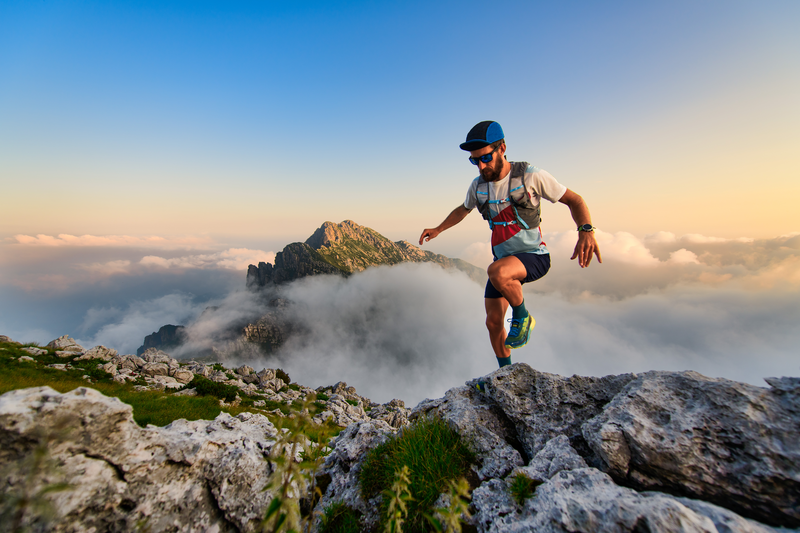
94% of researchers rate our articles as excellent or good
Learn more about the work of our research integrity team to safeguard the quality of each article we publish.
Find out more
REVIEW article
Front. Vet. Sci. , 28 November 2016
Sec. Animal Behavior and Welfare
Volume 3 - 2016 | https://doi.org/10.3389/fvets.2016.00108
There is a moral obligation to minimize pain in pigs used for human benefit. In livestock production, pigs experience pain caused by management procedures, e.g., castration and tail docking, injuries from fighting or poor housing conditions, “management diseases” like mastitis or streptococcal meningitis, and at parturition. Pigs used in biomedical research undergo procedures that are regarded as painful in humans, but do not receive similar levels of analgesia, and pet pigs also experience potentially painful conditions. In all contexts, accurate pain assessment is a prerequisite in (a) the estimation of the welfare consequences of noxious interventions and (b) the development of more effective pain mitigation strategies. This narrative review identifies the sources of pain in pigs, discusses the various assessment measures currently available, and proposes directions for future investigation.
Recent reviews have focused on the assessment and alleviation of pain in management procedures in pigs (1–3). A further review discussed the completeness of reporting on outcomes in pain mitigation studies involving neonatal piglets undergoing painful management procedures (4). Another discussed the reporting of pain management (or lack of) in pigs used in experimental surgery (5). The recognition and treatment of pain in pet pigs has also been reviewed (6).
This review focuses on the assessment of both “experimental” and “naturally occurring” pain, drawing examples from the farm animal and biomedical literature. The aim is to provide a basis for the future study of pain and pain assessment in pigs and to create tools for improving pig welfare.
Any pain assessment method must recognize that the pain experience – at least in humans – has three conceptual components, which, nevertheless, may be differentiated by brain imaging studies (7): sensory-discriminative, affective-motivational, and cognitive-evaluative, which interact with each other to form the pain experience (8). Cervero (9) described the sensory component as the detection and processing of noxious stimuli including reflex responses. The affective-motivational component is the aversive response or the “unpleasantness” of pain, and the cognitive component includes the worry about what the pain is, what it means, and how will it affect the future (9).
As pain is a subjective, personal experience, attempts to objectively measure it scientifically in animals are particularly challenging. Verbal self-report is considered the “gold standard” for pain assessment in humans, but even verbal pain communication has limitations (10). The International Association for the Study of Pain (IASP) state that an inability to communicate verbally does not negate the ability to experience pain, and the need for pain relief, and makes the ability to assess pain using non-verbal methods particularly important (11, 12).
Humans and vertebrates share similar neuroanatomical structures associated with pain perception, i.e., nociceptors, nociceptive pathways, and processing areas in the central nervous system (CNS) (13, 14), which justifies their extensive use in the study of human pain (15). Demonstrating that an animal is able to detect a noxious stimulus is straightforward and for many years, since the first methodology was described (16), scientists have used quantitative sensory testing (QST) to measure the thresholds at which animals respond. This simple, functional avoidance response is a basic ability and present, even in amebae. However, it does not encompass the complex nature of clinically relevant pain (9).
The ability to demonstrate that an animal perceives a painful experience as unpleasant or aversive is considerably more challenging. Nevertheless, many animals exhibit complex behavioral and physiological responses indicative of pain appreciation in situations where pain would be present in humans, and which are ameliorated by analgesics [e.g., Ref. (14)]. More recent behavioral paradigms have been developed to demonstrate the affective-motivational component of pain [e.g., Ref. (17)], but the ability of animals to experience the cognitive component of pain remains controversial (9).
Effective pain assessment tools should meet the requirements of the COnsensus-based Standards for the selection of health Measurement INstruments (COSMIN) taxonomy (18), and at least, be valid, reliable, and sensitive. Validity ensures the pain assessment device is measuring pain and not a related state such as anxiety, fear, or general sickness behavior. Reliability is tested to ensure the assessment tool is repeatable between (inter) and within (intra) observers and is not influenced by observer bias or observer subjectivity. Pain assessment tools should also be sensitive, i.e., be able to detect pain at low levels and increase linearly with the severity of pain.
Validation can be formally assessed against a “gold standard” measure (19). However, since there is no “gold standard” method of animal pain assessment; indicators must be thoroughly assessed using experimental approaches to ensure validity. Behavioral and physiological measures interpreted as indicative of pain in pigs have been investigated in experimental settings by (i) observing individuals before, during, and after a painful intervention or event; (ii) comparing individuals thought to be in pain to controls (no procedure, left undisturbed) or shams (no procedure but handled as if undergoing one); (iii) observing individuals with and without analgesia or analgesia delivered at different doses; and (iv) observing whether indicators of pain increase with the severity of pain. Ideally, a combination of these methods would be used to test the validity of pain assessment measures.
The ideal pain assessment tool should be well-validated. It would correctly differentiate animals in pain from those that are handled only or are left undisturbed. Its output would decrease or disappear with the use of effective analgesics compared with a placebo, and in a dose-dependent fashion. In addition, it would show a linear increase with rising pain intensity and would not “plateau” or show a “ceiling” effect. It would be specific to pain and not related to other features of the animals’ welfare state, such as fear, inappetence, immunosuppression, impaired mobility, or other sickness behaviors (though these are additionally relevant for assessing the overall level of impaired welfare or suffering in relation to injury or disease). For clinical use, it should be readily applied, inexpensive, non-invasive, provide immediate (not retrospective) information, and be repeatable over time (intraobserver reliability) and between observers (interobserver reliability). The ideal pain assessment device would equally appraise both sensory and emotional response to pain, i.e., measure what the pain means to the animal, rather than simply quantify the magnitude of noxious stimulation.
Pigs undergo painful procedures on farms, e.g., tail docking, teeth resection, castration, ear tagging, or notching (1) to enable identification, prevent injurious behavior, improve ease of management, and increase product quality (20). They are also injected with iron supplements, vaccines, and drugs, when necessary. The frequency with which these procedures are conducted on the farmed pig population has provided the opportunity to study acute and chronic pain responses and pain reduction strategies (21).
Tail docking (partial tail removal using clippers, cautery irons, knives, scalpels, or scissors) aims to reduce the incidence of, and damage caused by tail biting (22). Surgical castration entails scrotal incision, the blunt dissection of testicular from surrounding fascial tissue, and hyper-extension of the spermatic cord until it spontaneously ruptures or is cut (23) usually without anesthetic. The procedure aims to reduce or eliminate “boar taint,” and undesirable sexual behavior; castrated males are considered less aggressive and easier to manage (24). Teeth resection – the variable removal of needle (canine) teeth with cutting or grinding devices – is done to minimize bite injuries to littermates and the sow’s udder during the competition for functional teats (25). Ear notches or tags, tattoos, or injected transponders enable carcass identification, which is a prerequisite in meat quality improvement and public health schemes (26). All these management procedures induce tissue damage, which can potentially lead to a greater risk of infections and consequent pain.
Locomotor pain in pigs is indicated by lameness, by carrying a foot, favoring a leg, or being unable to get up and move around (27). Lameness is an important source of pain in terms of prevalence and severity (28), is ranked as the most important measure of pig welfare assessment in one survey of experts (29), and is thought to affect 10–20% of pigs on commercial farms (30). In a recent survey in the UK, lameness with minimal weight bearing in pigs was given a high score for pain by farmers (6.3 out of the 10) and veterinarians (7.0 out of the 10) (31). It is also a global concern in terms of economic loss, as locomotor problems are a common reason for early culling of sows, on-farm euthanasia, contribute to veterinary treatments costs, and carcass downgrading (32, 33). Lameness can be caused by injury due to poor housing conditions, non-infectious and infectious conditions, and degenerative diseases (34–36). Individuals can also be genetically predisposed to lameness (37), and lameness can be associated with inadequate nutrition, relating to growth rate (38). Additionally, a chemically induced transient lameness model was developed in sows to study clinical lameness detection and analgesic strategies (39).
The presence of anatomical and physiological structures associated with pain, as well as increasing experimental evidence, means it is generally accepted that a number of mammalian species experience pain at parturition (14, 40–42). Parturition pain originates from uterine contractions, fetal expulsion, and inflammation of the uterine tract (43). After birth, pain in human females results from intermittent uterine contractions during involution, when the uterus returns to its normal size and from tissue damage associated with birth (44). Evidence suggests pigs experience post-farrowing pain, as putative behavioral indicators of pain continue beyond the expulsion phase of farrowing in sows (45). Pain is greater during dystocia (46), but this is estimated to occur in only 0.25–1.0% of commercial sow farrowings (47), probably because the porcine fetus is small relative to the mother. In a survey of UK farmers and veterinarians, using a 11-point pain scale, respondents, respectively, awarded median scores of 3.8 and 4.5, for uncomplicated farrowings, and greater scores (6.7 and 7.3) to difficult farrowings in which an internal examination was required (31). This is important, given that farmer respondents to the survey considered 5.3% of gilts and 3.7% of sows to have difficulty farrowing and regularly used injectable oxytocin products to increase the frequency and intensity of uterine contractions, which are themselves associated with pain (48).
Diseases (49) including mastitis (34) and streptococcal meningitis (35) cause pain in pigs. Using the aforementioned pain scale, farmers and veterinarians awarded scores of 7.5 and 7.3, respectively, for infectious mastitis. In contrast, respiratory diseases were given moderate scores of 5.1 (by both farmers and veterinarians), while gastrointestinal diseases were also given moderate scores by farmers (5.6) and veterinarians (4.5) (31). Disease can result from poor climatic conditions, poor hygiene, inappropriate housing conditions, and genetics (49). Accurate information on the overall prevalence of disease in commercial pigs is lacking. One study investigating the clinical signs of disease in Danish finisher pigs provide some indication on the level of certain types of disease on-farm (50), and another Danish study provides information on the level of diarrhea and intestinal pathogens in pigs (51). Abattoir data is one method that has been used to estimate disease prevalence (52, 53) but is likely to underestimate the problem, since many diseased pigs may not make it to slaughter. In general, due to the nature of intensive pig farming, close contact between individual’s results in rapid transmission of disease within and between pig herds, and several endemic diseases are a feature of modern pork production (54). Therefore, as on-farm disease problems are widespread, painful disease states are a significant source of pain for a large number of pigs.
Pigs receive injuries when fighting, mounting, being handled ineptly, or reared under suboptimal conditions. Severe injuries like fractures and muscle tearing cause considerable pain, and leg fractures were awarded high scores by farmers (8.8) and veterinarians (9.5) (31). Other injuries include teat damage from biting piglets, cuts, bruises, and vulvar damage from aggressive interaction, while ear-, flank- and tail-biting occur under conditions of poor housing or environmental conditions (34). Shoulder ulcers are common in sows and are connected to many factors [for a review, see Ref. (55)] and were scored as moderately painful by farmers (5.6 out of the 10) and veterinarians (5.6 out of the 10) (31). The accurate prevalence of injury in commercial pigs is also unclear. Injury is another widespread problem associated with modern pork production (54) and was the most observed clinical sign observed in Danish finisher pigs (50). The prevalence of foot and limb lesions in preweaning piglets on commercial farms in Ireland ranged from 0 to 100% (56), and the overall prevalence on commercially representative pig farms in England was 39.6% (57). One study showed a high prevalence of lesions on the limbs (93%), body (20%) (58), and feet (59) of commercial sows in England. In addition, hernias are a relatively common condition in pigs (60), with umbilical hernias shown to affect 0.78% of finisher pigs in Denmark (50), and in severe cases, hernias can become ulcerated (61). Gastric ulceration is another management-related condition and potential source of pain in pigs, with one recent study in the UK showing 6% severe ulceration and 73% mild ulceration at slaughter (62).
The extensive use of pigs in biomedical research is justified on the basis of their physiological and anatomical similarity to humans, their large body size facilitates the collection of multiple samples, and they are omnivores with similar digestive systems to humans (63). Evidence-based information on postoperative pain management in pigs is lacking, despite the greater availability of analgesic drugs compared with farmed pigs (5). Pigs bred specifically for laboratory use, e.g., the Göttingen mini-pig, tends to be less vocal when manually restrained than commercial pigs. This may be the result of selective breeding for placidity or size and should not be taken to indicate an absence of pain or distress.
Spontaneously occurring behaviors arising or increasing in the context of painful conditions have been quantified in several studies, including observations of pigs before, during, and after painful events (39, 45, 64, 65), comparisons of those in pain with controls or shams (66–74), with and without analgesia or anesthesia (71, 75–82), and with varying degrees of severity (39, 65).
An early study of 4-week-old piglets identified “castration-specific” behaviors including trembling, leg shaking (jerking a hind leg back without scratching), sliding (sits and slides along on hind quarters), and tail jerking (64). Another study observed pain-specific behaviors after tail docking (tail wagging and jamming), teeth clipping (teeth champing), and ear notching (head shaking) (66). Other studies have observed more tail wagging, tail jamming, and bottom scooting (sitting, dragging bottom along the floor) in piglets following tail docking (67–69), and tail wagging associated with castration (70). In sows, shoulder rubbing has been associated with shoulder sores (73) and trembling, tail flicking, pulling the back leg forward, and pawing are putative pain indicators at parturition (45). Two studies showed no difference in “pain-related” behavior between individuals undergoing procedures and sham individuals for castration (71) and tail docking (72). This may have arisen because the scan sampling used may have missed relevant behaviors. Alternatively, there may have been fewer pain behaviors expressed because the observer was present. Both these issues have been discussed in relation to the use of spontaneous pain-related behavior in rodent pain assays (83).
Other pain-related behavior associated with castration include huddling up (lying with at least three legs tucked under body), spasms (quick, involuntary muscle contractions), stiffness (lying with extended, tensed legs), prostration (sit or stand motionless, head down), trembling (shivering as if cold), and rump scratching. Pre-castration treatment with the non-steroidal anti-inflammatory drug (NSAID) meloxicam resulted in less pain-related behavior afterward (75), irrespective of whether a local anesthetic was used. In another study, fewer piglets in a meloxicam-treated group exhibited pain-related behavior (78). As expected, little or no difference in pain-related behavior was seen after castration performed with and without general anesthesia (71, 76, 77). This is expected, given the use of anesthetic during the procedure, but no post-procedural analgesia. In addition, no difference in bottom scooting or huddling was seen following castration when long- or short-acting topical local anesthetics were applied to the severed spermatic cords and scrotal incisions (71).
Recent pig studies have measured leg loading and movement using pressure mats, force plates motion-capture systems, and accelerometers as objective measures of locomotor abnormalities associated with lameness. Among other things, lame individuals show asymmetrical weight distribution between legs, increased step frequency, stance time, and altered stride length (74, 84, 85). The opioid analgesic buprenorphine decreased pressure mat gait asymmetry (79), and the NSAID meloxicam decreased step frequency and produced better symmetry of pelvic limb movement (82) in pigs with naturally occurring lameness. In a chemically induced transient sow lameness model, leg loading and movement improved with the use of the NSAIDs meloxicam and flunixin (80), while the severity of lameness decreased and resolved by day 7 following induction (39, 65). However, another study using the same lameness model found no improvement with the use of the NSAIDs sodium salicylate or flunixin (81). The authors of the more recent study (80) suggested this difference could be due to the use of additional time-points compared with the previous study (81), which better coincided with when the drug is thought to be most effective. They also suggested that using more sows in the study created greater statistical power (80). In a more recent study, continuous behavioral observations, but not scan sampling, detected differences in lame sows treated with flunixin, whereas both techniques detected improvements to sows treated with meloxicam, in this case, demonstrating the greater efficacy of meloxicam over flunixin to treat lameness in sows (86).
Escape or avoidance behavior has been used as a measure of pain recorded during a pre- and post-procedural handling period (87), compared individuals undergoing painful and sham procedures (88), and those given a local anesthetic or not (87, 89). These behaviors, scored in terms of the frequency (75, 76, 88), duration (87), and/or intensity (76, 87, 89) of resistance movements have also been recorded during different periods of the castration procedure (89), and when different techniques were used (88), as these are likely to affect the severity of pain experienced. Duration and intensity scores of both defense behavior increased from the pretreatment to treatment period in piglets castrated without anesthetic, whereas they did not differ in sham-castrated piglets (87). When procedural time was taken into account, no differences in escape attempts and leg kicks were found between different methods of teeth resection, tail docking, identification, iron administration, and sham procedures (88). Not unexpectedly, a significant reduction in escape behavior during castration was exhibited in piglets given a local anesthetic (75, 87). These measures are useful in assessing pain associated with severe acute events, like tail docking or castration, in order to evaluate pain reduction strategies, such as the use of local anesthetics.
Posture and posture changes have been measured before, during, and after painful events (64, 90), compared with controls/shams (68, 69, 73, 91–94), with and without anesthesia or analgesia (75, 76, 82, 95–98) and with the severity of painful conditions (99).
Docked piglets tended to spend more time sitting than shams following the procedure, which may relieve pain at the tail while protecting the wound site (68). Wemelsfelder and Van Putten (64) reported longer latencies to lie down in piglets 1 day post-castration at each individual phase of the lying sequence. In addition, the lying sequence differed significantly. Another study described changes in posture after castration, which included weakened and protracted limbs, non-weight bearing on limbs, a “tip-toe” walking pattern, and back hunching (94). Other castration studies have shown contrasting results relating to posture: more sitting and standing and less lying was seen in one study (91), while another observed increased lying and decreased standing (92). Torrey et al. (69) observed more standing and less lying in tail-docked, ear-notched, and sham-processed piglets compared to undisturbed controls, indicating that the alteration in posture was due to handling, regardless of the painful procedure. Other studies did not find differences in the time spent in different postures in relation to painful procedures (64, 75, 76, 93).
The frequencies of posture changes and durations of postural behavior have been used in assessing pain in sows arising from farrowing (95–97, 99–101), the presence of shoulder ulcers (73), and lameness (82, 90, 98). Naloxone (an opioid antagonist) administration increased standing, lying ventrally, and postural changes in periparturient sows, indicating that endogenous opioids may have a role in reducing pain at farrowing, which is manifest by frequent posture changes (101). In contrast, Lawrence et al. (102) recorded decreased frequency and duration of standing in naloxone-treated sows. Posture and the number of posture changes have been used to evaluate the use of post-farrowing analgesics (95–97, 100). Haussmann et al. (100) demonstrated that sows administered butophanol for 3 days post-parturition adopted fewer body position changes between 48 and 72 h afterward. Sows administered meloxicam for three consecutive days spent less time lying on the third day post-partum compared with placebo-treated animals (97). No difference in the amount of standing or duration of standing bouts was detected between sows given a single injection of meloxicam or placebo post-farrowing (95). For sows given ketoprofen for 3 days post-farrowing, no overall treatment differences in posture were observed, but younger sows treated with ketoprofen had more body position changes (96). Sows with shoulder ulcers spent less time lying and standing still and exhibited more posture changes than healthy animals (73).
Naturally lame sows treated with meloxicam showed an increase in standing time after feeding compared with those given a placebo (82). Predictably, sows rendered lame by intra-articular chemical irritant injection showed increased lying and decreased standing behavior (90, 98). Chemically induced lame sows treated with meloxicam lay down less frequently than saline-treated individuals, although those treated with flunixin did not differ from those in the placebo group (98).
Other behavioral alterations have been measured before and after painful events (64) and compared with control or sham animals (66, 70, 71, 73, 91–94, 103–105) from which analgesics were given or withheld (71, 72, 75–77, 79, 92, 103, 105, 106). Avoiding social contact with littermates has been recorded after tail docking (72) and castration (71, 93). Isolation, i.e., the state of being alone or with just one litter mate, and desynchronization, defined as activity that differs from 75% of littermates, was apparent up to 3 days following castration (70, 93). Other castration studies, however, have revealed no differences in social cohesion (75–77). The effects of painful procedures on teat seeking, udder mouthing/massaging, and nursing behavior are inconsistent. One study found castrated piglets spent more time massaging the udder (93), while others have shown a reduction in the duration of suckling in castrated individuals (92, 93, 103), longer latencies to participate in udder massage (64), or reduced levels of activity at the udder (77). In addition, sows with shoulder ulcers spent less time nursing than those without (73). Piglets castrated under azaperone/ketamine anesthesia used significantly more teats post-castration compared to pre-castration, which caused disturbance in suckling behavior (106). In addition Mcglone and Hellman (103) found that piglets given a general anesthetic missed sucklings, whereas no other piglets did. Both reports seem to suggest a detrimental effect of anesthesia on suckling activity. Other studies found no difference in suckling behavior in relation to castration (71, 76, 91, 92, 104) or tail docking (72).
Pain usually reduces feeding behavior in pigs. A significant reduction in feed intake and drinking behavior is associated with castration in 7- and 8-week-old pigs (92, 103). Feed intake for sows given meloxicam or a saline placebo post-farrowing was not statistically different (97), but feed refusal during lactation was delayed in sows given ketoprofen post-farrowing (107). Feed intake was used to assess the benefit of ketoprofen compared with flunixin or untreated grower pigs experimentally infected with Actinobacillus pleuropneumoniae (108), and altered feed intake (using a 4-point scale in which 1 indicated no change and 4 inappetance) was used as part of a clinical index score to assess the efficacy of meloxicam in the treatment of sows with mastitis–metritis–agalactia (MMA) (109). Food consumption decreased after thoracotomy surgery in pigs (110), and time spent feeding has been used to assess the efficacy of pain mitigation strategies in pigs undergoing abdominal surgery (111) and a femoral fracture model (112). In a study using a feed reward collection test to assess sows’ motivation to obtain feed rewards with different levels of lameness, moderately and severely lame sows obtained fewer rewards than mildly and non-lame sows (113). Unexpectedly, tail-bitten pigs, which had been given ketoprofen, spent less time at the feeder compared with those given a saline placebo (114).
Additional behavioral variables measured in relation to pain include aggression (70, 71, 77), playing, nosing, chewing and licking (64, 70, 75, 77, 93, 111), general activity (70, 72, 75, 76, 79, 91, 93, 94, 105, 106, 110), and location within the pen (76, 92, 103, 106). Painful procedures appear to disturb in particular interactive behaviors (chew, lick, play, nose, and aggression) (70, 77, 93, 104). Surprisingly, some studies have found no differences in general activity associated with tail docking and castration (72, 75, 76, 91). One report indicated that the location of piglets in relation to the heat lamp was altered following castration (103), while other studies found no differences (70, 76, 92). Castrated piglets took longer to navigate a handling chute at 0 and 15 min post-castration, with a reduction in navigation time observed only at 0 min after administration of preemptive meloxicam (105). A greater latency to move was measured in castrated compared with sham-castrated individuals when piglets were returned to the pen following the procedure (94). Reduced activity levels were returned to pretreatment levels 3 days after thoracotomy surgery (110), and latencies to perform active behaviors (rooting, eating, and drinking) were shorter for pigs given additional analgesia after abdominal surgery (111). The administration of buprenorphine increased the activity of lame pig in an open field test (79), although opioids in the absence of pain can increase activity levels.
Experimental studies of animal pain frequently score changes in both specified and unspecified behaviors to produce rating scales. Scoring systems include the visual analog scale (VAS), which is usually scored on a 10-cm line, numerical rating scales (e.g., 0–4), simple descriptive scales (e.g., mild, moderate, and severe), and variable rating scales, where several parameters are scored and the total of these used to indicate severity. For example, one study investigating castration pain used a variable rating scale – referred to as a Global Behavior Score (GBS), which combined scores for the presence of prostration, tremors, tail movement, and self-isolation (78). Following laryngeal transplantation, mini pigs were assessed for pain twice daily by wound palpation, behavior, and locomotion and given a score between 0 (no pain) and 10 (worst possible pain) (115). Two types of analgesia for post-thoracotomy were assessed in pigs (110) using the scoring system presented by Morton and Griffiths (15). Using a 4-point scale from 0 = no change to 3 = gross change from normal, patients were scored on five parameters including body weight, appearance, clinical signs, unprovoked behavior, and response to an appropriate stimulus, with the highest possible score being 15 (15). Two other studies of postoperative pain in pigs utilized a combination of scores for several spontaneous behavioral measures in addition to responses to pressure on the wound and human presence in the pen (116, 117). Behavioral responses of pigs undergoing surgery involving skin and muscle incision were greater compared to those with skin incision only at 1 h post-surgery (116). Another study used a behavioral score to assess the efficacy of regional anesthesia in addition to systemic opioid analgesia in a porcine femoral fracture model, which was a modified VAS with a scale for vocalization, behavior, on approach to the pen, response to handling, willingness to walk, and overall pain (no pain to worst pain imaginable) (112).
Pain scoring has been applied post-procedurally to pigs used in biomedical research to optimize postoperative care and assess differences in treatment methods. Evidence that an element of pain assessment is incorporated in these studies is good, albeit rare (5, 112). However, while these scores might be useful in a clinical setting, they may not be a valid approach to experimental pain assessment, as duration, frequency, or relative importance of individual behaviors is not considered. A more valid approach was demonstrated in a tail-docking study comparing docked with sham-docked piglets, by the use of a pain score constructed on behaviors recorded for 1 min immediately after docking (67). Combinations of all the behaviors recorded were analyzed in cross-validated discriminant analyses, which enabled the combination of behaviors that best discriminated between docked and sham-docked piglets to be used as part of the overall pain score.
Scores have also been used to assess lameness by the simple presence or absence of lameness (32, 118, 119), simple descriptive scales to indicate the varying degree of severity (120–123), and variable rating scales including several parameters relating to lameness (30, 124). A clinical lameness score, combining lameness at rest and at walk, was used to assess the efficacy of an intramuscular injection of meloxicam compared to a placebo to treat non-infectious locomotor disorders (122). Mustonen et al. (123) looked at two doses of oral ketoprofen compared to a placebo to treat non-infectious lameness, which they scored on a 5-point scale from no lameness to severe lameness. Both studies saw a significant improvement with drug treatment, compared with the placebo (122, 123). Nonetheless, some caution is needed, as locomotor disorders do not necessarily result in pain, with individuals possibly affected by a biomechanical abnormality in the absence of pain. Subjective scores are prone to poor reliability (125), and one study showed poorer interobserver agreement at the lower end of the lameness scale (126). For example, no difference in motivation to obtain a food reward was detected between mildly lame and non-lame sows (113). However, it is possible that pigs may conceal signs of mild pain more easily than signs of severe lameness-pain, which could be advantageous in relation to access to resources.
Nociception defined as “the neural processes of encoding and processing noxious stimuli” (127) can be quantified in response to painful or potentially painful stimuli and is expressed as the minimum nociceptive threshold (i.e., latency or force/temperature) required to induce an avoidance response. Although QST only measures the sensitivity of the animals to detect a noxious stimulus, rather than pain, it allows the detection of abnormal thresholds caused by painful conditions, such as injuries or diseases in animals, including pigs. Jarvis et al. (128) measured the nociceptive threshold of pigs at pregnancy and parturition using a thermal stimulus provided by a CO2 infrared laser. The stimulation consisted of heat focused on the skin of sows, and it was interrupted with the occurrence of a physical response (e.g., a tail flick, movement of the back leg, or muscle twitch), providing an indication of the analgesic effect of endogenous opioids through detection of thresholds of sensitivity. A similar approach was used by Herskin et al. (129) to measure thermal nociceptive thresholds in two different anatomical locations on the pig (the back of the rear leg and the shoulder). The authors reported that increasing the power output of the laser decreased response latencies and altered the types of response from moving or lifting the leg to kicking, and from less obvious movement of the shoulder or body, to increased muscle twitching and rubbing of the area. Another nociceptive assay commonly used in laboratory species, the tail-withdrawal reflex induced by thermal stimulation, has also been applied to pigs. Immersion of the tail in warm water induced a tail flick, and response latencies were reduced by higher water temperatures (130). Other studies have used a modified version of the tail-withdrawal reflex test, using a plastic-coated electrical resistor, which is heated and pressed onto a selected area on the rump to induce a tail flick (131–133).
Mechanical nocistimulation have also found recent application in pigs. Herskin and Rasmussen (134) described thresholds of mechanical nociception in the pelvic limb of gilts using an electronic von Frey anesthesiometer, with four categories of behavioral response used to detect the threshold response, from slight leg movements to kicking. Sandercock et al. (135) measured mechanical thresholds at the tail root of pigs using von Frey filaments and at the foot with a plantar stimulator. The two methods were applied to investigate changes in thresholds of nociception with neonatal pain (tail docking) with or without prenatal stress. Tail docking did not result in any alterations in response to these tests but prenatally stressed pigs had higher thresholds to mechanical stimulation and longer response durations to cold stimulation. To determine the analgesic efficacy of ketoprofen in piglets, a handheld algometer was used to quantify mechanical thresholds pre- and posttreatment. The higher thresholds recorded in the ketoprofen-treated group compared to the placebo group suggested an anti-nociceptive effect between 12 and 24 h after drug administration, through the reduction of sensitivity of the peripheral nerve receptors (136).
The assessment of nociception threshold changes in pigs has been used in the study of traumatic pain – particularly lameness. In lame sows, a significant decrease in threshold values is revealed by pressure algometry (137, 138). The same methodologies have enabled researchers to assess the efficacy of NSAIDs in this context (80, 81). The assessment of nociceptive responses in pigs has received recent interest because their high homology with humans provides a translational model of induced cutaneous inflammatory pain. Methods for measuring thermal and mechanical nociceptive responses in awake pigs have been described, along with factors that may influence response thresholds (139–141). Models of cutaneous inflammatory pain, using capsaicin and UV-B irradiation have been used, demonstrating thermal and mechanical hyperalgesia in pigs (142, 143). Most recently, the choice of the pig as model for preclinical research has led to the characterization of postsurgical pain through the quantification of mechanical nociceptive thresholds (116, 144). Similarly, nociceptive stimuli delivered with an infrared diode laser have been employed to evoke withdrawal responses in the investigation of the efficacy of a novel analgesic (145).
To summarize the previous sections of discussion on behavioral indicators of pain in pigs, it is clear that spontaneous pain-related behaviors have most reliability in indicating pain across several studies. Some behavioral measures including posture, posture changes, altered social interaction, and exploration have demonstrated lower between-study reliability. Therefore, it seems behavioral indicators specific to the painful condition are the most reliable indicators of pain. The use of spontaneous pain-related behavior is not without problems; often the frequency of behavior is low and between-subject variation high, resulting in floor effects (83). Continuous behavioral observation is also time consuming, requires observer training, and is prone to interobserver variation (146). Therefore, specific behavioral indicators need to be carefully investigated and validated as pain indicators, as, for example, certain conditions involving physical injury or disease can result in loss of function, regardless of pain (125). In addition, behavioral expression of underlying welfare states has been shown to be altered by emotional contagion and social support, which could also be the case for pain (147–150). The social influence was mitigated in a tail-docking study, by observing pain-related behavior in piglets in isolation after the procedure (67); however, isolation in itself may alter the behavioral expression of pain (83). This time-consuming approach involving the detailed quantification and careful validation of behavior is useful in investigating pain in a research setting. However, the validation of complex behavioral patterns into simplified scores or rating scales, for the assessment and treatment of clinical pain on-farm and in biomedical research pigs, is much needed. Finally, QST is a useful method to investigate changes in peripheral nociceptive sensitivity as a consequence of injury or disease but does not address the multidimensional pain experience or how pain rather than peripheral sensitivity is ameliorated by analgesic treatment.
Pigs also vocalize when painful areas are palpated but resent handling and may vocalize regardless of pain (27, 151). Several studies have found differences in the calls of piglets undergoing a painful procedure as opposed to handling alone, including call rate, duration, and type of call (64, 66, 75, 76, 78). More detailed analyses of calls using sound analysis software have revealed further differences in calls in relation to painful stimuli (88, 152–156). “Painful grunts,” along with persistent straining have been observed in cases of dystocia (27).
A higher grunt frequency was detected during tail docking compared to teeth clipping and ear notching, and howls were measured during tail docking but not the other two procedures (66). Sonograms of seven castrated piglets were analyzed, comparing them before and during castration: differences included a broader number of frequencies within the sound, a change of pitch, and a shift in volume during castration compared with the handling period (64). Hansson et al. (75) used a decibel meter during castration and recorded the highest intensity level (in decibels) of piglets castrated with and without a local anesthetic (lidocaine). Piglets with the local anesthetic produced calls with a significantly lower intensity than those without. In a more detailed vocal analysis during castration, White et al. (152) compared piglets castrated at different ages and with and without a local anesthetic (lidocaine), and segments of calls with the highest energy frequency (HEF in kilohertz) were used for analysis. HEF was significantly higher in piglets without anesthesia and increased with age, but the HEF was lower during severing of the spermatic cord (152), which is thought to be the most painful part of surgical castration (154). Other studies investigating vocalization during castration categorize vocalization based on call frequency, high calls being ≥1000 Hz and low calls ≤1000 Hz and found the rate (91, 153, 154) and total duration (156) of high calls is associated with castration, tail docking. and ear notching (69). Piglets castrated with no analgesia “screamed” significantly louder than those given local anesthetic or that were sham-castrated (156). Also, analyzing pain-related vocalization in piglets during castration and with and without a local anesthetic (lidocaine), Marx et al. (155) identified three different call types from spectrograms: grunts, squeals, and screams. The number of screams, but not the other two call types, were significantly more frequent for piglets castrated without anesthesia, indicating an increase in the rate of screams is a good indicator of pain. Screams were differentiated from squeals by sound parameters, including a lower peak and main frequency (155).
Several studies on vocalization in relation to pain use a device called STREMODO (STREss MOnitor and DOcumentation unit), which uses linear prediction analysis (157) to extract features of calls and classifies them as stress calls, as opposed to non-stress calls or background noise (158, 159). Puppe et al. (160) used STREMODO to detect stress calls of piglets undergoing castration and measured different vocal parameters. The surgical and postsurgical period differed in call duration, peak frequency, pureness, and entropy of sound, but not number of calls, and the surgical and presurgical period differed in the pureness and entropy of the sound. Therefore, during castration, the calls became longer and clearer with lower pitch. Leidig et al. (87) used STREMODO to detect stress calls during castration and compared the total duration of stress calls with piglets undergoing castration with or without a local anesthetic (procaine) or handling alone. Duration of stress calls increased from the pretreatment handling period to the surgical period, except for the sham animals, and was significantly longer in the castrated group without anesthesia but was also increased on the injection of anesthesia. Sutherland et al. (71, 72) used STREMODO to compare vocalization in relation to castration and tail docking, observing more stress vocalization with the painful procedures.
The expression of the c-fos gene and its protein product Fos in neurons of the spinal cord are used as a measure of neural activity in response to painful stimuli [for review, see Ref. (161)]. In pigs, the number of spinal Fos-positive neurons has been measured following castration with no anesthesia, a local anesthetic, or a general anesthetic (162) and following laparotomy with local anesthesia or a saline control (163). Following surgical castration with no anesthetic, a greater number of neurons in the dorsal horn were Fos positive, compared with piglets given a local or general anesthetic (162). Pigs that underwent experimental laparotomy with a local anesthetic as well as a general anesthetic had fewer Fos-like-immunoreactive neurons in the dorsal horn than those given saline and had a similar number to controls (163).
Substance P (SP) is a neurotransmitter released directly from damaged nerve fibers at the site of tissue damage (164, 165); it has been related to pain perception (166), making it a potential biomarker of pain. SP was elevated in compressed spinal nerve roots, compared with uncompressed controls, in a porcine model (166). Piglet castration did not elicit a plasma SP response at 3 days old (167) or in piglets that were castrated, tailed docked, and had iron administered at 5 days old (168). A study of castration indicated that SP could be a useful pain biomarker in calves (169), and SP has been shown to have a systemic role (170), indicating that measurement of systemic concentrations, rather than just at the site of damage, could be useful. However, further study is needed to indicate if this is a useful systemic biomarker of pain in pigs.
Many studies have used measures of the activity of the hypothalamic–pituitary–adrenal (HPA) axis in relation to pain. The HPA axis is activated by physical and psychological stressors to promote recovery by increasing metabolism and reducing inflammation (21). In response to physical or psychological stress, corticotrophin-releasing hormone (CRH) is released by the hypothalamus, stimulating the secretion of adrenocorticotrophic hormone (ACTH) from the anterior pituitary, which acts on the adrenal gland to produce cortisol. Studies have investigated cortisol/ACTH levels in pigs with and without (controls/shams) a painful condition (68, 70, 72, 88, 93, 94, 104, 156, 171, 172), before, during, or after (68, 173–175), with or without anesthesia or analgesia (72, 78, 111, 156, 168), and associated with the severity of the pain (68, 88, 101, 171, 176).
In the periparturient period, sow corticoid levels increased from day 3 before parturition, peaking on the day of parturition and returning to pre-parturition levels on day 2 afterward (173). Cortisol and ACTH were higher during the pre-farrowing nest-building period in slatted crates compared to straw-bedded pens, indicating that restricting nest-building behavior is stressful (174, 175). However, after the onset of farrowing, no difference in levels of cortisol or ACTH between environment and the fact that these hormones remain elevated indicates that the process of parturition itself is stressful (173–175), and part of this could be pain related. Looking in more detail after the birth of the first piglet, cortisol concentration gradually increased with successive births (176) and was greater following the birth of larger piglets (101).
Studies have measured cortisol and/or ACTH in relation to tail docking (68, 72, 88, 171), teeth resection (88, 171), identification, iron administration (88), and castration (88, 94, 104, 156, 168, 171, 172). The majority of castration studies show an increase in cortisol and/or ACTH (88, 94, 104, 156, 171, 172). One study found no differences in cortisol and cortisone measured in urine 4 days following castration, sham castration, or piglets left undisturbed (70). The authors suggest that the sampling times may have been inadequate or that cortisol and cortisone in urine may not be good measures of sub-chronic and chronic pain. Cortisol has also been shown to increase with tail docking (68, 72), but not in all cases (68, 171), and no difference in cortisol between two different docking methods (side-cutter pliers or a hot cautery iron) was found (88). The authors of one study have four possible hypotheses for this lack of response that are as follows: (1) the HPA axis is not responsive to stress at 1-day-old; (2) it is highly responsive, and the maximum response is achieved by sampling; (3) the birth process; or (4) nociceptive stimuli from tail docking and teeth clipping is not enough to induce a response (171). For piglets that were ear-notched as opposed to tagged, cortisol tended to be higher 4 h after the procedure and for piglets with iron administered by injection rather than orally, cortisol was significantly higher 2 days after dosing (88). Cortisol tended to be higher 1 week post teeth grinding compared to clipping, but this difference disappeared with baseline values as a covariate (88), and another study showed an increase in cortisol and ACTH with teeth clipping and grinding up to 15 min following the procedures (171).
Piglets castrated with meloxicam administered before the procedure had lower cortisol and ACTH 30 min after compared to those given a saline placebo, and the meloxicam group had values of ACTH similar to controls (78). In addition, meloxicam administered via the transmammany route resulted in lower cortisol concentrations in castrated, tail-docked, and iron-injected piglets 10 h after the event (168). Another study found no differences in cortisol when castrated piglets were given local anesthetic, meloxicam, both, or no analgesia (156), and no difference in cortisol was found between tail docking with no anesthetic, topical anesthetic, or general anesthetic (72). Piglets castrated with or without anesthesia, had no difference in ACTH measured immediately afterward, despite obvious differences in other measures, and the authors suggest that handling or sampling had an effect or the collection time was inadequate (89). In an investigation of pain induced by abdominal surgery in pigs, epidural morphine was administered pre-op in combination with transdermal fentanyl patches post-op or a placebo of both. Pigs given the analgesia had significantly lower cortisol immediately after surgery, and levels decreased in both groups on the 3 days following surgery (111). In another study involving abdominal surgery, ACTH increased 15 min and remained significantly higher until 60 min post-surgery in pigs given a saline placebo in contrast to animals receiving local anesthesia, which showed no increase in ACTH (163).
The sympathetic adrenomedullary (SA) system, part of the autonomic nervous system (ANS), is involved in mobilizing the body for “fight-or-flight,” increasing heart and respiration rates, paling or flushing, slowing or stopping digestion, constricting blood vessels, liberating nutrients (blood or glucose), dilating blood vessels for muscles, dilating pupils, etc. Skin temperature is thought to be a good indicator of SA response (75) as blood vessels in the skin constrict as blood is directed to the muscles and/or internal organs in preparation for fight-or-flight. Ear temperature was significantly higher for castrated piglets given no analgesia compared to those given a local anesthetic or a local anesthetic with an NSAID (75). By contrast, castrated, tail-docked and iron-injected piglets with transmammary-administered meloxicam had higher cranial temperature than those given a placebo (168). In another study, eye temperature was increased in castrated piglets up to 4 h after the procedure, rectal temperature was also greater at 3 and 5 h post-procedure, but glucose and lactate were not altered (94).
In a study investigating electroencephalographic and cardiovascular responses to nociception under isoflurane anesthesia, pinching of the nasal septum, anus, periople, and inter-digital skin and clamping of the tail and hind claw were used (177). Electroencephalographic measures and heart rate did not alter, but mean arterial blood pressure (MAP) increased with noxious stimulation, therefore was the most sensitive measure of nociception. Castration without local anesthetic resulted in a significantly greater rise in MAP and fall in pulse rate compared to piglets given an intratesticular or intrafunicular local anesthetic (178).
Beta-endorphin (β-end) is one of three families of opioid peptides, it is involved in regulating the body’s response to stress, including pain [for review on biology and function, see Ref. (179)]. The endogenous opioid β-end has been measured around parturition and is thought to be involved in reducing pain (176), maintaining passivity (180), and regulating oxytocin release (102). The administration of naloxone reduced the nociceptive threshold of sows, but not fully, indicating an endogenous opioid mechanism is perhaps only partly involved in hypoalgesia around parturition (128). Teeth resection by grinding rather than clipping produced a significantly higher β-end concentration 4 h after the procedure, and cutting the spermatic cord as opposed to tearing produced a higher β-end following castration (88). No difference in β-end concentrations was found between castrated piglets with isoflurane, isoflurane, and nitrous oxide general anesthetics or no anesthesia (89) and between pigs given epidural morphine before and fentanyl patches after or placebos when undergoing abdominal surgery (111). Alterations in β-end found by teeth grinding vs. clipping and cut vs. tear methods of castration were discussed by the authors and could be associated with other factors, including the increased blood loss from cutting and the increase in β-end with ACTH and cortisol being catabolic (88). Similarly, the lack of difference in β-end following castration with and without anesthesia or abdominal surgery with and without analgesia could be due to the stress of blood sampling producing a response regardless of treatment or inadequate sampling times (89, 111).
Inflammation, an immediate response to injury or infection, is characterized by redness, swelling, heat, pain, and diminished function. However, the magnitude of the inflammatory response and the pain experienced are not necessarily proportional (181). Inflammation is also associated with the acute-phase response, which causes an increase (positive) or decrease (negative) in acute-phase proteins (or APPs) triggered by pro-inflammatory cytokines from injured or infected cells. Concentrations of APPs, including haptoglobin (Hp), C-reactive protein (CRP), and serum amyloid A (SAA) can assess the level of inflammation and/or tissue damage in pigs (182). Cytokines and acute-phase proteins have been measured in relation to tail docking (183), castration (75, 78, 93), and teeth resection (184), and with the administration of NSAIDs in relation to Streptococcus suis infection (185) and parturition (107) in pigs.
In piglets left intact, or tail-docked by hot cautery or blunt trauma methods, no difference in CRP levels was present at 3 weeks, although at 7 weeks, CRP levels were significantly greater in the intact animals, being correlated with tail-biting injury scores (183). The use of meloxicam administered before castration reduced the level of Hp compared to untreated controls but not to levels that showed statistical significance (78) and in groups given meloxicam following castration, fewer piglets had high levels of SAA (75). Another castration study measuring cytokines [tumor necrosis factor-alpha (TNF-α) and interleukin 1 beta (IL1-β)] and acute-phase proteins (CRP, SAA, and Hp) found no difference between castrated pigs and those that were handled only (93). However, both cytokines increased in castrated and handled groups indicating handling or sampling alone could initiate pro-inflammatory responses and CRP and Hp or, more likely, they could have been elevated as both groups were also tail-docked, teeth-clipped, and ear-notched previously. On day 1 following teeth clipping, grinding, or left intact, no difference in levels of CRP or SAA were found; however, on day 29 following treatments, both APPs were significantly higher than on day 1, and CRP was higher in teeth-clipped compared to ground piglets (184). The authors suggest that the acute-phase response could have been initiated by the process of weaning, face lesions in piglets with teeth left intact, or mouth lesions in those teeth ground or clipped.
In a study that used an endotoxin challenge, CRP and Hp increased, but no difference between groups given meloxicam or a placebo was seen; however, thromboxane B2 (a vasoconstrictor and platelet aggregator) was higher in the placebo group (186). Measurement of acute-phase proteins following subcutaneous injection of live Streptococcus suis showed a correlation with clinical signs of infection including the development of fever and lameness (185). The biomarkers serum aspartate aminotransferase (AST) and creatine kinase (CK) and the APPs Hp and SAA were measured in relation to the use of a post-farrowing NSAID (107). An increase in AST, CK, and SAA was seen with parturition indicating tissue damage and inflammation associated with the process of parturition, and a greater increase in these biomarkers was demonstrated with the use of the NSAID, which the authors suggested is likely to be due to local tissue irritation from the intramuscular injection (107).
Perceived pain in non-verbal patients cannot be directly measured, or based on other measures, it can only be inferred (151). This means there is no “gold standard” for pain assessment in pigs. Since validation is based on the measurement of an indicator against a “gold standard” (19), pain indicators must be thoroughly evaluated using experimental studies. As information on this topic is rapidly growing, a thorough examination of the literature is warranted. As discussed, a wide range of behavioral indicators have been used in the assessment of pain in pigs, in addition to physiological measures as potential biomarkers of pain. The majority of studies used several measures simultaneously, with different experimental approaches to evaluate the potential value of these measures as indicators of pain. These approaches are used to test validity, reliability, and sensitivity, which are important if measures are to be used in the clinical assessment of pain to provide treatment, to ethically evaluate the cost of pain, and to test the efficacy of existing and novel analgesic drugs (for veterinary and human use). Table 1 lists the indicators by the broad categories outlined in this review, showing the counts of studies that used these indicators, whether they have been validated using the approaches outlined in the Section “Introduction,” and some brief advantages and disadvantages as pain indicators. As shown in Table 1, the assessment of spontaneous “pain-related” behavior is the most promising method of assessing pain in pigs. This approach has been used extensively in the rodent literature to assess pain in order to provide the best possible treatment and refine painful techniques [e.g., Ref. (146)]. For clinical use, it is also useful to combine measures into scores of multiple behaviors and clinical signs. Physiological indicators are prone to low specificity to pain (Table 1); however, using multiple measures, including behavior and physiology, is useful to fully assess the impact of a painful condition or event on the individual.
Table 1. Summary of pain indicators used in experimental studies involving the assessment of pain in pigs and how they have been assessed in relation to pain using the following methods: (1) individuals studied before, during, and after a painful event; (2) comparing individuals thought to be in pain to controls or shams; (3) observing individuals with and without analgesia or anesthesia; and (4) observing whether indicators increase with the severity of pain, counts of studies using these measures, followed by some advantages and disadvantages in relation to validity, specificity, and reliability.
Behavior is often used as a welfare indicator because of the difficulty in interpreting physiological indicators. According to Dawkins, “the most obvious, least intrusive and potentially most powerful alternative of all [to assess welfare] is the animal’s behaviour” (187). Its unobtrusiveness means it is the most frequently used measure in the assessment of pain in research studies involving pigs. Behaviors (including posture, gait, tremors, and restlessness) are used by pork producers in Victoria, Australia in the on-farm assessment of pain in pigs (188). However, the continued use of behavior in pig pain studies, involving the validation and simplification of behavioral assessment is needed to facilitate its application in the clinical assessment of pain. In addition, simplifying and standardizing behavioral approaches to pain assessment would assist in comparing measures across studies, to better indicate the reliability of these measures.
Simple behavioral responses to noxious stimuli, including those used in QST, address the ability to respond to noxious stimuli, but not necessarily the more complex, multidimensional experience that is pain (9). In addition, combining the use of QST, with spontaneous pain-related behavior to assess pain reduction strategies is suggested, since spontaneous pain is not always directly linked to hypersensitivity (189). Complex spontaneous behaviors probably reflect pain better than simple reflex responses, but there are other approaches that could be used. Drawing on the recent advances in clinical models of pain research, which focuses predominantly on laboratory rodent, a large body of novel methodological approaches can be adapted for use in pigs. Pigs can be readily trained to perform well in various cognitive tests or operant conditioning tasks (190), making them a good model for assessing the affective-motivational dimension of pain, including conditioned place preference, conditioned place aversion, self-section of analgesia, and motivational trade-offs, which have been developed in rodent models (83).
Facial grimace scaling is being validated in an increasing number of species and may prove useful in pigs. In an eye-tracking study of pain behavior in rabbits, it was found that observers focused on the rabbits’ facial features rather than other body areas (irrespective of the observer’s experience) (191). A facial grimace scale was first created for laboratory mice (192), and others have since been created for rats (193), rabbits (194), horses (195), and sheep (196, 197). A Piglet Grimace Scale (PGS) has been developed by experienced observers, who identified several facial action units (FAUs) from images of piglets pre- and post-tail docking and castration (198). However, only one FAU (orbital tightening) differed in tail-docked piglets, pre- and post-procedure, and no FAUs differed for castrated piglets.
Another approach is to look at tests that involve motivational trade-offs. A recent study using lame sows trained to retrieve food rewards (113) and another study investigating how long castrated piglets take to navigate a handling chute (105) go some way to investigating a motivational trade-off in relation to pain. This approach could be investigated further in pigs, adapting examples of behavioral tests used in rodent models of pain, such as the place escape/avoidance paradigm (PEAP) (17), the “Escape test” (199), or the facial reward/conflict paradigm (200).
This review focuses on measurements reported in experimental studies investigating pain in pigs. However, most await validation, and many require simplification for field application. The use of methodologies established in other species deserves examination in pigs. Tests aimed at investigating affective states have been recently developed in pigs (201–205) and could be used to better assess the severity of pain states associated with livestock production methods. In a recent survey of pig farmers and veterinarians, the majority of respondents disagreed with the statement: “pigs are not as sensitive to pain as humans” (31). Therefore, research is warranted into the causes and consequences of pain in livestock production to evaluate the trade-off between the cost of painful procedures and the longer term welfare benefit or improvement in product quality (1). The increasing use of pigs in biomedical research [e.g., Ref. (5)] is justified because of their similarity with humans. It follows that model refinement and external scientific validity are optimized when the model and the modeled are treated identically, and this includes the effective management of pain. The creation of more clinically applicable pain assessment methods is a prerequisite to the burgeoning use of pigs in translational research.
SI did the majority of the writing and communicated with the coauthors to coordinate the document editing. RC provided advice on the biomedical research input to the review article and performed significant edits to the document. PG wrote a large part of the quantitative sensory testing section and provided advice and edits on the whole document. KR provided significant advice on the document structure, content, and editing, as this document evolved to its current form.
The authors declare that the research was conducted in the absence of any commercial or financial relationships that could be construed as a potential conflict of interest.
The authors are grateful to BBSRC and Zoetis for funding the first authors’ PhD project, “Addressing Pain at Parturition in the Pig” (BB/J500549/1). This review is adapted from the review of the literature in the first authors PhD thesis (206).
1. Sutherland MA. Welfare implications of invasive piglet husbandry procedures, methods of alleviation and alternatives: a review. N Z Vet J (2015) 63:52–7. doi: 10.1080/00480169.2014.961990
2. Dzikamunhenga RS, Anthony R, Coetzee J, Gould S, Johnson A, Karriker L, et al. Pain management in the neonatal piglet during routine management procedures. Part 1: a systematic review of randomized and non-randomized intervention studies. Anim Health Res Rev (2014) 15:14–38. doi:10.1017/S1466252314000061
3. O’Connor A, Anthony R, Bergamasco L, Coetzee J, Gould S, Johnson AK, et al. Pain management in the neonatal piglet during routine management procedures. Part 2: grading the quality of evidence and the strength of recommendations. Anim Health Res Rev (2014) 15:39–62. doi:10.1017/S1466252314000073
4. O’Connor A, Anthony R, Bergamasco L, Coetzee JF, Dzikamunhenga RS, Johnson AK, et al. Review: assessment of completeness of reporting in intervention studies using livestock: an example from pain mitigation interventions in neonatal piglets. Animal (2015) 10(4):1–11. doi:10.1017/S1751731115002323
5. Bradbury AG, Eddleston M, Clutton RE. Pain management in pigs undergoing experimental surgery; a literature review (2012-4). Br J Anaesth (2015) 116:37–45. doi:10.1093/bja/aev301
6. Mozzachio K, Tynes VV. Recognition and treatment of pain in pet pigs. In: Egger CM, Love L, Doherty T, editors. Pain Management in Veterinary Practice. Chichester, UK: John Wiley & Sons Ltd (2013). p. 383–9.
7. Flor H, Bushnell MC. Central imaging of pain. In: Hunt S, Koltzenburg M, editors. The Neurobiology of Pain. Oxford University Press (2005). p. 311–31.
8. Melzack R, Casey KL. Sensory, Motivational, and Central Control Determinants of Pain. A New Conceptual Model. Ski. Senses. Charles C. Thomas Publisher (1968). p. 423–39.
10. Schiavenato M, Craig KD. Pain assessment as a social transaction: beyond the “gold standard”. Clin J Pain (2010) 26:667–76. doi:10.1097/AJP.0b013e3181e72507
11. Charlton JE. Core Curriculum for Professional Education in Pain: A Report of the Task Force on Professional Education of the International Association for the Study of Pain. 3rd ed. Seattle: IASP Press (2005).
12. Merskey H. A need of a taxonomy. Pain terms: a list with definitions and notes on usage. Pain (1979) 6:247–52. doi:10.1016/0304-3959(79)90046-0
13. Bateson P. Assessment of pain in animals. Anim Behav (1991) 42:827–39. doi:10.1016/S0003-3472(05)80127-7
14. Sneddon LU, Elwood RW, Adamo SA, Leach MC. Defining and assessing animal pain. Anim Behav (2014) 97:201–12. doi:10.1016/j.anbehav.2014.09.007
15. Morton D, Griffiths P. Guidelines on the recognition of pain, distress and discomfort in experimental animals and an hypothesis for assessment. Vet Rec (1985) 116:431–6. doi:10.1136/vr.116.16.431
16. D’Amour FE, Smith DL. A method for determining loss of pain sensation. J Pharmacol Exp Ther (1941) 72:74–9.
17. Fuchs PN, McNabb CT. The place escape/avoidance paradigm: a novel method to assess nociceptive processing. J Integr Neurosci (2012) 11:61. doi:10.1142/S0219635212500045
18. Mokkink LB, Terwee CB, Patrick DL, Alonso J, Stratford PW, Knol DL, et al. The COSMIN Checklist Manual COSMIN. EMGO Institute for Health and Care Research (2012). 56 p.
19. Scott EM, Fitzpatrick JL, Nolan AM, Reid J, Wiseman ML. Evaluation of welfare state based on interpretation of multiple indices. Anim Welf (2003) 12:457–68.
20. Edwards SA, von Borell E, Bonneau M. Guest editorial: scientific and practical issues associated with piglet castration. Animal (2009) 3:1478. doi:10.1017/S1751731109990760
21. Mellor DJ, Cook CJ, Stafford KJ. Quantifying some responses to pain as a stressor. In: Moberg GP, Mench JA, editors. The Biology of Animal Stress: Basic Principles and Implications for Animal Welfare. Oxford: CAB International (2000). p. 171–98.
22. Hunter EJ, Jones TA, Guise HJ, Penny RH, Hoste S. The relationship between tail biting in pigs, docking procedure and other management practices. Vet J (2001) 161:72–9. doi:10.1053/tvjl.2000.0520
23. Prunier A, Bonneau M, Cinotti S, Gunn M, Fredriksen B, Giersing M, et al. A review of the welfare consequences of surgical castration in piglets and the evaluation of non-surgical methods. Anim Welf (2006) 15:277–89.
25. Fraser D. The “teat order” of suckling pigs: II. Fighting during suckling and the effects of clipping the eye teeth. J Agric Sci (1975) 84:393. doi:10.1017/S002185960005259X
26. Leslie E, Hernández-Jover M, Newman R, Holyoake P. Assessment of acute pain experienced by piglets from ear tagging, ear notching and intraperitoneal injectable transponders. Appl Anim Behav Sci (2010) 127:86–95. doi:10.1016/j.applanim.2010.09.006
27. Oldham JG. Clinical measurement of pain, distress and discomfort in pigs. In: Gibson TE, editor. The Detection and Relief of Pain in Animals. London: BVA Animal Welfare Foundation (1985). p. 88–90.
28. MacArthur Clark J. Pain and pain management – overview pain management: an international perspective. Sci Assess Manag Anim pain, OIE Technical Series (2008) 10:11–25.
29. Whay H, Main D, Green L, Webster A. Animal-based measures for the assessment of welfare state of dairy cattle, pigs and laying hens: consensus of expert opinion. Anim Welf (2003) 12:205–17.
30. Kilbride AL, Gillman CE, Green LE. A cross-sectional study of the prevalence of lameness in finishing pigs, gilts and pregnant sows and associations with limb lesions and floor types on commercial farms in England. Anim Welf (2009) 18:215–24.
31. Ison SH, Rutherford KMD. Attitudes of farmers and veterinarians towards pain and the use of pain relief in pigs. Vet J (2014) 202:622–7. doi:10.1016/j.tvjl.2014.10.003
32. Anil SS, Anil L, Deen J. Effect of lameness on sow longevity. J Am Vet Med Assoc (2009) 235:734–8. doi:10.2460/javma.235.6.734
33. Stavrakakis S, Guy JH, Warlow OME, Johnson GR, Edwards SA. Longitudinal gait development and variability of growing pigs reared on three different floor types. Animal (2014) 8:338–46. doi:10.1017/S175173111300222X
35. Muirhead MR, Alexander TJL. Recognising and Treating Pig Disease. Sheffield: 5M Enterprises Ltd (1998).
36. Jørgensen B, Arnbjerg J, Aaslyng M. Pathological and radiological investigations on osteochondrosis in pigs, associated with leg weakness. Zentralbl Veterinarmed A (1995) 42:489–504. doi:10.1111/j.1439-0442.1995.tb00404.x
37. Jørgensen B, Andersen S. Genetic parameters for osteochondrosis in Danish Landrace and Yorkshire boars and correlations with leg weakness and production traits. Anim Sci (2000) 71:427–34. doi:10.1017/S1357729800055442
38. Quinn AJ, Green LE, Lawlor PG, Boyle LA. The effect of feeding a diet formulated for developing gilts between 70kg and ~140kg on lameness indicators and carcass traits. Livest Sci (2015) 174:87–95. doi:10.1016/j.livsci.2014.12.016
39. Karriker LA, Abell CE, Pairis-Garcia MD, Holt WA, Sun G, Coetzee JF, et al. Validation of A lameness model in sows using physiological and mechanical measurements. J Anim Sci (2013) 91:130–6. doi:10.2527/jas.2011-4994
40. Tong C, Conklin DR, Liu B, Ririe DG, Eisenach JC. Assessment of behavior during labor in rats and effect of intrathecal morphine. Anesthesiology (2008) 108:1081–6. doi:10.1097/ALN.0b013e318167afb3
41. Catheline G, Touquet B, Besson JM, Lombard MC. Parturition in the rat: a physiological pain model. Anesthesiology (2006) 104:1257–65. doi:10.1097/00000542-200606000-00022
42. Mirza FG, Fakhoury AA, Rowley TJ, Flood PD. Role of capsaicin in a murine model of labor and delivery. Anesthesiology (2013) 118:430–5. doi:10.1097/ALN.0b013e31827e8765
43. Mainau E, Manteca X. Pain and discomfort caused by parturition in cows and sows. Appl Anim Behav Sci (2011) 135:241–51. doi:10.1016/j.applanim.2011.10.020
44. Deussen AR, Ashwood P, Martis R. Analgesia for relief of pain due to uterine cramping/involution after birth. Cochrane Database Syst Rev (2011) 11:CD004908. doi:10.1002/14651858.CD004908.pub2
45. Ison SH, Jarvis S, Rutherford KMD. The identification of potential behavioural indicators of pain in periparturient sows. Res Vet Sci (2016) 109:114–20. doi:10.1016/j.rvsc.2016.10.002
46. Dobromylskyj P, Flecknell P, Lascelles B, Pascoe P, Taylor P, Waterman-Pearson A. Management of postoperative and other acute pain. Pain Manag Anim (2000) 13:81–146. doi:10.1016/B978-0-7020-1767-4.50008-4
47. Jackson PG. Dystocia in the Sow. Handbook of Veterinary Obstetrics. 2nd ed. London: Elsevier Ltd (2004). p. 129–40.
48. Ison SH, Jarvis S, Rutherford KMD. A survey of sow management at farrowing in the UK. Anim Welf (2016) 25:309–17. doi:10.7120/09627286.25.3.309
49. Algers B. Applying science to animal welfare: injury and disease. Global Conference on Animal Welfare: An OIE Initiative. Paris (2004). p. 179–88.
50. Petersen HH, Nielsen E, Hassing A, Ersbøll AK, Nielsen JP. Prevalence of clinical signs of disease in Danish finisher pigs. Vet Rec (2008) 162:377. doi:10.1136/vr.162.12.377
51. Weber N, Nielsen JP, Jakobsen AS, Pedersen LL, Hansen CF, Pedersen KS. Occurrence of diarrhoea and intestinal pathogens in non-medicated nursery pigs. Acta Vet Scand (2015) 57:64. doi:10.1186/s13028-015-0156-5
52. Correia-Gomes C, Smith RP, Eze JI, Henry MK, Gunn GJ, Williamson S, et al. Pig abattoir inspection data: can it be used for surveillance purposes? PLoS One (2016) 11:e0161990. doi:10.1371/journal.pone.0161990
53. Harley S, More S, Boyle L, Connell NO, Hanlon A. Good animal welfare makes economic sense: potential of pig abattoir meat inspection as a welfare surveillance tool. Ir Vet J (2012) 65:11. doi:10.1186/2046-0481-65-11
54. Clegg SR, Sullivan LE, Bell J, Blowey RW, Carter SD, Evans NJ. Detection and isolation of digital dermatitis treponemes from skin and tail lesions in pigs. Res Vet Sci (2016) 104:64–70. doi:10.1016/j.rvsc.2015.12.003
55. Herskin MS, Bonde MK, Jørgensen E, Jensen KH. Decubital shoulder ulcers in sows: a review of classification, pain and welfare consequences. Animal (2010) 5:757–66. doi:10.1017/S175173111000203X
56. Quinn AJ, Boyle LA, KilBride AL, Green LE. A cross-sectional study on the prevalence and risk factors for foot and limb lesions in piglets on commercial farms in Ireland. Prev Vet Med (2015) 119:162–71. doi:10.1016/j.prevetmed.2015.02.016
57. Gillman CE, KilBride AL, Ossent P, Green LE. A cross-sectional study of the prevalence of foot lesions in post-weaning pigs and risks associated with floor type on commercial farms in England. Prev Vet Med (2009) 91:146–52. doi:10.1016/j.prevetmed.2009.05.023
58. KilBride AL, Gillman CE, Green LE. A cross sectional study of the prevalence, risk factors and population attributable fractions for limb and body lesions in lactating sows on commercial farms in England. BMC Vet Res (2009) 5:30. doi:10.1186/1746-6148-5-31
59. Kilbride AL, Gillman CE, Green LE. A cross-sectional study of prevalence and risk factors for foot lesions and abnormal posture in lactating sows on commercial farms in England. Anim Welf (2010) 19:473–80.
60. Schild SLA, Rousing T, Jensen HE, Barington K, Herskin MS. Do umbilical outpouchings affect the behaviour or clinical condition of pigs during 6h housing in a pre-transport pick-up facility? Res Vet Sci (2015) 101:126–31. doi:10.1016/j.rvsc.2015.06.005
61. Barington K, Dich-Jørgensen K, Jensen HE. A retrospective study of forensic cases of skin ulcerations in Danish pigs from 2000 to 2014. Acta Vet Scand (2016) 58:1–5. doi:10.1186/s13028-016-0229-0
62. Swaby H, Gregory NG. A note on the frequency of gastric ulcers detected during post-mortem examination at a pig abattoir. Meat Sci (2012) 90:269–71. doi:10.1016/j.meatsci.2011.06.015
63. Gigliuto C, De Gregori M, Malafoglia V, Raffaeli W, Compagnone C, Visai L, et al. Pain assessment in animal models: do we need further studies? J Pain Res (2014) 7:227–36. doi:10.2147/JPR.S59161
64. Wemelsfelder F, van Putten G. Behaviour as a Possible Indicator for Pain in Piglets. Report. Res. Inst. Anim. Prod. Zeist: Instituut voor Veeteeltkundig Onderzoek’Schoonoord (1985).
65. Mohling CM, Johnson AK, Coetzee JF, Karriker LA, Abell CE, Millman ST, et al. Kinematics as objective tools to evaluate lameness phases in multiparous sows. Livest Sci (2014) 165:120–8. doi:10.1016/j.livsci.2014.04.031
66. Noonan G, Rand J, Priest J, Ainscow J, Blackshaw J. Behavioural observations of piglets undergoing tail docking, teeth clipping and ear notching. Appl Anim Behav Sci (1994) 39:203–13. doi:10.1016/0168-1591(94)90156-2
67. Rutherford KMD, Robson SK, Donald RD, Jarvis S, Sandercock DA, Scott EM, et al. Pre-natal stress amplifies the immediate behavioural responses to acute pain in piglets. Biol Lett (2009) 5:452–4. doi:10.1098/rsbl.2009.0175
68. Sutherland MA, Bryer PJ, Krebs N, McGlone JJ. Tail docking in pigs: acute physiological and behavioural responses. Animal (2008) 2:292–7. doi:10.1017/S1751731107001450
69. Torrey S, Devillers N, Lessard M, Farmer C, Widowski T. Effect of age on the behavioral and physiological responses of piglets to tail docking and ear notching. J Anim Sci (2009) 87:1778–86. doi:10.2527/jas.2008-1354
70. Hay M, Vulin A, Génin S, Sales P, Prunier A. Assessment of pain induced by castration in piglets: behavioral and physiological responses over the subsequent 5 days. Appl Anim Behav Sci (2003) 82:201–18. doi:10.1016/S0168-1591(03)00059-5
71. Sutherland MA, Davis BL, Brooks TA, McGlone JJ. Physiology and behavior of pigs before and after castration: effects of two topical anesthetics. Animal (2010) 4:2071–9. doi:10.1017/S1751731110001291
72. Sutherland MA, Davis BL, McGlone JJ. The effect of local or general anesthesia on the physiology and behavior of tail docked pigs. Animal (2011) 5:1237–46. doi:10.1017/S175173111100019X
73. Larsen T, Kaiser M, Herskin MS. Does the presence of shoulder ulcers affect the behaviour of sows. Res Vet Sci (2015) 98:19–24. doi:10.1016/j.rvsc.2014.11.001
74. Meijer E, Oosterlinck M, van Nes A, Back W, van der Staay FJ. Pressure mat analysis of naturally occurring lameness in young pigs after weaning. BMC Vet Res (2014) 10:193. doi:10.1186/s12917-014-0193-8
75. Hansson M, Lundeheim N, Nyman G, Johansson G. Effect of local anaesthesia and/or analgesia on pain responses induced by piglet castration. Acta Vet Scand (2011) 53:34–43. doi:10.1186/1751-0147-53-34
76. Rault JL, Lay DC. Nitrous oxide by itself is insufficient to relieve pain due to castration in piglets. J Anim Sci (2011) 89:3318–25. doi:10.2527/jas.2011-4104
77. Van Beirendonck S, Driessen B, Verbeke G, Geers R. Behavior of piglets after castration with or without carbon dioxide anesthesia. J Anim Sci (2011) 89:3310–7. doi:10.2527/jas.2010-3104
78. Keita A, Pagot E, Prunier A, Guidarini C. Pre-emptive meloxicam for postoperative analgesia in piglets undergoing surgical castration. Vet Anaesth Analg (2010) 37:367–74. doi:10.1111/j.1467-2995.2010.00546.x
79. Meijer E, van Nes A, Back W, van der Staay FJ. Clinical effects of buprenorphine on open field behaviour and gait symmetry in healthy and lame weaned piglets. Vet J (2015) 206:298–303. doi:10.1016/j.tvjl.2015.10.016
80. Pairis-Garcia MD, Johnson AK, Stalder KJ, Karriker LA, Coetzee JF, Millman ST. Measuring the efficacy of flunixin meglumine and meloxicam for lame sows using nociceptive threshold tests. Anim Welf (2014) 23:219–29. doi:10.7120/09627286.23.2.219
81. Tapper KR, Johnson AK, Karriker LA, Stalder KJ, Parsons RL, Wang C, et al. Pressure algometry and thermal sensitivity for assessing pain sensitivity and effects of flunixin meglumine and sodium salicylate in a transient lameness model in sows. Livest Sci (2013) 157:245–53. doi:10.1016/j.livsci.2013.07.017
82. Conte S, Bergeron R, Gonyou H, Brown J, Rioja-Lang FC, Connor ML, et al. Use of an analgesic to identify pain-related indicators of lameness in sows. Livest Sci (2015) 180:203–8. doi:10.1016/j.livsci.2015.08.009
83. Mogil JS. Animal models of pain: progress and challenges. Nat Rev Neurosci (2009) 10:283–94. doi:10.1038/nrn2606
84. Conte S, Bergeron R, Gonyou H, Brown J, Rioja-Lang FC, Connor L, et al. Measure and characterization of lameness in gestating sows using force plate, kinematic, and accelerometer methods. J Anim Sci (2014) 92:5693–703. doi:10.2527/jas.2014-7865
85. Grégoire J, Bergeron R, D’Allaire S, Meunier-Salaün MC, Devillers N. Assessment of lameness in sows using gait, footprints, postural behaviour and foot lesion analysis. Animal (2013) 7:1163–73. doi:10.1017/S1751731113000098
86. Whalin L, Pairis-Garcia M, Proudfoot K, Stalder K, Johnson A. Validating behavioral sampling techniques for lame sows administered flunixin meglumine and meloxicam. Livest Sci (2016) 191:103–7. doi:10.1016/j.livsci.2016.07.017
87. Leidig M, Hertrampf B, Failing K, Schumann A, Reiner G. Pain and discomfort in male piglets during surgical castration with and without local anaesthesia as determined by vocalisation and defence behaviour. Appl Anim Behav Sci (2009) 116:174–8. doi:10.1016/j.applanim.2008.10.004
88. Marchant-Forde JN, Lay DC, McMunn KA, Cheng HW, Pajor EA, Marchant-Forde RM. Postnatal piglet husbandry practices and well-being: the effects of alternative techniques delivered separately. J Anim Sci (2009) 87:1479–92. doi:10.2527/jas.2008-1080
89. Walker B, Jäggin N, Doherr M, Schatzmann U. Inhalation anaesthesia for castration of newborn piglets: experiences with isoflurane and isoflurane/NO. J Vet Med A Physiol Pathol Clin Med (2004) 51:150–4. doi:10.1111/j.1439-0442.2004.00617.x
90. Parsons RL, Millman ST, Coetzee JF, Karriker LA, Mohling CM, Pairis-Garcia MD, et al. Sow behavioral responses to transient, chemically induced synovitis lameness. Acta Agric Scand Sec A Anim Sci (2015) 65:122–5. doi:10.1080/09064702.2015.1110617
91. Taylor A, Weary D, Lessard M, Braithwaite L. Behavioural responses of piglets to castration: the effect of piglet age. Appl Anim Behav Sci (2001) 73:35–43. doi:10.1016/S0168-1591(01)00123-X
92. McGlone JJ, Nicholson RI, Hellman JM, Herzog DN. The development of pain in young pigs associated with castration and attempts to prevent castration-induced behavioral changes. J Anim Sci (1993) 71:1441–6.
93. Llamas Moya S, Boyle L, Lynch P, Arkins S. Effect of surgical castration on the behavioural and acute phase responses of 5-day-old piglets. Appl Anim Behav Sci (2008) 111:133–45. doi:10.1016/j.applanim.2007.05.019
94. Lonardi C, Scollo A, Normando S, Brscic M, Gottardo F. Can novel methods be useful for pain assessment of castrated piglets? Animal (2015) 9:871–7. doi:10.1017/S1751731114003176
95. Tenbergen R, Friendship R, Cassar G, Amezcua MR, Haley D. Investigation of the use of meloxicam post farrowing for improving sow performance and reducing pain. J Swine Heal Prod (2014) 22:10–5.
96. Viitasaari E, Raekallio M, Heinonen M, Valros A, Peltoniemi O, Hänninen L. The effect of ketoprofen on post-partum behaviour in sows. Appl Anim Behav Sci (2014) 158:16–22. doi:10.1016/j.applanim.2014.06.005
97. Mainau E, Ruiz-de-la-Torre JL, Dalmau A, Salleras JM, Manteca X. Effects of meloxicam (Metacam®) on post-farrowing sow behaviour and piglet performance. Animal (2012) 6:494–501. doi:10.1017/S1751731111001790
98. Pairis-Garcia MD, Johnson AK, Stalder KJ, Abell CA, Karriker LA, Coetzee JF, et al. Behavioural evaluation of analgesic efficacy for pain mitigation in lame sows. Anim Welf (2015) 24:93–9. doi:10.7120/09627286.24.1.093
99. Mainau E, Dalmau A, Ruiz-de-la-Torre JL, Manteca X. A behavioural scale to measure ease of farrowing in sows. Theriogenology (2010) 74:1279–87. doi:10.1016/j.theriogenology.2010.05.034
100. Haussmann MF, Lay DC, Buchanan HS, Hopper JG. Butorphanol tartrate acts to decrease sow activity, which could lead to reduced pig crushing. J Anim Sci (1999) 77(8):2054–9. doi:10.2527/1999.7782054x
101. Jarvis S, Lawrence AB, Mclean KA, Deans LA, Chirnside J, Calvert SK. The effect of piglet expulsion in the sow on plasma cortisol, adrenocorticotropic hormone and β-endorphin. Reprod Domest Anim (1999) 34:89–94. doi:10.1111/j.1439-0531.1999.tb01389.x
102. Lawrence AB, Petherick JC, McLean K, Gilbert CL, Chapman C, Russell JA. Naloxone prevents interruption of parturition and increases plasma oxytocin following environmental disturbance in parturient sows. Physiol Behav (1992) 52:917–23. doi:10.1016/0031-9384(92)90371-8
103. Mcglone JJ, Hellman JM. Local and general anesthetic effects on behavior and performance of two- and seven-week-old castrated and uncastrated piglets. J Anim Sci (1988) 66:3049–58. doi:10.2527/jas1988.66123049x
104. Caroll JA, Berg EL, Strauch TA, Roberts MP, Kattesh HG. Hormonal profiles, behavioral responses, and short-term growth performance after castration of pigs at three, six, nine, or twelve days of age. J Anim Sci (2006) 84:1271–8. doi:10.2527/2006.8451271x
105. Bilsborrow K, Seddon YM, Brown J, Waldner C, Stookey MS. An investigation of a novel behavioural test to assess pain in piglets following castration. Can J Anim Sci (2016) 96(3):376–85. doi:10.1139/cjas-2015-0109
106. Schmidt T, König A, von Borell E. Impact of general injection anaesthesia and analgesia on post-castration behaviour and teat order of piglets. Animal (2012) 6:1998–2002. doi:10.1017/S1751731112001334
107. Viitasaari E, Hänninen L, Heinonen M, Raekallio M, Orro T, Peltoniemi O, et al. Effects of post-partum administration of ketoprofen on sow health and piglet growth. Vet J (2013) 198:153–7. doi:10.1016/j.tvjl.2013.06.013
108. Swinkels J, Pijpers A, Vernooy J, Van Nes A, Verheijden J. Effects of ketoprofen and flunixin in pigs experimentally infected with Actinobacillus pleuropneuniae. J Vet Pharmacol Ther (1994) 17:299–303. doi:10.1111/j.1365-2885.1994.tb00249.x
109. Hirsch AC, Philipp H, Kleemann R. Investigation on the efficacy of meloxicam in sows with mastitis-metritis-agalactia syndrome. J Vet Pharmacol Ther (2003) 26:355–60. doi:10.1046/j.1365-2885.2003.00524.x
110. Harvey-Clark CJ, Gilespie K, Riggs KW. Transdermal fentanyl compared with parenteral buprenorphine in post-surgical pain in swine: a case study. Lab Anim (2000) 34:386–98. doi:10.1258/002367700780387750
111. Malavasi LM, Nyman G, Augustsson H, Jacobson M, Jensen-Waern M. Effects of epidural morphine and transdermal fentanyl analgesia on physiology and behaviour after abdominal surgery in pigs. Lab Anim (2006) 40:16–27. doi:10.1258/002367706775404453
112. Royal JM, Settle TL, Bodo M, Lombardini E, Kent ML, Upp J, et al. Assessment of postoperative analgesia after application of ultrasound-guided regional anesthesia for surgery in a swine femoral fracture model. J Am Assoc Lab Anim Sci (2013) 52:265–76.
113. Bos EJ, Nalon E, Maes D, Ampe B, Buijs S, van Riet MMJ, et al. Effect of locomotion score on sows’ performances in a feed reward collection test. Animal (2015) 9:1698–703. doi:10.1017/S1751731115001275
114. Viitasaari E, Raekallio M, Valros A, Peltoniemi O, Hänninen L, Heinonen M. The effect of ketoprofen on feeding behavior of tail-bitten pigs. Porc Heal Manag (2015) 1(11):1–7. doi:10.1186/s40813-015-0005-y
115. Murison PJ, Jones A, Mitchard L, Burt R, Birchall MA. Development of perioperative care for pigs undergoing laryngeal transplantation: a case series. Lab Anim (2009) 43:338–43. doi:10.1258/la.2009.008101
116. Castel D, Willentz E, Doron O, Brenner O, Meilin S. Characterization of a porcine model of post-operative pain. Eur J Pain (2014) 18:1–10. doi:10.1002/j.1532-2149.2013.00399.x
117. Reyes L, Tinworth KD, Li KM, Yau DF, Waters KA. Observer-blinded comparison of two nonopioid analgesics for postoperative pain in piglets. Pharmacol Biochem Behav (2006) 73:521–8. doi:10.1016/S0091-3057(02)00820-1
118. Dalmau A, Geverink NA, Van Nuffel A, van Steenbergen L, Van Reenen K, Hautekiet V, et al. Repeatability of lameness, fear and slipping scores to assess animal welfare upon arrival in pig slaughterhouses. Animal (2010) 4:804. doi:10.1017/S1751731110000066
119. Zoric M, Nilsson E, Lundeheim N, Wallgren P. Incidence of lameness and abrasions in piglets in identical farrowing pens with four different types of floor. Acta Vet Scand (2009) 51:23. doi:10.1186/1751-0147-51-23
120. Jensen TB, Bonde MK, Kongsted AG, Toft N, Sørensen JT. The interrelationships between clinical signs and their effect on involuntary culling among pregnant sows in group-housing systems. Animal (2010) 4:1922–8. doi:10.1017/S1751731110001102
121. Courboulay V, Delarue E. Welfare assessment in 82 pig farms: effect of animal age and floor type on. Anim Welf (2009) 18:515–21.
122. Friton G, Philipp H, Schneider T, Kleeman R. Investigation on the clinical efficacy and safety of meloxicam (Metacam) in the treatment of non-infectious locomotor disorders in pigs. Berl Munch Tierarztl Wochenschr (2003) 116:421–6.
123. Mustonen K, Ala-Kurikka E, Orro T, Peltoniemi O, Raekallio M, Vainio O, et al. Oral ketoprofen is effective in the treatment of non-infectious lameness in sows. Vet J (2011) 190:55–9. doi:10.1016/j.tvjl.2010.09.017
124. Main DC, Clegg J, Spatz A, Green LE. Repeatability of a lameness scoring system for finishing pigs. Vet Rec (2000) 147:574–6. doi:10.1136/vr.147.20.574
125. Weary DM, Niel L, Flower FC, Fraser D. Identifying and preventing pain in animals. Appl Anim Behav Sci (2006) 100:64–76. doi:10.1016/j.applanim.2006.04.013
126. D’Eath RB. Repeated locomotion scoring of a sow herd to measure lameness: consistency over time, the effect of sow characteristics and inter-observer reliability. Anim Welf (2012) 21:219–31. doi:10.7120/09627286.21.2.219
127. Loeser JD, Treede RD. The Kyoto protocol of IASP basic pain terminology. Pain (2008) 137:473–7. doi:10.1016/j.pain.2008.04.025
128. Jarvis S, McLean KA, Chirnside J, Deans LA, Calvert SK, Molony V, et al. Opioid-mediated changes in nociceptive threshold during pregnancy and parturition in the sow. Pain (1997) 72:153–9. doi:10.1016/S0304-3959(97)00027-4
129. Herskin MS, Ladewig J, Arendt-Nielsen L. Measuring cutaneous thermal nociception in group-housed pigs using laser technique – effects of laser power output. Appl Anim Behav Sci (2009) 118:144–51. doi:10.1016/j.applanim.2009.02.016
131. Rushen J, De Passillé AM, Schouten W. Stereotypic behavior, endogenous opioids, and postfeeding hypoalgesia in pigs. Physiol Behav (1990) 48:91–6. doi:10.1016/0031-9384(90)90267-8
132. Rushen J, Ladewig J. Stress-induced hypoalgesia and opioid inhibition of pigs’ responses to restraint. Physiol Behav (1991) 50:1093–6. doi:10.1016/0031-9384(91)90566-7
133. Rushen J, Foxcroft G, De Passillé AM. Nursing-induced changes in pain sensitivity, prolactin, and somatotropin in the pig. Physiol Behav (1993) 53:265–70. doi:10.1016/0031-9384(93)90203-R
134. Herskin M, Rasmussen J. Pigs in pain – porcine behavioural responses towards mechanical nociceptive stimulation directed at the hind legs. J Pain (2010) 1:175–6. doi:10.1016/j.sjpain.2010.05.026
135. Sandercock DA, Gibson IF, Rutherford KMD, Donald RD, Lawrence AB, Brash HM, et al. The impact of prenatal stress on basal nociception and evoked responses to tail-docking and inflammatory challenge in juvenile pigs. Physiol Behav (2011) 104:728–37. doi:10.1016/j.physbeh.2011.07.018
136. Fosse TK, Toutain PL, Spadavecchia C, Haga HA, Horsberg TE, Ranheim B. Ketoprofen in piglets: enantioselective pharmacokinetics, pharmacodynamics and PK/PD modelling. J Vet Pharmacol Ther (2011) 34:338–49. doi:10.1111/j.1365-2885.2010.01236.x
137. Nalon E, Maes D, Piepers S, van Riet MMJ, Janssens GPJ, Millet S, et al. Mechanical nociception thresholds in lame sows: evidence of hyperalgesia as measured by two different methods. Vet J (2013) 198:386–90. doi:10.1016/j.tvjl.2013.08.016
138. Mohling CM, Johnson AK, Coetzee JF, Karriker LA, Stalder KJ, Abell CE, et al. Evaluation of mechanical and thermal nociception as objective tools to measure painful and nonpainful lameness phases in multiparous sows. J Anim Sci (2014) 92:3073–81. doi:10.2527/jas.2013-7521
139. Janczak AM, Ranheim B, Fosse TK, Hild S, Nordgreen J, Moe RO, et al. Factors affecting mechanical (nociceptive) thresholds in piglets. Vet Anaesth Analg (2012) 39:628–35. doi:10.1111/j.1467-2995.2012.00737.x
140. Di Giminiani P, Petersen LJ, Herskin MS. Nociceptive responses to thermal and mechanical stimulations in awake pigs. Eur J Pain (2013) 17:638–48. doi:10.1002/j.1532-2149.2012.00228.x
141. Di Giminiani P, Stausholm JS, Viitasaari E, Petersen LJ, Herskin MS. The effect of social isolation, gender and familiarity with the experimental procedure on tests of porcine nociceptive thresholds. Vet Anaesth Analg (2015) 42:648–56. doi:10.1111/vaa.12254
142. Di Giminiani P, Petersen LJ, Herskin MS. Capsaicin-induced neurogenic inflammation in pig skin: a behavioural study. Res Vet Sci (2014) 96:447–53. doi:10.1016/j.rvsc.2014.03.023
143. Di Giminiani P, Petersen LJ, Herskin MS. Characterization of nociceptive behavioural responses in the awake pig following UV-B-induced inflammation. Eur J Pain (2014) 18:20–8. doi:10.1002/j.1532-2149.2013.00340.x
144. Castel D, Sabbag I, Brenner O, Meilin S. Peripheral neuritis trauma in pigs: a neuropathic pain model. J Pain (2016) 17:36–49. doi:10.1016/j.jpain.2015.09.011
145. Brown JD, Saees M, Do L, Braz J, Basbaum AI, Iadarola MJ, et al. CT-guided injection of a TRPV1 agonist around dorsal root ganglia decreases pain transmission in swine. Sci Transl Med (2015) 7:1–9. doi:10.1126/scitranslmed.aac6589
146. Roughan JV, Flecknell PA. Evaluation of a short duration behaviour-based post-operative pain scoring system in rats. Eur J Pain (2003) 7:397–406. doi:10.1016/S1090-3801(02)00140-4
147. Mogil JS. Social modulation of and by pain in humans and rodents. Pain (2015) 156:S35–41. doi:10.1097/01.j.pain.0000460341.62094.77
148. Reimert I, Bolhuis JE, Kemp B, Rodenburg TB. Social support in pigs with different coping styles. Physiol Behav (2014) 129:221–9. doi:10.1016/j.physbeh.2014.02.059
149. Reimert I, Rodenburg TB, Ursinus WW, Kemp B, Bolhuis JE. Responses to novel situations of female and castrated male pigs with divergent social breeding values and different backtest classifications in barren and straw-enriched housing. Appl Anim Behav Sci (2014) 151:24–35. doi:10.1016/j.applanim.2013.11.015
150. Reimert I, Bolhuis JE, Kemp B, Rodenburg TB. Indicators of positive and negative emotions and emotional contagion in pigs. Physiol Behav (2013) 109:42–50. doi:10.1016/j.physbeh.2012.11.002
151. Dobromylskyj P, Fleckenell P, Lascelles A, Livingston P, Taylor P, Waterman-Pearson A. Pain assessment. In: Flecknell PA, Waterman-Pearson A, editors. Pain Management in Animals. London: Harcourt Publishers Limited (2000). p. 53–80.
152. White RG, DeShazer JA, Tressler CJ, Borcher GM, Davey S, Waninge A, et al. Vocalization and physiological response of pigs during castration with or without a local anesthetic. J Anim Sci (1995) 73:381–6. doi:10.2527/1995.732381x
153. Weary DM, Braithwaite LA, Fraser D. Vocal response to pain in piglets. Appl Anim Behav Sci (1998) 56:161–72. doi:10.1016/S0168-1591(97)00092-0
154. Taylor A, Weary D. Vocal responses of piglets to castration: identifying procedural sources of pain. Appl Anim Behav Sci (2000) 70:17–26. doi:10.1016/S0168-1591(00)00143-X
155. Marx G, Horn T, Thielebein J, Knubel B, von Borell E. Analysis of pain-related vocalization in young pigs. J Sound Vib (2003) 266:687–98. doi:10.1016/S0022-460X(03)00594-7
156. Kluivers-Poodt M, Houx BB, Robben SRM, Koop G, Lambooij E, Hellebrekers LJ. Effects of a local anaesthetic and NSAID in castration of piglets, on the acute pain responses, growth and mortality. Animal (2012) 6:1469–75. doi:10.1017/S1751731112000547
157. SchÖn PC, Puppe B, Manteuffel G. Linear prediction coding analysis and self-organizing feature map as tools to classify stress calls of domestic pigs (Sus scrofa). J Acoust Soc Am (2001) 110:1425–31.
158. Manteuffel G, Schön P. Measuring pig welfare by automatic monitoring of stress calls. Bornimer Agrartech Berichte (2002) 29:110–8.
159. SchÖn P, Puppe B, Manteuffel G. Automated recording of stress vocalisations as a tool to document impaired welfare in pigs. Anim Welf (2004) 13(2):105–10.
160. Puppe B, Schön PC, Tuchscherer A, Manteuffel G. Castration-induced vocalisation in domestic piglets, Sus scrofa: complex and specific alterations of the vocal quality. Appl Anim Behav Sci (2005) 95:67–78. doi:10.1016/j.applanim.2005.05.001
161. Harris JA. Using c-fos as a neural marker of pain. Brain Res Bull (1998) 45:1–8. doi:10.1016/S0361-9230(97)00277-3
162. Svendsen O. Castration of piglets under carbon dioxide (CO2) anaesthesia. J Vet Pharmacol Ther (2006) 1:54–6. doi:10.1111/j.1365-2885.2006.00759_6.x
163. Lykkegaard K, Lauritzen B, Tessem L, Weikop P, Svendsen O. Local anaesthetics attenuates spinal nociception and HPA-axis activation during experimental laparotomy in pigs. Res Vet Sci (2005) 79:245–51. doi:10.1016/j.rvsc.2004.11.017
164. Ochroch EA, Mardini IA, Gottschalk A. What is the role of NSAIDs in pre-emptive analgesia? Drugs (2003) 63:2709–23. doi:10.2165/00003495-200363240-00002
165. O’Connor TM, O’Connell J, O’Brien DI, Goode T, Bredin CP, Shanahan F. The role of substance P in inflammatory disease. J Cell Physiol (2004) 201:167–80. doi:10.1002/jcp.20061
166. Cornefjord M, Olmarker K, Farley DB, Weinstein JN, Rydevik B. Neuropeptide changes in compressed spinal nerve roots. Spine (Phila Pa 1976) (1995) 20:670–3.
167. Sutherland MA, Davis BL, Brooks TA, Coetzee JF. The physiological and behavioral response of pigs castrated with and without anesthesia or analgesia. J Anim Sci (2012) 90:2211–21. doi:10.2527/jas.2011-4260
168. Bates JL, Karriker LA, Stock ML, Pertzborn KM, Baldwin LG, Wulf LW, et al. Impact of transmammary-delivered meloxicam on biomarkers of pain and distress in piglets after castration and tail docking. PLoS One (2014) 9:e113678. doi:10.1371/journal.pone.0113678
169. Coetzee JF, Lubbers BV, Toerber SE, Gehring R, Thomson DU, White BJ, et al. Plasma concentration of substance P and cortisol in beef calves after castration or simulated castration. Am J Vet Res (2008) 69:751–62. doi:10.2460/ajvr.69.6.751
170. Hong HS, Lee J, Lee E, Kwon YS, Lee E, Ahn W, et al. A new role of substance P as an injury-inducible messenger for mobilization of CD29(+) stromal-like cells. Nat Med (2009) 15:425–35. doi:10.1038/nm.1909
171. Prunier A, Mounier AM, Hay M. Effects of castration, tooth resection, or tail docking on plasma metabolites and stress hormones in young pigs. J Anim Sci (2005) 83:216–22. doi:10.2527/2005.831216x
172. Marsálek P, Svoboda M, Smutná M, Blahová J, Vecerek V. Neopterin and biopterin as biomarkers of immune system activation associated with castration in piglets. J Anim Sci (2011) 89:1758–62. doi:10.2527/jas.2010-3157
173. Molokwu ECI, Wagner W. Endocrine physiology of the puerperal sow. J Anim Sci (1973) 36:1158–63.
174. Lawrence AB, Petherick J, McLean K, Deans L, Chirnside J, Gaughan A, et al. The effect of environment on behaviour, plasma cortisol and prolactin in parturient sows. Appl Anim Behav Sci (1994) 39:313–30. doi:10.1016/0168-1591(94)90165-1
175. Jarvis S, Lawrence AB, McLean KA, Deans LA, Chirnside J, Calvert SK. The effect of environment on behavioural activity, ACTH, β-endorphin and cortisol in pre-farrowing gilts. Anim Sci (1997) 65:465–72. doi:10.1017/S1357729800008663
176. Jarvis S, Lawrence AB, McLean KA, Chirnside J, Deans LA, Calvert SK. The effect of environment on plasma cortisol and beta-endorphin in the parturient pig and the involvement of endogenous opioids. Anim Reprod Sci (1998) 52:139–51. doi:10.1016/S0378-4320(98)00090-6
177. Haga HA, Tevik A, Moerch H. Electroencephalographic and cardiovascular indicators of nociception during isoflurane anaesthesia in pigs. Vet Anaesth Analg (2001) 28:126–31. doi:10.1046/j.1467-2987.2001.00051.x
178. Haga HA, Ranheim B. Castration of piglets: the analgesic effects of intratesticular and intrafunicular lidocaine injection. Vet Anaesth Analg (2005) 32:1–9. doi:10.1111/j.1467-2995.2004.00225.x
179. Akil H, Watson SJ, Young E, Lewis ME, Khachaturian H, Walker JM. Endogenous opioids: biology and function. Annu Rev Neurosci (1984) 7:223–55. doi:10.1146/annurev.ne.07.030184.001255
180. Jarvis S, McLean KA, Calvert SK, Deans LA, Chirnside J, Lawrence AB. The responsiveness of sows to their piglets in relation to the length of parturition and the involvement of endogenous opioids. Appl Anim Behav Sci (1999) 63:195–207. doi:10.1016/S0168-1591(99)00013-1
181. Wall PD. On the relation of injury to pain. Pain (1979) 6:253–64. doi:10.1016/0304-3959(79)90047-2
182. Petersen HH, Enøe C, Nielsen EO. Observer agreement on pen level prevalence of clinical signs in finishing pigs. Prev Vet Med (2004) 64:147–56. doi:10.1016/j.prevetmed.2004.05.002
183. Sutherland MA, Bryer PJ, Krebs N, Mcglone JJ. The effect of method of tail docking on tail-biting behaviour and welfare of pigs. Anim Welf (2009) 18(4):561–70.
184. Llamas Moya S, Boyle L, Lynch P, Arkins S. Influence of teeth resection on the skin temperature and acute phase response in newborn piglets. Anim Welfare (2006) 15:291–7.
185. Sorensen NS, Tegtmeier C, Andresen LO, Piñeiro M, Toussaint MJM, Campbell FM, et al. The porcine acute phase protein response to acute clinical and subclinical experimental infection with Streptococcus suis. Vet Immunol Immunopathol (2006) 113:157–68. doi:10.1016/j.vetimm.2006.04.008
186. Friton G, Schmidt H, Schrödl W. Clinical and anti-inflammatory effects of treating endotoxin-challenged pigs with meloxicam. Vet Rec (2006) 159:552.
188. Wilson R, Holyoake P, Cronin G, Doyle R. Managing animal wellbeing: a preliminary survey of pig farmers. Aust Vet J (2014) 92:206–12. doi:10.1111/avj.12169
189. Mogil JS, Crager SE. What should we be measuring in behavioral studies of chronic pain in animals? Pain (2004) 112:12–5. doi:10.1016/j.pain.2004.09.028
190. Kornum BR, Knudsen GM. Cognitive testing of pigs (Sus scrofa) in translational biobehavioral research. Neurosci Biobehav Rev (2011) 35:437–51. doi:10.1016/j.neubiorev.2010.05.004
191. Leach MC, Coulter CA, Richardson CA, Flecknell PA. Are we looking in the wrong place? Implications for behavioural-based pain assessment in rabbits (Oryctolagus cuniculi) and beyond? PLoS One (2011) 6:e13347. doi:10.1371/journal.pone.0013347
192. Langford DJ, Bailey AL, Chanda ML, Clarke SE, Drummond TE, Echols S, et al. Coding of facial expressions of pain in the laboratory mouse. Nat Methods (2010) 7:447–9. doi:10.1038/nmeth.1455
193. Sotocinal SG, Sorge RE, Zaloum A, Tuttle AH, Martin LJ, Wieskopf JS, et al. The Rat Grimace Scale: a partially automated method for quantifying pain in the laboratory rat via facial expressions. Mol Pain (2011) 7:55. doi:10.1186/1744-8069-7-55
194. Keating SCJ, Thomas AA, Flecknell PA, Leach MC. Evaluation of EMLA cream for preventing pain during tattooing of rabbits: changes in physiological, behavioural and facial expression responses. PLoS One (2012) 7:e44437. doi:10.1371/journal.pone.0044437
195. Dalla Costa E, Minero M, Lebelt D, Stucke D, Canali E, Leach MC. Development of the Horse Grimace Scale (HGS) as a pain assessment tool in horses undergoing routine castration. PLoS One (2014) 9:e92281. doi:10.1371/journal.pone.0092281
196. McLennan KM, Rebelo CJB, Corke MJ, Holmes MA, Leach MC, Constantino-Casas F. Development of a facial expression scale using footrot and mastitis as models of pain in sheep. Appl Anim Behav Sci (2016) 176:19–26. doi:10.1016/j.applanim.2016.01.007
197. Guesgen MJ, Beausoleil NJ, Leach M, Minot EO, Stewart M, Stafford KJ. Coding and quantification of a facial expression for pain in lambs. Behav Processes (2016) 132:49–56. doi:10.1016/j.beproc.2016.09.010
198. Di Giminiani P, Brierley VL, Scollo A, Gottardo F, Malcolm M, Edwards SA, et al. The assessment of facial expressions in piglets undergoing tail docking and castration: towards the development of the Piglet Grimace Scale. Front Vet Sci (2016) 3:100. doi:10.3389/fvets.2016.00100
199. Mauderli AP, Acosta-rua A, Vierck CJ. An operant assay of thermal pain in conscious, unrestrained rats. J Neurosci Methods (2000) 97:19–29. doi:10.1016/S0165-0270(00)00160-6
200. Neubert JK, Widmer CG, Malphurs W, Rossi HL, Vierck CJ, Caudle RM. Use of a novel thermal operant behavioral assay for characterization of orofacial pain sensitivity. Pain (2005) 116:386–95. doi:10.1016/j.pain.2005.05.011
201. Douglas C, Bateson M, Walsh C, Bédué A, Edwards SA. Environmental enrichment induces optimistic cognitive biases in pigs. Appl Anim Behav Sci (2012) 139:65–73. doi:10.1016/j.applanim.2012.02.018
202. Düpjan S, Ramp C, Kanitz E, Tuchscherer A, Puppe B. A design for studies on cognitive bias in the domestic pig. J Vet Behav Clin Appl Res (2013) 8:485–9. doi:10.1016/j.jveb.2013.05.007
203. Murphy E, Nordquist RE, van der Staay FJ. Responses of conventional pigs and Göttingen miniature pigs in an active choice judgement bias task. Appl Anim Behav Sci (2013) 148:64–76. doi:10.1016/j.applanim.2013.07.011
204. Murphy E, Nordquist RE, van der Staay FJ. A review of behavioural methods to study emotion and mood in pigs, Sus scrofa. Appl Anim Behav Sci (2014) 159:9–28. doi:10.1016/j.applanim.2014.08.002
205. Murphy E, Kraak L, van den Broek J, Nordquist RE, van der Staay FJ. Decision-making under risk and ambiguity in low-birth-weight pigs. Anim Cogn (2014) 18:561–72. doi:10.1007/s10071-014-0825-1
Keywords: pig, pain, pain assessment, welfare, review
Citation: Ison SH, Clutton RE, Di Giminiani P and Rutherford KMD (2016) A Review of Pain Assessment in Pigs. Front. Vet. Sci. 3:108. doi: 10.3389/fvets.2016.00108
Received: 14 September 2016; Accepted: 15 November 2016;
Published: 28 November 2016
Edited by:
Jeremy N. Marchant-Forde, USDA-ARS Livestock Behavior Research Unit, USAReviewed by:
Yolande Maria Seddon, University of Saskatchewan, CanadaCopyright: © 2016 Ison, Clutton, Di Giminiani and Rutherford. This is an open-access article distributed under the terms of the Creative Commons Attribution License (CC BY). The use, distribution or reproduction in other forums is permitted, provided the original author(s) or licensor are credited and that the original publication in this journal is cited, in accordance with accepted academic practice. No use, distribution or reproduction is permitted which does not comply with these terms.
*Correspondence: Sarah H. Ison, c2hpc29uQG1zdS5lZHU=
†Present address: Sarah H. Ison, Animal Behavior and Welfare, Department of Animal Science, Michigan State University, East Lansing, MI, USA
Disclaimer: All claims expressed in this article are solely those of the authors and do not necessarily represent those of their affiliated organizations, or those of the publisher, the editors and the reviewers. Any product that may be evaluated in this article or claim that may be made by its manufacturer is not guaranteed or endorsed by the publisher.
Research integrity at Frontiers
Learn more about the work of our research integrity team to safeguard the quality of each article we publish.