- 1Department of Veterinary Clinical Medicine, University of Illinois, Urbana, IL, United States
- 2Department of Comparative Biosciences, University of Illinois, Urbana, IL, United States
- 3Department of Animal Sciences, University of Illinois, Urbana, IL, United States
- 4Corporación Colombiana de Investigación Agropecuaria - Agrosavia, Bogota, Colombia
The etiology of cystic ovarian follicles (COF) remains a conundrum with steroidogenic, immunological, and metabolic dysfunctions linked to its development. Studies suggest that COF development may occur as a result of disruption of the insulin signaling pathway and the severity of a negative energy balance in dairy cows, but mid to late lactation cows diagnosed with COF are unlikely to have issues with energy metabolism. Herein, we characterized the mRNA expression of steroidogenic (LHCGR, StAR, CYP11A1, 3β-HSD, CYP19A), immunological (IL-1β, IL-6, IL-8, TLR-4, TNF), and metabolic markers (IGF-1, IRS1) in follicular fluid; and plasma and follicular fluid levels of E2, IL-1β, glucose, and NEFA in early and mid-late lactation COF cows. Lactating dairy cows were diagnosed as having COF (n = 11, follicle >20 mm persistent for 7 days, absence of corpus luteum, and flaccid uterus) while 11 herdmates cycling with a dominant follicle were classified as the control cows. Cows diagnosed with COF were classified as early lactation (COF-E, n = 5) cows, <35 days in milk (DIM); or mid-late lactation (COF-M/L, n = 6), ≥118 DIM cows. Results revealed that mRNA expression StAR was greater (P < 0.01) in COF-E cows than COF-M/L cows and the control cows. The mRNA expression CYP19A1 was lower (P < 0.01) in COF-E cows and COF-M/L cows than in the control cows. The mRNA expression IL-6 and IRS-1 tended to be greater and lower, respectively, in COF-M/L cows compared to the control cows. The mRNA expression IGF-1 was greater (P < 0.01) in COF-E and COF-M/L cows than in the control cows. The plasma and follicular fluid concentration of NEFA was greater (P < 0.05) in COF-E cows than in COF-M/L and the control cows. Cows with COF-E had disturbances in steroidogenic and metabolic markers, while cows with COF-M/L had steroidogenic, immunological, and metabolic dysregulations, suggesting that COF pathogenesis may vary between early and mid-late lactation dairy cows.
Introduction
The etiology of cystic ovarian follicles (COF) remains a puzzle, but hormonal, immunological, and metabolic imbalances have been linked to its development (1–5). Cows diagnosed with COF have a dysfunctional hypothalamic-pituitary-ovarian axis signature that includes parabasal concentration of progesterone, increased peripheral estradiol levels, increased LH pulse frequency and amplitude, and reduced LH and FSH receptors that translate into a lack of LH surge and ovulation (6–10).
The steroidogenic markers associated with this dysfunctional signature is not well elucidated. Previous studies indicated a higher expression of 3β-HSD in estrogen-active cyst, but not young growing cysts (11). A more recent study found a higher or lower protein expression steroid acute regulatory protein (StAR), cytochrome P450 aromatase (CYP19A1), and 3β-hydroxysteroid dehydrogenase (3β-HSD) in the theca and granulosa cells of COF depending on the follicular stage of development (5). For example, the protein expression of CYP19A1 was lower in the preantral follicles of COF cows than in control cows, but CYP19A1 was higher in the antral follicles of COF cows than in the control cows suggesting that the criteria used for comparisons can impact the results and its interpretation for the development of COF (5). The number of days postpartum when COF are diagnosed, cyst turnover, and response to the therapy are revised and vary considerably (12). Thus, the characterization of steroidogenic markers between early and mid-late lactation cows developing COF might help to improve the understanding the differences in COF pathogenesis.
Another key dysregulation reported in cows with COF is the expression of immunological markers such as cytokines – key regulators of cell proliferation, follicular development, atresia, ovulation, steroidogenesis, and angiogenesis (13–15). Few investigations were performed with dairy cows, using either a model with induced follicle persistence or naturally occurring COF (3, 16, 17). The first study using a model of persistent follicles (5, 10 and 15 days of follicular persistence with day 0 as expected ovulation) revealed that interleukin 8 (IL-8) and tumor necrosis factor-α (TNF) was higher early (0 and/or 5 days of follicular persistence), and IL-6 was higher from 0 to 10 days of persistence, in relation to dominant follicles from the control group (3). A second study of persistent follicles found that persistent follicles at different stages of persistence had a higher expression of IL-1 receptor antagonist (IL-1RA), IL-1 receptor II (IL-1RII), and IL-4 than the control group (17). The single study characterizing pro-inflammatory cytokines in cows with spontaneous COF, revealed that there was no differences in mRNA expression of IL-1β, IL-6, and TNF measured by real-time polymerase chain reaction between cystic follicles and dominant follicles, but the expression of these pro-inflammatory cytokines was higher in cystic follicles when measured by immunohistochemistry (16). Altogether, these studies suggest that more research is needed to elucidate the role of pro-inflammatory cytokines in the etiology of COF.
Follicular steroidogenesis and follicular cell proliferation are modulated by metabolic hormones such as insulin and insulin growth factor-1 (IGF-1) (18–20). A wealth of information has linked COF development with the disruption of the insulin signaling pathway, other critical metabolic sensors, and the severity of a negative energy balance in dairy cows (1, 4, 5, 21–23). It has been shown that cows developing COF have reduced insulin and non-esterified fatty acids (NEFA) and increased IGF-1 in the plasma during the weeks preceding cyst development (21–23). The follicular fluid concentration of IGF-1 have been reported to be reduced in induced and spontaneous COF (24) and the mRNA expression was decreased in induced but not in spontaneous COF (25). The expression of the insulin receptor and subunit alpha p85 of PI3K were reported to be lower in COF cows than in the tertiary follicles of the control cows (1). Another recent study detected that cystic cows' metabolic changes included lower plasma insulin, lower follicular fluid glucose and triacylglycerol, and higher follicular fluid NEFA, beta-hydroxybutyrate, and cholesterol (4). Early postpartum dairy cows undergo a negative energy balance and have higher odds of having metabolism disturbances. Despite all of the evidence of metabolic disorder in cows with COF, few studies have attempted to evaluate if these disturbances are concurrent and relate to steroidogenesis and immunological markers, and why cows in mid-late lactation develop COF despite not having the same metabolic challenges.
Cystic ovarian follicles remain a significant cause of reproductive failure in lactating dairy cows. This leads to economic losses due to an increase in the time required from calving to conception and culling rates, while the response to therapy is also highly inconsistent (12). Further elucidation of COF concurrent disturbances on steroidogenesis, pro-inflammatory cytokines, and energy metabolites in early and mid-late lactation dairy cows might allow the development of surrogate measures to mitigate the negative impact of COF and its unresponsiveness to therapy. There is, therefore, a critical need to characterize concurrent steroidogenesis, immunological, and metabolic markers of cows with spontaneous COF diagnosed at early and mid-lactation. We hypothesize that cows diagnosed with COF at early lactation have different steroidogenesis, pro-inflammatory, and metabolic disturbances than cows diagnosed with COF at mid-late lactation. The aims of the current study were to characterize the mRNA expression of steroidogenic, immunological, and metabolic markers in the follicular fluid of cows diagnosed with COF at early and mid-late lactation. We also assessed plasma and follicular concentrations of E2, IL-1β, glucose, and NEFA.
Materials and Methods
Ethics
All experimental procedures carried out in this study were approved by the Institutional Animal Care and Use Committees at the University of Illinois—Urbana-Champaign (Protocol # 14-283).
Experimental Design and Reproductive Management
Lactating dairy cows from the University of Illinois' dairy herd were examined weekly by ultrasound, and a cohort of 11 cows classified as having COF (follicle >20 mm persistent for 7 days, absence of corpus luteum, and flaccid uterus) (26) were enrolled in the study. Cows diagnosed with COF were classified as early lactation cows (COF-E, n = 5)—diagnosed <35 days in milk (DIM) cows, or mid-late lactation cows (COF-M/L, n = 6)—detected ≥118 DIM. A group of 11 cows previously synchronized, that were confirmed to be cycling with a dominant follicle (presence of corpus luteum >16 mm and follicle >9 mm) were enrolled as the controls at 60 DIM. The reproductive management for the University of Illinois' dairy farm includes weekly examination by a University of Illinois Veterinarian starting at 21 DIM, using transrectal ultrasonography (Easy Scan, BCF Technology, Rochester, MN, USA) with a 7.5 MHz linear array probe.
Cows in the control group were synchronized with a modified Presynch-OvSynch as previously described (27). Briefly, cows received two injections of prostaglandin F2 alpha—PGF—(Lutalyse, 25 mg dinoprost tromethamine, Zoetis Animal Health, Kalamazoo, MI USA) at 34 ± 3 and 48 ± 3 DIM, then 12 days later at 60 ± 3 DIM began the OvSynch program. The program consists of a first GnRH at 60 ± 3 DIM, followed by PGF treatment 7 days later at 67 ± 3 DIM, a second GnRH 56 h after PGF at 69 ± 3 DIM, and then artificial insemination 16-−20 h later at 70 ± 3 DIM. Cows received an ultrasonography examination at the 60 ± 3 DIM, and only those with a corpus luteum larger than 16 mm and a follicle larger than 9 mm were eligible to be enrolled as the control for cows diagnosed as having COF. The goal was to assure that cows in the control group had an active corpus luteum and a dominant follicle.
Cows Descriptive Characteristics
The average (range) follicle diameters retrieved at follicular aspiration were 42.2 mm (31.0–60.0 mm), 30.3 mm (25.0–40.0 mm), and 14.1 mm (9.0–19.0 mm), respectively, for the COF/E, COF-M/L and control cows. The average (range) days in milk were 32 days (30–34 days), 210 days (118–382 days), and 60 days (57–63 days), respectively, for the COF/E, COF-M/L, and control cows. The daily milk production averages (ranges) in the month of diagnosis were 41.6 kg (25.6–54.8 kg), 32.1 kg (7.6–52.6 kg), and 39.3 kg (22.1–50.7 kg), respectively, for the COF/E, COF-M/L, and control cows. The average number of lactations were 2.8 (1–6), 2.3 (1–4), and 2.7 (2–6), respectively, for the COF/E, COF-M/L, and control cows.
Ovum Pick-Up for Follicular Fluid Collection
The follicle of COF cows and the dominant follicles of the control cows were aspirated to collect follicular fluid and cells. The control group had the dominant follicle aspirated at 60 ± 3 DIM. Briefly, the area above the first intercoccygeal space was clipped and disinfected using gauze embedded with an iodine solution and 70% ethanol. Then the first intercoccygeal space was injected with 5 ml of lidocaine (2% lidocaine hydrochloride solution, Hospira, Inc., Lake Forest, IL, USA) and 5 min were allowed to let the anesthesia to take effect. The vulva and perineal area were cleaned and disinfected with gauze embedded in an iodine solution. The ultrasound scanner equipped with a 7.5 MHz curvilinear probe (IBEX, E.I. Medical Imaging, Loveland, Colorado) was used for the follicle aspiration procedure. The ultrasound probe was placed within a custom-made handle. The handle enclosed the probe cord and fixed the head of the probe at a 30° angle relative to the needle guide. The device received a sterile lubricant and was introduced in the vagina slightly posterior to the cervix, toward the ovary containing the follicle that was re-measured and aspirated. The aspiration needle (18 G, WTA, Cravinhos, SP, Brazil) was guided through the stroma of the ovary and into the dominant follicle or cyst. The follicular fluid was collected and frozen at −80°C for a subsequent analysis of hormones. The follicular cells were immediately pelleted by centrifugation, at 4°C for 10 min at 2,000 × g and the follicular fluid was transferred. The cells were homogenized with Qiazol reagent (Qiagen, Hilden, Germany), snap-frozen in liquid nitrogen, and stored at −80°C.
Markers Selection, RNA Extraction, and Quantitative PCR
The rationale for the markers investigated in the current study was to concurrently analyze genes that are the hallmark of an active innate immune response (IL-1β, IL-6, IL-8, TLR-4, TNF), genes that represent key steps of steroidogenic cascade (LHCGR, StAR, CYP11A1, 3β-HSD, CYP19A), and essential metabolic markers (IGF-1, IRS1) in two types of COF cows (early and mid-late lactation) that have a different metabolic profile. These genes have been reported to be altered in COF cows as described in the introduction, but an integrative empirical approach could shed light if discrepancies between key disrupted biological pathways of COF pathogenesis in early postpartum and mid to late lactation exist.
The RNA of follicular cells was extracted using the miRNeasy kit (Qiagen, Hilden, Germany), according to manufacturer's protocols, as detailed in our previous study (28). Quantitative PCR was performed using 4 ml diluted cDNA combined with 6 ml of a mixture composed of 5 ml of SYBR Green master mix (Quanta Biosciences, Gaithersburg, MD, USA), 0.4 ml each of 10 mm forward and reverse primers, and 0.2 ml DNase/RNase free water in a MicroAmpTM Optical 384–Well Reaction Plate (Applied Biosystems, CA) as detailed in our previous study (28).
Primers were designed using Primer Express 2.0 with a minimum amplicon size of 80 bp (when possible amplicons of 100–120 bp were chosen) and limited 30 G þ C (Applied Biosystems) (Table 1). When possible, primer sets were designed to fall across exon-exon junctions. Primers were aligned against publicly available databases using BLASTN at NCBI (29) and the UCSC's Cow (Bos taurus) Genome Browser Gateway. Before qPCR, primers were tested in a 20 μl PCR reaction using the same protocol described for qPCR excepting for the final dissociation protocol. For primer testing we used a universal reference cDNA (RNA mixture from different bovine samples) to ensure identification of desired genes. Five μl of the PCR product was run in a 2% agarose gel stained with SYBR safe. Only those primers that did not present primer-dimers and a single band at the expected size in the gel and those that had the right amplification product (verified by sequencing) were used for qPCR. The accuracy of a primer pair also was evaluated by the presence of a unique peak during the dissociation step at the end of qPCR. The extraction and qPCR analysis was performed using previously established protocols at Dr. Loor's Lab at the University of Illinois (30, 31). The final data were normalized using the geometric mean of GAPHD, β-ACT, and H2AFZ, which were validated as suitable internal control genes in bovine liver and adipose tissue.
Blood and Follicular Fluid Metabolite Analyses
Blood samples were collected at the day of follicle aspiration using evacuated tubes (Becton Dickinson, Franklin Lakes, NJ) containing K2 EDTA for plasma separation. Samples were placed immediately on ice and kept refrigerated until arrival to the laboratory. Blood tubes were centrifuged at 2,000 × g for 15 min at 5°C, and an aliquot of 2 ml of plasma was frozen at −80°C until analysis. Concentrations of E2 were measured using radioimmunoassay according to previous procedures which were slightly modified (32–34). The modifications were the substitution of a second antibody precipitation procedure in place of the charcoal extraction procedure. We used 100 μl 3-iodo-estradiol-17β (4.0 pg/tube, Carson, CA, USA). For plasma estradiol a volume of 200 μl of plasma diluted with 3 ml of benzene was used: toluene (2:1 vol/vol) solvent, whereas for follicular fluid the assay was diluted 1:1000. The estradiol assay was sensitive to 0.5 pg/ml and had an intra-assay CV of 9% and an inter-assay CV of 11%. Plasma and follicular fluid concentration pro-inflammatory cytokine IL-1β was measured using a bovine ELISA (Cat. No. ESS0027; Thermo Scientific, Rockford, IL). Concentrations of NEFA and glucose on plasma and follicular samples were analyzed using commercial kits at the Veterinary Diagnostics Laboratory, College of Veterinary Medicine, University of Illinois.
Statistical Analyses
A power analysis was performed to identify the required number of cows for the study using G Power*Power version 3.1 for Mac (Universität Düsseldorf, Germany). We based the power calculation on a previous study that reported a ~6-fold change decrease in CYP19A1 mRNA expression in COF cows when compared with cows with large antral follicles (5). Accordingly, we anticipate a 6-fold reduction of the CYP19A1 mRNA expression in COF cows when compared to cyclic herdmates having large pre-antral follicles. Thus, using a one tailed-test, a 5% type I error, and a power of 80%, a sample size of five cows per group was deemed necessary. Models included the main effect of the group (COF-E, COF-M/L, and control group) and the variables of interest mRNA expression of steroidogenic (LHCGR, StAR, CYP11A1, 3β-HSD, CYP19A), immunological (IL-1β, IL-6, IL-8, TLR-4, TNF), and metabolic markers (IGF-1, IRS1) in follicular fluid; and plasma and follicular fluid levels of E2, IL-1β, glucose, and NEFA. All models were analyzed using the GLIMMIX procedure of SAS version 9.4 (SAS/STAT; SAS Institute Inc., Cary, NC). Data were analyzed using models that fit a Gaussian or a logarithmic distribution, and residuals were assessed for normality. The covariance structure that resulted in the smallest Bayesian information criterion was selected. Relative expression values were obtained by raising the PCR amplification values to the power of delta-delta threshold cycle (ΔΔCT) obtained from ΔCT least-square mean differences of pairwise comparisons among treatments and controls as the reference group (35). The ΔCT values, subjected to statistical analysis, were generated by normalization of CT values from target genes with the geometric mean of CT values from reference genes as previously described (36). Correlation analyses were performed for the plasmatic and follicular fluid concentration of E2, glucose, NEFA, and IL-1 β. Confidence intervals for graphical representation of relative expression were generated from the lower and upper confidence limits obtained for ΔCT least-square mean differences as described by Yuan et al. (35). Differences with P < 0.05 were considered significant and 0.05 < P ≤ 0.10 were considered to be a tendency toward a statistical difference.
Results
LHCGR, StAR, CYP11A1, 3β-HSD, CYP19A1 mRNA Expression
Cows diagnosed with COF-E and COF-M/L had a similar (P = 0.59) mRNA expression of luteinizing/choriogonadotropin hormone receptor (LHCGR) than that of the control herdmates (Figure 1A). Cow diagnosed with COF-E had a (P < 0.01) greater mRNA expression of StAR than that of COF-M/L and the control cows (Figure 1B). The mRNA expression of CYP11A1 was similar (P = 0.35) in COF-E, COF-M/L, and the control herdmates (Figure 1C). Likewise, no differences (P = 0.69) in mRNA expression of 3β-HSD in the COF cows and the control herdmates were detected (Figure 1D). The mRNA expression of CYP19A1 was lower (P < 0.001) in the COF-E and COF-M/L cows than in the control herdmates (Figure 1E).
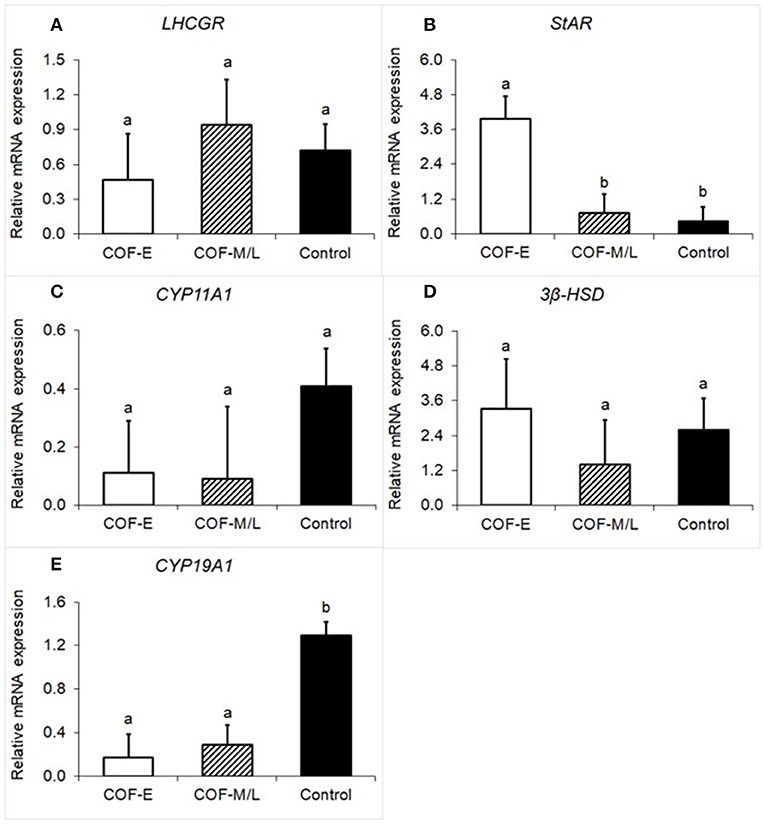
Figure 1. Fold-change in mRNA expression for five steroidogenic enzyme genes (A) LHCGR, (B) StAR, (C), CYP11A1, (D) 3β-HSD, and (E) CYP19A1 detected in follicular fluid in cows diagnosed with COF at early (COF-E) and mid-late (COF-M/L) lactation and the control cows. Different letters (a,b) represent different (0.05 < P ≤ 0.10) least-square means. Error bars represent the mean standard error.
IL-1β, IL-6, IL-8, TLR-4, TNF mRNA Expression
There were no differences (P = 0.36) for IL-1β mRNA expression between the COF-E, COF-M/L, and control cows (Figure 2A). Cows diagnosed with COF-M/L tend to have a higher mRNA expression (P < 0.09) of IL-6 than the control cows, but no differences (P = 0.75) between COF-E cows and the control cows were detected (Figure 2B). There were no differences (P = 0.36) for IL-8 mRNA expression between the COF-E, COF-M/L and control cows (Figure 2C). Likewise, no differences (P = 0.33) for TLR-4 mRNA expression were detected between the COF-E, COF-M/L, and control cows (Figure 2D). The mRNA expression of TNF was greater (P = 0.03) in COF-M/L cows than in the control cows, whereas in the COF-E cows and control cows, TNF mRNA expression did not differ (P > 0.05) as depicted in Figure 2E.
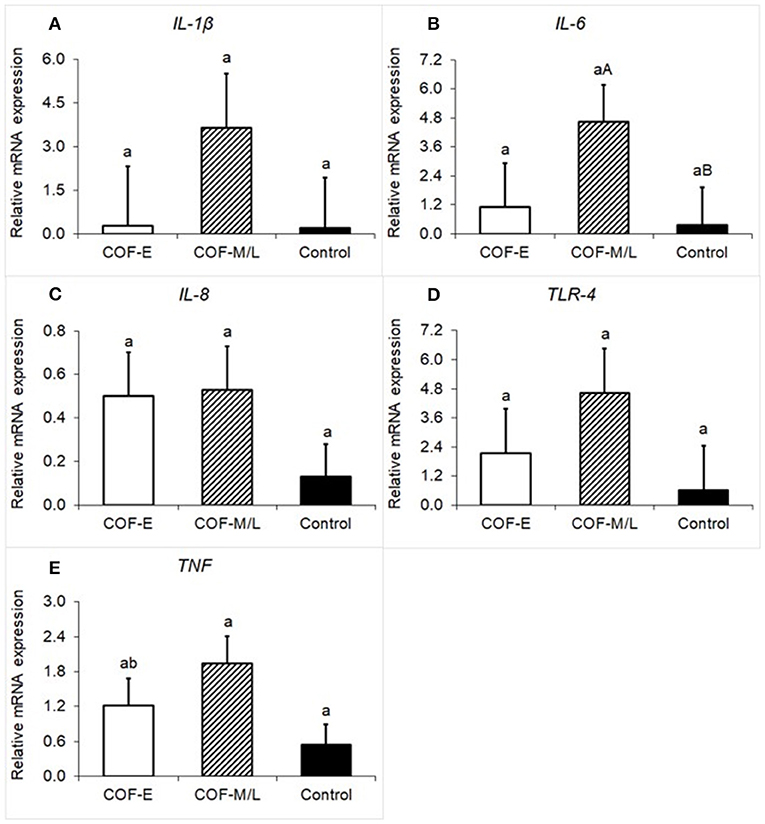
Figure 2. Fold-change in mRNA expression for five pro-inflammatory genes (A) IL-1β, (B) IL-6, (C) IL-8 (D), TLR-4, and (E) TNF detected in the follicular fluid in cows diagnosed with COF at early (COF-E) and mid-late (COF-M/L) lactation and the control cows. Different letters (a,b) represent different (P < 0.05) least-square means and capital letter (A,B) represent tendencies (0.05 < P ≤ 0.10). Error bars represent the mean standard error.
IGF-1 and IRS1 mRNA Expression
Cows diagnosed with COF-E and COF-M/L had a greater (P < 0.02) mRNA expression of IGF-1 than the control herdmates (Figure 3A). Moreover, cows diagnosed with COF-M/L tended to have a (P = 0.07) lower mRNA expression of IRS1 than the control herdmates (Figure 3B), but COF-E cows and the control cows had similar (P = 0.17) mRNA expression (Figure 3B).
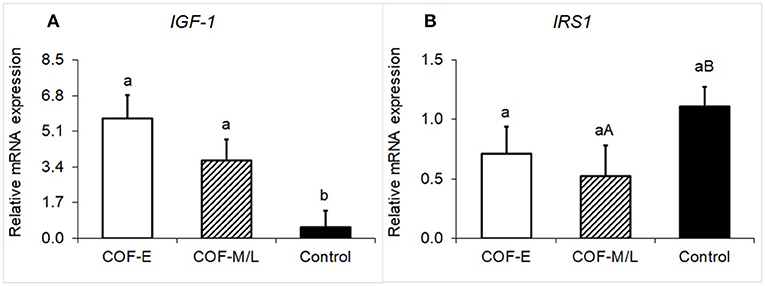
Figure 3. Fold-change in the mRNA expression for two energy metabolite genes (A) IGF-1 and (B) IRS1, detected in the follicular fluid in cows diagnosed with COF at early (COF-E) and mid-late (COF-M/L) lactation and the control cows. Different letters (a,b) represent different (P < 0.05) least-square means and capital letter (A,B) represent tendencies (0.05 < P ≤ 0.10). Error bars represent the mean standard error.
E2, Glucose, NEFA, and IL-1β Concentrations in Plasma
Cows diagnosed with COF-E and COF-M/L had similar levels of E2 in the plasma (P = 0.92) than the control cows (Figure 4A). Likewise, the plasma concentration of glucose in cows diagnosed with COF in early and mid-late lactation did not differ (P = 0.97) from the control herdmates (Figure 4B). On the other hand, cows diagnosed with COF-E had a greater plasma concentration (P = 0.02) of NEFA than the COF-M/L and control cows (Figure 4C). The plasma concentrations of IL-1β did not differ (P > 0.05) between the COF-E, COF-M/L, and control cows (Figure 4D).
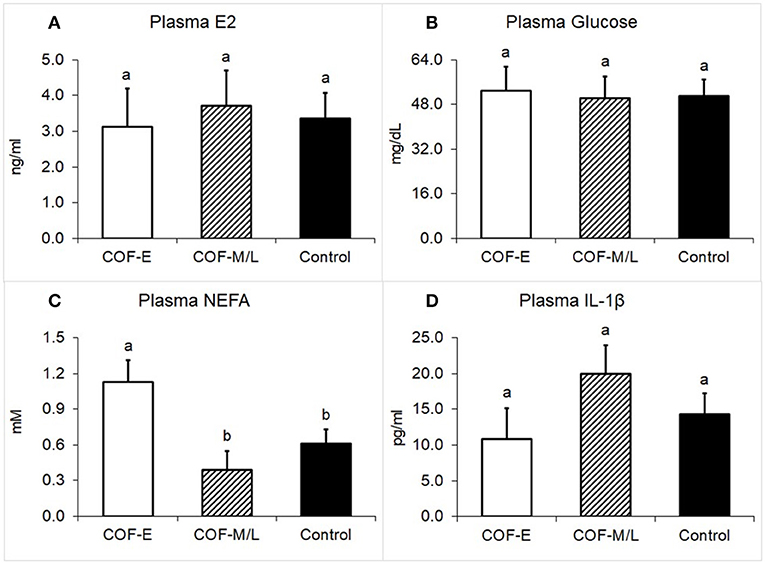
Figure 4. Plasma concentration for (A) E2, (B) Glucose, (C) NEFA, and (D) IL-1β in cows diagnosed with COF at early (COF-E) and mid-late (COF-M/L) lactation and the control cows. Different letters (a,b) represent different (P < 0.05) least-square means. Error bars represent the mean standard error.
E2, Glucose, NEFA, and IL-1β Concentrations in Follicular Fluid
Cows diagnosed with COF-E and COF-M/L had similar levels of E2 in the follicular fluid (P = 0.38) than the control cows (Figure 5A). The concentration of glucose in follicular fluid of cows diagnosed with COF-E and COF-M/L was similar (P = 0.40) to that of the control cows (Figure 5B). Cows diagnosed with COF-E had a higher concentration in the follicular fluid of NEFA (P < 0.001) than the control cows and a lower follicular fluid concentration of NEFA than the COF-M/L cows (Figure 5C). Also, the NEFA concentration in the follicular fluid of the COF-M/L cows was greater (P = 0.02) than in the control herdmates (Figure 5C) The follicular fluid concentration of IL-1β tended to be greater for the COF-M/L cows (P = 0.05) than for the COF-E cows, and the follicular fluid concentration of IL-1β was greater (P = 0.02) in the COF-M/L cows than in the control cows (Figure 5D). However, no differences (P = 0.98) in the follicular fluid concentration of IL-1β between the COF-E and control cows were detected (Figure 5D).
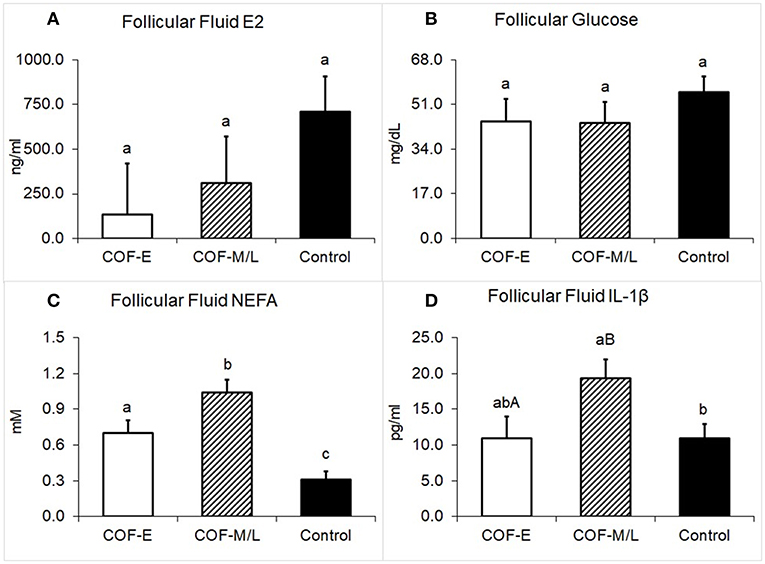
Figure 5. Follicular fluid concentration for (A) E2, (B) Glucose, (C) NEFA, and (D) IL-1β in cows diagnosed with COF at early (COF-E) and mid-late (COF-M/L) lactation and the control cows. Different letters (a,b) represent different (P < 0.05) least-square means and capital letter (A,B) represent tendencies (0.05 < P ≤ 0.10). Error bars represent the mean standard error.
Correlation of Plasma and Follicular Fluid Concentration of E2, Glucose, NEFA, and IL-1β
An analysis of associations for plasma and follicular fluid for metabolites E2, glucose, NEFA, and IL-1β in the COF-E, COF-M/L, and control cows revealed no significant relationship between follicular and plasma concentrations of these metabolites (Table 2).
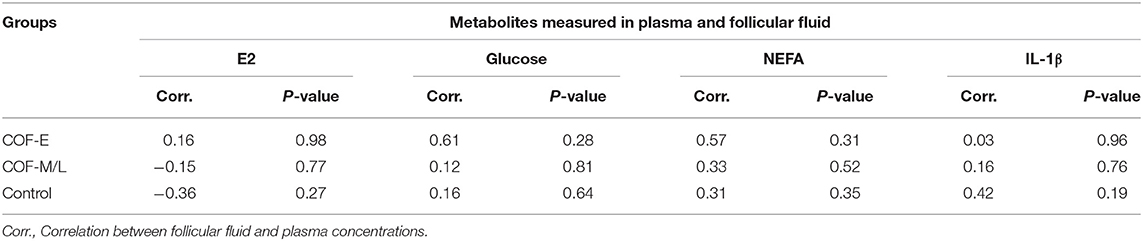
Table 2. Correlation analysis of the plasma and follicular fluid concentration of E2, glucose, NEFA, and IL-1β in cows diagnosed with COF at early (COF-E) and mid-late (COF-M/L) lactation and the control cows.
Discussion
As hypothesized, concurrent dysregulation of steroidogenic, immunological, and metabolic markers occurred in cows diagnosed with spontaneous COF and the time in lactation for diagnosis influenced the gene expression, plasma, and follicular fluid concentration disturbances found in the current study. Amongst the steroidogenic markers, differences between COF cows and the control cows were found only for StAR and CYP19A1. Interestingly, the mRNA expression of StAR was increased in COF cows at early but not mid-late lactation when compared to the control cows. Similarly, a recent study examined the mRNA expression of StAR in theca and granulosa and theca cells in small (<5 mm), medium (5–10 mm) and large antral follicles (>10 mm) with COF. It was reported that the StAR protein expression was higher in the granulosa cells of small antral follicles of cows with COF than in those of control cows (5). Another study that classified COF in cysts and young cysts (follicular structures >15 mm within 3–5 day of removal of previous cyst) reported an increase in StAR mRNA expression in the granulosa cells of cows with cysts, but not with young cysts, suggesting that the stages of development of the cyst might influence the characterization of steroidogenic disturbances reported in COF (37). Altogether, these previous findings along with ours, suggest that StAR, a limiting step in the biosynthesis of steroid hormones that facilitates the translocation of cholesterol from the outer to the inner mitochondrial membrane (38), is either not compromised or overexpressed in cows diagnosed with COF. The discrepancies in the pathogenesis of COF might be related to the time postpartum in which the disease occurred.
Our findings for CYP19A1 concurred with previous studies that evaluated the mRNA expression in the granulosa of large follicles in comparison to follicular cysts, confirming that cows diagnosed with COF, independent of the number of days postpartum for diagnosis, have a lower mRNA expression of CYP19A1 (37, 39). It has been suggested that reduced mRNA expression of CYP19A1 combined with the increased expression of StAR, a sign of potentially increased cholesterol availability, might indicate that cows with COF are undergoing luteinization (40–45). It is reasonable to speculate that the profile of steroidogenic disturbances in COF early postpartum, but not in mid-late lactation, is suggestive of increased luteinization and parabasal levels of progesterone found in some cases of cystic cows. However, progesterone was not measured in the current study and, therefore, we need to be careful to interpret these results. Indeed, the lack of progesterone analysis limited the ability of the current study to distinguish if cysts were estradiol-dominant, progesterone-dominant, or low-steroidogenic active cysts as previously reported (46).
Immunological marker disturbances were only present in mid-late lactation cows diagnosed with COF. Indeed, cows diagnosed with COF-M/L had increased mRNA levels of TNF and follicular fluid of IL-1β, and tendencies for increased mRNA expression of IL-6 when compared to the control cows. Previous studies had shown that cytokines are master modulators of the follicle milieu and fate, controlling cell proliferation, follicular development, atresia, ovulation, steroidogenesis, and angiogenesis (13–15). Our findings are consistent with previous studies using persistent follicle models that reveal increased mRNA expression of IL-1RA, IL-1RII, IL-4, IL-6, IL-8, and TNF depending on the length of persistence (3, 17). A single study characterized pro-inflammatory cytokines in cows with spontaneous COF, revealing no differences in mRNA expression of IL-1β, IL-6, and TNF (16). In this study the number of days postpartum reported for the diagnosis of COF was 55 ± 9.3, and the results were consistent with the current findings for COF early postpartum (16). The similarities between the abovementioned study and our COF-E cows, and the discrepancy between COF-E and COF-M/L cows in the current study, may indicate that the number of days postpartum might be a factor to consider to fully characterize COF pathogenesis.
It is unclear which factors might trigger the increased pro-inflammatory makers of cows diagnosed with COF-M/L, but not in COF-E cows when compared to the control cows. A recent study revealed that cows diagnosed with COF had an increased presence of lipopolysaccharide (LPS) and an increased expression of TLR-4, with the latter being consistent with COF-M/L cows in the current study (39). Although we did not measure LPS in the present study, we speculate that COF-M/L cows might have had increased levels of LPS in the follicle milieu, causing the disturbing profile of inflammatory markers reported herein. A potential process for an increased accumulation of LPS in these mid-late lactation COF cows might include a leaky gut or bacterial infection at the mammary gland (47–49). Further research needs to investigate if factors such as mastitis and gastrointestinal integrity issues are associated with endotoxemia and the development of COF in mid-late lactation dairy cows.
Our findings for IGF-1 in COF-E and COF-M/L cows differed from a previous study that reported no differences in IGF-1 mRNA expression in cows with spontaneous COF and reduced mRNA expression of IGF-1 when compared to induced COF (50). Ortega et al. (24) measured the follicular fluid concentration of IGF-1 and reported a decrease in IGF-1. On the other hand, increased plasma levels of IGF-1 have been reported to be consistently higher during the first 16 weeks postpartum (22). The relationship between plasma and follicular fluid concentration of IGF-1 is controversial. A study reported that the plasma and follicular fluid of IGF-1 are highly correlated (51), whereas other studies either indicated that IGF-I in follicular fluid was lower (52), or greater (24) than plasma IGF-I concentration. Unfortunately, we did not measure IGF-1 in the plasma to help elucidate the specific relationship in the current study. Altogether, our findings as well as those of previous studies, indicate that there is a dysregulation on IGF-1. However, the exact behavior of this metabolic sensor in cystic cows needs to be further investigated.
The decreased tendency for mRNA expression of IRS-1 in COF-M/L cows is another finding in the current study that resembles a previous study (1). The last study indicated this insulin receptor (IRS-1) was reduced in cows with spontaneous COF when compared to other follicular structures (1). Insulin is a pivotal player involved with steroidogenesis, follicular development, and ovulation (53, 54). Indeed, insulin exerts its metabolic effect through the phosphatidylinositol 3-kinase (PI3K) pathway (55, 56). The regulatory PI3K subunit binds to phosphotyrosine residues on IRS-1 that operate like a docking system for molecules binding to and activating cellular kinases involved in downstream signaling pathways involved in follicular development (53, 55, 56). The downregulation of IRS-1 found in the earlier study (1) and the tendency for the decreased expression of current research might suggest that this receptor could be a factor contributing to a disturbance that leads to COF.
As expected, early postpartum but not mid-late lactation COF cows had increased plasmatic levels of NEFA when compared to control cows. Intriguingly, the follicular fluid concentrations of NEFA were increased in the COF-E and COF-M/L cows when compared to control cows. Early postpartum dairy cows are likely to be under a negative energy balance and have a metabolic profile with a disrupted insulin pathway (Low insulin and IGF-1) and increased lipolysis that result in increased plasmatic levels of NEFA (57, 58). Our findings, however, are consistent with a recent study that revealed increased follicular fluid levels of NEFA in cows diagnosed with COF, but no differences in the plasma concentration of NEFA (4). The abovementioned cows with COF were in lactation from between 173 and 255 days, which is consistent with the range for COF-M/L cows (118–382 DIM) in the current study and help to explain the present findings.
There has been a wealth of information in the literature showing the detrimental impact of NEFA for granulosa cell survival and proliferation, steroidogenesis, and follicular and oocyte development (59–62). It is, therefore, reasonable to speculate that the increased follicular concentration of NEFA found in COF cows in the current study may compromise the granulosa cell function and steroidogenesis. Our COF-E cows' levels of NEFA in the follicular fluid and plasma were similar to those reported previously (4). On the other hand, COF-M/L cows had greater levels of NEFA in the follicular fluid than in the plasma. It has been suggested that because VLDL-triacylglycerol is not capable of passing the follicle wall (63, 64), an increased follicular fluid concentration of NEFA might be a result of triacylglycerol hydrolysis, and its availability would favor its oxidation to obtain energy as shown in COF-M/L cows. In the current study, we found that COF-M/L cows had an increased expression of immunological markers, which might suggest an increased energy requirement in follicle milieu (65). The pattern of activated pro-inflammatory cytokines might be the driving factor for metabolic changes found in COF-M/L cows in the current study.
Conclusions
The results of the present study confirm that concurrent disruptions in steroidogenic, immunological, and metabolic markers occur in cows diagnosed with COF and the pattern of dysregulation varies according to the number of days postpartum. Disturbances in cows diagnosed with COF at early postpartum were limited to steroidogenesis and metabolic markers, while COF-M/L cows had steroidogenic, immunological and metabolic marker disorders. Altogether, the current study provides evidence that the characterization of mechanisms associated with COF development in early and mid-late lactation needs to be further investigated to develop therapies that can mitigate the negative impact of COF in dairy cows with a distinct pathogenesis.
Data Availability Statement
The datasets generated for this study are available on request to the corresponding author.
Ethics Statement
The animal study was reviewed and approved by Institutional Animal Care and Use Committees at the University of Illinois—Urbana-Champaign.
Author Contributions
FL analyzed the data and wrote the manuscript. FL, DA, and FC designed the study. FL and FC revised the manuscript. FL, DA, TE, CS, SS, DF, and FC participated in the acquisition of the data. DA, TE, CS, and SS performed the laboratory analysis.
Funding
Support for this project was received from the Department of Animal Sciences at the University of Illinois, as part of FC's start-up package.
Conflict of Interest
The authors declare that the research was conducted in the absence of any commercial or financial relationships that could be construed as a potential conflict of interest.
Acknowledgments
The authors gratefully acknowledge the veterinarians, and the staff at the Dairy Research Unit involved in the collection of the data.
References
1. Hein GJ, Panzani CG, Rodriguez FM, Salvetti NR, Diaz PU, Gareis NC, et al. Impaired insulin signaling pathway in ovarian follicles of cows with cystic ovarian disease. Anim Reprod Sci. (2015) 156:64–74. doi: 10.1016/j.anireprosci.2015.02.010
2. Rodriguez FM, Colombero M, Amweg AN, Huber E, Gareis NC, Salvetti NR, et al. Involvement of PAPP-A and IGFR1 in cystic ovarian disease in cattle. Reprod Domest Anim. (2015) 50:659–68. doi: 10.1111/rda.12547
3. Stassi AF, Baravalle ME, Belotti EM, Rey F, Gareis NC, Diaz PU, et al. Altered expression of cytokines IL-1alpha, IL-6, IL-8 and TNF-alpha in bovine follicular persistence. Theriogenology. (2017) 97:104–12. doi: 10.1016/j.theriogenology.2017.04.033
4. Gareis NC, Angeli E, Huber E, Salvetti NR, Rodriguez FM, Ortega HH, et al. Alterations in key metabolic sensors involved in bovine cystic ovarian disease. Theriogenology. (2018) 120:138–46. doi: 10.1016/j.theriogenology.2018.07.045
5. Gareis NC, Huber E, Hein GJ, Rodriguez FM, Salvetti NR, Angeli E, et al. Impaired insulin signaling pathways affect ovarian steroidogenesis in cows with COD. Anim Reprod Sci. (2018) 192:298–312. doi: 10.1016/j.anireprosci.2018.03.031
6. Kawate N, Inaba T, Mori J. A quantitative comparison in the bovine of steroids and gonadotropin receptors in normally developing follicles and in follicular and luteinized cysts. Anim Reprod Sci. (1990) 23:273–81. doi: 10.1016/0378-4320(90)90041-D
7. Cook DL, Parfet JR, Smith CA, Moss GE, Youngquist RS, Garverick HA. Secretory patterns of LH and FSH during development and hypothalamic and hypophysial characteristics following development of steroid-induced ovarian follicular cysts in dairy cattle. J Reprod Fertil. (1991) 91:19–28. doi: 10.1530/jrf.0.0910019
8. Stock AE, Fortune JE. Ovarian follicular dominance in cattle: relationship between prolonged growth of the ovulatory follicle and endocrine parameters. Endocrinology. (1993) 132:1108–14. doi: 10.1210/endo.132.3.8440173
9. Hamilton SA, Garverick HA, Keisler DH, Xu ZZ, Loos K, Youngquist RS, et al. Characterization of ovarian follicular cysts and associated endocrine profiles in dairy cows. Biol Reprod. (1995) 53:890–8. doi: 10.1095/biolreprod53.4.890
10. Hatler TB, Hayes SH, Laranja da Fonseca LF, Silvia WJ. Relationship between endogenous progesterone and follicular dynamics in lactating dairy cows with ovarian follicular cysts. Biol Reprod. (2003) 69:218–23. doi: 10.1095/biolreprod.102.012179
11. Calder MD, Manikkam M, Salfen BE, Youngquist RS, Lubahn DB, Lamberson WR, et al. Dominant bovine ovarian follicular cysts express increased levels of messenger RNAs for luteinizing hormone receptor and 3 beta-hydroxysteroid dehydrogenase delta(4),delta(5) isomerase compared to normal dominant follicles. Biol Reprod. (2001) 65:471–6. doi: 10.1095/biolreprod65.2.471
12. Garverick HA. Ovarian follicular cysts in dairy cows. J Dairy Sci. (1997) 80:995–1004. doi: 10.3168/jds.S0022-0302(97)76025-9
13. Koch AE, Polverini PJ, Kunkel SL, Harlow LA, DiPietro LA, Elner VM, et al. Interleukin-8 as a macrophage-derived mediator of angiogenesis. Science. (1992) 258:1798–801. doi: 10.1126/science.1281554
14. Garcia-Velasco JA, Arici A. Chemokines and human reproduction. Fertil Steril. (1999) 71:983–93. doi: 10.1016/S0015-0282(99)00120-X
15. Bornstein SR, Rutkowski H, Vrezas I. Cytokines and steroidogenesis. Mol Cell Endocrinol. (2004) 215:135–41. doi: 10.1016/j.mce.2003.11.022
16. Baravalle ME, Stassi AF, Velazquez MM, Belotti EM, Rodriguez FM, Ortega HH, et al. Altered expression of pro-inflammatory cytokines in ovarian follicles of cows with cystic ovarian disease. J Comp Pathol. (2015) 153:116–30. doi: 10.1016/j.jcpa.2015.04.007
17. Stassi AF, Baravalle ME, Belotti EM, Amweg AN, Angeli E, Velazquez MML, et al. Altered expression of IL-1beta, IL-1RI, IL-1RII, IL-1RA and IL-4 could contribute to anovulation and follicular persistence in cattle. Theriogenology. (2018) 110:61–73. doi: 10.1016/j.theriogenology.2017.12.048
18. Spicer LJ, Chamberlain CS, Maciel SM. Influence of gonadotropins on insulin- and insulin-like growth factor-I (IGF-I)-induced steroid production by bovine granulosa cells. Domest Anim Endocrinol. (2002) 22:237–54. doi: 10.1016/S0739-7240(02)00125-X
19. Silva JM, Hamel M, Sahmi M, Price CA. Control of oestradiol secretion and of cytochrome P450 aromatase messenger ribonucleic acid accumulation by FSH involves different intracellular pathways in oestrogenic bovine granulosa cells in vitro. Reproduction. (2006) 132:909–17. doi: 10.1530/REP-06-0058
20. Kawashima C, Fukihara S, Maeda M, Kaneko E, Montoya CA, Matsui M, et al. Relationship between metabolic hormones and ovulation of dominant follicle during the first follicular wave post-partum in high-producing dairy cows. Reproduction. (2007) 133:155–63. doi: 10.1530/REP-06-0046
21. Zulu VC, Sawamukai Y, Nakada K, Kida K, Moriyoshi M. Relationship among insulin-like growth factor-I, blood metabolites and postpartum ovarian function in dairy cows. J Vet Med Sci. (2002) 64:879–85. doi: 10.1292/jvms.64.879
22. Vanholder T, Leroy JL, Dewulf J, Duchateau L, Coryn M, de Kruif A, et al. Hormonal and metabolic profiles of high-yielding dairy cows prior to ovarian cyst formation or first ovulation post partum. Reprod Domest Anim. (2005) 40:460–7. doi: 10.1111/j.1439-0531.2005.00601.x
23. Probo M, Comin A, Cairoli F, Faustini M, Kindahl H, De Amicis I, et al. Selected metabolic and hormonal profiles during maintenance of spontaneous ovarian cysts in dairy cows. Reprod Domest Anim. (2011) 46:448–54. doi: 10.1111/j.1439-0531.2010.01688.x
24. Ortega HH, Palomar MM, Acosta JC, Salvetti NR, Dallard BE, Lorente JA, et al. Insulin-like growth factor I in sera, ovarian follicles and follicular fluid of cows with spontaneous or induced cystic ovarian disease. Res Vet Sci. (2008) 84:419–27. doi: 10.1016/j.rvsc.2007.05.010
25. Rodriguez FM, Salvetti NR, Panzani CG, Barbeito CG, Ortega HH, Rey F. Influence of insulin-like growth factor-binding proteins-2 and−3 in the pathogenesis of cystic ovarian disease in cattle. Anim Reprod Sci. (2011) 128:1–10. doi: 10.1016/j.anireprosci.2011.08.007
26. Vanholder T, Opsomer G, de Kruif A. Aetiology and pathogenesis of cystic ovarian follicles in dairy cattle: a review. Reprod Nutr Dev. (2006) 46:105–19. doi: 10.1051/rnd:2006003
27. Souza AH, Ayres H, Ferreira RM, Wiltbank MC. A new presynchronization system (Double-Ovsynch) increases fertility at first postpartum timed AI in lactating dairy cows. Theriogenology. (2008) 70:208–15. doi: 10.1016/j.theriogenology.2008.03.014
28. Acosta DAV, Denicol AC, Tribulo P, Rivelli MI, Skenandore C, Zhou Z, et al. Effects of rumen-protected methionine and choline supplementation on the preimplantation embryo in Holstein cows. Theriogenology. (2016) 85:1669–79. doi: 10.1016/j.theriogenology.2016.01.024
29. Johnson M., Zaretskaya I., Raytselis Y., Merezhuk Y., McGinnis S., Madden T. L. (2008). NCBI BLAST: a better web interface. Nucleic Acids Res. 36, W5–W9. doi: 10.1093/nar/gkn201
30. Khan MJ, Hosseini A, Burrell S, Rocco SM, McNamara JP, Loor JJ. Change in subcutaneous adipose tissue metabolism and gene network expression during the transition period in dairy cows, including differences due to sire genetic merit. J Dairy Sci. (2013) 96:2171–82. doi: 10.3168/jds.2012-5794
31. Khan MJ, Jacometo CB, Graugnard DE, Correa MN, Schmitt E, Cardoso F, et al. Overfeeding dairy cattle during late-pregnancy alters hepatic PPARα-regulated pathways including hepatokines: impact on metabolism and peripheral insulin sensitivity. Gene Regul Syst Bio. (2014) 8:97–111. doi: 10.4137/GRSB.S14116
32. Rozell TG, Keisler DH. Effects of oestradiol on LH, FSH and prolactin in ovariectomized ewes. J Reprod Fertil. (1990) 88:645–53. doi: 10.1530/jrf.0.0880645
33. Kirby CJ, Smith MF, Keisler DH, Lucy MC. Follicular function in lactating dairy cows treated with sustained-release bovine somatotropin. J Dairy Sci. (1997) 80:273–85. doi: 10.3168/jds.S0022-0302(97)75935-6
34. Butler ST, Pelton SH, Butler WR. Insulin increases 17 beta-estradiol production by the dominant follicle of the first postpartum follicle wave in dairy cows. Reproduction. (2004) 127:537–45. doi: 10.1530/rep.1.00079
35. Yuan JS, Reed A, Chen F, Stewart CN Jr. Statistical analysis of real-time PCR data. BMC Bioinformatics. (2006) 7:85. doi: 10.1186/1471-2105-7-85
36. Vandesompele J, De Preter K, Pattyn F, Poppe B, Van Roy N, De Paepe A, et al. Accurate normalization of real-time quantitative RT-PCR data by geometric averaging of multiple internal control genes. Genome Biol. (2002) 3:RESEARCH0034. doi: 10.1186/gb-2002-3-7-research0034
37. Monniaux D, Clemente N, Touze JL, Belville C, Rico C, Bontoux M, et al. Intrafollicular steroids and anti-mullerian hormone during normal and cystic ovarian follicular development in the cow. Biol Reprod. (2008) 79:387–96. doi: 10.1095/biolreprod.107.065847
38. Stocco DM. StAR protein and the regulation of steroid hormone biosynthesis. Annu Rev Physiol. (2001) 63:193–213. doi: 10.1146/annurev.physiol.63.1.193
39. Shimizu T, Ishizawa S, Magata F, Kobayashi M, Fricke PM, Miyamoto A. Involvement of lipopolysaccharide in ovarian cystic follicles in dairy cow: expressions of LPS receptors and steroidogenesis-related genes in follicular cells of cystic follicles. Anim Reprod Sci. (2018) 195:89–95. doi: 10.1016/j.anireprosci.2018.05.010
40. Voss AK, Fortune JE. Levels of messenger ribonucleic acid for cytochrome P450 17 alpha-hydroxylase and P450 aromatase in preovulatory bovine follicles decrease after the luteinizing hormone surge. Endocrinology. (1993) 132:2239–45. doi: 10.1210/en.132.5.2239
41. Pescador N, Soumano K, Stocco DM, Price CA, Murphy BD. Steroidogenic acute regulatory protein in bovine corpora lutea. Biol Reprod. (1996) 55:485–91. doi: 10.1095/biolreprod55.2.485
42. Bao B, Calder MD, Xie S, Smith MF, Salfen BE, Youngquist RS, et al. Expression of steroidogenic acute regulatory protein messenger ribonucleic acid is limited to theca of healthy bovine follicles collected during recruitment, selection, and dominance of follicles of the first follicular wave. Biol Reprod. (1998) 59:953–9. doi: 10.1095/biolreprod59.4.953
43. Ivell R, Bathgate R, Walther N. Luteal peptides and their genes as important markers of ovarian differentiation. J Reprod Fertil Suppl. (1999) 54:207–16.
45. Voss AK, Fortune JE. Levels of messenger ribonucleic acid for cholesterol side-chain cleavage cytochrome P-450 and 3 beta-hydroxysteroid dehydrogenase in bovine preovulatory follicles decrease after the luteinizing hormone surge. Endocrinology. (1993) 132:888–94. doi: 10.1210/endo.132.2.8425501
46. Roth Z, Biran D, Lavon Y, Dafni I, Yakobi S, Braw-Tal R. Endocrine milieu and developmental dynamics of ovarian cysts and persistent follicles in postpartum dairy cows. J Dairy Sci. (2012) 95:1729–37. doi: 10.3168/jds.2011-4513
47. Green MJ, Green LE, Medley GF, Schukken YH, Bradley AJ. Influence of dry period bacterial intramammary infection on clinical mastitis in dairy cows. J Dairy Sci. (2002) 85:2589–99. doi: 10.3168/jds.S0022-0302(02)74343-9
48. Quesnell RR, Klaessig S, Watts JL, Schukken YH. Bovine intramammary Escherichia coli challenge infections in late gestation demonstrate a dominant antiinflammatory immunological response. J Dairy Sci. (2012) 95:117–26. doi: 10.3168/jds.2011-4289
49. Kvidera SK, Dickson MJ, Abuajamieh M, Snider DB, Fernandez MVS, Johnson JS, et al. Intentionally induced intestinal barrier dysfunction causes inflammation, affects metabolism, and reduces productivity in lactating Holstein cows. J Dairy Sci. (2017) 100:4113–27. doi: 10.3168/jds.2016-12349
50. Rodriguez FM, Salvetti NR, Colombero M, Stangaferro ML, Barbeito CG, Ortega HH, et al. Interaction between IGF1 and IGFBPs in bovine cystic ovarian disease. Anim Reprod Sci. (2013) 140:14–25. doi: 10.1016/j.anireprosci.2013.04.012
51. Spicer LJ, Crowe MA, Prendiville DJ, Goulding D, Enright WJ. Systemic but not intraovarian concentrations of insulin-like growth factor-I are affected by short-term fasting. Biol Reprod. (1992) 46:920–5. doi: 10.1095/biolreprod46.5.920
52. Spicer LJ, Enright WJ, Murphy MG, Roche JF. Effect of dietary intake on concentrations of insulin-like growth factor-I in plasma and follicular fluid, and ovarian function in heifers. Domest Anim Endocrinol. (1991) 8:431–7. doi: 10.1016/0739-7240(91)90011-8
53. Diskin MG, Mackey DR, Roche JF, Sreenan JM. Effects of nutrition and metabolic status on circulating hormones and ovarian follicle development in cattle. Anim Reprod Sci. (2003) 78:345–70. doi: 10.1016/S0378-4320(03)00099-X
54. Kawashima C, Matsui M, Shimizu T, Kida K, Miyamoto A. Nutritional factors that regulate ovulation of the dominant follicle during the first follicular wave postpartum in high-producing dairy cows. J Reprod Dev. (2012) 58:10–6. doi: 10.1262/jrd.11-139N
55. Taniguchi CM, Emanuelli B, Kahn CR. Critical nodes in signalling pathways: insights into insulin action. Nat Rev Mol Cell Biol. (2006) 7:85–96. doi: 10.1038/nrm1837
56. Youngren JF. Regulation of insulin receptor function. Cell Mol Life Sci. (2007) 64:873–91. doi: 10.1007/s00018-007-6359-9
57. Lucy MC. Fertility in high-producing dairy cows: reasons for decline and corrective strategies for sustainable improvement. Soc Reprod Fertil Suppl. (2007) 64:237–54. doi: 10.5661/RDR-VI-237
58. Wathes DC, Fenwick M, Cheng Z, Bourne N, Llewellyn S, Morris DG, et al. Influence of negative energy balance on cyclicity and fertility in the high producing dairy cow. Theriogenology. (2007) 68 (suppl. 1):S232–41. doi: 10.1016/j.theriogenology.2007.04.006
59. Leroy JL, Vanholder T, Mateusen B, Christophe A, Opsomer G, de Kruif A, et al. Non-esterified fatty acids in follicular fluid of dairy cows and their effect on developmental capacity of bovine oocytes in vitro. Reproduction. (2005) 130:485–95. doi: 10.1530/rep.1.00735
60. Vanholder T, Leroy JL, Soom AV, Opsomer G, Maes D, Coryn M, et al. Effect of non-esterified fatty acids on bovine granulosa cell steroidogenesis and proliferation in vitro. Anim Reprod Sci. (2005) 87:33–44. doi: 10.1016/j.anireprosci.2004.09.006
61. Leroy JL, Rizos D, Sturmey R, Bossaert P, Gutierrez-Adan A, Van Hoeck V, et al. Intrafollicular conditions as a major link between maternal metabolism and oocyte quality: a focus on dairy cow fertility. Reprod Fertil Dev. (2011) 24:1–12. doi: 10.1071/RD11901
62. Van Hoeck V, Leroy JL, Arias Alvarez M, Rizos D, Gutierrez-Adan A, Schnorbusch K, et al. Oocyte developmental failure in response to elevated nonesterified fatty acid concentrations: mechanistic insights. Reproduction. (2013) 145:33–44. doi: 10.1530/REP-12-0174
63. Shalgi R, Kraicer P, Rimon A, Pinto M, Soferman N. Proteins of human follicular fluid: the blood-follicle barrier. Fertil Steril. (1973) 24:429–34. doi: 10.1016/S0015-0282(16)39730-8
64. Leese HJ, Lenton EA. Glucose and lactate in human follicular fluid: concentrations and interrelationships. Hum Reprod. (1990) 5:915–9. doi: 10.1093/oxfordjournals.humrep.a137219
Keywords: ovarian cyst, cytokines, steroidogenic enzymes, metabolism, dairy cows
Citation: Lima FS, Acosta DAV, Egan TR, Skenandore C, Sulzberger S, French DD and Cardoso FC (2019) Steroidogenic, Metabolic, and Immunological Markers in Dairy Cows Diagnosed With Cystic Ovarian Follicles at Early and Mid-Late Lactation. Front. Vet. Sci. 6:324. doi: 10.3389/fvets.2019.00324
Received: 30 May 2019; Accepted: 10 September 2019;
Published: 26 September 2019.
Edited by:
Arumugam Kumaresan, National Dairy Research Institute (ICAR), IndiaReviewed by:
Mallikarjun Bidarimath, College of Veterinary Medicine, Cornell University, United StatesDivakar Justus Ambrose, University of Alberta, Canada
Copyright © 2019 Lima, Acosta, Egan, Skenandore, Sulzberger, French and Cardoso. This is an open-access article distributed under the terms of the Creative Commons Attribution License (CC BY). The use, distribution or reproduction in other forums is permitted, provided the original author(s) and the copyright owner(s) are credited and that the original publication in this journal is cited, in accordance with accepted academic practice. No use, distribution or reproduction is permitted which does not comply with these terms.
*Correspondence: Fabio S. Lima, flima@illinois.edu