- 1Departamento de Morfología, Centro de Ciencias Básicas, Universidad Autónoma de Aguascalientes, Aguascalientes, Mexico
- 2CONACYT, Centro de Investigaciones Biológicas del Noreste (CIBNOR), La Paz, Mexico
- 3Departamento de Fisiología y Farmacología, Centro de Ciencias Básicas, Universidad Autónoma de Aguascalientes, Aguascalientes, Mexico
- 4Groupe de Recherche sur la Maladies Infectieuses en Production Animale (GREMIP), Faculté de Médecine Vétérinaire, Université de Montréal, St-Hyacinthe, QC, Canada
- 5Departamento de Microbiología Molecular, Instituto de Biotecnología, Universidad Nacional Autónoma de México, Cuernavaca, Mexico
Actinobacillus pleuropneumoniae, the etiological agent of porcine pleuropneumonia, represents one of the most important health problems in the swine industry worldwide and it is included in the porcine respiratory disease complex. One of the bacterial survival strategies is biofilm formation, which are bacterial communities embedded in an extracellular matrix that could be attached to a living or an inert surface. Until recently, A. pleuropneumoniae was considered to be an obligate pathogen. However, recent studies have shown that A. pleuropneumoniae is present in farm drinking water. In this study, the drinking water microbial communities of Aguascalientes (Mexico) swine farms were analyzed, where the most frequent isolated bacterium was Escherichia coli. Biofilm formation was tested in vitro; producing E. coli biofilms under optimal growth conditions; subsequently, A. pleuropneumoniae serotype 1 (strains 4074 and 719) was incorporated to these biofilms. Interaction between both bacteria was evidenced, producing an increase in biofilm formation. Extracellular matrix composition of two-species biofilms was also characterized using fluorescent markers and enzyme treatments. In conclusion, results confirm that A. pleuropneumoniae is capable of integrates into biofilms formed by environmental bacteria, indicative of a possible survival strategy in the environment and a mechanism for disease dispersion.
Introduction
Actinobacillus pleuropneumoniae is a Gram-negative coccobacillus, pleomorphic, facultative anaerobe, non-spore forming, encapsulated (1) and a member of the Pasteurellaceae family (2–4). A. pleuropneumoniae is the etiological agent of porcine pleuropneumonia; one of the most important health problems in the swine industry worldwide, and along with other porcine respiratory pathogens, this pathogen is also included in the Porcine Respiratory Disease Complex (5–7). Isolates can be classified into two biotypes depending on their requirement for nicotinamide adenine dinucleotide (NAD-dependant and NAD-independant). There are 16 recognized serovars (8). In Mexico, swine pleuropneumonia is widespread (6, 7, 9–11). Infection usually occurs through air or by direct contact. The microorganism is able to colonize the tonsils and to adhere to the alveolar epithelium. In general, the initial step is the bacterial colonization and adhesion to host cells (12).
Biofilms are microorganisms three-dimensional complex communities embedded in an extracellular matrix, where displayed characteristic phenotypes are similar to the free-living organisms, also known as planktonic (13–18). Biofilms have a dynamic structure in which a multitude of metabolic interactions between neighboring cells are developed (19). Naturally, the dominant growth of microorganisms is through multi-species consortia, regulated by a variety of important intra- and inter-specific interactions in development, composition, structure, and function (20–22). These bacterial microbial communities constitute a multi-species society, with its own “rules and patterns of behavior” (23).
Recently, (24) described the involvement of biofilm formation during the infection process of A. pleuropneumoniae. However, few studies have been done on its ability to survive outside of the pig so as to be considered an obligate pathogen. Assavacheep and Rycroft (25) investigated the survival of A. pleuropneumoniae under controlled laboratory conditions. In aqueous suspension, survival was improved by the presence of NaCl and mucin; as well as lowered temperature. Our group has detected the presence of A. pleuropneumoniae in drinking water from pig farms in Mexico using antibodies and a specific PCR for the gene of the ApxIV toxin (6, 26). Subsequently, we evaluated the ability of A. pleuropneumoniae to form multi-species biofilms with other swine bacterial pathogens in the absence of pyridine compounds (nicotinamide mononucleotide [NMN], riboside nicotinamide [NR], or nicotinamide adenine dinucleotide [NAD]) that are essential for growth of A. pleuropneumoniae (27). A. pleuropneumoniae was able to grow with all species tested in the absence of pyridine compounds. Furthermore, A. pleuropneumoniae was able to form strong biofilms when mixed with Streptococcus suis, Bordetella bronchiseptica, or Staphylococcus aureus. Notably, in the presence of Pasteurella multocida, and Escherichia coli, A. pleuropneumoniae was able to form a two-species biofilm, although this was weaker than the biofilms formed with other bacteria (27).
In this study, Escherichia coli strains isolated from the microbial community of drinking water of swine farms of the State of Aguascalientes were characterized and evaluated to explore their possible interaction with A. pleuropneumoniae to form two-species biofilms, suggesting a possible mechanism used by A. pleuropneumoniae to survive in the drinking water in pig farms, and in the environment. Furthermore, changes in the composition of the extracellular matrix during the formation of these two-species biofilms were also characterized.
Materials and Methods
Sampling of Drinking Water in Swine Farms
The study was performed in the swine farm of the Universidad Autonoma de Aguascalientes, Aguascalientes, Mexico. The farm is used for breeding and fattening pigs for teaching-learning purposes. Random water samples were aseptically obtained from drinkers located on the floor of barnyard from select areas on the farm (26). Water samples were taken directly from the drinkers at its deepest zone with 50 ml sterile Corning tubes. Samples were stored at room temperature until used for bacteria isolation.
Isolation of Bacteria From Drinking Water Samples
Samples were centrifuged at 10,000 × g for 10 min to recover the bacteria and the supernatant was discarded. The obtained pellets were re-suspended in the remaining volume. Dilutions were made in distilled water in the order 103, 104, 105, 106, plated on BHI (agar brain-heart infusion, Bioxon, Mexico) and incubated at 37°C for 24 h. Colonies of each bacterium were plated alone on BHI agar and incubated at 37°C for 24 h. All isolated bacteria were stored in glycerol 30% and stored at −80°C.
Characterization of Isolates
Once isolation of bacteria from drinking water was made, morphological characterization of the colonies and the biochemical tests such as Gram stain, catalase and oxidase were performed. All the strains were evaluated by Api NE biochemistry test (BioMérieux, France), according to the manufacturer's instructions.
Confirmation of E. Coli Isolation
Escherichia coli isolation was confirmed by PCR as previously reported (28) by de presence of uidA gene, which encodes the beta-glucuronidase enzyme. Phylogenetic group of each strain was identified (29). Escherichia coli isolated from drinking water were screened for the presence of selected virulence genes usually associated with the E. coli strains responsible for extra-intestinal infections, including: fyuA (yersiniabactin receptor), kpsMTII (capsular polysaccharide genes), and papC [P fimbriae, (30)]. In order to detect the genes agn43 [antigen 43, (31)], fimH [minor component of type 1 fimbriae, (32)], hlyA [haemolysin, (33)], and afa [afimbrial adhesins, (30)] a multiplex PCR was designed with the following conditions: 94°C for 5 min followed by 40 cycles of 30 sec at 94°C, 1 min at 60°C and 1 min at 68°C with a final elongation step at 72°C for 10 min. For the sequences of the primers see Table 1. The amplification products were observed by electrophoresis in 1.5% agarose gel stained with 1 μg ethidium bromide ml−1.
The strains used for positive controls were: E. coli strains H10407, E22, CFT073, ECOR 70, 042, EDL933, and ECOR 36. All control strains were kindly provided by Laboratoire de référence pour Escherichia coli, EcL, Faculté de Médecine Vétérinaire, Université de Montréal.
Escherichia Coli Biofilm Formation
Mono-species biofilms of E. coli isolates were obtained as previously described (34, 35) with modification. Culture medium for the formation of these mono-species biofilm was Luria Bertani (LB). Briefly, overnight cultures of E. coli were diluted 1/100 in LB broth plus glycerol (0.20%). A volume (100 μl) was aliquoted by triplicate in wells of a sterile 96-well microtiter plate (Costar® 3599, Corning, NY, USA). E. coli strains ATCC 25922 (clinical isolate [American Type Culture Collection, Manassas, VA, US]) and L17608 (swine isolate) were used as positive controls. Wells containing sterile broth were used as negative controls. Following an incubation of 24 h at 37°C, the plate was washed by immersion in water. Biofilms were then stained with 0.1% (w/v) crystal violet for 2 min, rinsed once with distilled water, dried at 37°C for 30 min, and then, 100 μl of ethanol (70%) were added to the wells. Absorbance was measured at 590 nm using a spectrophotometer. For the qualitative determination of the ability of E. coli to form biofilm, the previously described methodology was carried out (36).
Integration of Actinobacillus Pleuropneumoniae in Biofilms Formed by Escherichia Coli
To analyze the incorporation of A. pleuropneumoniae in preformed E. coli biofilms, a previously described methodology with several modifications was used (27). For this test, two strains of A. pleuropneumoniae (reference strain 4074 and swine isolated strain 719), both belonging to serotype 1 and biotype 1, were used. Briefly, overnight cultures of A. pleuropneumoniae grown in BHI broth plus NAD (15 μg/ml) and E. coli grown in LB culture media, were diluted 1/100 in LB broth plus glycerol (0.20%). A volume (200 μl) was aliquoted by triplicate in wells of a sterile 96-well microtiter plate (Costar® 3599, Corning, NY, USA) using the following template: 100 μl A. pleuropneumoniae in BHI (glycerol 0.20%) plus 100 μl E. coli in LB (glycerol 0.20%) and incubated 24 h at 37°C. Wells containing sterile broth or A. pleuropneumoniae (100 μl of bacteria plus 100 μl of LB-glycerol 0.20%) were used as blank and negative control, respectively (A. pleuropneumoniae it is unable to grow and form biofilms under these conditions). Wells containing E. coli ATCC 25922 and L17608 (100 μl of bacteria plus 100 μl of LB-glycerol 0.20%) were used as positive controls for biofilm formation.
Colony Forming Unit (CFU) Counts of Mono and Two-Species Biofilms
To confirm the presence of A. pleuropneumoniae and E. coli in the biofilms, the colony forming units (CFU) were counted, using selective growth media and colony morphology. The CFU test was performed as previously described (27, 37) with modifications. Briefly, the medium was carefully removed from each well by pipetting and washed with 200 μl of sterile water. Twenty microliters of NaCl 0.85% were added. A tip was used to scrape the bottom and completely disintegrate the biofilm, taking 20 μl to perform serial dilutions in saline solution 0.85% (from 10−2 to 10−7). Finally, 100 μl of the dilution were plated on BHI, BHI plus NAD, and Blood agar plus NAD (A. pleuropneumoniae causes beta-hemolysis), incubated 24 h at 37°C, and the CFU count was performed.
Confocal Laser Scanning Microscopy (CLSM)
In order to study the morphology of mono and two-species biofilms, E. coli biofilms with or without A. pleuropneumoniae 719 were prepared as described above and stained with FilmTracer FM 1-43 (Invitrogen, Eugene, OR), Wheat Germ Agglutinin (WGA-Oregon Green 488, Molecular Probes), Film Tracer TM SYPRO® Ruby biofilm matrix stain (Molecular Probes), or BOBOTM-3 iodide (Molecular Probes) according to manufacturer's instructions (fluorescent markers stain bacterial membranes, N-acetyl-Dglucosamine [PGA] and N-acetylneuraminic acid residues, proteins and extracellular DNA or eDNA, respectively). After 30 min of incubation at room temperature, the fluorescent marker solution was removed, and the biofilms were washed with water. After that, the biofilms were observed by confocal laser scanning microscopy (CLSM; LMS 700 ZEISS; Carl Ziess Microscopy, Jena, Germany) and images were acquired using Zen Black 2012 (black edition) software (ZEISS).
Enzymatic Treatments of Two-Species Biofilms
The enzymatic treatment assays were performed as described previously (3) for proteinase K and DNase I, and (38) for cellulase. Biofilms were prepared as described above and 50 μL of proteinase K (500 μg/mL in 50 mM Tris-HCl pH 7.5, 1 mM CaCl2), 50 μL of DNase I (500 μg/mL in 150 mM NaCl, 1 mM CaCl2), or 50 μL of cellulase (40 μU/ml in 100 mM C2H3NaO2, 50% DMSO) were added directly to the biofilms. Samples with proteinase K or DNase I were incubated for 1 h at 37°C, and with cellulose were incubated 30 min at 37°C. Control wells were treated with 50 μL of the buffer without the enzyme. Biofilms were washed and stained with crystal violet and the absorbance was measured at 590 nm.
Phenotype Assay: Congo-Red and Calcofluor
Congo-red and calcofluor assays were performed in order to determine the production of fimbriae-curli and cellulose, and were performed as described previously (38). For the assay, a 2 μl drop of bacterial culture was taken from liquid medium, and was placed on Luria-Bertani salt-free plates (LB; Difco Laboratories, Detroit, MI), containing 0.02% of Congo-red (Sigma®, C-6767) and 0.002% of Coomassie brilliant blue G (Sigma®, F3546-5G) for fimbriae detection, and containing 0.02% calcofluor (fluorescent brightener 28, Sigma-Aldrich® F-3543) dissolved in 1 mM HEPES for cellulose detection. For the two-species assay, a 1:1 dilution of the bacterial cultures (E. coli plus A. pleuropneumoniae) was performed in respective culture media. Twelve strains were placed per plate with a centimeter of distance between each drop. After seeding, the plates were allowed to dry for 5 to 10 min face-up and then incubated for 48 hat 30°C. The fluorescence of the colonies was verified by UV light illumination (360 nm) after overnight incubation at 30°C. E. coli CFT073 and E. coli ATCC 25922 were used as positive and negative controls, respectively (39).
Scanning Electron Microscopy
The two-species biofilm formed by A. pleuropneumoniae 719-E. coli ATCC 25922 was observed under electron microscopy (SEM). Mono-species biofilms of A. pleuropneumoniae and E. coli were used as positive controls and were grown as described previously. The two-species biofilm was prepared as described above. Samples were processed as described Loera-Muro et al. (26), and were observed with a Jeol LV-5900 scanning electron microscope. The bacteria dimensions were measured with the microscope software. The experiment was repeated three times, measured between 3 and 5 bacteria in three different fields.
Statistical Analysis
Statistical significance analyses (p-value < 0.05) of differences in biofilms were determined by Two-way ANOVA followed by Tukey's test using GraphPad Prism version 4.0 (GraphPad Software, San Diego, CA, USA).
Results
Identification of Bacteria From Drinking Water of Swine Farms
A total of 10 samples of drinking water from pig farm were obtained. After performing the isolation of bacteria from water, 52 colonies were selected for identification, 63.46% (33/52) of them were Gram-negative and 36.54% (19/52) were Gram-positive. Gram-negative bacteria belonged to the following species: Escherichia coli (30.30%, 10/33), Enterobacter cloacae (3.03%, 1/33), Pseudomonas aeruginosa (9.09%, 3/33), P. fluorescens (9.09%, 3/33), Photobacterium damselae (3.03%, 1/33), Salmonella spp. (12.12%, 4/33), Ochrobactrum anthropic (9.09%, 3/33), Pasteurella pneumotropica (3.03%, 1/33), Cryseumonas luteola (3.03%, 1/33), Kluyvera spp. (6.06%, 2/33), Citrobacter freuindii (6.06%, 2/33), Buttiauxella agrestis (3.03%, 1/33), and Cedecea lapagei (3.03%, 1/33). Bacteria identified as E. coli were selected for characterization of two-species biofilm formation with A. pleuropneumoniae since E. coli was the main species in the samples.
Characterization of the E. Coli Isolates
Ten E. coli isolates were characterized by PCR. All isolates belonged to the group of extra-intestinal pathogenic E. coli [strains carrying ExPEC related genes: fyuA, papC, kpsMTII, afa, fimH, agn43, and hlyA (30–33)]. Phylogroups detected include: D (40%, 4/10), B1 (20%, 2/10), A (20%, 2/10), C and E (10%, 1/10) groups (Table 2).
Escherichia Coli Biofilm Formation and Integration of A. Pleuropneumonia Into biofilms
Mono-species biofilm formation of 10 E. coli isolates and two controls, belonging to the strains ATCC 25922 and L17608 were performed (Figure 1). Integration of A. pleuropneumoniae (4074 and 719 strains) in biofilms formed by E. coli was also tested. For A. pleuropneumoniae 4074, statistically significant (p < 0.05; Figure 1A) increments of biofilm formation were detected among the isolates EcL17608 and 8–3. In the case of A. pleuropneumoniae 719, 58 percent of the E. coli strains (7/12 strains), showed statistically significant p < 0.05; Figure 1B) increments in biofilm formation.
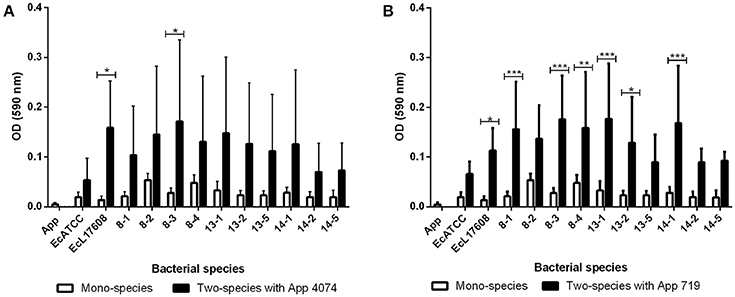
Figure 1. Mono-species and two-species biofilms formed by E. coli isolates from drinking water of a swine farm alone or with A. pleuropneumoniae. (A) Mono-species biofilms of control A. pleuropneumoniae 4074 and each E. coli isolate and two-species biofilms formed with A. pleuropneumoniae 4074 and each E. coli isolate. (B) Mono-species biofilms of control A. pleuropneumoniae 719 and each E. coli isolate, and two-species biofilms formed with A. pleuropneumoniae 719 and each E. coli isolate. All the statistically significant differences in the production between mono-species and two-species biofilms are pointed. *p < 0.05, **p < 0.01, ***p < 0.001, ****p < 0.0001.
Moreover, based on the results of the assay for two-species biofilm formation, the methodology of (36) was applied for the qualitative determination of the ability to form biofilm. The optical density of control (ODc) was defined as the mean OD of the negative control and strains were classified as non-adherent (OD ≤ ODc), weakly adherent (ODc < OD ≤ 2 × ODc), moderately adherent (2 × ODc < OD ≤ 4 × ODc), or strongly adherent (OD > 4 × ODc). In the mono-species biofilms, only 8–2 and 8–4 E. coli isolates were able to form a weak adherent biofilm (Figure 2). However, biofilm formed by A. pleuropneumoniae 4074 or 719 with E. coli increased biofilm formation from weakly adherent to moderately adherent in some combinations (Figure 2). In the case of two-species biofilms with A. pleuropneumoniae 4074, six isolates increased their adhesion ability to weakly adherent (8–1, 13–2, 13–5, 14–1, 14–2, and 14–5 isolates) and the remaining isolates increased their adhesion ability to moderately adherent (8–2, 8–3, 13–1 isolates and the control Ec L17608). The control ATCC 25922 did not suffer any modification (Figure 2). For A. pleuropneumoniae 719, six of the isolates increased their adhesion ability to moderately adherent (8–1, 8–2, 8–3, 8–4, 13–1, and 14–1) and the remaining six isolates including the controls ATCC 25922 and Ec L17608 increased their adhesion ability to weakly adherent (13–2, 13–5, 14–2, and 14–5 isolates).
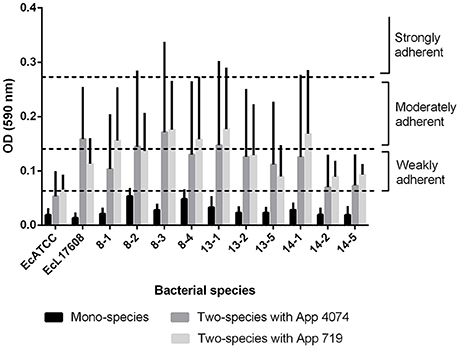
Figure 2. Classification of biofilm formation capacity as weak, moderate and strongly adherent in E. coli mono-species biofilms and E. coli- A. pleuropneumoniae two-species biofilms (E. coli- A. pleuropneumoniae 4074 and E. coli- A. pleuropneumoniae 719). In all cases it can be observed that addition of A. pleuropneumoniae promotes increases on biofilm production, from weak when are mono-species to moderate when are two-species.
Colony Forming Unit Counts of Mono and Two-Species Biofilms
In the case of the CFU count, the numbers of A. pleuropneumoniae and E. coli found in two-species biofilms were similar in almost all cases (Figure 3). No significant differences between populations were shown. These results suggest strongly that A. pleuropneumoniae has the ability to be incorporated into biofilms produced by environmental bacteria, which supports that A. pleuropneumoniae is using the multi-species biofilms as a survival strategy in the environment, at least for 72 h of interaction. For all subsequent assays, A. pleuropneumoniae reference strain 719 was selected because this strain isolated from pigs has a high capacity for biofilm formation.
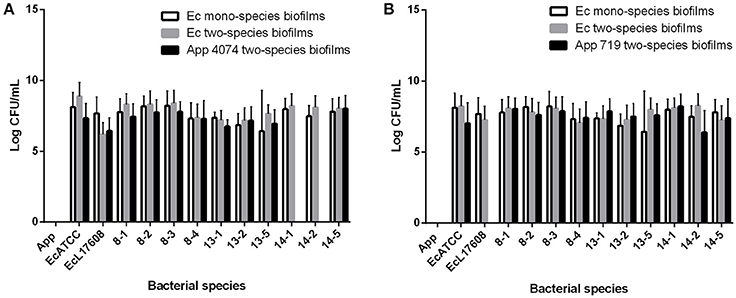
Figure 3. Colony forming units (CFU) counts from mono and two-species biofilms formed by A. pleuropneumoniae with the E. coli isolates from drinking water of a swine farm. (A) CFU of two-species biofilms formed by A. pleuropneumoniae 4074 with each E. coli isolate. (B) CFU of two-species biofilms formed by A. pleuropneumoniae 719 with each E. coli isolate. Ec mono-species biofilms bar represent the CFU of E. coli bacteria from the mono-species biofilms. Ec two-species biofilms and App two-species biofilms bar represent the CFU of each bacterium from the two-species biofilms.
Two-Species Biofilms Matrix Composition
Confocal laser scanning microscopy (CLSM) and the dye FM-143 was carried out to visualize biofilm morphology from both, mono- and two-species biofilm. Observation of several fields on each sample evidenced by increments in most of the two-species biofilms formed with different E. coli strains and the A. pleuropneumoniae isolates, as compared to the mono-species biofilms of E. coli (Figure 4). As a whole, these images are in accordance with the results obtained by the crystal violet technique. Likewise, it was observed that the biofilms morphology had some changes (Figures 4, 5). Otherwise, biofilm matrix components were characterized by CLSM in combination with different dyes directed mainly toward PGA, eDNA, and proteins. These three macromolecules were detected in the extracellular matrix (Figure 6). In some cases, the production of proteins, PGA and eDNA, stained with SYPRO Ruby, WGA, and BOBO-3; respectively, showed an increase in biofilms formed by two-species compared to mono-species biofilms (Figure 6). The data obtained from the SYPRO Ruby stain, which labels most classes of proteins, showed a protein increase in the two-species biofilms E. coli 14–2 and EcATCC with A. pleuropneumoniae 719. The isolates 8–4, 13–1, 13–2, 13–5, 14–1, and EcATCC were stained with WGA, suggesting the increase in the presence of PGA or at least in the presence of N-acetyl-d-glucosamine and N-acetylneuraminic acid residues in the biofilm matrix. BOBO-3 iodide that stains extracellular DNA showed an increase only among the isolates 14-2 and EcATCC.
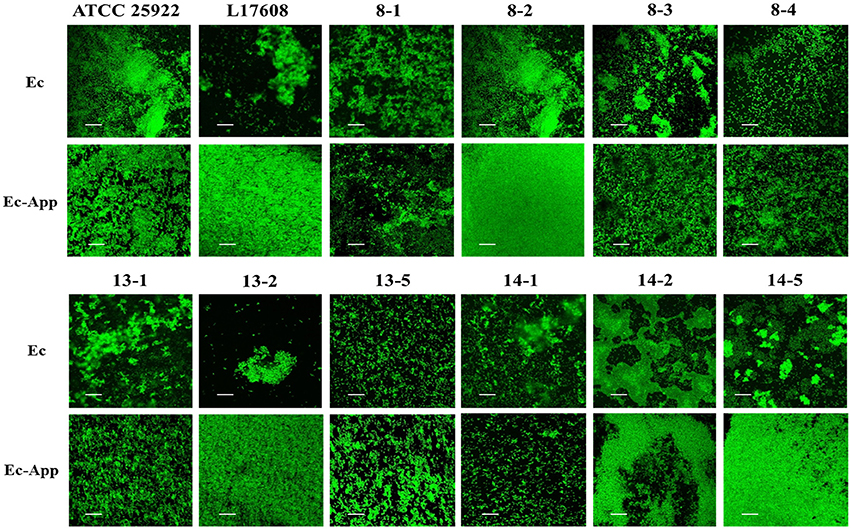
Figure 4. Escherichia coli strains in mono- or two-species biofilms with A. pleuropneumoniae (strain 719) observed by confocal laser scanning microscopy. Biofilms were stained with FM-143. The panel shows views from the top of biofilms. Ec; E. coli, App; A. pleuropneumoniae 719. Scale bar 30 μm.
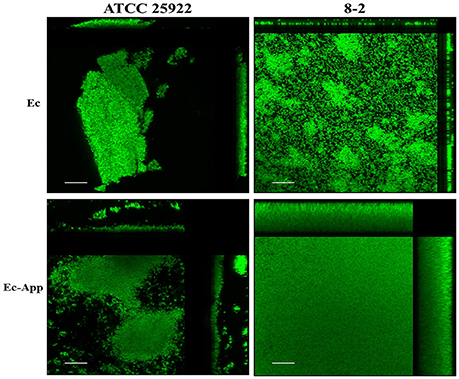
Figure 5. Confocal laser scanning of mono or two-species biofilms formed by E. coli alone or with A. pleuropneumoniae. Images show the increment in the biofilms when the bacteria are in multi-species (stained with FM-143). Side panels (top and right) show cross-section views from the sides of the biofilms Ec; E. coli, App; A. pleuropneumoniae 719. Scale bar 30 μm.
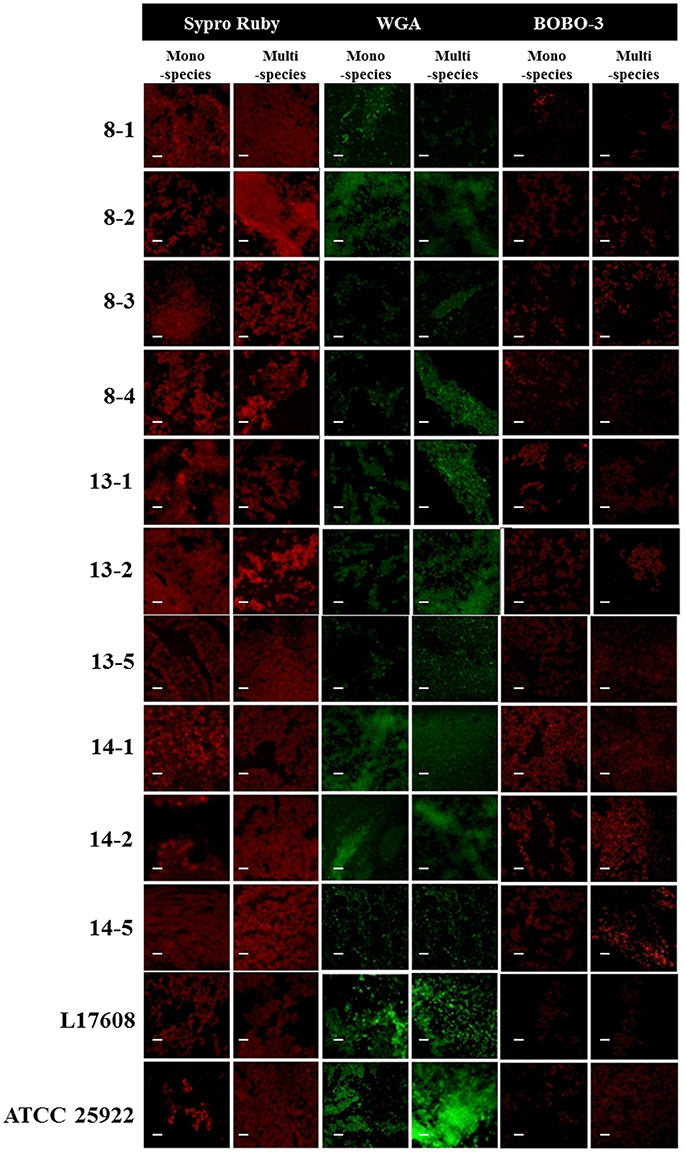
Figure 6. Escherichia coli strains in mono- or two-species biofilms with A. pleuropneumoniae (strain 719) observed by confocal laser scanning microscopy. Images show the mono-species biofilms of E. coli isolates and two-species biofilms of E. coli isolates and A. pleuropneumoniae 719 in LB media stained with wheat-germ agglutinin (WGA)-Oregon green, SYPRO Ruby, and BOBO-3 (all from Invitrogen, Eugene, OR). Scale bar 30 μm.
Congo-red has been used extensively to supplement nutrient agar to distinguish the production of the extracellular matrix components cellulose and curli fimbriae from non-cellulose curliated bacteria. Likewise, the phenotype on calcofluor plates served as an indicator of cellulose production. In this work, the presence of few fimbriae-curli forming, and cellulose producer strains was observed (13-1, 13-2, 13-5, 14-1, 14-2, and 14-5, Figure 7). Also, changes were observed when the E. coli strains were together to A. pleuropneumoniae in the cellulose and curli production. These results confirm changes in the production of extracellular matrix components in two-species biofilms as compared to the E. coli mono-species.
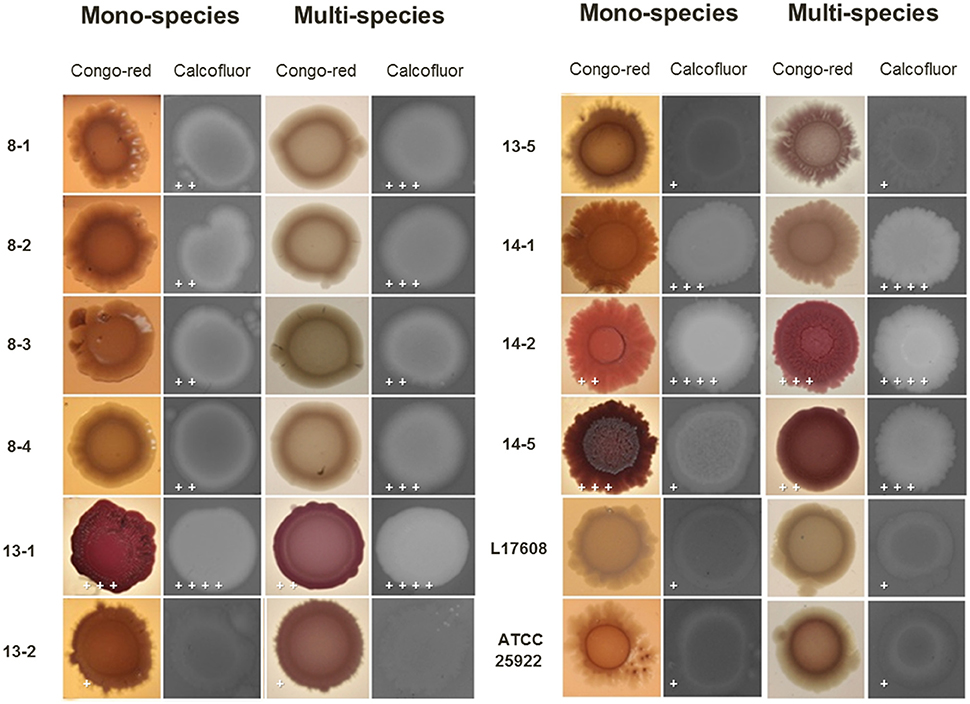
Figure 7. Congo-red and calcofluor binding assays on mono or two-species colonies formed by E. coli alone or with A. pleuropneumoniae. Congo-red assay evidenced curli-producing bacteria when E. coli was growing on congo-red-supplemented nutrient agar. Moreover, changes in cellulose production were detected by the calcofluor assay. The plus sings indicate differences on production observed in the colonies of one or two-species. The crosses indicate the qualitative production of cellulose seen in each of the biofilms (+ low, ++ medium, +++ medium high and ++++ high).
To determine the structural roles played by the compounds forming the extracellular matrix, enzymatic treatments were performed on the two and mono-species biofilms (Figure 8). Treatment with proteinase K, DNase I and cellulose provoked reduction in all two-species biofilms. It was more important that the effect observed in the mono-species biofilms. These results indicate that when biofilms of two-species are being formed, the cellulose, as well as proteins and eDNA, take a structural function as occur in mono-species biofilms.
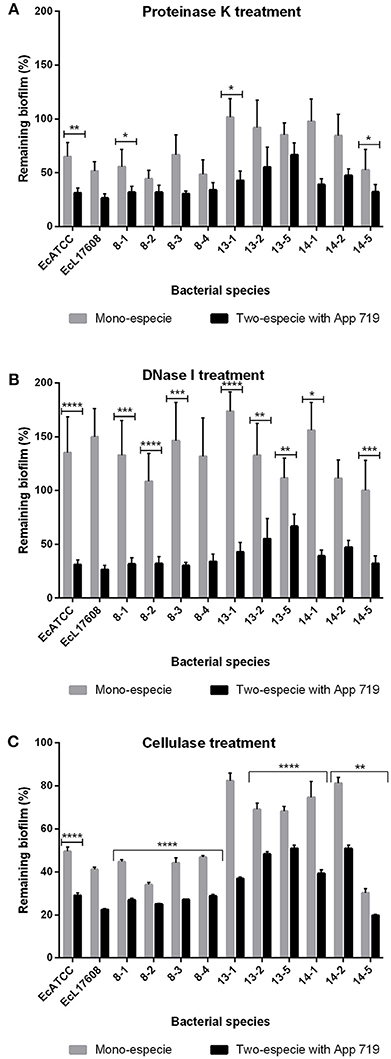
Figure 8. Mono or two-species biofilms of E. coli and A. pleuropneumoniae enzymatic treatment. Dispersion by (A) proteinase K, (B) DNase I, and (C) cellulase of mono or two-species biofilms formed by E. coli strains alone or with A. pleuropneumoniae strain 719 grown in LB media. *p < 0.05, **p < 0.01, ***p < 0.001, ****p < 0.0001.
Scanning Electron Microscopy
Actinobacillus pleuropneumoniae and E. coli two-species biofilms were analyzed by SEM (Figure 9). It was possible to observe the presence of two populations in the two-species biofilms, an abundant population of larger bacteria, and a minor population of smaller bacteria (p < 0.01, Figure 9D). It was also interesting to observe fimbriae-like or curli-like structures, and their promotion of interaction between all the bacteria present in the biofilm. Moreover, these structures appear more abundant in the E. coli mono-species biofilm (Figure 9B) than in the E. coli—A. pleuropneumoniae two-species biofilm (Figure 9C).
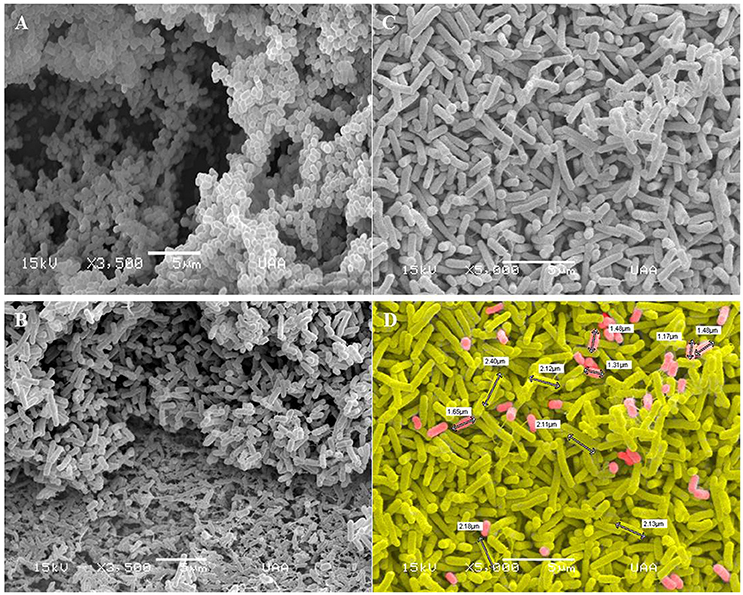
Figure 9. Scanning electron microscopy images of biofilms formed by A. pleuropneumoniae and E. coli. Mono-species and two-species biofilms constituted by (A) A. pleuropneumoniae (719), (B) E. coli (ATCC 25922) (Magnification: 3500x) and (C–D) A. pleuropneumoniae and E. coli (719 and ATCC 25922 respectively) (Magnification: 5000x). Fimbria- and curli-like structures in biofilms formed by E. coli and E. coli- A. pleuropneumoniae are indicated. (D) shows the sizes of bacteria (white labels); cells were painted to distinguish the two apparent populations of bacteria. Scale bar 5 μm.
Discussion
Porcine pleuropneumonia caused by A. pleuropneumoniae, is one of the most important porcine respiratory diseases which is spread by direct contact between the carrier-infected pig and an uninfected pig or by aerosols (1–5). The indirect route of transmission via surface was not considered very important and therefore the ability of A. pleuropneumoniae to survive in the environment outside of its host is not yet known (25). Previous studies from our group demonstrated that A. pleuropneumoniae is able to grow in unsuitable environments when forming multi-species biofilms with other respiratory pathogens of pigs that are also part of the porcine respiratory disease complex (6, 17, 27). In this study, a total of 10 samples of drinking water were taken from a swine farm in the State of Aguascalientes where previously Loera-Muro et al. (26) detected the presence of A. pleuropneumoniae in drinking water using a specific PCR for apxIV gene. We found that some microorganisms that form the microbial community of drinking water of swine farms were bacteria such as Escherichia, Enterobacter, Pseudomonas, Photobacterium, Salmonella, Ochrobactrum, Pasteurella, Cryseumonas, Kluyvera, Citrobacter, and Buttiauxella. The more abundant culturable bacterium isolated from samples of drinking water from a swine farm in the State of Aguascalientes was E. coli. All E. coli isolates belonged to the group of extra-intestinal pathogens (ExPEC). ExPEC are facultative pathogens, which can reside in the gastrointestinal tract of a certain fraction of the human and animal population. They possess several virulence traits that allow them to colonize different niches including urogenital tract resulting in urinary tract infections (UTIs), meningitis and sepsis in animals and humans (40). In pigs, these pathogens could cause fatal pneumonia, severe septicaemia and haemorrhagia; thus, they also represent a latent risk for human health (41–43). The presence of ExPEC strains may indicate a zoonotic potential risk posed by swine farms to cause infections by ExPEC stains in both, pigs and humans, mainly farm workers.
To seek out interactions during biofilm formation in two-species biofilms between the swine respiratory pathogen A. pleuropneumoniae (strain 4074 and 719), and E. coli isolated from drinking water, different approaches were undertaken. An increase in biofilm formation was evidenced by crystal violet staining, when two-species biofilms were compared to those obtained with the E. coli mono-species assay. This result suggests an interaction between both bacteria affecting bacterial distribution and probably biomass production as reported by others (44). Furthermore, by applying the methodology of (36), for the qualitative determination of E. coli's ability to form biofilm, this increment was observed. When A. pleuropneumoniae was combined with any of the E. coli strains, the biofilm classification changed from non-adherent to weakly adherent or moderately adherent. In addition, we were able to recover A. pleuropneumoniae from the biofilm in most cases, which was unexpected considering biofilm was cultured in optimal conditions for E. coli growth, but not for A. pleuropneumoniae. Thus, the ability of A. pleuropneumoniae to form two-species biofilm with E. coli isolated from drinking water was confirmed. This interaction occurs because E. coli possibly supplies some nutrients that promote A. pleuropneumoniae growth (27).
There are several reports on the advantages obtained during multi-species biofilm formation. Liu et al. (45) determined the capacity to incorporate the bacteria E. coli O157:H7 in pre-formed biofilms with bacteria obtained during the fresh produce processing environments. When co-cultured with E. coli O157:H7, Burkholderia caryophylli, and Ralstonia insidiosa exhibited increases in biofilm biomass, which were around 180 and 63%, respectively; as well as in the thickness of the biofilm. Biyikoglu et al. (46) reported that Actinomyces oris and Veillonella parvula promoted biofilm growth of all Fusarium nucleatum strains tested in their study. Both studies reported similar effects: increases in biofilms when they are formed by multiple species, such as in our case. The results presented here are in accordance with the study carried out by Bridier et al. (47), where pathogenic Staphylococcus aureus grown in mixed biofilm with the Bacillus subtilis ND medical strain, was protected from peracetic acid (PAA), an oxidizing agent, thus enabling its persistence in the environment. Standar et al. (37) also showed that two-species combinations of Streptococcus mitis with either Streptococcus mutans or Aggregatibacter actinomycetemcomitans favored bacterial interactions influencing biofilm mass, biofilm structure and cell viability. The result reported by Standar et al. (37) is similar to that observed in our study where E. coli in presence of A. pleuropneumoniae favored an increase in biofilm formation, allowing it to survive even under conditions unfit for its development. Likewise, the integration of pathogenic bacteria in biofilms formed by other bacteria was shown by Stewart et al. (48), where Legionella pneumophila 130b persisted within a two-species biofilm formed by Klebsiella pneumoniae and Flavobacterium sp., or by K. pneumonia, and P. aeuroginosa. Furthermore, the authors reported that Legionella pneumophila 130b was able to colonize biofilms formed by single-species such as K. pneumoniae and Pseudomonas fluorescens, and persist in the environment. Finally, (49) using the chinchilla otitis media model concluded that the biofilm formation and persistence on the middle-ear mucosal surface by pneumococcal is facilitated by Haemophillus influenzae coinfection. In this study, A. pleuropneumoniae was able to colonize and incorporate into biofilms formed by E. coli, which might allow it survive in hostile conditions, outside of its host, persisting in the environment as a source for transmission to other pigs.
Considering our results, an interaction is evidenced, between bacteria, A. pleuropneumoniae and the E. coli environmental isolates. It is unknown whether the increase in biofilm produced when going from mono to two-species is due to the incorporation of A. pleuropneumoniae into these biofilms, or a more complex interaction is, causing E. coli to over-produce biofilm, via the generation of extracellular matrix components, like cellulose, curli, antigen 43, DNA, β-1,6-N-acetylglucosamine (β-1,6-GlcNAc), capsule sugars, and colanic acid (50, 51). Our working model considers that A. pleuropneumoniae is incorporated into E. coli biofilms, thus in order to survive and grow in this hostile environment, at least for 72 h, it promotes an increment in biofilm, followed by interactions between both bacteria, resulting in the final increment seen in the two-species biofilms. Moreover, it was also possible to observe that the components of the extracellular matrix in the two-species biofilms changed their function, promoting greater structural stability to the biofilm. In the enzymatic assays a decrease in the biofilm formed by E. coli and A. pleuropneumoniae was seen, when compared to the biofilms formed only by E. coli strains. This change in the structural function of components in the extracellular matrix when going from mono-species to multi-species biofilm had already been reported by our group previously with A. pleuropneumoniae (27). However, little is known with regards to other bacterial species (52, 53).
In conclusion, our data suggests that A. pleuropneumoniae has the ability to integrate and form multi-species biofilms with environmental bacteria, which could allow it to survive outside of the host, specifically in water, establishing relationships with bacteria from the microbial community of water such as E. coli; therefore suggesting a possible mechanism for porcine pleuropneumonia persistence or transmission.
Author Contributions
FR-C directed the biofilms experiments with Escherichia coli, mono species, and di-species. AL-M advised the biofilm experiments with Actinobacillus pleuropneumoniae, mono species, and di-species. NV-P, CB-G, and AM-F conducted the experiments with biofilms for both species, and they analyzed them by confocal microscopy. FA-G, JH, MJ, and RO advised the management of bacterial strains. F-AG also advised the microbiological analysis. AG-B proposed the research line, is the responsible of the project that support this work, directed five thesis involved in the work.
Funding
Universidad Autonoma de Aguascalientes, Mexico Award number: PIBT16-3. This project was supported by a grant from CONACYT, Mexico (No. 258863). The project also was supported by Special Resource UAA for Research 2017.
Conflict of Interest Statement
The authors declare that the research was conducted in the absence of any commercial or financial relationships that could be construed as a potential conflict of interest.
Acknowledgments
We thank the workers of education and production farm of the Autonomous University of Aguascalientes, especially to MVZ Raúl Romero, who supported in obtaining samples, as well as the technical assistance to Irving Uriel Aguirre Tavera and Rogelio Salinas Gutiérrez.
References
1. Brockmeier, S, Halbur, P, and Thacker, E Porcine respiratory disease complex. In: Brogden K, Guthmiller J, editors. Polymicrobial Diseases. Washington, DC: ASM Press; American Society for Microbiology. (2002). p 231–257.
2. Dousse, F, Thomann, A, Brodard, I, Korczak, B, Schlatter, Y, Kuhnert, P, et al. Routine phenotypicidentification of bacterial species of the family Pasteurellaceae isolated from animals. J Vet Diagn Invest. (2008) 20:716–24. doi: 10.1177/104063870802000602
3. Tremblay, Y, Deslandes, V, and Jacques, M Actinobacillus pleuropneumoniae genes expression in biofilms cultured under static conditions and in a drip-flow apparatus. BMC Genomics (2013) 14:364. doi: 10.1186/1471-2164-14-364
4. Hathroubi, S, Fontaine-Gosselin, SE, Tremblay, YDN, Labrie, J, and Jacques, M Sub-inhibitory concentrations of penicillin G induce biofilm formation by field isolates of Actinobacillus pleuropneumoniae. Vet Microbiol. (2015) 179:277–86. doi: 10.1016/j.vetmic.2015.06.011
5. Opriessnig, T, Giménez-Lirola, LG, and Halbur, PG Polymicrobial respiratory disease in pigs. Anim Health Res Rev. (2011) 12:133–48. doi: 10.1017/S1466252311000120
6. Loera-Muro, VM, Loera-Muro, A, Morfín-Mata, M, Jacques, M, Avelar-González, FJ, Ramírez-Castillo, F, et al. Porcine respiratory pathogens in swine farms environment in Mexico. OJAS (2014) 4:196–205. doi: 10.4236/ojas.2014.44025
7. Loera-Muro, A, Avelar-González, F, Loera-Muro, V, Jacques, M, and Guerrero-Barrera, AL Presence of Actinobacillus pleuropneumoniae, Streptococcus suis, Pasteurella multocida, Bordetella bronchiseptica, Haemophilus parasuis and Mycoplasma hyopneumoniae in upper respiratory tract of swine in farms from Aguascalientes, Mexico. OJAS (2013) 3:132–7. doi: 10.4236/ojas.2013.32020
8. Bossé, JT, Li, Y, Sárközi, R, Gottschalk, M, Angen, Ø, Nedbalcova, K, et al. A unique capsule locus in the newly designated Actinobacillus pleuropneumoniae serovar 16 and development of a diagnostic PCR. J Clin Microbiol. (2017). 55:902–7 doi: 10.1128/JCM.02166-16
9. Ciprián-Carrasco, A, and Mendoza-Elvira, S Importancia de identificar el serotipo de Actinobacillus pleuropneumoniae en la pleuroneumonía contagiosa porcina por la clase de citolisina excretada. Porcirama (1995) 5:6–20.
10. Williams, J, Torres-León, M, Echeverria-Coello, P, and Matos-Medina, M Aislamiento e identificación de Actinobacillus pleuropneumoniae en pulmones de cerdos con pleuroneumonía crónica sacrificados en el rastro municipal de Mérida, Yucatán, México. Rev Biomed. (2000) 11:175–81.
11. Serrano-Rubio, L, Tenorio-Gutiérrez, V, Suárez, F, Reyes-Cortés, R, Rodríguez-Mendiola, M, Arias-Castro, C, et al. Identification of Actinobacillus pleuropneumoniae biovars 1 and 2 in pigs using a PCR assay. Mol Cell Probes (2008) 22:305–12. doi: 10.1016/j.mcp.2008.09.001
12. Jeannotte, M, Abul, M, Dubreuil, J, and Jacques, M Binding of Actinobacillus pleuropneumoniae to phosphatidylethanolamine. Infect Immun. (2003) 71:4657–63. doi: 10.1128/IAI.71.8.4657-4663.2003
13. Jacques, M, Aragon, V, and Tremblay, Y Biofilm formation in bacterial pathogens of veterinary importance. Anim Health Res Rev. (2010) 11:97–121. doi: 10.1017/S1466252310000149
14. Ganguly, S, and Mitchell, A Mucosal biofilms of Candida albicans. Curr Opin Microbiol. (2011) 14: 380–5. doi: 10.1016/j.mib.2011.06.001
15. Trappetti, C, Ogunniyi, A, Oggioni, M, and Paton, J Extracellular matrix formation enhances the ability of Streptococcus pneumoniae to cause invasive disease. PLoS ONE (2011) 6:e19844. doi: 10.1371/journal.pone.0019844
16. Tremblay, YD, Hathroubi, S, and Jacques, M Bacterial biofilms: their importance in animal health and public health. Can J Vet Res. (2014) 78:110–6.
17. Hathroubi, S, Loera-Muro, A, Guerrero-Barrera, AL, Tremblay, YDN, and Jacques, M Actinobacillus pleuropneumoniae biofilms: Role in pathogenicity and potential impact for vaccination development. Anim Health Res Rev. (2017) 7:1–14. doi: 10.1017/S146625231700010X
18. Loera-Muro, A, Ramírez-Castillo, FY, Avelar-González, FJ, and Guerrero-Barrera, AL Biopelículas multi-especie: asociarse para sobrevivir. Invest Cien Univ Autón Aguasc. (2012) 54:49–56.
19. Ramadam, H Chronic rhinosinusitis and bacterial biofilms. Curr Opin Otolaryngol Head Neck Surg. (2006) 14:183–6. doi: 10.1097/01.moo.0000193177.62074.fd
20. Bowen, W, and Koo, H Biology of Streptococcus mutans-derived glucosyltransferases: role in extracellular matrix formation of cariogenic biofilms. Caries Res. (2011) 45:69–86. doi: 10.1159/000324598
21. Høiby, N, Ciofu, O, Johansen, H, Song, Z, Moser, C, Jensen, P, et al. The clinical impact of the bacterial biofilms. Int J Oral Sci. (2011) 3:55–65. doi: 10.4248/IJOS11026
22. Sanchez-Vizuete, P, Orgaz, B, Aymerich, S, Le, D, and Briandet, R Pathogens protection against the action of disinfectants in multispecies biofilms. Front Microbiol. (2015) 6:705. doi: 10.3389/fmicb.2015.00705
23. Bordi, C, and De Bentzmann, S Hacking into bacterial biofilms: a new therapeutic challenge. Ann Intensive Care (2011) 1:19. doi: 10.1186/2110-5820-1-19
24. Tremblay, YD, Labrie, J, Chénier, S, and Jacques, M Actinobacillus pleuropneumoniae grows as aggregates in the lung of pigs: is it time to refine our in vitro biofilm assays?. Microb. Biotechnol. (2016) 10:756–60. doi: 10.1111/1751-7915.12432
25. Assavacheep, P, and Rycroft, A Survival of Actinobacillus pleuropneumoniae outside the pig. Res Vet Sci. (2012) 94:22–6. doi: 10.1016/j.rvsc.2012.07.024
26. Loera-Muro, V, Jacques, M, Tremblay, Y, Avelar-González, F, Loera-Muro, A, Ramírez, E, et al. Detection of Actinobacillus pleuropneumoniae in drinking water from pig farms. Microbiology (2013) 159:536–44. doi: 10.1099/mic.0.057992-0
27. Loera-Muro, A, Jacques, M, Avelar-González, FJ, Labrie, J, Tremblay, Y, Oropeza, R, et al. Actinobacillus pleuropneumoniae growth in no-supplemented NAD media and form multispecies biofilm. BMC Microbiol. (2016) 16:128. doi: 10.1186/s12866-016-0742-3
28. Ramírez-Castillo, FY, Avelar, FJ, Garneau, P, Márquez, F, Guerrero-Barrera, AL, and Harel, J Presence of multi-drug resistant pathogenic Escherichia coli in the San Pedro River located in the State of Aguascalientes, Mexico. Front Microbiol. (2013) 4:147. doi: 10.3389/fmicb.2013.00147
29. Clermont, O, Christenson, JK, Denamur, E, and Gordon, D The Clermont Escherichia coli phylo-typing method revisited: improvement of specificity and detection of new phylo-groups. Environ Microbiol Rep. (2013) 5:58–65. doi: 10.1111/1758-2229.12019
30. Johnson, JR, and Stell, AL Extended virulence genotypes of Escherichia coli strains from patients with urosepsis in relation to phylogeny and host compromise. J Infect Dis. (2000) 181:261–72. doi: 10.1086/315217
31. Vollmerhausen, TL, and Katouli, M Molecular characterisation of Escherichia coli isolated from hospitalised children and adults with urinary tract infection. Eur J Clin Microbiol Infect Dis. (2014) 33:975–82. doi: 10.1007/s10096-013-2035-1
32. Paul, S, Linardopoulou, EV, Billig, M, Tchesnokovam, V, Price, LB, Johnson, JR, et al. Role of homologous recomtination in adaptative diversification of extraintestinal Escherichia coli. J Bacteriol. (2013) 195:231–42. doi: 10.1128/JB.01524-12
33. Yamamoto, S, Terai, A, Yuri, K, Kurazono, H, Takeda, Y, and Yoshida, O Detection of urovirulence factors in Escherichia coli by multiplex polymerase chain reaction. FEMS Immunol Med Microbiol. (1995) 12:85–90. doi: 10.1111/j.1574-695X.1995.tb00179.x
34. Labrie, J, Pelletier-Jacques, G, Deslandes, V, Ramjjet, M, Nash, J, and Jacques, M Effects of growth conditions on biofilm formation by Actinobacillus pleuropneumoniae. Vet Res. (2010) 41:3–10. doi: 10.1051/vetres/2009051
35. Wu, C, Labrie, J, Tremblay, Y, Haine, D, Mourez, M, and Jacques, M Zinc as an agent for the prevention of biofilm formation by pathogenic bacteria. J Appl Microbiol. (2013) 115:30–40. doi: 10.1111/jam.12197
36. Novais, A, Vuotto, C, Pires, J, Montenegro, C, Donelli, G, Coque, TM, et al. Diversity and biofilm-production ability among isolates of Escherichia coli phylogroup D belonging to ST69, ST393 and ST405 clonal groups. BMC Microbiol. (2013) 13:144. doi: 10.1186/1471-2180-13-144
37. Standar, K, Kreikemeyer, B, Redanz, S, Münter, WL, Laue, M, and Podbielski, A Setup of an in vitro test system for basic studies on biofilm behavior of mixed-species cultures with dental and periodontal pathogens. PLoS ONE (2010) 5:e13135. doi: 10.1371/journal.pone.0013135
38. Vogeleer, P, Tremblay, YD, Jubelin, G, Jacques, M, and Harel, J Biofilm-forming abilities of shiga toxin-producing escherichia coli isolates associated with human infections. Appl Environ Microbiol. (2015) 82:1448–58. doi: 10.1128/AEM.02983-15
39. Hancocok, V, Ferrieres, L, and Klemm, P Biofilm formation by asymptomatic and virulent urinary tract infectious Escherichia coli strains. FEMS Microbiol Lett. (2007) 267:30–7. doi: 10.1111/j.1574-6968.2006.00507.x
40. Vogeleer, P, Tremblay, YD, Mafu, AA, Jacques, M, and Harel, J Life on the outside: role of biofilms in environmental persistence of Shiga-toxin producing Escherichia coli. Front Microbiol. (2014) 5:317. doi: 10.3389/fmicb.2014.00317
41. Kong, L-C, Guo, X, Wang, Z, Gao, Y-H, Jia, B-Y, Liu, S-M, et al. Whole genome sequencing of an ExPEC that caused fatal pneumonia at a pig farm in Changchun, China. BMC Vet Res. (2017) 13:169. doi: 10.1186/s12917-017-1093-5
42. Reisner, G, von Berg, S, Hillen, S, Clemens, N, Huisinger, M, Burkhart, E, et al. Haemorrhagic septicaemia in a pig caused by extrainestinal pathogenic Escherichia coli (ExPEC) as a differential diagnosis in classical swine fever-case report and review of the literature. Berl Munch Tierarztl Wochenschr. (2010) 123:119–24. doi: 10.2376/0005-9366-123-119
43. Jakobsen, L, Spangholm, DJ, Pedersen, K, Jensen, LB, Emborg, HD, Agersø, Y, et al. Broiler chickens, broiler chicken meat, pigs and pork as sources of ExPEC related virulence genes and resistance in Escherichia coli isolates from community-dwelling humans and UTI patients. Int J Food Microbiol. (2010).142:264–72. doi: 10.1016/j.ijfoodmicro.2010.06.025
44. Burmølle, M, Ren, D, Bjarnsholt, T, and Sørensen, SJ Interactions in multispecies biofilms: do they actually matter?. Trends Microbiol. (2014) 22:84–91. doi: 10.1016/j.tim.2013.12.004
45. Liu, N, Nou, X, Lefcourt, AM, Shelton, D, and Lo, YM Dual-species biofilm formation by Escherichia coli O157:H7 and environmental bacteria isolated from fresh-cut processing facilities. Int J Food Microbiol. (2014) 171:15–20. doi: 10.1016/j.ijfoodmicro.2013.11.007
46. Biyikoglu, B, Ricker, A, and Diaz, PI Strain-specific colonization patterns and serum modulation of multi-species oral biofilm development. Anaerobe (2012) 18:459–70. doi: 10.1016/j.anaerobe.2012.06.003
47. Bridier, A, Sanchez-Vizuete, MP, Le Coq, D, Aymerich, S, Meylheuc, T, Thomas, V, et al. Biofilms of a Bacillus subtilis Hospital Isolate Protect Staphylococcus aureus from Biocide Action. PLoS ONE (2012) 7:e44506. doi: 10.1371/journal.pone.0044506
48. Stewart, CR, Muthye, V, and Cianciotto, NP Legionella pneumophila Persists within Biofilms Formed by Klebsiella pneumoniae, Flavobacterium sp., and Pseudomonas fluorescens under Dynamic Flow Conditions. PLoS ONE (2012) 7:e50560. doi: 10.1371/journal.pone.0050560
49. Weimer, KE, Armbruster, CE, Juneau, RA, Hong, W, Pang, B, and Swords, WE Coinfection with Haemophilus influenzae promotes pneumococcal biofilm formation during experimental otitismedia and impedes the progression of pneumococcal disease. J Infect Dis. (2010) 202:1068–75. doi: 10.1086/656046
50. Beloin, C, Roux, A, and Ghigo, JM Escherichia coli biofilms. Curr Top Microbiol Immunol. (2010) 322:249–89.
51. Hufnagel, DA, Depas, WH, and Chapman, MR The Biology of the Escherichia coli Extracellular Matrix. Microbiol Spectr. (2015) 3:3. doi: 10.1128/microbiolspec.MB-0014-2014
52. Dominiak, D, Nielsen, JN, and Nielsen, PH Extracellular DNA is abundant and important for microcolony strength in mixed microbial biofilms. Environ Microbiol. (2011) 13:710–21. doi: 10.1111/j.1462-2920.2010.02375.x
Keywords: Actinobacillus pleuropneumoniae, Escherichia coli, respiratory pathogen, pleuropneumonia, biofilms, drinking water
Citation: Ramírez-Castillo FY, Loera-Muro A, Vargas-Padilla ND, Moreno-Flores AC, Avelar-González FJ, Harel J, Jacques M, Oropeza R, Barajas-García CC and Guerrero-Barrera AL (2018) Incorporation of Actinobacillus pleuropneumoniae in Preformed Biofilms by Escherichia coli Isolated From Drinking Water of Swine Farms. Front. Vet. Sci. 5:184. doi: 10.3389/fvets.2018.00184
Received: 26 March 2018; Accepted: 18 July 2018;
Published: 14 August 2018.
Edited by:
Claudio Verdugo, Universidad Austral De Chile, ChileReviewed by:
Wolfgang Ludwig Köster, University of Saskatchewan, CanadaShankar Thangamani, Midwestern University, United States
Copyright © 2018 Ramírez-Castillo, Loera-Muro, Vargas-Padilla, Moreno-Flores, Avelar-González, Harel, Jacques, Oropeza, Barajas-García and Guerrero-Barrera. This is an open-access article distributed under the terms of the Creative Commons Attribution License (CC BY). The use, distribution or reproduction in other forums is permitted, provided the original author(s) and the copyright owner(s) are credited and that the original publication in this journal is cited, in accordance with accepted academic practice. No use, distribution or reproduction is permitted which does not comply with these terms.
*Correspondence: Alma L. Guerrero-Barrera, YWxndWVycmVAY29ycmVvLnVhYS5teA==