- 1Behavioural Ecology Group, Wageningen University, Wageningen, Netherlands
- 2Adaptation Physiology Group, Wageningen University, Wageningen, Netherlands
- 3Commonwealth Scientific and Industrial Research Organisation (CSIRO), Armidale, NSW, Australia
High fearfulness could disrupt learning and likely affects judgment in animals, especially when it is part of an animals’ personality, i.e., trait anxiety. Here, we tested whether high fearfulness affects discrimination learning and judgment bias (JB) in laying hens. Based on the response to an open field at 5 weeks of age, birds were categorized as fearful (FC) by showing no walking or vocalizing or non-fearful (NFC) by showing walking and vocalizing. At adult age, birds (n = 24) were trained in a go–go task to discriminate two cues (white or black) with a small or large reward. Birds that reached training criteria were exposed to three unrewarded ambiguous cues (25, 50, and 75% black) to assess JB. Task acquisition took longer for FC birds than for NFC birds, due to a left side bias, and more sessions were needed to unlearn this side bias. Changes in trial setup increased response latencies for FC birds but not for NFC birds. A larger number of FC birds than NFC birds chose optimistically in the last ambiguous trial (25% black). FC birds had a longer latency to choose in the ambiguous trial (75% black) compared to NFC birds. Prior choice in ambiguous trials and a preceding large or small trial affected latencies and choices for both types of birds. Our study showed that fearfulness was associated with differences in discrimination learning ability and JB. It appeared that FC birds used a rigid response strategy during early learning phases by choosing a specific side repeatedly irrespective of success. FC birds were more affected by changes in the setup of the trials in comparison to NFC birds. We speculate that FC birds are more sensitive to changes in environmental cues and reward expectancy. These factors could explain how high fearfulness affects learning.
Introduction
Discrimination tasks are well-used cognitive tasks to assess learning in humans and animals (1–4). In these tasks, cues are accompanied by different types of outcomes (reward, punishment, or social stimulus) (5). The level of acquisition of these associations is measured by the number of correct runs and the number of trials to reach a training criterion. These measurements are interpreted as a subject’s cognitive performance to associate in a specific task (5). Cognition entails the ability to process and acquire new information and learn associations and to store this information, memorize it, and use it in future decision-making (6). A subject’s fearfulness may influence its learning style and strategy. As a trait, fearfulness identifies a feature of an animal’s personality (7) reflecting how an animal interacts with its social and physical environment (8, 9) making fearfulness likely to play a role in cognition and judgment. By having a highly fearful predisposition, i.e., a tendency to develop anxiety, animals may give more attention to certain cues over others (10), thereby being biased to negative or threatening cues (11). Trait anxiety can lead to selective information-processing (12), disrupted motivation (13), and attention, memory, and judgment bias (JB) (11, 14). JB has been shown to play a large role in an animal’s expectation of a certain outcome (15). JB refers to interpreting an ambiguous cue to predict as having a positive or negative outcome, thereby reflecting pessimistic or optimistic tendencies (16), which can be affected by anxiety (17). Highly anxious humans, for example, interpret ambiguous stimuli as threatening compared to humans with low anxiety (18, 19). Likewise, young laying hen chicks with high anxiety are more inhibited to approach an ambiguous stimulus compared to chicks with low anxiety (20). It is unknown whether JB exists in adult chickens with a fearful predisposition. Earlier, we found that in adult laying hens, low learning success correlated with high fear and stress levels (21). It is unknown whether differences in learning relate to predisposed high fearfulness assessed at a young age.
Here, we aimed to answer the question, whether having a fearful predisposition affects discrimination learning and JB in laying hens. To answer this question we assessed responses of laying hen chicks for 5 min in a novel open field (OF) test at 5 weeks of age—a validated measurement for anxiety in many species (22) including chickens (23). In young chicks, short exposure (minutes) to the OF test facilitates behavior indicative for anxiety tendencies while long exposure (hours) reflects the tendency for a more depressive-like state (23). The OF has been validated with anxiolytics. For example, anxiety-induced suppression of activity in the OF is abolished after treatment with an anxiolytic (24), which also affects JB (25). When isolated, young chicks (up to ~10 days of age) aim to social reinstate by high-pitched vocalizations (distress or alarm calls) (26). When slightly older (i.e., 4–5 weeks of age) chicks respond to threatening situations, like exposure to a novel arena or stimuli, by becoming passive to reduce detection by a predator or highly active seeking to escape and social reinstate. Passive responses/low levels of activity in the OF indicate high fear (27) and are generally interpreted as reflecting predisposed high anxiety (20). Activity in the OF in laying hen chicks of 4–5 weeks of age is regulated by 10 quantitative trait loci in chickens, identical to OF behavior of mice and human mental disorders associated with anxiety (28). Our chicks which responded passively to exposure in the OF at 5 weeks of age also tended to have longer duration of tonic immobility (TI) at an adult age (29). These studies support that a low level of activity in the OF when young reflects a fearful predisposition of an animal. Here, we assessed differences of birds characterized as fearful or non-fearful in cognitive performance and JB in a two-choice discrimination task at adult age. We also assessed if our characterization of fearfulness is reflected by their response to a novel environment/social isolation test at 1 week of age, as executed earlier in chickens to assess separation anxiety (30).
Materials and Methods
This experiment was approved by the Animal Care and Use Committee of Wageningen University & Research (the Netherlands) in accordance with Dutch legislation on the treatment of experimental animals the ETS123 (Council of Europe 1985) and the 86/609/EEC Directive. This experiment was conducted from October 2012 until July 2013. 217 chicks were tested for FC and NFC characterization for a larger experiment (29). From these, we retained 24 birds for this analysis based on most contrasting OF behavior and without feather damage so as to assure that birds were not fearful as a consequence of being feather pecked.
Housing Conditions from 1 to 5 Weeks of Age
White leghorn laying hybrid chicks (Dekalb White) arrived from the hatchery at 1 day of age. Chicks were housed in a communal pen until 5 weeks of age. The communal pen measured 8 m × 8 m with wire walls of 2.5 m height. Chicks had ad libitum access to mashed food (commercial pullet starter 1 diet) from five rectangular feeders and water from three round water towers in addition to 25 water nipples which were supplied evenly throughout the pen. Artificial fluorescent light was provided. In the first week of life, light was on for 4 h followed by a 4 h dark period. From day 8 of age onward light was provided for 8 h per day, where at the end of every week 1 h was added until 15 h light per day were reached. This setting was maintained throughout the experiment. Temperature was gradually decreased from 33°C by lowering the temperature by 1°C weekly until 19°C was reached at 10 weeks of age. Pens were supplied with wood shavings on the floor and from day 21 of age wooden rectangular perches were provided. At 1 week of age chicks were weighed individually and marked with a plastic label through the skin of the neck which had a specific number/color combination to enable bird ID.
Housing Conditions from 5 Weeks of Age Onward
From 5 weeks of age onward, chicks were housed in groups of eight. Pens (2 m × 1 m) consisted of a floor area with wood shavings, an elevated wooden perch (at 50 cm height), a rectangular feeding trough [50 cm (l)] at 15 cm height on one side of the pen, and a drinking line with five water nipples at 20 cm height on the other side of the pen. Birds had ad libitum access to pelleted food (commercial layer diet from Agruniek Rijnvallei, the Netherlands) and water and received scattered grain and straw on the floor each day around 8:00 a.m. The inner walls between pens had a cardboard cover of 50 cm high to prevent visual contact with birds from adjacent pens.
Behavioral Observations
Novel Environment Test
At 1 week of age, chicks were individually subjected to a novel environment/social isolation test (NET). We chose to incorporate this test, so as to assess repeatability of responses in a similar kind of anxiety test as the OF. Chicks were placed in a white plastic bucket with a diameter of 40 cm and of 50 cm height. Latency to walk, latency to vocalize, and number of vocalizations (distress calls) were recorded. Behavior was scored for 60 s by direct observation via a video camera by an observer out-of-sight of the test subject.
Open Field Test
At 5 weeks of age, chicks were individually subjected to an open field (OF) test (Figure 1). The OF was a wooden square construction of 125 cm × 125 cm × 125 cm with three black walls, one transparent wall through which video recordings were obtained, and a black floor with white tape to separate four evenly distributed squares of the floor surface and a wire mesh on top of the OF. A 90-W light bulb was placed 50 cm above the OF, in addition to the fluorescent light of the room, which ensured an equal distribution of light (±25 LUX) over the test apparatus (measured with a Voltcraft MS-1300 LUX device, Conrad, Oldenzaal, the Netherlands). Chicks were randomly chosen and individually caught from the communal pen and brought to the testing room in a cardboard box. In the testing room, a chick was placed in the middle of the OF which was kept dark until the start of the test. Behavior was scored for 5 min by one observer from live video recordings (tunnel security camera with external recorder) in an adjacent room using Observer XT 7.0.2 (Noldus, Wageningen, the Netherlands). The latency to walk, the latency to vocalize, and the number of vocalizations were recorded. For the characterization we focused on latency to walk and/or vocalize to characterize chicks. A fearful chicken (FC) was characterized by a latency longer than 300 s to walk and vocalize, reflecting a freezing non-vocalizing chicken. A non-fearful bird (NFC) was characterized by vocalizing before 30 s and walking before 200 s to create a stringent contrast with FC birds. From the total population we chose the 24 most extreme cases representing FC and NFC birds.
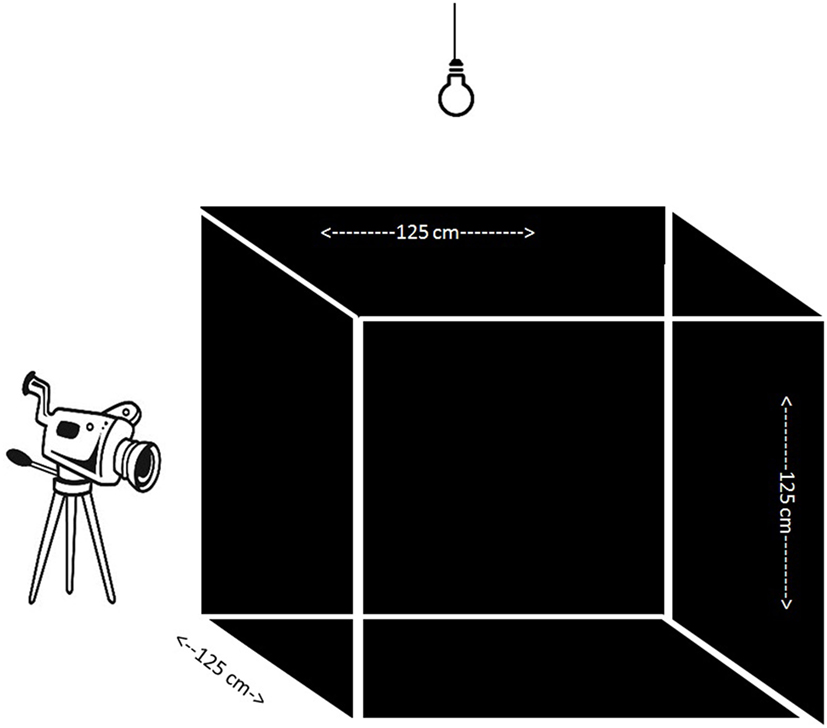
Figure 1. Open field test apparatus containing three black walls and one transparent through which camera recording took place. An extra light bulb enabled evenly distributed light in the apparatus.
Go–Go Two Choice Visual Discrimination Task
From 35–42 weeks of age, 24 hens were trained in a go–go discrimination task. Hens were required to make a choice whereby we could assess their discrimination abilities. Here, they had to learn to associate two different combinations of cue types associated with a different food reward size. As a form of Pavlovian conditioning (5), hens thus needed to learn the association between two background colors and two symbols. This test was designed by Hernandez et al. (31), adapted for chickens based on starling research by Bateson and Matheson (32). Each training week was defined by four consecutive days of training. A training day consisted of 10 consecutive trials per bird. A maximum of 28 training days were performed. On training day 29 a JB test was executed. The training and testing took place in a sound-dampened test room, by live observations supported by camera recordings. Two trainers executed the training. One trainer always handled the birds and observed the behavior while the other trainer always handled the apparatus and cue cards.
Test Apparatus
A wooden test apparatus with a semitriangular shape was used [identical to Ref. (21)]. Walls were of 50 cm height. The floor had a black surface (l × w = 200 cm × 120 cm). The apparatus contained a separate start box (l × w = 60 cm × 25 cm) with a wooden guillotine door. At the opposite of the start box, two feeders (8 cm × 12 cm × 15 cm) were exposed through slots (Figure 2). On top of the feeders, metal lids (l × w = 11.8 cm × 26 cm) with plasticized cues (see below) were presented. Lids could be removed separately from the feeders providing birds access to the food rewards. Ten cm above the feeders, through a narrow slot (l × w = 1 cm × 17 cm), rectangular metal cue cards with plasticized cues (l × w = 11 cm × 16 cm) were presented on which the same cue was shown as on the lid of the corresponding feeder. Visual markings (with gray scotch tape) around the feeders on the floor (l × w = 40 cm × 50 cm) indicated decision lines. A choice for one of the feeders was recorded when both legs and feet of the bird crossed a decision line.
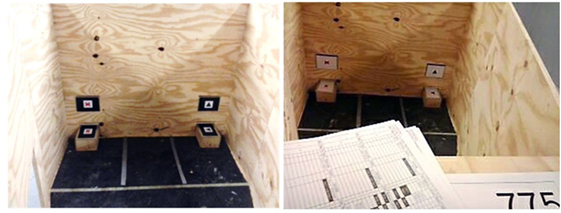
Figure 2. Inside the test apparatus. Cues are represented on feeder lids and metal cue cards above feeders. Decision lines are represented by gray scotch tape markings on the floor of the apparatus. White cue cards indicate small reward trial, and black cue cards indicate large reward trials. Papers represent location from which observations took place and number is hen id.
Cues
Birds needed to learn under which symbol (green Δ or red X) a food reward could be found. Symbols were shown either on a black or on a white background (Figure 3A). Background color indicated size of food reward; five mealworms were associated with black (RGB 248 0% transparency) and one mealworm was associated with white (RGB 248 95% transparency). Trials always contained one background color (i.e., L trials = large reward: black background; S trials = small reward: white background), which was fixed for all birds. The training of large and small rewards was to allow the birds to associate one symbol with reward and the other with no reward in a “small reward situation” (white background) and then the symbols had the opposite meaning for the large rewards (black background).
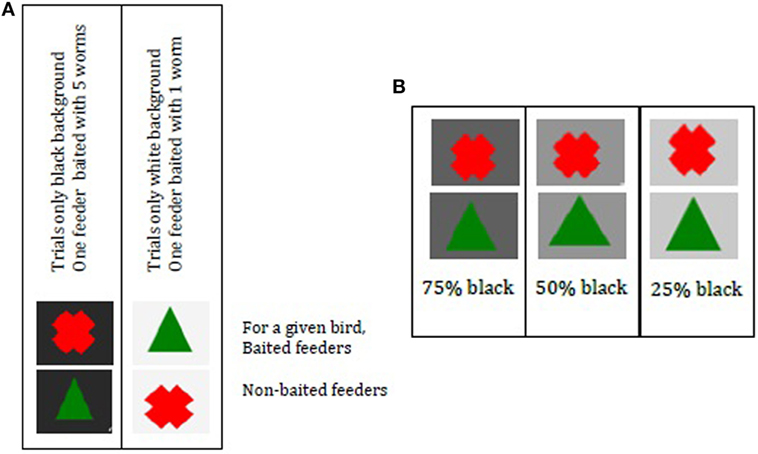
Figure 3. (A) Cue cards to indicate reward size and symbols to indicate baited feeder. Per trial one color was used where one feeder was baited. The background color indicated reward size, black indicated the possibility to find five worms, and white indicated the possibility to find one worm. (B) Cue cards with ambiguous background color 75, 50, and 25% black. Per trial, two feeders were present with only one background color. Choice for a symbol was associated with predicting a large or small reward (A). Ambiguous trials were unrewarded. Note: if a bird would have a reward under cross black and triangle white [as in (A)], her choice for cross under gray would represent an optimistic bias. Her choice for triangle would represent a pessimistic bias.
One feeder was baited per trial. For a given bird, black + red X was five worms, while black + green Δ had no reward. For that same bird, white + green Δ was one worm and white + red X had no worm (see Figure 3A). Reward–cue combinations were balanced across FC and NFC.
Birds were exposed to ambiguous cues in the JB test at day 29. These had gray-toned backgrounds, 25, 50, or 75% black (RGB) [Figure 3B; adapted from Ref. (31)]. If birds were trained that the black background yielded a large reward when the green Δ is chosen, then the white background yielded a small reward when the red X was chosen and vice versa. Consequently, a choice for the green Δ during the ambiguous trials indicated that the bird was interpreting the gray background to be more similar to black than white—an “optimistic” response anticipating the larger reward.
Training
The training period consisted of four training phases: phase 1: acquisition; phase 2: S trials; phase 3: one L trial; and phase 4: consolidation of L + S cues (three L trials). Training was identical as described by Ref. (21, 31) with the exception of phase 2. Furthermore, the training criteria were set to 80% correct choices over two consecutive days. See Table 1 for details of training setup and sample sizes per period.
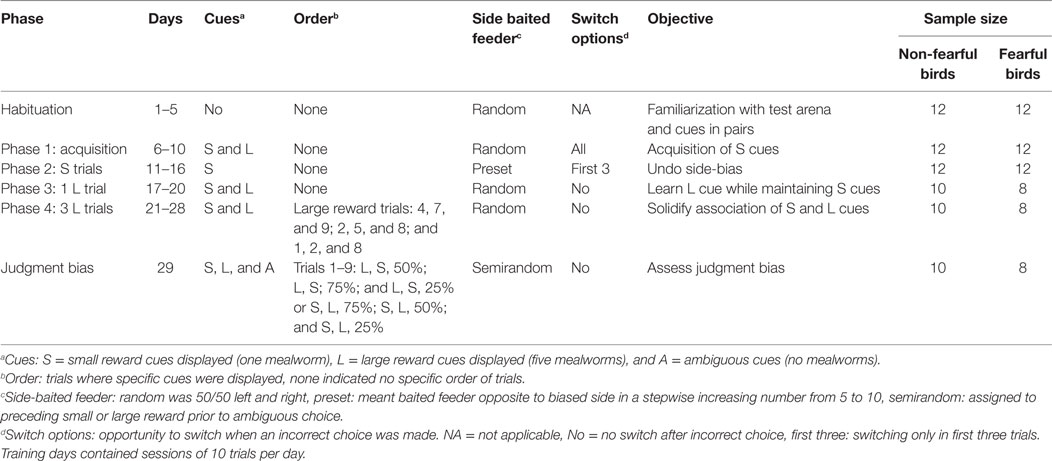
Table 1. Description of training phases for judgment bias in fearful and non-fearful characterized chickens.
Habituation
Birds were habituated in their home pen to feeders and mealworms on days 1 and 2. We recorded whether birds ate from the feeders in the home pen. From day 3 onward, birds were habituated in the test apparatus in pairs of similar characterization from the same pen (both FC and NFC). This was conducted to limit isolation stress for the test subject. On day 3, hens had access to the whole apparatus with open feeders filled with worms. We recorded whether birds ate from the feeders in the test apparatus. On day 4, birds started from the start box with the guillotine door open. Once the worms were consumed from the feeders birds were caught and placed in the start box again, and a consecutive trial was executed with refilled feeders. This setup was repeated on days 5 and 6.
Phase 1: Acquisition Phase
From day 7 onward data collection took place on an individual level. Cue cards on lids and walls were both present. The acquisition phase contained four sessions with five S and five L trials. The location of the baited feeder was always randomized for both S and L trials. This setup was used as we wanted birds to focus on the combination of background colour and symbol rather than on location of the baited feeder. Birds were allowed to switch between feeders following an initial incorrect choice.
Phase 2: Adapted Phase
During phase 2 only S trials were given. In phase 1, we noticed that 70% of the birds repeatedly chose the feeder on one side irrespective of finding a reward (i.e. more than 7 out of 10 trials). We calculated birds’ side choices when no reward was obtained by subtracting the number of side choices from the number of rewards given on that particular side. A more negative number indicated a side bias (i.e., going to left but not finding a reward). In our previous study (21), side bias severely hampered learning; therefore, birds with a side bias were subjected to an adapted training scheme. We chose to adapt the training so as to increase the number of individuals learning the task. From day 11 till 16 the rewarded feeder was opposite to the preferred side. Birds that did not develop a side bias remained on a randomized scheme with S trials. We increased the number of times the baited feeder was on the non-preferred side, stepwise from 5 to 10 trials per session. Birds could switch between feeders in the first three trials, hereafter wrong choices remained unrewarded. The criterion to move to the next phase was 80% correct S trials on randomized choices given on two consecutive days (criterion phase 3).
Phase 3: 1 Large Reward Trial Phase
On day 17 implementation of one L trial was initiated. Four sessions containing one L trial and nine S trials were used. We chose one L trial to slowly increase the complexity of the test, aiming to maintain learning performance for most birds. The criterion to move to the next phase was 80% accuracy to S trials and 100% to the L trial on two consecutive days (criterion phase 4).
Phase 4: Consolidation Phase
On days 21–28, birds were subjected to seven S and three L trials per session. These sessions had predefined L trials, similar for all birds, in the following recurring order: trials 4, 7, and 9; trials 2, 5, and 8; and trials 1, 2, and 8. We chose to use three L trials vs. seven S trials to maintain the motivation of birds for the S trials and not create omission in S trials because of preference for the large reward. We recorded a shorter latency to choose in L trials than in S trials (data not shown). The criterion for the JB test (criterion JB test) was reached if birds achieved a learning score of 80% of both L and S trials on two consecutive days.
JB Task
The JB test consisted of nine trials. In three of those trials ambiguous cues were presented of different % of RGB: 25, 50, and 75% black background (see Figure 3B). The test also included three L trials with black background and three S trials with white background. When a hen approached the symbol associated with the large reward (black), her choice would be interpreted as optimistic. When a hen approached the symbol associated with the small reward (white), her choice would be interpreted as pessimistic (33). The trial preceding the ambiguous trial can affect latency to choose (31). Therefore, two trial sequences were used, preceding small trials (L, S, 50% gray; L, S, 75% gray; and L, S, 25% gray) or preceding large trials (S, L, 75% gray; S, L, 50% gray; and S, L, 25% gray). We chose to use unrewarded ambiguous cues and expose birds once to each of these cues once. Rewarded or unrewarded ambiguous trials can affect consecutive choices in ambiguous trials (14). However, we wanted to compare with earlier studies with the same setup (21, 31).
Statistical Analysis
Data were analyzed by SAS 9.3 (SAS, Cary, NC, USA). Variables were checked for normal distribution of residuals based on a general linear model (GLM) with a fixed effect of characterization (FC or NFC) and the error term of characterization nested within pen to correct for animals from the same pen. Because FC birds all had the maximum latency to walk and vocalize in the OF we could not conduct a linear regression analysis. Therefore, instead, models with a fixed effect of characterization were applied. The GLM was used to test the effect of characterization on the latency to walk in the NET, number of sessions to obtain the different training criteria, and latency to choose in the JB test. Latencies to choose on days 17 (one L) and 21 (three L trials) were tested with a t-test for group comparison. Spearman correlations were calculated between latency to walk in the NET and OF. Repeated measurements of latency to choose, learning score (representing the percentage of correct choices based on calculation of errors), and side bias (representing the number of choices to a particular side corrected for baited side) were analyzed with a MIXED model. Fixed effects were characterization, with the repeated effect of time and the interaction between characterization and time. The random effect included pen. The model was run by phase to check for differences in performance between phases. A binominal logit link GENMOD model tested effects of characterization on binominal variables: number of birds that developed side bias (yes/no), number of birds that met the training criteria (yes/no), and number of birds that chose optimistically in ambiguous trials (yes/no). To test if choice in the last ambiguous trial (25% black) was affected by choice in the preceding ambiguous trials we added this to the GENMOD model as a fixed factor. In the JB test, we tested if having an L or S trial prior to the ambiguous trial affected the latency to choose by adding preceding L or S trial as a fixed effect in the GLM. Data are expressed as mean ± SEM with P smaller than 0.05 classified as statistically significant.
Results
Behavioral Response to the Fear Tests
In the NET at 1 week of age, FC birds tended to have a longer latency to walk compared to NFC birds (56.8 ± 2.2 vs. 45.2 ± 5.0 s, F1,21 = 3.83, P = 0.06). No differences in vocalization responses between NFC and FC birds were found in the NET (latency: 13 ± 4 vs. 10.7 ± 5 s, F1,21 = 0.09, P = 0.76 and vocalizations: 37.1 ± 9 vs. 55.4 ± 8 s, F1,21 = 1.09, P = 0.17). In the OF at 5 weeks of age, none of the FC birds walked or vocalised within the test duration of 300 s. NFC birds had an average latency to walk of 134.5 ± 12.8 s (max: 147 s, mean: 103 s, and min: 4 s) and average latency to vocalize of 20.9 ± 6.3 s (max: 71.4 s, mean: 10.5, and min: 0.4 s). Latency to walk in the NET and OF was positively correlated (r = 0.73, P = 0.05). Latency to vocalize or vocalizations in the NET and OF were not correlated, also not with walking latency (for all combinations: r > 0.15, P > 0.35).
Training Sessions to Criteria
Eighteen out of 24 birds learned the task, 10 NFC birds (80%) and 8 FC birds (70%) (see Table 2). FC birds needed more sessions to reach the criterion of phase 3 (16.1 ± 1.2 vs. 12.8 ± 1.0, F1,20 = 5.27, P = 0.03) and the criterion of phase 4 (19 ± 1.2 vs. 16.2 ± 1.0, F1,18 = 6.10, P = 0.03). No significant difference existed in the number of sessions to reach the final criterion between FC and NFC birds (25.5 + 1.1 vs. 23.2 + 1.3, F1,18 = 2.23, P = 0.15). The number of sessions needed to unlearn side biases tended to be higher for FC birds than for NFC birds (5.7 + 0.3 vs. 3.14 + 1.0, F1,18 = 4.21, P = 0.06). A larger proportion of the FC birds needed adjusted training compared to NFC birds (92 vs. 50%, χ2 = 6.02, P = 0.01).
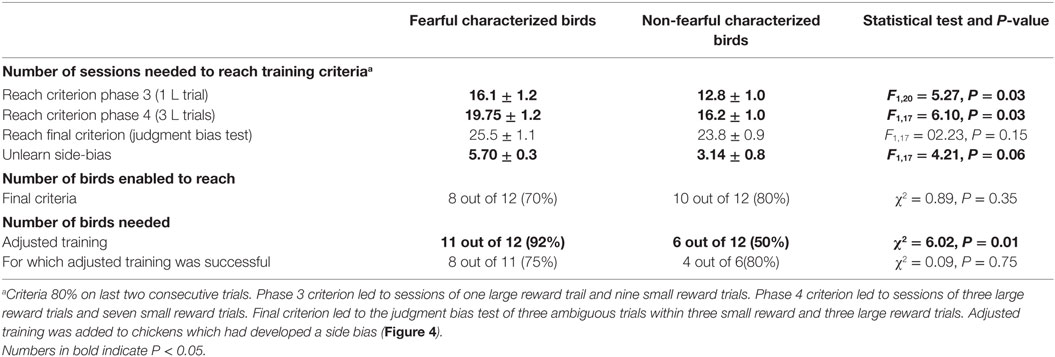
Table 2. The number of fearful and non-fearful characterized birds able to reach criteria in a visual association task.
Learning Performance, Side Choices, and Side Bias
Learning performance fluctuated but increased over time, irrespective of characterization (day: F22,424 = 3.92, P < 0.0001; day × characterization, F22,575 = 0.46, P = 0.93, Figure 4). But, FC birds consistently had a lower learning performance than NFC birds (F1,575 = 14.03, P < 0.01; Figure 4). Specifically, FC birds were significantly worse than NFC birds during the acquisition phase (phase 1: percentage of correct runs 45.4 ± 2.9 vs. 54.6 ± 2.8%, F1,76 = 4.62, P = 0.03), in the adapted learning phase (phase 2: 51.2 ± 3.5 vs. 62.0 ± 3.2%, F1,188 = 11.79, P = 0.007), and the final learning phase (phase 4: 60.5 ± 2.9 vs. 68.2 ± 2.8%, F1,132 = 5.95, P = 0.02). No differences in learning performance were found in phase 3 (56.8 ± 5.8 vs. 61.8 ± 5.6%, F1,55 = 1.43, P = 0.24). Overall, FC birds had a stronger side bias toward the left side than NFC birds, with significance of P < 0.05 in phases 1 and 2 (Figure 5).
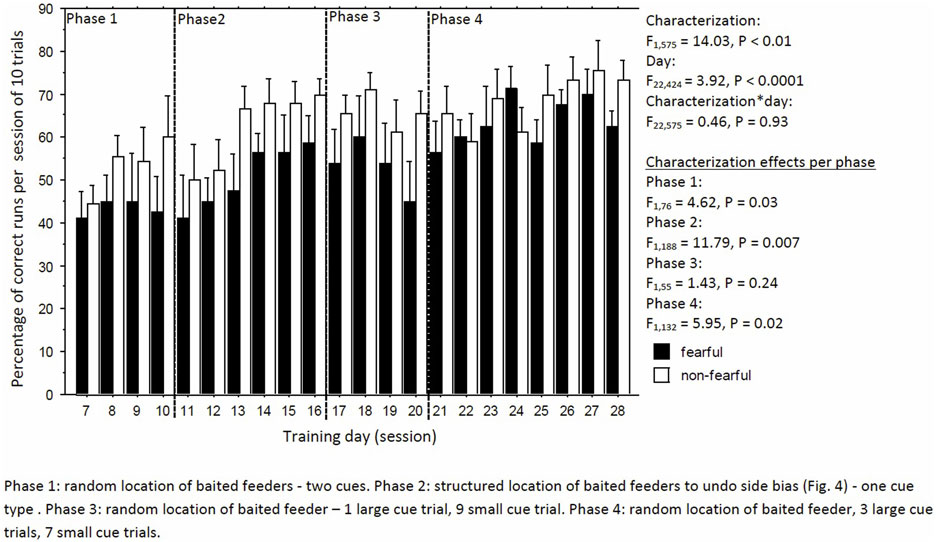
Figure 4. The percentage of correct runs per trial of 10 runs of fearful and non-fearful characterized birds.
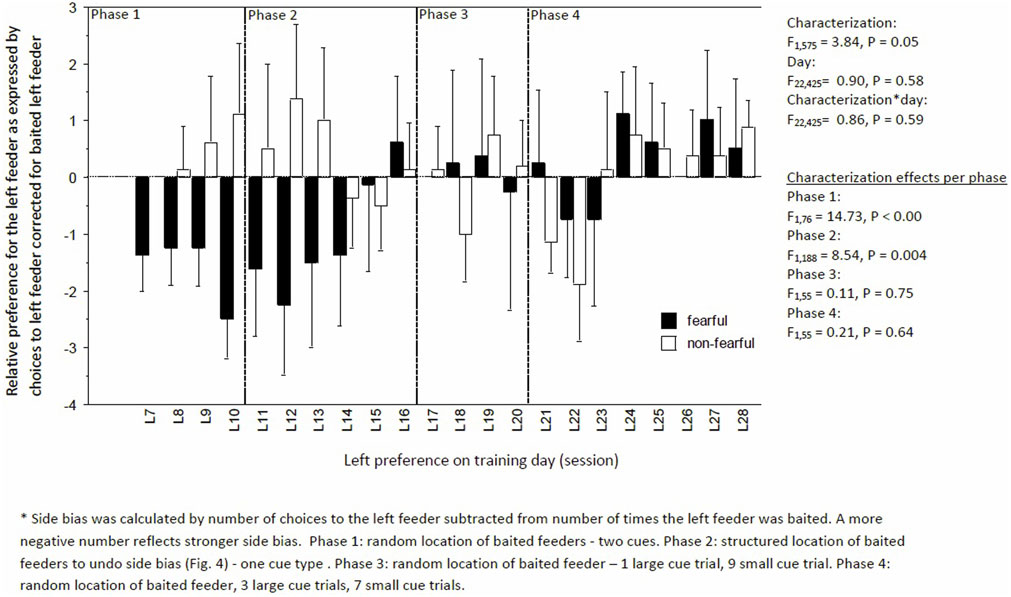
Figure 5. Side bias to the left feeder corrected for the times the feeder was baited of fearful and non-fearful characterized birds. A negative number indicated a stronger bias to the left.
Latency to Make a Choice
The latency to make a choice decreased over sessions for both types of birds except on two specific days where the setup of trials was changed (characterization: F1,424 = 0.18, P = 0.67: day × characterization: F22,424 = 1.82, P = 0.02: Figure 6). On days 17 and 21, latency to choose was affected by characterization. On day 17, introduction of an L trial led to a longer latency to choose in the L trial for FC birds than for NFC birds (t16 = 1.18, P = 0.00). On day 21, introduction of three L trials led to a longer latency to choose in the S trials for FC but not for NFC birds (t16 = 2.38, P = 0.03).
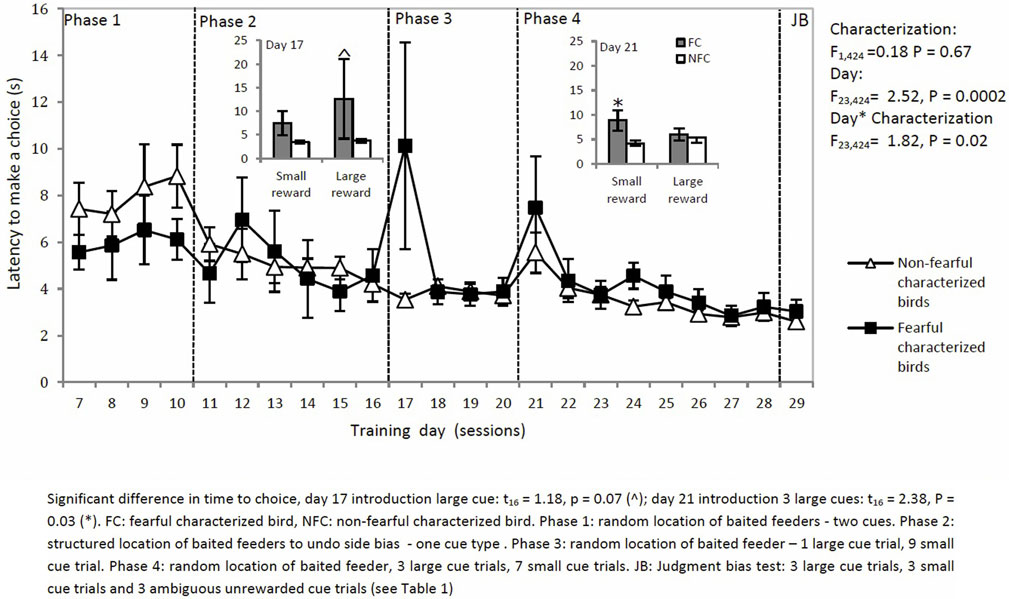
Figure 6. The latency to make a choice in a two-choice discrimination task for fearful and non-fearful characterized birds.
JB Test
Choices in the JB Test
In the JB test, nine trials were given. Per trial birds could choose between a feeder with a triangle or cross. Three trials had a black background on the feeders (five worms to be found under either a triangle or cross), three trials had a white background on the feeders (one worm to be found either under triangle or cross), and three trials had a gray background on the feeder (25, 50, and 75% of black) with no worm to be found under triangle or cross. If in the gray trials a bird chose for a symbol predicting five worms this would indicate optimistic bias. The last gray trial was 25% black. We found most effects on this last ambiguous trial. This was more often chosen as predicting a large reward in FC birds compared to NFC birds (Table 3; Wald χ2 = 4.22, P = 0.04). This indicated that FC birds judged this background to predict a large reward resembling an “optimistic” judgment. The choice in the 25% black ambiguous trial was affected by the choice in the 75% black ambiguous trial (Wald χ2 = 4.33, P = 0.04). If FC birds had chosen for 75% black reflecting the S reward, they chose more often than NFC birds for 25% black reflecting the L reward—this was the opposite for NFC birds (Table 3). The choice of the 50% black or 75% black ambiguous cue was not affected by characterization (χ2 = 0.08, P = 0.77; χ2 = 1.43, P = 0.24, Table 3).
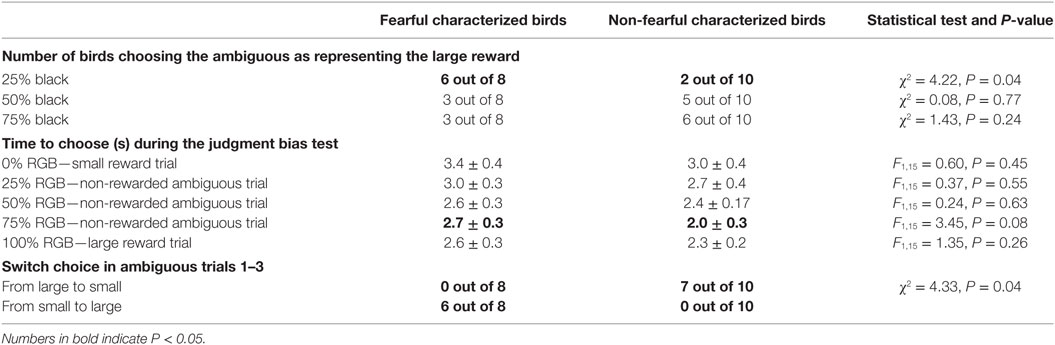
Table 3. Choices and time to choose for a specific feeder in the judgment bias task for fearful and non-fearful characterized birds.
Latency to Choose in the Judgment Bias Test
Latency to choose in the 75% ambiguous trial tended to be longer for FC birds than for NFC birds (3.54 ± 0.48 vs. 2.49 ± 0.22 s, F1,15 = 3.45, P = 0.08). Latency to choose in the S, L and 25 and 50% ambiguous trial was not affected by characterization (Table 3). A preceding L trial increased latency to choose in the 50% ambiguous trial (β 0.8 s: F1,17 = 25.7, P = 0.02).
Discussion
We characterized laying hen chicks at 5 weeks of age as fearful (FC) or non-fearful (NFC) on the basis of their freezing response in the OF. As adults, hens were exposed to a discrimination task with two cue types (white, black) and associated reward sizes; small (one worm) or large (five worms), respectively. Seventy percent of the birds learned the task, but of these 75% needed extensive adjusted training. Compared to NFC birds, FC birds had a lower cognitive performance, needed more trials to reach the training criterion, and needed more sessions of adapted training. Changes in trial setup increased response latencies for FC birds but not for NFC birds. These results indicate that predisposed fearfulness negatively affects learning in chickens.
In support of our characterization based on OF behavior, latency to walk in OF and NET were positively correlated. In both tests, an animal is exposed—post-handling—to a brightly lit novel environment while being in social isolation. Despite the lack of an actual imminent threat, animals which freeze, i.e., not walk, are assumed to perceive this situation as threatening (33). Walking in the OF can also reflect exploration, novelty seeking, escape behavior, and can relate to impulsivity (34). The freezing response seen in FC birds, however, more likely reflects an adaptive mechanism which reduces detection by predators, as a form of passive coping and excessive fear. As mentioned in the introduction, the response of chickens in the OF is repeatable (28) and heritable (35), but in mice the response strength declines due to habituation when repeated multiple times (36). Although the OF was conducted once, our characterization of FC birds was supported by a longer TI in FC birds vs. NFC birds at juvenile and adult age (29) and by a longer latency to walk in the NET. In many species, freezing in the OF is indicative of anxiety (22). Anxiety has been associated with alterations in learning in other species (37, 38) and pessimistic JB in young chicks (20). FC birds had a lower learning performance and needed more trials to learn the task than NFC birds. These results are comparable to those found in chickens which were fearful of a novel object and did not pass the criteria for a JB test (39). However in mice, an increase in proportion of time spent in the middle of the OF correlated positively with more efficient learning of a specific test based on operant approaching, discrimination, and on other cognitive domains (40).
FC birds were less successful in learning and performed more left side choices (i.e., side bias) compared to NFC birds. Hens with high fear and stress levels can develop a side bias as we showed earlier (21). In rats which froze in the OF more side errors were made in a Y-maze task (41) and in a water maze task (38) based on place recognition memory. These rats exhibited a stimulus–response strategy which linked to inherent high trait anxiety (41). Our result on side bias in chickens here and that in Ref. (21) direct to a stimulus–response learning strategy adapted by FC hens. Stimulus–response learning strategies are specific motor actions in a repeating sequence or to always turn left or right to reach a goal (41). These responses “rely on cues that signal a specific sequence toward a goal, or upon a discrete cue proximal to a goal that signals its location” (41). These responses are known to be rigid and less adaptive to changing conditions (42). FC birds also needed more sessions to unlearn their side bias compared to NFC birds. In the adaptive learning phase, we reinforced birds’ incorrect choices so that birds would switch to the other feeder and find a reward. Despite this possibility, many birds continued to make side errors and appeared stuck on a specific side, which we referred to as a side bias. This could reflect a habit. Habits involve a specific sequence of repetitive motor behavior and develop outside of awareness (43, 44). While goal-directed learning is rapidly acquired and regulated by its outcome, habit learning is reflexive, elicited by antecedent stimuli rather than its consequences. Habits can occur over the course of days or years and become remarkably fixed (42). Animals with high anxiety show a bias toward habit learning (42). Consistent findings on side bias in fearful hens, here and previously (21), could indicate that fearful animals are sensitive to form habits.
In general, there were no differences in time to make a choice between FC and NFC birds. FC birds only had a longer latency to choose the L reward within a session when sessions included an L trial for the first time. When established behavior responses to the S cue were no longer valid, FC birds were more affected than NFC birds as shown by a longer latency to choose S rewards. Fearful birds might have an increased sensitivity to environmental changes and respond more strongly to changes in cues. This is supported by the longer latency to choose compared to NFC birds for the 75% gray ambiguous cue—the cue most closely resembling the 100% black cue. Fearful birds may be more sensitive to negative outcome of an incorrect choice, i.e., finding no reward while expecting it. Sensitivity to loss, also known as reward sensitivity, has been suggested as a symptom of high anxiety (45). This could explain our results on JB and learning difficulty in FC birds, but further testing is needed to validate this assumption.
Contrary to our hypothesis, more FC birds showed an optimistic-like response to the 25% ambiguous cue compared to NFC birds. While anxious individuals have been shown to display pessimistic behavior (46, 47), similar counterintuitive results have been found (48–50). We are cautious to generalize our conclusions on JB here. The setup of our JB test, i.e., using unrewarded ambiguous cues, could have influenced choice (14). Furthermore, our data can be skewed toward animals which eventually learned the task (5).
We noticed that many birds switched in the final ambiguous trial—with FC birds from S to L and NFC birds from L to S. Birds’ choices could be affected by ambiguous cues being unrewarded. Finding no reward when expecting it can cause frustration and stress which may enhance learning about ambiguous cues being unrewarded. It has been postulated that stressed animals learn these negative associations faster than non-stressed animals (16). This could facilitate a win-stay/lose-shift or win-shift/lose-stay strategy in future ambiguous trials (51). Furthermore, longer latencies to approach ambiguous cues have been postulated to reflect a loss of ambiguity (14). In support of this suggestion, the choice and latency in the final ambiguous trial (25% black) were related to the choice in the 75% black ambiguous trial. Furthermore, FC birds had a longer latency in the 75% black trial and switched more than NFC birds. Partially or fully rewarding ambiguous trials and using a single ambiguous cue could be a way to overcome these potential confounding effects (14). JB can be used to assess emotional valence of birds creating more understanding of what it means to have a fearful predisposition and how it can affect an animal’s welfare.
Our study shows that FC chickens have more difficulty in combining sources of information (cue color, reward size, and location) than NFC chickens. We showed that FC chickens tended to use and stick to a stimulus–response strategy more strongly than NFC chickens. Our results suggest that differences in learning can derive from an animal’s fearful predisposition.
Ethics Statement
This experiment was approved by the Animal Care and Use Committee of Wageningen University & Research (the Netherlands) in accordance with Dutch legislation on the treatment of experimental animals the ETS123 (Council of Europe 1985) and the 86/609/EEC Directive.
Author Contributions
EH: design experiment, conduct experiment, data analysis, and writing. CL and BR: help with experiment and writing.
Conflict of Interest Statement
The authors declare that the research was conducted in the absence of any commercial or financial relationships that could be construed as a potential conflict of interest.
Acknowledgments
The animal caretakers of the Animal Research Facility (CARUS: care for animals in research and science) are extensively thanked for their help in this experiment. Technicians, Monique Seur Ooms and Fleur Bartels (Adaptation Physiology Group), and Lydia Nieuwe Weme (Behavior Ecology Group) of Wageningen University are acknowledged for their contributions. Dr Carlos Hernandez is thanked for sharing his experience with this test setup. Andrea Welsink, MSc student of the Wageningen University (the Netherlands), is acknowledged for her contribution to this study. This experiment was part of the postdoctoral project of Dr. Elske N. de Haas, financially supported by the Dr. J. L. Dobberke Foundation for Comparative Psychology.
Funding
This experiment was part of the postdoctoral project of Dr. Elske N. de Haas, financially supported by the Dr. J. L. Dobberke Foundation for Comparative Psychology.
Abbreviations
FC, fearful characterized; NFC, non-fearful characterized; L, large reward trails; S, small reward trails; NET, novel environment test; OF, open field test; TI, tonic immobility.
References
1. Verschure P, Pennartz CMA, Pezzulo G. The why, what, where, when and how of goal-directed choice: neuronal and computational principles. Philos Trans R Soc Lond B Biol Sci (2014) 369(1655):14. doi:10.1098/rstb.2013.0483
2. Dyer AG, Chittka L. Bumblebees (Bombus terrestris) sacrifice foraging speed to solve difficult colour discrimination tasks. J Comp Physiol A Neuroethol Sens Neural Behav Physiol (2004) 190(9):759–63. doi:10.1007/s00359-004-0547-y
3. Tahamtani FM, Nordgreen J, Nordquist RE, Janczak AM. Early life in a barren environment adversely affects spatial cognition in laying hens (Gallus gallus domesticus). Front Vet Sci (2015) 2:3. doi:10.3389/fvets.2015.00003
4. Albiach-Serrano A, Bräuer J, Cacchione T, Zickert N, Amici F. The effect of domestication and ontogeny in swine cognition (Sus scrofa scrofa and S. s. domestica). Appl Anim Behav Sci (2012) 141(1–2):25–35. doi:10.1016/j.applanim.2012.07.005
5. Griffin AS, Guillette LM, Healy SD. Cognition and personality: an analysis of an emerging field. Trends Ecol Evol (2015) 30(4):207–14. doi:10.1016/j.tree.2015.01.012
6. Rowe C, Healy SD. Measuring variation in cognition. Behav Ecol (2014) 25(6):1287–92. doi:10.1093/beheco/aru090
7. Andreatini R, Bacellar LFS. Animal models: trait or state measure? The test-retest reliability of the elevated plus-maze and behavioral despair. Prog Neuropsychopharmacol Biol Psychiatry (2000) 24(4):549–60. doi:10.1016/S0278-5846(00)00092-0
8. Kalueff AV, Keisala T, Minasyan A, Tuohimaa P. Influence of paternal genotypes on F1 behaviors: lessons from several mouse strains. Behav Brain Res (2007) 177(1):45–50. doi:10.1016/j.bbr.2006.11.005
9. Lister RG. Ethologically-based animal-models of anxiety disorders. Pharmacol Ther (1990) 46(3):321–40. doi:10.1016/0163-7258(90)90021-S
10. McNaughton N. Cognitive dysfunction resulting from hippocampal hyperactivity – a possible cause of anxiety disorder? Pharmacol Biochem Behav (1997) 56(4):603–11. doi:10.1016/S0091-3057(96)00419-4
11. Bar-Haim Y, Lamy D, Pergamin L, Bakermans-Kranenburg MJ, van IJzendoorn MH. Threat-related attentional bias in anxious and nonanxious individuals: a meta-analytic study. Psychol Bull (2007) 133(1):1–24. doi:10.1037/0033-2909.133.1.1
12. Beck JG, Stanley MA, Averill PM, Baldwin LE, Deagle EA III. Attention and memory for threat in panic disorder. Behav Res Ther (1992) 30(6):619–29. doi:10.1016/0005-7967(92)90007-4
14. Roelofs S, Boleij H, Nordquist RE, van der Staay FJ. Making decisions under ambiguity: judgment bias tasks for assessing emotional state in animals. Front Behav Neurosci (2016) 10:119. doi:10.3389/fnbeh.2016.00119
15. Paul ES, Harding EJ, Mendl M. Measuring emotional processes in animals: the utility of a cognitive approach. Neurosci Biobehav Rev (2005) 29(3):469–91. doi:10.1016/j.neubiorev.2005.01.002
16. Mendl M, Burman OHP, Parker RMA, Paul ES. Cognitive bias as an indicator of animal emotion and welfare: emerging evidence and underlying mechanisms. Appl Anim Behav Sci (2009) 118(3–4):161–81. doi:10.1016/j.applanim.2009.02.023
17. Carreras R, Arroyo L, Mainau E, Peña R, Bassols A, Dalmau A, et al. Effect of gender and halothane genotype on cognitive bias and its relationship with fear in pigs. Appl Anim Behav Sci (2016) 177:12–8. doi:10.1016/j.applanim.2016.01.019
18. Liebman SE, Allen GJ. Anxiety sensitivity, state anxiety and perceptions of facial emotions. J Anxiety Disord (1995) 9(4):257–67. doi:10.1016/0887-6185(95)00007-B
19. Lilley PR, Cobham VE. Interaction of anxiety sensitivity and induced physiological state: effect on behaviour and interpretation of ambiguous scenarios. Aust J Psychol (2005) 57(2):77–86. doi:10.1080/00049530412331283390
20. Salmeto AL, Hymel KA, Carpenter EC, Brilot BO, Bateson M, Sufka KJ. Cognitive bias in the chick anxiety-depression model. Brain Res (2011) 1373:124–30. doi:10.1016/j.brainres.2010.12.007
21. de Haas EN, Lee C, Hernandez CE, Naguib M, Rodenburg TB. Individual differences in personality in laying hens are related to learning a colour cue association. Behav Processes (2017) 134:37–42. doi:10.1016/j.beproc.2016.11.001
22. Forkman B, Boissy A, Meunier-Salaün MC, Canali E, Jones RB. A critical review of fear tests used on cattle, pigs, sheep, poultry and horses. Physiol Behav (2007) 92(3):340–74. doi:10.1016/j.physbeh.2007.03.016
23. Sufka KJ, Feltenstein MW, Warnick JE, Acevedo EO, Webb HE, Cartwright CM. Modeling the anxiety-depression continuum hypothesis in domestic fowl chicks. Behav Pharmacol (2006) 17(8):681–9. doi:10.1097/FBP.0b013e3280115fac
24. Jain A, Dvorkin A, Fonio E, Golani I, Gross CT. Validation of the dimensionality emergence assay for the measurement of innate anxiety in laboratory mice. Eur Neuropsychopharmacol (2012) 22(2):153–63. doi:10.1016/j.euroneuro.2011.07.001
25. Destrez A, Deiss V, Belzung C, Lee C, Boissy A. Does reduction of fearfulness tend to reduce pessimistic-like judgment in lambs? Appl Anim Behav Sci (2012) 139(3–4):233–41. doi:10.1016/j.applanim.2012.04.006
26. Rogers LJ. The Development of Brain and Behaviour in the Chicken. Wallingford, United Kingdom: CAB International (1995). 273 p.
28. Johnsson M, Williams MJ, Jensen P, Wright D. Genetical genomics of behavior: a novel chicken genomic model for anxiety behavior. Genetics (2016) 202(1):327. doi:10.1534/genetics.115.179010
29. De Haas EN, Bolhuis JE, Henskens M, Grimberg C, Rodenburg TB. Time budget, social interactions, and fearfulness of laying hens characterized as fearful and non-fearful in groups with different compositions. Proceedings of the Benelux ISAE Conference 2013. Sterksel, The Netherlands: (2013).
30. de Haas EN, Bolhuis JE, Kemp B, Groothuis TG, Rodenburg TB. Parents and early life environment affect behavioral development of laying hen chickens. PLoS One (2014) 9(3):e90577. doi:10.1371/journal.pone.0090577
31. Hernandez CE, Hinch GN, Lea J, Ferguson D, Lee C. Acute stress enhances sensitivity to a highly attractive food reward without affecting judgement bias in laying hens. Appl Anim Behav Sci (2015) 163:135–43. doi:10.1016/j.applanim.2014.12.002
32. Bateson M, Matheson SM. Performance on a categorisation task suggests that removal of environmental enrichment induces ’pessimism’ in captive European starlings (Sturnus vulgaris). Anim Welf (2007) 16:33–6.
33. Faure JM, Jones RB, Bessei W. Fear and social motivation as factors in open-field behaviour of the domestic chick. Biol Behav (1983) 8:103–16.
34. Kembro JM, Satterlee DG, Schmidt JB, Perillo MA, Marin RH. Open-field temporal pattern of ambulation in Japanese quail genetically selected for contrasting adrenocortical responsiveness to brief manual restraint. Poult Sci (2008) 87(11):2186–95. doi:10.3382/ps.2008-00108
35. Rodenburg TB, et al. Heritability of feather pecking and open-field response in laying hens at two different ages. Poult Sci (2003) 82:861–7. doi:10.1093/ps/82.6.861
36. Olsen RH, Johnson LA, Zuloaga DG, Limoli CL, Raber J. Enhanced hippocampus-dependent memory and reduced anxiety in mice over-expressing human catalase in mitochondria. J Neurochem (2013) 125(2):303–13. doi:10.1111/jnc.12187
37. Green MR, McCormick CM. Effects of stressors in adolescence on learning and memory in rodent models. Horm Behav (2013) 64(2):364–79. doi:10.1016/j.yhbeh.2012.09.012
38. Grissom EM, Hawley WR, Bromley-Dulfano SS, Marino SE, Stathopoulos NG, Dohanich GP. Learning strategy is influenced by trait anxiety and early rearing conditions in prepubertal male, but not prepubertal female rats. Neurobiol Learn Mem (2012) 98(2):174–81. doi:10.1016/j.nlm.2012.06.001
39. Wichman A, Keeling LJ, Forkman B. Cognitive bias and anticipatory behaviour of laying hens housed in basic and enriched pens. Appl Anim Behav Sci (2012) 140(1–2):62–9. doi:10.1016/j.applanim.2012.05.006
40. Matzel LD, Han YR, Grossman H, Karnik MS, Patel D, Scott N, et al. Individual differences in the expression of a “general” learning ability in mice. J Neurosci (2003) 23(16):6423–33.
41. Hawley WR, Grissom EM, Dohanich GP. The relationships between trait anxiety, place recognition memory, and learning strategy. Behav Brain Res (2011) 216(2):525–30. doi:10.1016/j.bbr.2010.08.028
42. Gasbarri A, Pompili A, Packard MG, Tomaz C. Habit learning and memory in mammals: behavioral and neural characteristics. Neurobiol Learn Mem (2014) 114:198–208. doi:10.1016/j.nlm.2014.06.010
43. Graybiel AM. Habits, rituals, and the evaluative brain. Annu Rev Neurosci (2008) 31:359–87. doi:10.1146/annurev.neuro.29.051605.112851
44. Graybiel AM, Grafton ST. The striatum: where skills and habits meet. Cold Spring Harb Perspect Biol (2015) 7(8):a021691. doi:10.1101/cshperspect.a021691
45. Slessareva E, Muraven M. Sensitivity to punishment and self-control: the mediating role of emotion. Pers Indiv Dif (2004) 36(2):307–19. doi:10.1016/S0191-8869(03)00087-4
46. Miranda R, Mennin DS. Depression, generalized anxiety disorder, and certainty in pessimistic predictions about the future. Cognit Ther Res (2007) 31(1):71–82. doi:10.1007/s10608-006-9063-4
47. MacLeod AK, Byrne A. Anxiety, depression, and the anticipation of future positive and negative experiences. J Abnorm Psychol (1996) 105(2):286–9. doi:10.1037/0021-843X.105.2.286
48. Verbeek E, Ferguson D, Lee C. Are hungry sheep more pessimistic? The effects of food restriction on cognitive bias and the involvement of ghrelin in its regulation. Physiol Behav (2014) 123(0):67–75. doi:10.1016/j.physbeh.2013.09.017
49. Doyle RE, Lee C, Deiss V, Fisher AD, Hinch GN, Boissy A. Measuring judgement bias and emotional reactivity in sheep following long-term exposure to unpredictable and aversive events. Physiol Behav (2011) 102(5):503–10. doi:10.1016/j.physbeh.2011.01.001
50. Sanger ME, Doyle RE, Hinch GN, Lee C. Sheep exhibit a positive judgement bias and stress-induced hyperthermia following shearing. Appl Anim Behav Sci (2011) 131(3–4):94–103. doi:10.1016/j.applanim.2011.02.001
Keywords: ambiguity, anxiety, chickens, cognition, fearfulness, judgment, response strategy
Citation: de Haas EN, Lee C and Rodenburg TB (2017) Learning and Judgment Can Be Affected by Predisposed Fearfulness in Laying Hens. Front. Vet. Sci. 4:113. doi: 10.3389/fvets.2017.00113
Received: 04 April 2017; Accepted: 30 June 2017;
Published: 27 July 2017
Edited by:
Sabine G. Gebhardt-Henrich, University of Bern, SwitzerlandReviewed by:
Marie J. Haskell, Scotland’s Rural College, United KingdomMarisa Erasmus, Purdue University, United States
Copyright: © 2017 de Haas, Lee and Rodenburg. This is an open-access article distributed under the terms of the Creative Commons Attribution License (CC BY). The use, distribution or reproduction in other forums is permitted, provided the original author(s) or licensor are credited and that the original publication in this journal is cited, in accordance with accepted academic practice. No use, distribution or reproduction is permitted which does not comply with these terms.
*Correspondence: Elske N. de Haas, ZWxza2UuZGVoYWFzJiN4MDAwNDA7d3VyLm5s, ZW5kZWhhYXMmI3gwMDA0MDtnbWFpbC5jb20=