- Prestage Department of Poultry Science, North Carolina State University, Raleigh, NC, USA
Anti-nutritional factors (ANFs) in feed ingredients can challenge gut health and reduce nutrient utilization. Birds typically activate their innate immune system as a protective response against the adverse effects of ANF, which often involves the secretion of mucin. Although dietary supplementation of exogenous enzymes are commonly used to alleviate the adverse effects of ANF on apparent nutrient digestibility, little is known about how they affect gut health, particularly in relation to the morphological development and mucin secretion of enteric mucosa. We carried out two trials to examine the effect of dietary supplementation of different types of exogenous enzymes on gut health of by accessing the effect of jejunum morphological development and ileal enteric adherent mucin thickness layer in turkeys. Dietary β-mannanase supplementation reduced ileal adherent mucin thickness layer (804 vs 823 μg/g; p < 0.05), while a commercial blend of xylanase, amylase, and protease (XAP) reduced ileal adherent mucin layer thickness (589 vs 740 μg/g; p < 0.05); thus reducing the apparent endogenous loss of nutrients. Both enzyme supplements also affected gut morphological characteristics. In comparison to the control treatment, dietary β-mannanase supplementation improved the jejunum tip width (219 vs 161; p < 0.05), base width (367 vs 300; p < 0.05), surface area (509,870 vs 380, 157; p < 0.05) and villi height/crypt depth ratio (7.49 vs 5.70; p < 0.05), and XAP improved the crypt depth (p < 0.05). In conclusion, dietary supplementation of exogenous enzymes may help alleviate the adverse effects of ANF on nutrient utilization by directly or indirectly removing the mucosal irritation that stimulates enteric mucin secretion.
Introduction
The gut is a very complex and diverse ecosystem, and maintenance of gut health is a high nutrient-consuming task. Croom et al. (1) reported that energy required for gut maintenance accounts for about 25% of the total basal metabolic needs of an animal. The requirements may even be higher during an event of enteric distress or microbial pathogen challenge, which can significantly impact the partitioning of energy and other nutrients away from growth, thus reducing the overall feed conversion efficiency. Gut health can be maintained by a balance between the protective function of intestinal mucosa mucin secretion and the symbiotic community of microorganisms that competitively exclude pathogenic microbes (2).
Dietary composition plays an important role in maintaining the healthy gut ecosystem. Non-starch polysaccharides (NSPs), such as arabinoxylan and β-mannan, are one of the dietary components that influence the gut ecosystem. Their complex water-soluble structure increases digesta viscosity (3), which may entrap macronutrients, such as fat, protein, and starch, and reduces digestive enzyme–substrate interactions (4). Consequently, digestion and nutrient absorption in the foregut is impeded, which adversely effects nutrient supply for growth and reduces feed conversion efficiency. Furthermore, viscous β-mannan and arabinoxlyan may carry undigested nutrients from foregut into the hindgut where they become substrate for the fermentation of competitive microflora that alters the enteric ecosystem stability (5).
Excessive NSPs in the diet, such as arabinoxylan and β-mannan, may lead to the proliferation of undesirable pathogenic intestinal microflora, such as E. coli and Clostridium spp. (5–7). These enteric pathogens initiate a mucosal inflammatory response, leading to enteric distress, and suppressed gut morphological development. Consequently, this could have a biological effect on intestinal health by changing the mucosal morphological architecture and diverting more nutrients away from productive purposes toward intestinal maintenance. Enteric inflammation, due to enteric pathogen challenge, is associated with the activation of the innate immune system, which is positively associated with the stimulation of intestinal mucous secretion (8). Mucin, which is secreted by the goblet cells, is the protein-rich component of mucous. Although, little information is available on the influence of dietary arabinoxylan and β-mannan on enteric mucosa morphological development and health, atrophic shortening and thickening of jejunum villi, along with increased number of goblet cells per villus, has been observed in broilers fed a diet containing β-glucans which is a similar NSPs (9).
To our knowledge, there are limited published data available on the influence of enzymes on intestinal health, particularly as it affects gut morphology and intestinal adherent mucin secretion in turkey poults. This paper is a report of results from two experiments that evaluated the influence of dietary enzyme supplementation of turkeys fed high and low-energy diets, modified by dietary fat supplementation, on gut health as assessed by changes in mucosa morphometric characteristics and adherent ileal mucosa mucin layer thickness.
Materials and Methods
Experiment 1
Experimental diet
The experiment was designed as a 2 × 2 factorial arrangement of two dietary inclusion levels of β-mannanase (0 and 0.05% CTCzyme®, CTCBio, Inc., Korea), supplying 0 and ~500 U endo-beta-d-mannanase/kg of diet, respectively, and two dietary levels of metabolizable energy (high and low) that differed by 150 kcal ME/kg by the addition of supplemental beef tallow fat. All experimental diets were corn-SBM based formulations. The high-energy basal diet was made and divided into two lots, and each was mixed with either 0.05% of vermiculite, or 0.05% of β-mannanase. A second low-energy basal diet (150 kcal/kg less than the high-energy basal diet) was also made and divided into two parts, each was also mixed with either 0.05% of vermiculite or 0.05% of β-mannanase. Vermiculite was added as a non-nutritional filler in place of the enzyme in the experimental control diets. All experimental diets were formulated to meet or exceed NRC (10) requirements for turkeys (Table 1). All diets were supplemented with 2.0% Celite® (Celite Corp., Lompar, CA, USA) which served as an acid insoluble ash indigestible reference marker for the determination of digestibility coefficients. The calculated and analyzed composition of the experimental diets, including the supplemental enzymes, is reported in Table 1. All diets were produced at the North Carolina State University Feed Mill Educational Unit (Raleigh, NC, USA).
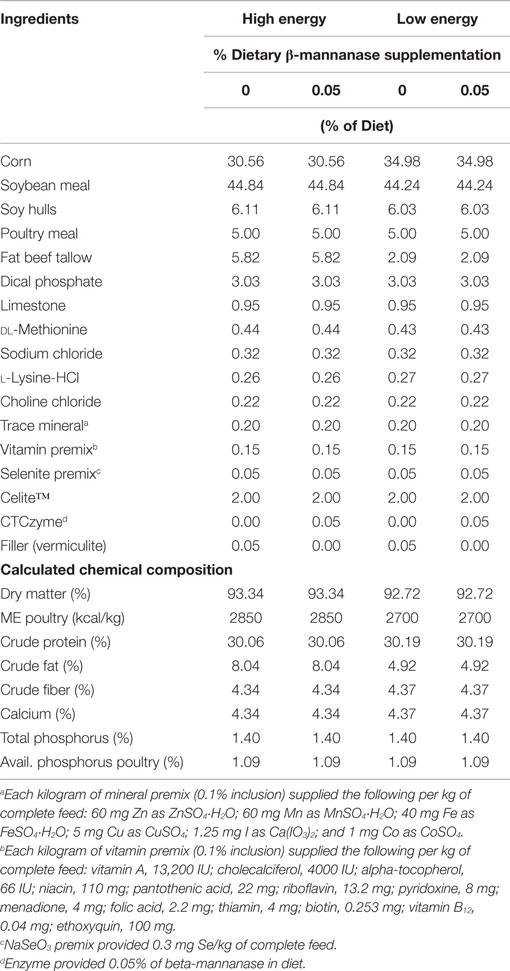
Table 1. Dietary ingredient composition and nutrient composition of starter diets fed to turkey hens from 1 to 28 days of age.
Bird Husbandry and Tissue Sampling
Four hundred thirty-two Nicholas hen poults were obtained from a commercial hatchery (Prestage Farms Hatchery, Clinton, NC, USA) and randomly assigned to one of 48 wire-floored cages with nine poults per cage (Alternative Design cages, Alternative Design Manufacturing and Supply, Inc., Siloam Springs, AR, USA). Each bird was identified with a numbered neck-tag in sequence for each replicate cage group. Four experimental treatment groups were randomly assigned among 48 cages. Feed and water was available ad libitum. Birds were reared until 28 days of age. From 1 to 7 days, the birds were provided 23 h of light to 1 h darkness, and 14 L:10 D after 7 days. On 7, 14, and 21 days, a 3-cm segment of the jejunum, from the end of the duodenal loop toward the Meckel’s diverticulum, was sampled from each of four poults per cage, gently flushed with saline, and fixed in 10% formalin solution for subsequent histological analysis. Another gut segment, representing 1 cm into the ileal section from the Meckel’s diverticulum, was collected from each of the same four poults per cage for mucin histochemical analysis.
Experiment 2
Experimental Diets
The objective of the second experiment was to determine the effect of the dietary supplementation of an experimental blend of xylanase, amylase, and protease (XAP) and a direct-fed microbial (DFM) product (DuPont, St. Louis, MO, USA) on gut morphological development and ileal adherent mucin layer thickness in turkeys. The experiment was designed as a 2 × 4 factorial, with two inclusion levels of DDGS and four levels of different type of feed supplements [negative control (NC), 2% supplemental fat, XAP, and XAP + DFM]. Two experimental corn-soybean meal basal diets, containing 6 or 18% DDGS, were pelleted and crumbled and subsequently divided into four lots. The first lot was retained as the NC, the second lot was supplemented with 2% supplemental fat, the third lot was supplemented with the enzyme blend XAP, and the fourth lot was supplemented with the combination of XAP + DFM. The enzyme activities per gram of the XAP blend was 2000 FTU of xylanase, 200 FTU of amylase, and 5000 FTU of protease, and the XAP + DFM also contained 75,000 cfu of Bacillus subtilis per gram of diet. This same experimental diet preparation procedure was used for all feed phases. All experimental diets were formulated to meet or exceed the NRC (10) nutritional recommendations for turkeys (Table 2). All diets were manufactured at the North Carolina State University Feed Mill Educational Unit (Raleigh, NC, USA).
Bird Husbandry and Tissue Sampling
Eight hundred sixty-four 1-day old female poults (Hybrid Converter Hens, Cold Springs Farm, Thamesford, Ontario, Canada) were randomly assigned to 48 litter floor pens containing 18 poults per pen. Six replicate pens were randomly assigned per dietary treatment. The poults had access to ad libitum feed and water and raised according to standard commercial practices. At 42 days of age, six birds per treatments were euthanized by cervical dislocation to collect tissue for histological assessment. A 6-cm section from the mid-portion of the jejunum was taken from each bird, gently flushed with saline, and fixed in 10% formalin solution for subsequent morphometric analysis. Ileal sections were also taken each of the euthanized birds for mucosal adherent mucin secretion as previously described.
Histological and Histochemical Analysis
Histological Analyses
The tissue samples were immediately rinsed with saline, and fixed in 10% neutral-buffered formalin solution for at least 72 h before processing. A total of four sections of about 2–3 mm in length were taken from a 3-cm fixed jejunum section collected from each sampled bird. These smaller sections were placed in tissue cassettes and submerged in 10% buffered formalin solution until processed at the Histopathology Laboratory (NC State University, College of Veterinary Medicine, Raleigh, NC, USA). The fixed jejunum sections were embedded in paraffin wax, and 5 μm thick transverse sections were cut with a microtome. The 5 μm-cut sections were placed on slides and were stained with Lilee Meyer hematoxylin and counter-stained with eosin yellow. A light-microscope (LEICA-DMR light-microscope, Leica Camera AG, Solms, Germany) was used to visualize the transverse sections placed on slides. The images were captured using a Spot-LTCR digital camera (Diagnostic Instruments, Inc., Sterling Heights, MI, USA) and analyzed using Image Tool software (UTHSCSA Image Tool Software, Version 3.0, the University of Texas, San Antonio, TX, USA). Villus height, villus apical width at the tip of the villus, villus basal width at the crypt-villus junction, crypt depth, and muscularis depth were measured on 10 villi per sampled poult. The villi height:crypt depth ratio for each poult was calculated by dividing the average of the 10 villi heights measured per poult by the average of the 10 crypt depths measured on the same poult. The following mathematical formula was used to determine apparent villus surface area {[(villus tip + villus base)/2] × villus height}, according to Iji et al. (11).
Histochemical Analyses – Measurement of Adherent Ileal Mucin Layer Thickness
The epithelial-adherent mucin layer thickness was assessed histochemically with Alcian blue stain, based on the affinity of the stain for mucin (12). The thickness of the ileal mucus adherent layer was estimated based on the modification of Parman’s method (13). A 1-cm section of ileal tissue from each sampled bird was removed and placed in 10 g/L Alcian blue dye solution in buffer containing 160 mmol/L sucrose and 50 mmol/L sodium acetate, pH 5.8. After 6 h of incubation, excess dye was extracted with 250 mmol/L sucrose. The absorbed dye was extracted from the tissue by incubation in 10 g/L docusate sodium salt solution overnight at room temperature. Samples were centrifuged at 700 g, plated on 96-well plate, and the optical densities were measured at 620 nm using Alcian blue solution as a standard. The amount of absorbed dye was reported as micrograms of Alcian blue per gram of ileal tissue.
Statistical Analysis
The experiments were analyzed as completely randomized designs. Pen or cage means were respectively used as the experimental unit for the statistical analysis of adherent ileal mucin secretion. For histological analysis, 10 villi measurements were averaged per tissue sample collected, and this average number served as the experimental unit for the statistical analysis. Data were analyzed using JMP software (Version 10, SAS Institute, Cary, NC, USA). ANOVA was used to examine the main effect of dietary treatment factors, and their interaction on parameters evaluated. Means were separated using the LS Means at P < 0.05.
Animal Ethics
Care of the birds used in all experiments conformed to the Guide for Care and Use of Agricultural Animals in Research and Teaching (14). Moreover, all animal husbandry practices and euthanasia performed during the conduct of these experiments were conducted according to protocol # 12-014-A approved by Institutional Animal Care and Use Committee at North Carolina State University.
Results
Experiment 1
Table 3 summarizes the effect of dietary energy level and β-mannanase supplementation on villi morphological characteristics and ileal adherent mucin thickness layer of turkey hens at 21 days of age.
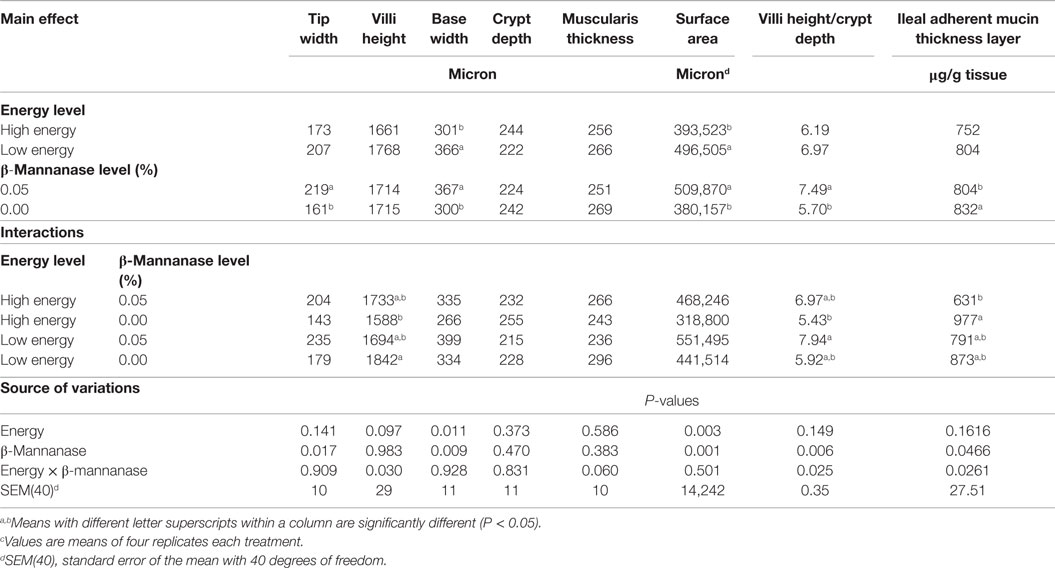
Table 3. Effect of dietary energy level and β-mannanase supplementation on jejunum villi morphological characteristics and ileal adherent mucin thickness layer of turkey hens at 28 days of age.c
Gut Morphological Development
There were no significant treatment effects observed on jejunum mucosa morphology at 7 and 14 days; however, there was a significant treatment effect observed at 28 days. Increasing dietary energy level was associated with an 18% decrease in the villus base width and a 21% decrease in villus surface area (P < 0.05). In contrast, dietary supplementation of β-mannanase increased the villus tip width by 36% and villus height/crypt depth by 32% (P < 0.05), while the villus base width and surface area was increased by about 22.5 and 34%, respectively (P < 0.05).
Ileal Adherent Mucin Layer Thickness
There was no significant main effect of dietary energy; however, poults fed the β-mannanase supplemented diet had a 4% reduction in ileal adherent mucin layer thickness as compared to the unsupplemented control diet. Furthermore, the supplementation of β-mannanase to the high-energy diet reduced the ileal adherent mucin layer thickness by about 36% as compared to the high-energy diet without supplementation (P < 0.05), but neither of these diets were significantly different from the results observed among birds fed the low-energy diets with or without the enzyme supplementation (P > 0.05).
Experiment 2
Table 4 summarizes the influence of dietary DDGS level, and XAP and DFM supplementation on the morphological measurements of jejunum villi and mucosa of turkey hens at 42 days.
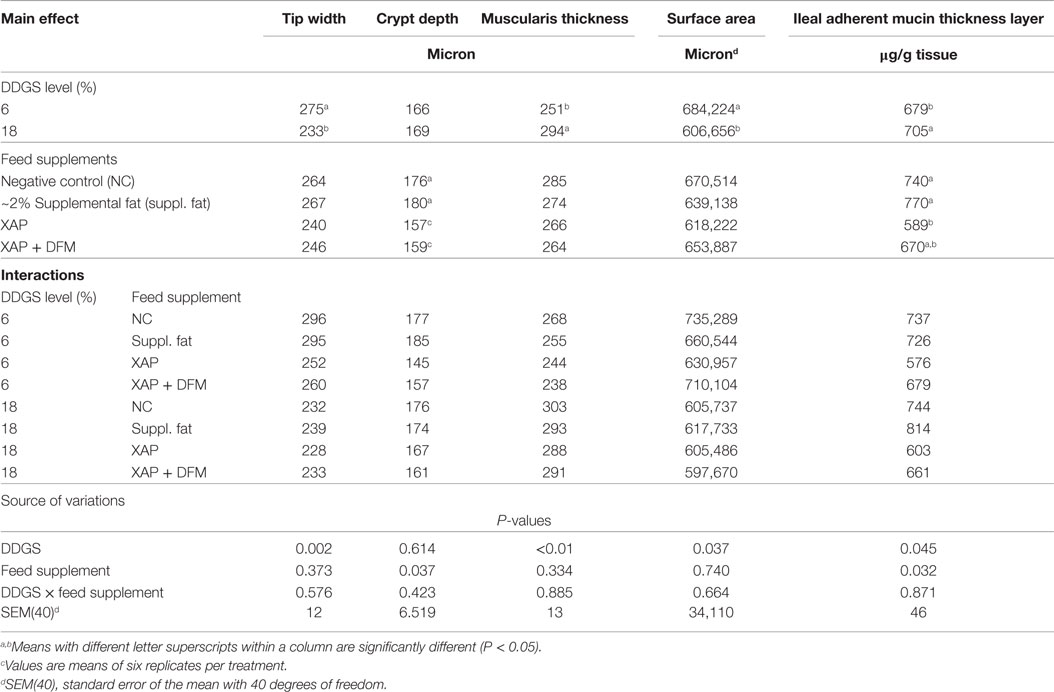
Table 4. Influence of dietary DDGS level, and XAP and DFM supplementation on the morphological measurements of jejunum villi and mucosa of turkey hens at 42 days.c
Gut Morphological Development
Some effects were observed on mucosa morphology development at 42 days of age. Dietary inclusion of 18% DDGS was associated with a 15% decrease in villus tip width (P < 0.05) and 11% decrease in villi surface area (P < 0.05) relative to the 6% DDGS treatment. However, the 18% dietary inclusion of DDGS treatment had a 13% increase in muscularis thickness (P < 0.05) relative to the 6% DDGS inclusion. DDGS inclusion did not have significant effect on the villi height, crypt depth, villi height/crypt depth, and villi base width. The dietary supplements did not have any effect on villi tip width, height, villi height/crypt depth, villi base width, muscularis thickness, and villi surface area, although they did affect the crypt depth. Poults-fed diets supplemented with XAP alone or in combination with DFM had reduced crypt depth by about 12 and 11%, respectively, when compared with the supplemental fat and NC (P < 0.05).
Ileal Adherent Mucin Layer Thickness
The results of the ileal adherent mucin layer thickness are also presented in Table 4. Increasing the dietary level of DDGS increased the ileal adherent mucin layer thickness observed in poults at 42 days of age (P < 0.05). However, in comparison to the supplemental fat and NC treatments, dietary supplementation of XAP reduced the ileal adherent mucin layer thickness by 24 and 20%, respectively (P < 0.05); but neither response was different from XAP + DFM.
Discussion
Gut Morphological Development
Dietary soluble and insoluble β-mannans and arabinoxylans have been reported to exhibit some anti-nutritional properties and adverse effects on growth performance of poultry. The high arabinoxylan content in DDGS along with its inferior amino acid digestibility and variability of other nutrients limits the dietary inclusion of DDGS in poultry feed to <6%. Likewise, the viscous nature of β-mannan in diets, that contain a lot of soybean meal, has been observed to cause physiological and morphological changes to the gastrointestinal tract in poultry, which can impede efficient nutrients utilization (15, 16).
The general hypothesis tested in the two studies reported herein was that the supplementation with β-mannanase, XAP, or a combination of XAP + DFM will reduce the anti-nutritional effects and improve apparent nutrient utilization by reducing endogenous nutrient losses associated with enteric mucin secretion, enhancing gut morphological development, and improving gut health. Because dietary inclusion of the enzymes may help degrade the NSPs, their anti-nutritional effects may, thereby, be diminished and thus improve nutrient utilization, as residual enteric substrates are altered to positively favor symbiotic microflora and microbial diversity over the pro-inflammatory pathogenic ones in the hindgut ecosystem, consequently improving the gut health.
Measured changes in intestinal morphology, such as shorter villi and deeper villi crypts, have been used as indicators of gut health and enteric distress (17). Tall mucosal villi increase the surface area available for nutrients absorption (18, 19). There is correlation between the crypt depth and the rate of proliferation of the epithelial cells (20, 21). Epithelial regeneration starts from the villi crypt, so a deep crypt is an indication of rapid enterocyte turnover and increased mucosal tissue maintenance requirements (17, 22). The rapid enterocyte proliferation and the epithelial cell turnover rate greatly impacts protein and energy requirements of the small intestine mucosa (23). Diet composition may produce microscopic alterations in the intestinal mucosa, and it is possible that the change in morphology of the gastrointestinal tract may be associated with dietary NSP levels (24).
Some studies have demonstrated that enzyme treatment can influence the morphological development of intestinal villi (19, 25, 26). In the first trial, we observed that dietary inclusion of β-mannanase had a positive effect on jejunum mucosal morphology among poults sampled at 28 days: villus surface area and tip and base widths were all increased. Although there are few publications demonstrating the effect of β-mannanase on gut morphology, the work of Mehri et al. (27) corroborated our findings. There was an increase in the villi surface area and jejunum villi height/crypt depth ratio by dietary β-mannanase supplementation. Mehri et al. (27) observed an increase in villus height and crypt depth in the duodenum when β-mannanase was supplemented to corn–soy diets at 700 and 900 g/ton.
In the second experiment, the level of arabinoxylan, a NSP anti-nutritional factor (ANF), was calculated to increase by about 50% as the dietary inclusion level DDGS increased from 6 to 18% (Table 2). Choct and Annison (28) have shown anti-nutritional effects of depressed apparent metabolizable energy when 3% pentosans, primarily comprised of arabinoxylans, was added to broiler diets. Relative to the 6% DDGS treatment, dietary inclusion of 18% DDGS adversely affected jejunum mucosal morphology as indicated by reduced villi surface area, tip width and increased thickness of the villi muscularis. The poor jejunum morphological development may be associated with relatively higher NSP content in the 18% DDGS diet. Iji (29) reported that NSP can negatively impact gut morphology by increasing crypt depth of both the jejunum and ileum, thus accelerating enterocyte turnover. Although we evaluated the effects of a blend of supplemental enzymes containing xylanase in the second experiment, xylanase supplementation alone has been reported to increase villus height of the duodenum, jejunum, and ileum, and the villus height/crypt depth of these three segments (19). Furthermore, the dietary inclusion of DFM may also impact the gut morphology by increasing the jejunum and ileal villi heights (30, 31). Dietary supplementation of the enzyme blend of XAP and XAP + DFM combination had a beneficial effect on gut morphology development. The addition of the enzyme blend with or without the DFM reduced the crypt depth, indicating reduced mucosal distress, in comparison to the NC or fat-supplemented diet.
Apparently, the effects observed on enteric mucosa morphology in both trials may have been associated with the changes in the substrate characteristics within the enteric ecosystem by the dietary supplementations (β-mannanase, XAP, and XAP + DFM), which in turn altered the fermentation of resident enteric microflora (32).
Adherent Ileal Mucin Secretion
Establishment of a symbiotic enteric ecosystem minimizes the inflammatory symptoms of enteric distress caused by the proliferation of enteric pathogens, which is associated with increased intestinal mucin secretion (33, 34). Parsaie et al. (35) reported that distressed intestinal morphology, as indicated by the shortened villi and deepened crypts, may cause increased mucosal secretions, primarily as mucin. Indeed, some research reports have shown that the dietary inclusion of enzymes reduce the mucosal blanket secretions in monogastric animals (36, 37).
In addition to the improved gut morphological development observed in this study, a reduction in the ileal adherent mucin layer thickness was observed with dietary β-mannanase supplementation, especially in the high-energy (fat) diets. Few studies have been reported on the influences of β-mannanase on mucin secretion. However, research results reported trial by Mehri et al. (27) indirectly validated our observation. They reported that dietary β-mannanase supplementation reduced the number of mucosal goblet cells per unit of epithelial surface area in broilers. Since mucin is secreted by the epithelial goblet cells, this observation agrees with our observations that dietary β-mannanase supplementation significantly reduces intestinal adherent mucin layer thickness.
We also observed that the addition of XAP reduced ileal mucin thickness layer. Bedford et al. (36) also noted that xylanase supplementation improved the apparent digestibility of threonine, which was associated with a decrease in intestinal mucin secretion. Pirgozliev et al. (37) also observed a decrease in the quantity of threonine excreted by the birds fed with a xylanase-supplemented diet, which he attributed to less secretion of intestinal mucin.
Conclusion
Improved gut morphological development of the mucosa villi in the jejunum and ileum and the reduced adherent ileal mucin thickness layer could likely contribute to the improved apparent nutrient utilization, which reflects in the better growth performance in poultry often observed when their diets are supplemented with exogenous enzymes. Enteric microflora profile has been demonstrated to be influenced by nutrient abundance (32). Digesta viscosity increases as dietary anti-nutritional NSPs increases, which impairs foregut digestion and absorption of fats, starches, and proteins, and causes more of these nutrients to pass to the hindgut where they “feed” competitive pathogenic bacteria. Proliferation of pathogenic bacteria, like C. perfringens, causes enteric tissue inflammation, mucosal leakage, and increased mucin secretion as a protective innate immune response. With protein being a major component of mucin, this increase in mucin secretion can further exacerbate the proliferation of putrefying bacterial pathogens and also contribute to the significantly reduced apparent nitrogen retention. Dietary supplementation of NSP enzymes can be an effective means to counter the adverse effects of high digesta viscosity and cause the hind gut microflora to shift toward a more symbiotic ecosystem.
The research results reported herein demonstrate that dietary supplementation of either β-mannanase, XAP, or XAP + DFM can improve gut health in turkey poults as indicated by improved morphological development of the enteric mucosa and reduction in adherent ileal mucin secretion, thereby increasing productive use of nutrients toward better growth performance. Further studies are still needed to evaluate the effect of other supplemental enzymes on gut health, especially as the trend of limiting the use of antibiotic growth promoters in animal feed continues.
Conflict of Interest Statement
The authors declare that the research was conducted in the absence of any commercial or financial relationships that could be construed as a potential conflict of interest.
Funding
Funding was provided by the NC Agricultural Foundation, Inc., Raleigh, NC, USA, and National Institute of Food and Agriculture Project Number NC06343, United State Department of Agriculture.
References
1. Croom J, Edens FW, Ferket PR. The impact of nutrient digestion and absorption on poultry performance and health. In: Ferket PR, editor. Proceedings of the 27th Annual Carolina Poultry Nutrition Conference. Raleigh, NC: Carolina Feed Industry Association, Research Triangle Park (2000). p. 65–73.
2. Ferket PR. Alternatives to antibiotics in poultry production: responses, practical experience and recommendations. In: Lyons TP, Jacques KA, editors. Nutritional Biotechnology in the Feed and Food Industries: Proceedings of Alltech’s 20th Annual Symposium. Lexington, KY: Nottingham University Press (2004). p. 56–67.
3. Lee JT, Bailey CA, Cartwright AL. β-Mannanase ameliorates viscosity-associated depression of growth in broiler chickens fed guar germ and hull fractions. Poult. Sci (2003) 82:1925–31.
4. Smits CH, Veldman NA, Verstegen MWA, Beynen AC. Dietary carboxymethylcellulose with high instead of low viscosity reduces macronutrient digestion in broiler chickens. J Nutr (1997) 127:483–7.
5. Choct M, Hughes RJ, Wang J, Bedford MR, Morgan AJ, Annison G. Increased small intestinal fermentation is partly responsible for the anti-nutritive activity of non-starch polysaccharides in chickens. Br Poult Sci (1996) 37:609–21. doi: 10.1080/00071669608417891
6. Apajalahti J, Bedford MR. Improve bird performance by feeding its microflora. World Poult (1999) 15:20–3.
7. Choct M, Dersjant-Li Y, McLeish J, Peisker M. Soy oligosaccharides and soluble non-starch polysaccharides: a review of digestion, nutritive and anti-nutritive effects in pigs and poultry. Asian-Aust J Anim Sci (2010) 23(10):1386–98. doi:10.5713/ajas.2010.90222
8. Deplancke B, Gaskins HR. Microbial modulation of innate defense: goblet cells and the intestinal mucus layer. Am J Clin Nutr (2001) 73(l):1131S–41S.
9. Viveros A, Brenes A, Pizarro M, Castario M. Effect of enzyme supplementation of a diet based on barley, and autoclave treatment, on apparent digestibility, growth performance and gut morphology of broilers. Ani Feed Sci Tech (1994) 48:237–51. doi:10.1016/0377-8401(94)90175-9
10. NRC. Nutrient Requirements of Poultry. 9th rev. ed. Washington, DC: National Academy Press (1994).
11. Iji PA, Saki A, Tivey DR. Body and intestinal growth of broiler chicks on a commercial starter diet. 1. Intestinal weight and mucosal development. Br Poult Sci (2001) 42:505–13. doi:10.1080/00071660120073151
12. McManusi FA, Mowry RW. Staining Methods Histology and Histochemical. New York: Paul B. Hoeber, Inc. (1960). 423 p.
13. Parman NS, Desai JK. A review of the current methodology for the evaluation of gastric and duodenal anti-ulcer agents. Indian J Pharmacol (1993) 25:120–35.
14. FASS. Guide for the Care and Use of Agricultural Animals in Research and Teaching. 3rd ed. Champaign, IL: Federation of Animal Science Society (2010).
15. Cassidy MM, Lightfoot FG, Grau LE, Story JA, Kritchevsky D, Vahouny GH. Effect of chronic intake of dietary fibers on the ultra-structural topography of rat jejunum and colon: a scanning electron microscopy study. Am J Clin Nutr (1981) 34:218–28.
16. Jacobs LR. Effects of dietary fiber on mucosal growth and cell proliferation in the small intestine of the rat: a comparison of oat bran, pectin, and guar with total fiber deprivation. Am J Clin Nutr (1983) 37:954–60.
17. Yason CV, Summers BA, Schat KA. Pathogenesis of rotavirus infection in various age groups of chickens and turkeys: pathology. Am J Vet Res (1987) 6:927–38.
18. Awad W, Ghareeb K, Böhm J. Intestinal structure and function of broiler chickens on diets supplemented with a symbiotic containing Enterococcus faecium and oligosaccharides. Int J Mol Sci (2008) 9:2205–16. doi:10.3390/ijms9112205
19. Luo D, Yanga F, Yang X, Yao J, Shi B, Zhou Z. Effects of xylanase on performance, blood parameters, intestinal morphology, microflora and digestive enzyme activities of broilers fed wheat-based diets. Asian-Aust J Anim Sci (2009) 22(9):1288–95. doi:10.5713/ajas.2009.90052
20. Brunsgaard G. Weaning and the weaning diet influence the villous height and crypt depth in the small intestine of pigs and alter the concentrations of short chain fatty acids in the large intestine and blood. J Nutr (1998) 128:947–53.
21. Yasar S, Forbes JM. Performance and gastro-intestinal response of broiler chickens fed on cereal grain-based foods soaked in water. Br Poult Sci (1999) 40:65–76. doi:10.1080/00071669987854
22. Paulus U, Potten CS, Loeffler M. A model of the control of cellular regeneration in the intestinal crypt after perturbation based solely on local stem cell regulation. Cell Prolif (1992) 25:559–78. doi:10.1111/j.1365-2184.1992.tb01460.x
23. Simon O. Metabolism of proteins and amino acids. In: Bock HD, Eggum BO, Low AG, Simon O, Zebrowska T, editors. Protein Metabolism and Farm Animals. Evaluation, Digestion, Absorption and Metabolism. Berlin, Germany: Oxford University Press and VEB Dt Landwirtschaftsverlag (1989). p. 271–336.
24. Yamauchi K. Review of chicken intestinal villus histological alterations related with intestinal function. J Poult Sci (2002) 39:229–42. doi:10.2141/jpsa.39.229
25. Brenes A, Smith M, Guenter W, Marquardt RR. Effect of enzyme supplementation on the performance and digestive tract size of broiler chickens fed wheat and barley based diets. Poult Sci (1993) 72:1731–9. doi:10.3382/ps.0721731
26. Amat C, Planas JM, Moretó M. Kinetics of hexose uptake by the small and large intestine of the chicken. Am J Physiol (1996) 271(4 Pt 2):R1085–9.
27. Mehri M, Adibmoradi M, Samie A, Shivazad M. Effects of β-Mannanase on broiler performance, gut morphology and immune system. African J Biotech (2010) 9(37):6221–8. doi:10.5897/AJB09.1371
28. Choct M, Annison G. Anti-nutritive activity of wheat pentosans in broiler diets. Br Poult Sci (1990) 31:811–21. doi:10.1080/00071669008417312
29. Iji PA. The impact of cereal non-starch polysaccharides on intestinal development and function in broiler chickens. World’s Poult. Sci J (1999) 55:375–87.
30. Chichowski M, Croom WJ, Edens FW, MacBride BW, Qiu R, Chiang CC, et al. Microarchitecture and spatial relationship between bacteria and ileal, cecal and colonic epithelium in chicks fed a direct-fed microbial, PrimaLac, and Salinomycin. Poult Sci (2007) 86:1121–32. doi:10.1093/ps/86.6.1121
31. Samli HE, Senkoylu N, Koc F, Kante M, Agma A. Effects of Enterococcus faecium and dried whey on broiler performance, gut histomprphology and microbiota. Arch Anim Nutr (2007) 61:42–9. doi:10.1080/17450390601106655
32. Knarreborg A, Simon R, Engberg M, Jensen BB, Tannock GW. Effects of dietary fat source and subtherapeutic levels of antibiotic on the bacterial community in the ileum of broiler chickens at various ages. Appl Environ Microb (2002) 68:5918–24. doi:10.1128/AEM.68.12.5918-5924.2002
33. Fukata T, Sasai K, Miyamoto T, Baba E. Inhibitory effects of competitive exclusion and fructooligosaccharide, singly and in combination, on Salmonella colonization of chicks. J Food Protect (1999) 62:229–33.
34. Grizard D, Barthomeuf C. Non-digestible oligosaccharides used as prebiotic agents: mode of production and beneficial effects on animal and human health. Reprod Nutr Dev (1999) 39:563–88. doi:10.1051/rnd:19990505
35. Parsaie S, Shariatmadari F, Zamiri MJ, Khajeh K. Influence of wheat-based diets supplemented with xylanas, bile acid and antibiotics on performance, digestive tract measurements and gut morphology of broilers compared with a maize-based diet. Br Poult Sci (2007) 48:594–600. doi:10.1080/00071660701615788
36. Bedford MR, Scott TA, Silversides FG, Classen HL, Swift ML, Pack M. The effect of wheat cultivar, growing environment, and enzyme supplementation on digestibility of amino acids by broilers. Can J Anim Sci (1998) 78:335–42. doi:10.4141/A98-012
Keywords: supplemental enzymes, gut health, enteric mucosal morphology, mucin, turkeys
Citation: Ayoola AA, Malheiros RD, Grimes JL and Ferket PR (2015) Effect of dietary exogenous enzyme supplementation on enteric mucosal morphological development and adherent mucin thickness in Turkeys. Front. Vet. Sci. 2:45. doi: 10.3389/fvets.2015.00045
Received: 30 August 2015; Accepted: 28 September 2015;
Published: 13 October 2015
Edited by:
Michael Kogut, USDA-ARS, USACopyright: © 2015 Ayoola, Malheiros, Grimes and Ferket. This is an open-access article distributed under the terms of the Creative Commons Attribution License (CC BY). The use, distribution or reproduction in other forums is permitted, provided the original author(s) or licensor are credited and that the original publication in this journal is cited, in accordance with accepted academic practice. No use, distribution or reproduction is permitted which does not comply with these terms.
*Correspondence: Peter R. Ferket, peter_ferket@ncsu.edu