagent was used for measuring protease activity and iodine solution for measuring amylase activity to detect non-hydrolyzed starch according to Jiang (32) and Worthington (33). The protease and amylase activities were expressed as “U per mg of protein.” On the basis of the protocol described by Borlongan (34) and Jin (35) with olive oil as substrate, the specific activity of lipase was assessed. The activity of lipase was expressed as intestinal content “U per g intestine content.”
Immunological Assays
The RA-50 chemistry analyzer (Bayer) used the total serum protein using ready-made kits of Spinreact Company Spain. ELISA kit of Cusabio; Wuhan, Hubei, China was used for immunoglobulin M (IgM) determination. The IgM result was expressed in mg per dl. According to Secombes (36), the nitro-blue-tetrazolium (NBT) was used for the detection of the respiratory burst activity of the blood using a micro-plate reader (Optica, Mikura Ltd., UK) at 630 nm.
The activity of lysozyme was measured by following Parry et al. (37). The result was expressed as “a 0.001/min reduction in absorption.” Following Rainger and Rowley (38), serum bactericidal activity against Aeromonas hydrophila was detected. As a survival index (SI), the results were recorded by this equation.
SI = CFU at the end/CFU at the beginning/100.
By following Kawahara et al. (39), the phagocytic activity and phagocytic index were determined by these equations:
Phagocytic activity = macrophages containing yeast/macrophages total number × 100.
Phagocytic index = phagocytized cells number/phagocytic cells number.
Oxidative Status
Serum activities of antioxidant enzymes [superoxide dismutase (SOD), catalase (CAT), and glutathione peroxidase (GPx)] and concentration of lipid peroxidation [malonaldehyde (MDA)] were determined by using kits of Cusabio Biotech Co., Ltd; China.
Statistical Analysis
All data obtained were analyzed by using one-way ANOVA SPSS version 22 except quadratic effects of various dietary FPBM levels on the observed response variables by polynomial contrasts and the optimum FPBM level by polynomial regression analysis (40).
Results
Nutrient Composition of PBM, FPBM, and Formulated Diets
Data of PBM, FPBM, and formulated diets analysis (Table 2) revealed that crude protein and ether extract contents are higher in FPBM (65 and 8.1%) compared to non-fermented PBM (60.2 and 7.9%). Similar trend was observed for gross energy between FPBM and non-fermented PBM and was in the range of 470.68–508.73 kcal/g. Though experimental diets were formulated to be balanced in protein (31.32–31.88%) and lipid (6.04–6.55%), the analyzed essential amino acids showed relatively higher values in FPBM compared to non-fermented PBM. The dietary change had a slight deviation in essential amino acids quantity, the nutritional requirement of Nile tilapia in essential amino acids was fulfilled even at higher substitution of FM in our study.
Tilapia Growth Performance and Biometrics
All growth performance parameters demonstrated in Table 3 where rates of the survival were 93.3 and 98.6% without significant (P > 0.05) alterations. The condition factor also was not significantly affected by the inclusion of FPBM (P > 0.05).
It was observed that the inclusion of FPBM has a significant influence on fish performance parameters including FBW, WG, SGR, FI, and FCR where P-value was 0.003, 0.003, 0.002, 0.005, and 0.04, respectively (Table 3). The groups of fish fed the FPBM10 and FPBM20 diets showed significantly (P < 0.05) higher weight gain and specific growth rate, and lower feed conversion ratio than those fed the Con and FPBM40 diets. Moreover, inclusion of 40% FPBM led to significant reduction of feed intake compared to the other treatments. The growth performance and dietary FPBM levels were expressed by polynomial regression equations where, FBW (y = −0.0108x2 + 0.4121x + 41.113, R2 = 0.9699, optimal dose = 19.08%), WG (y = −0.1047x2 + 3.9733x + 287.63, R2 = 0.9795, optimal dose = 18.97%), SGR (y = −0.0004x2 + 0.0164x + 2.2576, R2 = 0.9829, optimal dose = 20.5%), and FCR (y = 0.0003x2 – 0.0127x + 1.3555, R2 = 0.9049, optimal dose = 21.17%) (Table 7).
Digestive Enzymes Activity
FPBM at all the tested levels improved intestinal protease activity and lipase activity was enhanced at 10–30% inclusion levels (P < 0.05) (Table 4). Furthermore, the FPBM10 and FPBM20 groups revealed significantly higher amylase activity than the other treatments (P < 0.05). The lipase, amylase, and protease and dietary FPBM levels were expressed by regression equations (quadratic) where, amylase (y = −0.0108x2 + 0.377x + 31.913, R2 = 0.8208, optimal dose = 17.45%), lipase (y = −0.0318x2 + 1.1851x + 23.374, R2 = 0.8936, optimal dose = 18.63%), and protease (y = −0.0069x2 + 0.3469x + 29.239; R2 = 0.9711, optimal dose = 25.14%) (Table 7).
Immune Responses
The FPBM10 group exhibited significantly (P < 0.05) higher phagocytic activity than the control group and phagocytic index was enhanced by dietary inclusion of 10–30% FPBM (Table 5). However, inclusion of over 30% FPBM led to significant reduction of lysozyme, phagocytic, and bactericidal activities compared to the control group (P < 0.05). Further, FPBM10 and FPBM20 diets increased the serum IgM levels, while NBT was significantly increased by feeding FPBM10 diet compared with FPBM30 and FPBM40 groups (P < 0.05) (Table 5). However, the blood total protein was non-significantly affected by FPBM inclusion in tilapia diet (P > 0.05). The relationships between the immune responses and dietary FPBM levels were, lysozyme activity (y = −0.0229x2 + 0.5396x + 53.515, R2 = 0.9086, optimal dose = 11.76%), IgM (y = −0.0018x2 + 0.049x + 4.1333, R2 = 0.8607, optimal dose = 13.61%), lysozyme activity (y = −0.0067x2 + 0.15x + 31.087, R2 = 0.893, optimal dose = 13.61%), and NBT (y = −5E−05x2 + 0.0014x + 0.2355, R2 = 0.7548, optimal dose = 14%) (Table 7).
Oxidative Status
The blood oxidative parameters (SOD, CAT, GPx, and MDA) of tilapia fed FPBM were displayed in Table 6. The group fed the FPBM30 diet showed significantly higher GPx than the control group (P <0.05). The relationship between GPx and dietary FPBM levels is y = −0.0046x2 + 0.2094x + 26.038, R2 = 0.8513, optimal dose = 22.76% (Table 7).
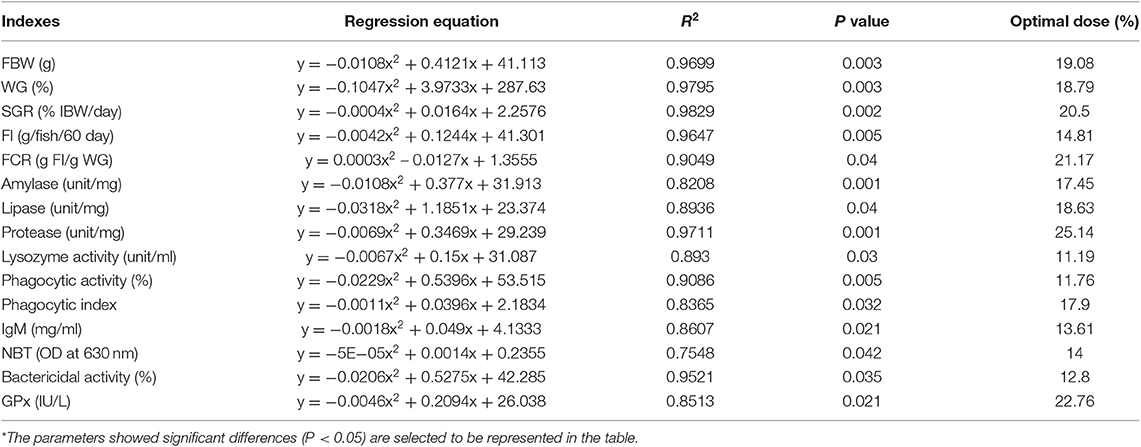
Table 7. Regression analysis based on different parameters of Nile tilapia fed FPBM diets for 60 days*.
Discussion
Tilapia Growth Performance
The intensification of using a blend of fermented animal proteins has been made it necessary to formulate the most cost-effective balanced feed with the sound nutrition. Most of the studies concluded that the growth performance retardation was detected in the fish fed 75% of FM substitution, irrespective of the inclusion of PBM (10, 12, 13, 27, 41–46). In contrast, the results of the present study revealed that FPBM was successfully included up to 40% in the diet and did not impair Nile tilapia feed efficiency (FI and FCR) and growth performance. Moreover, by including FPBM at 20%, fish obtained better growth performance compared to control. Similarly, Juvenile L. rohita tolerates up to 75% replacement of FM with FPBM (16).
In this study, the survival rate of fish fed diets with FPBM remained high during the trial. This agrees with numerous studies that tested FPBM protein in the diets of L. rohita (16) and Indian major carp (20). The obtained results in the current study revealed that growth parameters of fish were increased at 19.1–25.14% levels of FPBM. However, fish fed the diet with high level of FPBM (40%) showed reduced growth performance and feed utilization. In the case of high inclusion level (40%), the low digestive enzyme activity and feed palatability are among the factors, which could reduce the feed efficiency and accordingly the growth of fish (46). Fuertes et al. (6) reported that low feed intake and low feed efficiency ratio (increased FCR) resulted from high PBM inclusion levels in crayfish (Pacifastacus leniusculus). The fermentation process can increase the apparent digestibility of nutrients in animals (5, 47). The improved feed efficiency in FPBM20 level could be attributed to the level of peptides which increased as a result of the fermentation process by enzymatic degradation, where small-size peptides resulted from protein fractions degradation. These small-size peptides can be absorbed more efficiently by cells of the intestine (48).
The lowest FCR was observed for fish fed the diet with FPBM where 20% was included and did not significantly different from those obtained for the groups fed FPBM10 and FPBM30 levels, suggesting beneficial role of using S. cerevisiae to ferment PBM. However, the highest inclusion level of FPBM significantly (P < 0.05) increased FCR similar to the control group. It also indicates that the inclusion of high level of FPBM (40%) would not produce any stress or negative impact on the feed efficiency of Nile tilapia.
Digestive Enzymes Activity
The digestive enzymes activity can increase the feed utilization of diet in the fish intestine (49). FPBM in the experimental diets increased the amylase, lipase, and protease activities. Numerically, the highest amylase and lipase values were in FPBM10 and FPBM20 groups, while the protease activity was increased up to 40% of FPBM inclusion. However, the poorest values were noticed with the groups fed with Con. It is believed that the production of metabolites like enzymes, antimicrobial substances is not only higher in quantity during fermentation and would also be more beneficial in enhancing the nutritive values of ingredients (5, 50, 51). High levels of inclusion may weaken the digestion and use of feed by affecting digestive enzyme activity. The decreased amylase and lipase activities in fish fed FPBM40 in the current study compared to those fed the lowest levels due to the high levels of fibers and non-digestible nutrients. The effect of experimental diets, in relating to FPBM inclusion, on the activity of digestive enzymes is scarce. However, it is a necessity for further investigation in digestive enzymes at gene transcriptional level.
Immune Responses
Fermentation of PBM resulted in improved immune components measured in the current study. In this study, fish fed FPBM30 diet did not impair the measured immune responses “e.g., activities of lysozyme, phagocytosis, and bactericidal,” while the IgM and NBT levels increased in fish fed FPBM10 and FPBM20 diets. Lysozyme is a proteolytic enzyme with the double role of killing bacteria by disrupting their cell wall and triggering other immune responses such as the complement system and phagocytic cells (52). Lysozyme plays thus an important role in the innate immune system and higher lysozyme levels have been related to better fish immune status. Phagocytosis and bactericidal activity are vital cellular immune responses that can protect fish from pathogens through discovering the existing of infectious pathogens (53, 54). The lysozyme activity increased in Con, FPBM10, and FPBM20 groups and started to decrease by feeding more than 30% FPBM. A similar improvement in blood phagocytic and bactericidal activities were observed in the current study by feeding Con, FPBM10, FPBM20, and FPBM30 diets when compared to fish fed FPBM40 diet.
Natural antibodies such as immunoglobulins (IgM) play a key role in both innate and adaptative immunity, producing specific antibody responses against various antigens (55). The activity of respiratory bursts (NBT) is also necessary for the assessment of the fish general health (36, 56). Respiratory burst has a critical role in fish immune system by increasing the levels of phagocytes which can release the high levels of ROS in fish cells (54, 57). In this study, we observed that FPBM10 and FPBM20 diets increased the serum IgM levels, while NBT was significantly increased by feeding FPBM10 diet compared with FPBM30 and FPBM40 groups in Nile tilapia.
The measured immune responses (lysozyme, phagocytosis, bactericidal, and NBT activities as well as IgM level) were decreased in fish the highest inclusion level of FPBM (FPBM40), which could be as a result from impaired immunity and increased oxidative stress caused by low protein quality and higher fiber fractions in FPBM40 diet, agreeing with previous studies on the importance of optimum animal protein source used in diet formulation (5, 16, 20).
Oxidative Status
The oxidative emphasis normally happens when the creation and elimination of free radicals (ROS) are unbalanced since the oxidative damage of cultured species is directly related to the quality of diet (58). MDA is a product of lipid peroxides and high levels of ROS, which can cause damage to cell's DNA, protein, and cytoplasm (59). In this experiment, fish fed FPBM30 diet showed improved GPx without affecting the MDA level indicating improved antioxidant activity which can be attributed to the presence of bioactive compounds that may improve the antioxidant enzyme activity and regulate the ROS production (60). As an antioxidant, GPx mainly exists in cell metabolism, playing a protective role in cells by the disproportionation of toxic ROS to inactive hydrogen peroxide and oxygen molecules (61).
Conclusion
In conclusion, appropriate dietary FPBM level improved the general performances of Nile tilapia. Based on the measured parameters, the recommended inclusion levels of dietary FPBM for Nile tilapia is 11.17–25.14% diet. Fermented PBM shown to have an enhanced nutritional status in terms of higher protein and essential amino acids in comparison to the respective untreated ones. Therefore, it is believed that FPBM would be more effective rather using PBM in tilapia feed, with a great potential in reducing the pressure on FM.
Data Availability Statement
All datasets generated for this study are included in the article/supplementary material.
Ethics Statement
The experiment was conducted in accordance with the guideline of Kafrelshiekh University, Egypt. All animal protocols were approved by the Institutional Animal Care and Use Committee of Faculty of Agriculture, Kafrelsheikh University.
Author Contributions
All persons listed as authors have read, contributed to preparing the manuscript as given below: MD and MM carried out fish maintenance and sample collection. FM, AS, AA, SF, and HA carried out the experimental design, required analysis, and statistical analyses. KA-G, SM, and FA-M carried out data interpretation.
Conflict of Interest
The authors declare that the research was conducted in the absence of any commercial or financial relationships that could be construed as a potential conflict of interest.
Acknowledgments
The authors express their sincere appreciation to the Researchers Supporting Project (RSP2019-93), King Saud University, Riyadh, Saudi Arabia.
References
1. Saeidi asl MR, Adel M, Caipang CMA, Dawood MAO. Immunological responses and disease resistance of rainbow trout (Oncorhynchus mykiss) juveniles following dietary administration of stinging nettle (Urtica dioica). Fish Shellfish Immunol. (2017) 71:230–8. doi: 10.1016/j.fsi.2017.10.016
2. Moss AS, Koshio S, Ishikawa M, Yokoyama S, Nhu TH, Dawood MAO, et al. Replacement of squid and krill meal by snail meal (Buccinum striatissimum) in practical diets for juvenile of kuruma shrimp (Marsupenaeus japonicus). Aquac Res. (2018) 49:3097–106. doi: 10.1111/are.13772
3. Dossou S, Koshio S, Ishikawa M, Yokoyama S, Dawood MAO, El Basuini MF, et al. Effect of partial replacement of fish meal by fermented rapeseed meal on growth, immune response and oxidative condition of red sea bream juvenile, Pagrus major. Aquaculture. (2018) 490:228–35. doi: 10.1016/j.aquaculture.2018.02.010
4. Dossou S, Koshio S, Ishikawa M, Yokoyama S, Dawood MAO, El Basuini MF, et al. Growth performance, blood health, antioxidant status and immune response in red sea bream (Pagrus major) fed Aspergillus oryzae fermented rapeseed meal (RM-Koji). Fish Shellfish Immunol. (2018) 75:253–62. doi: 10.1016/j.fsi.2018.01.032
5. Dawood MAO, Koshio S. Application of fermentation strategy in aquafeed for sustainable aquaculture. Rev Aquac. (2019). doi: 10.1111/raq.12368
6. Fuertes J, Celada J, Carral J, Saez-Royuela M, Gonzalez-Rodriguez A. Replacement of fish meal with poultry by-product meal in practical diets for juvenile crayfish (Pacifastacus leniusculus Dana, Astacidae) from the onset of exogenous feeding. Aquaculture. (2013) 404:22–7. doi: 10.1016/j.aquaculture.2013.04.019
7. Hekmatpour F, Ghafle Marmmazi J, Zakeri M, Mousavi SM. Potential of poultry by-product meal as a main protein source in diets formulated for juvenile sobaity (Sparidentex hasta). Iran J Fish Sci. (2018) 18:873–90. Available online at: http://jifro.ir/article-1-2189-en.html
8. Hill JC, Alam MS, Watanabe WO, Carroll PM, Seaton PJ, Bourdelais AJ. Replacement of menhaden fish meal by poultry by-product meal in the diet of juvenile red porgy. N Am J Aquac. (2019) 81:81–93. doi: 10.1002/naaq.10074
9. Dawson MR, Alam MS, Watanabe WO, Carroll PM, Seaton PJ. Evaluation of poultry by-product meal as an alternative to fish meal in the diet of juvenile Black sea bass reared in a recirculating aquaculture system. N Am J Aquac. (2018) 80:74–87. doi: 10.1002/naaq.10009
10. El-Sayed AF. Total replacement of fish meal with animal protein sources in Nile tilapia, Oreochromis niloticus (L.), feeds. Aquac Res. (1998) 29:275–80. doi: 10.1046/j.1365-2109.1998.00199.x
11. Parés-Sierra G, Durazo E, Ponce MA, Badillo D, Correa-Reyes G, Viana MT. Partial to total replacement of fishmeal by poultry by-product meal in diets for juvenile rainbow trout (Oncorhynchus mykiss) and their effect on fatty acids from muscle tissue and the time required to retrieve the effect. Aquac Res. (2014) 45:1459–69. doi: 10.1111/are.12092
12. Abdel-Warith A, Russell P, Davies S. Inclusion of a commercial poultry by-product meal as a protein replacement of fish meal in practical diets for African catfish Clarias gariepinus (Burchell 1822). Aquac Res. (2001) 32:296–305. doi: 10.1046/j.1355-557x.2001.00053.x
13. Nengas I, Alexis MN, Davies SJ. High inclusion levels of poultry meals and related byproducts in diets for gilthead seabream Sparus aurata L. Aquaculture. (1999) 179:13–23. doi: 10.1016/S0044-8486(99)00148-9
14. Dossou S, Koshio S, Ishikawa M, Yokoyama S, El Basuini MF, Zaineldin AI, et al. Effects of replacing fishmeal with fermented and non-fermented rapeseed meal on the growth, immune and antioxidant responses of red sea bream (Pagrus major). Aquac Nutr. (2019) 25:508–17. doi: 10.1111/anu.12876
15. Kaviraj A, Mondal K, Mukhopadhyay PK, Turchini GM. Impact of fermented mulberry leaf and fish offal in diet formulation of Indian major carp (Labeo rohita). Proc Zool Soc. (2013) 66:64–73. doi: 10.1007/s12595-012-0052-1
16. Samaddar A, Kaviraj A, Saha S. Utilization of fermented animal by-product blend as fishmeal replacer in the diet of Labeo rohita. Aquac Rep. (2015) 1:28–36. doi: 10.1016/j.aqrep.2015.03.004
17. Dawood MAO, Koshio S. Recent advances in the role of probiotics and prebiotics in carp aquaculture: a review. Aquaculture. (2016) 454:243–51. doi: 10.1016/j.aquaculture.2015.12.033
18. Jannathulla R, Dayal JS, Vasanthakumar D, Ambasankar K, Panigrahi A, Muralidhar M. Apparent digestibility coefficients of fungal fermented plant proteins in two different penaeid shrimps—A comparative study. Aquac Res. (2019) 50:1491–500. doi: 10.1111/are.14024
19. Plaipetch P, Yakupitiyage A. Effect of replacing soybean meal with yeast-fermented canola meal on growth and nutrient retention of Nile tilapia, Oreochromis niloticus (Linnaeus 1758). Aquac Res. (2014) 45:1744–53. doi: 10.1111/are.12119
20. Mondal K. Nutritional evaluation of fermented poultry feather feal in the formulated diets of fingerlings of Catla catla (Hamilton). Electron J Biol. (2014) 10:118–24. Available online at: http://ejbio.imedpub.com/nutritional-evaluation-of-fermented-poultry-feather-meal-in-the-formulated-diets-of-fingerlings-of-catla-catla-hamilton.php?aid=6003
21. Glencross BD, Booth M, Allan GL. A feed is only as good as its ingredients – a review of ingredient evaluation strategies for aquaculture feeds. Aquac Nutr. (2007) 13:17–34. doi: 10.1111/j.1365-2095.2007.00450.x
22. Nazari H, Salarzadeh A, Safari O, Yahyavi M. Screening of selected feedstuffs by juvenile pacu, Piaractus brachypomus (Cuvier, 1818). Aquac Nutr. (2018) 24:1729–37. doi: 10.1111/anu.12807
23. Safari O, Shahsavani D, Paolucci M, Mehraban Sang Atash M. Screening of selected feedstuffs by sub-adult narrow clawed crayfish, Astacus leptodactylus leptodactylus Eschscholtz, (1823). Aquaculture. (2014) 420–421:211–8. doi: 10.1016/j.aquaculture.2013.11.009
25. Amin A, El Asely A, Abd El-Naby AS, Samir F, El-Ashram A, Sudhakaran R, et al. Growth performance, intestinal histomorphology and growth-related gene expression in response to dietary Ziziphus mauritiana in Nile tilapia (Oreochromis niloticus). Aquaculture. (2019) 512:734301. doi: 10.1016/j.aquaculture.2019.734301
26. Dawood MA, Shukry M, Zayed MM, Omar AA, Zaineldin AI, El Basuini MF. Digestive enzymes, immunity and oxidative status of Nile tilapia (Oreochromis niloticus) reared in intensive conditions. Sloven Vet Res. (2019) 56(Suppl. 22):99–108. doi: 10.26873/SVR-747-2019
27. National Research Council. 2011. Nutrient Requirements of Fish and Shrimp. Washington, DC: The National Academies Press. doi: 10.17226/13039.
28. Abdelhamid A, Khalil F, El-Barbary M, Zaki V, Husien H. Feeding Nile tilapia on biogen to detoxify aflatoxin diet. In: Annual Scientific Conference of Animal and Fish Production 1. Mansoura: Mansoura University (2002). p. 207–30.
29. Association of Official Analytical Chemists. Method 2007-04. Association of Official Analytical Chemists, Washington, DC (2007).
30. Lowry OH, Rosebrough NJ, Farr AL, Randall RJ. Protein measurement with the Folin phenol reagent. J Biol Chem. (1951) 193:265–75.
31. Anson ML. The estimation of pepsin, trypsin, papain, and cathepsin with hemoglobin. J Gen Physiol. (1938) 22:79. doi: 10.1085/jgp.22.1.79
32. Jiang C. Activity Measuring for Implemental Enzyme. Shanghai: Science and Technology Press (1982).
33. Worthington V. Worthington Enzyme Manual: Enzymes and Related Biochemicals Worthingthon Chemical. Freehold, NJ: Worthington Biochemical Corporation (1993) 399 p.
34. Borlongan IG. Studies on the digestive lipases of milkfish, Chanos chanos. Aquaculture. (1990) 89:315–25. doi: 10.1016/0044-8486(90)90135-A
35. Jin Z. The Evaluation Principle and Method of Functional Food. Beijing: Beijing Publishers (1995).
36. Secombes CJ. Isolation of salmonid macrophages and analysis of their killing activity. Techniq Fish Immunol. (1990) 1:137–54.
37. Parry J, Richard M, Chandan RC, Shahani KM. A rapid and sensitive assay of muramidase. Proc Soc Exp Biol Med. (1965) 119:384–6. doi: 10.3181/00379727-119-30188
38. Rainger G, Rowley A. Antibacterial activity in the serum and mucus of rainbow trout, Oncorhynchus mykiss, following immunisation with Aeromonas salmonicida. Fish Shellfish Immunol. (1993) 3:475–82. doi: 10.1006/fsim.1993.1046
39. Kawahara E, Ueda T, Nomura S. In vitro phagocytic activity of white-spotted char blood cells after injection with Aeromonas salmonicida extracellular products. Fish Pathol. (1991) 26:213–4. doi: 10.3147/jsfp.26.213
40. Yossa R, Verdegem M. Misuse of multiple comparison tests and underuse of contrast procedures in aquaculture publications. Aquaculture. (2015) 437:344–50. doi: 10.1016/j.aquaculture.2014.12.023
41. Fowler L. Poultry by-product meal as a dietary protein source in fall chinook salmon diets. Aquaculture. (1991) 99:309–21. doi: 10.1016/0044-8486(91)90251-2
42. Kureshy N, Davis DA, Arnold C. Partial replacement of fish meal with meat-and-bone meal, flash-dried poultry by-product meal, and enzyme-digested poultry by-product meal in practical diets for juvenile red drum. N Am J Aquac. (2000) 62:266–72. doi: 10.1577/1548-8454(2000)062<0266:PROFMW>2.0.CO;2
43. Steffens W. Replacing fish meal with poultry by-product meal in diets for rainbow trout, Oncorhynchus mykiss. Aquaculture. (1994) 124:27–34. doi: 10.1016/0044-8486(94)90351-4
44. Takagi S, Shimeno S, Hosokawa H, Ukawa M. Replacement of fish meal by combined inclusion of alternative protein sources in a diet for yearling red sea bream, Pagrus major. Aquac Sci. (2000) 48:545–52. doi: 10.11233/aquaculturesci1953.48.545
45. Webster CD, Tiu LG, Morgan AM, Gannam A. Effect of partial and total replacement of fish meal on growth and body composition of sunshine bass Morone chrysops× M. saxatilis fed practical diets. J World Aquac Soc. (1999) 30:443–53. doi: 10.1111/j.1749-7345.1999.tb00992.x
46. Yang Y, Xie S, Cui Y, Zhu X, Lei W, Yang Y. Partial and total replacement of fishmeal with poultry by-product meal in diets for gibel carp, Carassius auratus gibelio Bloch. Aquac Res. (2006) 37:40–8. doi: 10.1111/j.1365-2109.2005.01391.x
47. Upadhaya SD, Kim IH. Ileal digestibility of nutrients and amino acids in unfermented, fermented soybean meal and canola meal for weaning pigs. Anim Sci J. (2015) 86:408–14. doi: 10.1111/asj.12305
48. Hong K-J, Lee C-H, Kim SW. Aspergillus oryzae GB-107 fermentation improves nutritional quality of food soybeans and feed soybean meals. J Med Food. (2004) 7:430–5. doi: 10.1089/jmf.2004.7.430
49. Dawood MA, El-Dakar A, Mohsen M, Abdelraouf E, Koshio S, Ishikawa M, et al. Effects of using exogenous digestive enzymes or natural enhancer mixture on growth, feed utilization, and body composition of Rabbitfish, Siganus rivulatus. J Agric Sci Technol. (2014) 4:180–7.
50. Ortuño J, Cuesta A, Rodriguez A, Esteban MA, Meseguer J. Oral administration of yeast, Saccharomyces cerevisiae, enhances the cellular innate immune response of gilthead seabream (Sparus aurata L.). Vet Immunol Immunopathol. (2002) 85:41–50. doi: 10.1016/S0165-2427(01)00406-8
51. Peppler HJ. Yeast extracts. In: Economic Microbiology, ed AH Rose (London: Academic Press), (1982) 293–312.
52. Saurabh S, Sahoo P. Lysozyme: an important defence molecule of fish innate immune system. Aquac Res. (2008) 39:223–39. doi: 10.1111/j.1365-2109.2007.01883.x
53. Lamas J, Ellis AE. Atlantic salmon (Salmo salar) neutrophil responses to Aeromonas salmonicida. Fish Shellfish Immunol. (1994) 4:201–19. doi: 10.1006/fsim.1994.1019
54. Rodríguez I, Chamorro R, Novoa B, Figueras A. β-Glucan administration enhances disease resistance and some innate immune responses in zebrafish (Danio rerio). Fish Shellfish Immunol. (2009) 27:369–73. doi: 10.1016/j.fsi.2009.02.007
55. Magnadottir B. Immunological control of fish diseases. Mar Biotechnol. (2010) 12:361–79. doi: 10.1007/s10126-010-9279-x
56. Uribe C, Folch H, Enríquez R, Moran G. Innate and adaptive immunity in teleost fish: a review. Vet Med. (2011) 56:486–503. doi: 10.17221/3294-VETMED
57. Miyazaki T. A simple method to evaluate respiratory burst activity of blood phagocytes from Japanese flounder. Fish Pathol. (1998) 33:141–2. doi: 10.3147/jsfp.33.141
58. Apel K, Hirt H. Reactive oxygen species: metabolism, oxidative stress, and signal transduction. Annu Rev Plant Biol. (2004) 55:373–99. doi: 10.1146/annurev.arplant.55.031903.141701
59. Yao J, Wang J-Y, Liu L, Li Y-X, Xun A-Y, Zeng W-S, et al. Anti-oxidant effects of resveratrol on mice with DSS-induced ulcerative colitis. Arch Med Res. (2010) 41:288–94. doi: 10.1016/j.arcmed.2010.05.002
60. Sharma DK, Ansari BA. Effects of deltamethrin on CAT, LPO and GSH in tissues of zebra fish Danio rerio. Res J Environ Toxicol. (2013) 7:38–46. doi: 10.3923/rjet.2013.38.46
61. Dawood MAO, Moustafa EM, Gewaily MS, Abdo SE, AbdEl-kader MF, SaadAllah MS, et al. Ameliorative effects of Lactobacillus plantarum L-137 on Nile tilapia (Oreochromis niloticus) exposed to deltamethrin toxicity in rearing water. Aquatic Toxicol. (2019) 219:105377. doi: 10.1016/j.aquatox.2019.105377
Keywords: digestive enzymes, immunity, Nile tilapia, oxidative status, poultry by product meal, yeast fermentation
Citation: Dawood MAO, Magouz FI, Mansour M, Saleh AA, Asely AME, Fadl SE, Ahmed HA, Al-Ghanim KA, Mahboob S and Al-Misned F (2020) Evaluation of Yeast Fermented Poultry By-Product Meal in Nile Tilapia (Oreochromis niloticus) Feed: Effects on Growth Performance, Digestive Enzymes Activity, Innate Immunity, and Antioxidant Capacity. Front. Vet. Sci. 6:516. doi: 10.3389/fvets.2019.00516
Received: 01 November 2019; Accepted: 31 December 2019;
Published: 24 January 2020.
Edited by:
Samad Rahimnejad, University of South Bohemia in České Budějovice, CzechiaCopyright © 2020 Dawood, Magouz, Mansour, Saleh, Asely, Fadl, Ahmed, Al-Ghanim, Mahboob and Al-Misned. This is an open-access article distributed under the terms of the Creative Commons Attribution License (CC BY). The use, distribution or reproduction in other forums is permitted, provided the original author(s) and the copyright owner(s) are credited and that the original publication in this journal is cited, in accordance with accepted academic practice. No use, distribution or reproduction is permitted which does not comply with these terms.
*Correspondence: Mahmoud A. O. Dawood, mahmoud.dawood@agr.kfs.edu.eg; mahmouddawood55@gmail.com