- 1Wake Forest Institute for Regenerative Medicine, Winston Salem, Winston Salem, NC, United States
- 2Department of Laboratory Medicine, Lund University, Lund, Sweden
- 3Department of Urology, Skåne University Hospital, Lund, Sweden
- 4Department of Clinical Sciences, Lund University, Lund, Sweden
Studies on patients with bladder outflow obstruction who have undergone surgery for benign prostatic hyperplasia, successfully relieving the obstruction, have revealed a persistence of storage symptoms associated with detrusor overactivity (DO) in 20% to 40% of patients. To study the underlying mechanisms, we have used a common rat model of obstruction/de-obstruction, assuming that non-voiding contractions can be used as a surrogate parameter for DO in humans. Using microarray analysis and electron microscopic images from obstructed and de-obstructed bladder tissue we have tried to identify changes that could serve as a basis for the search of new targets for drugs. Even if voiding function is rapidly normalized after release of outflow obstruction and many of the morphological changes are reversed, the microarray analysis revealed that the de-obstructed rat bladder has gene expressions, structural, and functional properties that make it distinctly different from both control and obstructed bladders. We suggest that whole bladder arrays can be used for identifying cellular mechanisms that could be targets for drugs meant for treatment of persistent DO and LUTS after de-obstruction. Based on available array information for some membrane receptors and morphologic structures with corresponding changes in bladder function, it seems worthwhile to re-assess the development potential for e.g., endothelin receptor antagonists, purinergic receptor antagonists and Rho-kinase inhibitors.
Introduction
In patients with a clinical diagnosis of benign prostatic hyperplasia (BPH) and bladder outlet obstruction (BOO), lower urinary tract symptoms (LUTS) can be categorized as storage, voiding, and postmicturition symptoms (1). The storage (irritative) symptoms (overactive bladder syndrome: OAB; detrusor overactivity, DO: cystometric diagnosis) have been associated with the changes in the bladder caused by the obstruction, whereas the voiding (obstructive) symptoms are linked to the enlarged prostate (the static component), and the tone of its smooth muscle (the dynamic component). In many studies on BOO patients who have undergone surgery for BPH successfully relieving the obstruction, persistence of storage symptoms associated with DO has been reported. In fact, DO has been shown to persist after prostatectomy in 20% to 40% of patients treated for the disease (2–6).
The links between DO/LUTS and the changes in the bladder occurring after relief of BOO have not been clarified. The persistent symptoms have been attributed to changes within the central nervous system or to changes within the bladder (7). Interestingly, Seaman et al. (8) found that residual or recurrent obstruction was a contributing factor in less than half of all patients who had persistent symptoms. Cumming and Chisholm (9) suggested that persistence of symptoms after prostatectomy may be explained by failure of postoperative detrusor reinnervation. However, studies in pigs with experimental outflow obstruction/de-obstruction showed that the relationship between the degree of reinnervation and the persistence of DO is unclear and that there is a complex relationship between outflow obstruction and detrusor damage (10).
The relations between symptoms, BOO, bladder function and changes in the bladder before and after de-obstruction can, for obvious reasons, not be studied in humans. Rats subjected to BOO will develop hyperactive voiding, characterized by increased frequency and non-voiding contractions (NVCs), which persist after de-obstruction in a significant proportion of the animals (7, 11). Assuming that NVCs can be used as a surrogate parameter for DO in humans, the rat model of obstruction/de-obstruction may offer an opportunity to study what occurs in the bladder after de-obstruction. Studies have been performed on the functional and structural bladder changes that occur after de-obstruction using different approaches (12–17). In this study we have focused on the molecular changes in the rat detrusor muscle observed after obstruction/de-obstruction as revealed by mRNA analysis, and on morphological changes studied by electron microscopy. The aim of the study was to use these changes as a basis for the search for new targets for drugs meant for treatment of persistent DO and LUTS after de-obstruction.
Methods of data collection
Some of the microarray data used in this study have been discussed in other publications (see e.g., 18-20) and are publicly available (GEO accession numbers GSE47080, GSE104540). Electron microscopic images of own unpublished, normal, obstructed and de-obstructed bladders were examined. Literature searches on PubMed, Scopus and literature lists of published publications in the field were performed.
Results and discussion
Functional voiding changes in obstruction and de-obstruction
The obstructed bladder has been extensively characterized cystometrically (18–21). Micturition pressure, threshold pressure, bladder capacity, micturition volume, and residual volume increase, and bladder compliance is decreased. Non-voiding bladder activity (NVCs) is consistently observed.
After release of outflow obstruction, voiding function tends to normalize. Cystometrically, there is a decrease in residual volume, bladder capacity, maximal voiding pressure, voiding amplitude, bladder contraction time. Bladder compliance is increased (18, 21–23). Bladder overactivity can be observed two weeks after de-obstruction in up to 46% of the animals (21). Malmgren et al. (22) showed that the in vitro response to carbachol and to electrical stimulation was similar in normal and obstructed bladders. However, after removal of the obstruction a supersensitivity to carbachol as well as to electrical stimulation had developed, suggesting an increased excitability of the detrusor muscle cells (22).
Spontaneous contractile activity and cellular connections
The spontaneous contractile activity that can be observed in isolated bladder tissue and during bladder filling in vivo is one of the factors that may be important for DO and LUTS [e.g., 27-29]. It is obvious that many different factors can contribute or influence this activity (24). including structures in the mucosa (e.g., urothelium, nerves, interstitial cells) and detrusor muscle changes. In this context it would be of particular interest to discuss the role of changes in the detrusor muscle in the generation of DO and LUTS that persist after relief of outflow obstruction.
Non-voiding contractions
Drake et al. (25) suggested that the detrusor is arranged into component modules, each of which is capable of contracting autonomously. During bladder filling such a module, or active autonomous unit, may create a contraction which may originate in an individual myocyte that in turn activates a few nearby cells within the unit (Figure 1). The innervation (afferent and motor) of the cells is intact but there is no activity in the motor nerves. One cell may act as a pacemaker and activate the others. Such a spreading pattern was suggested to be generated solely by mechanical stimulation (transmural pressure) (26). When several active units are contracting simultaneously, this can be reflected by increases in intravesical pressure in isolated perfused bladders or in intact bladders during cystometry (NVCs). The contractions can in vivo also generate afferent activity leading to sensations of urge and urgency.
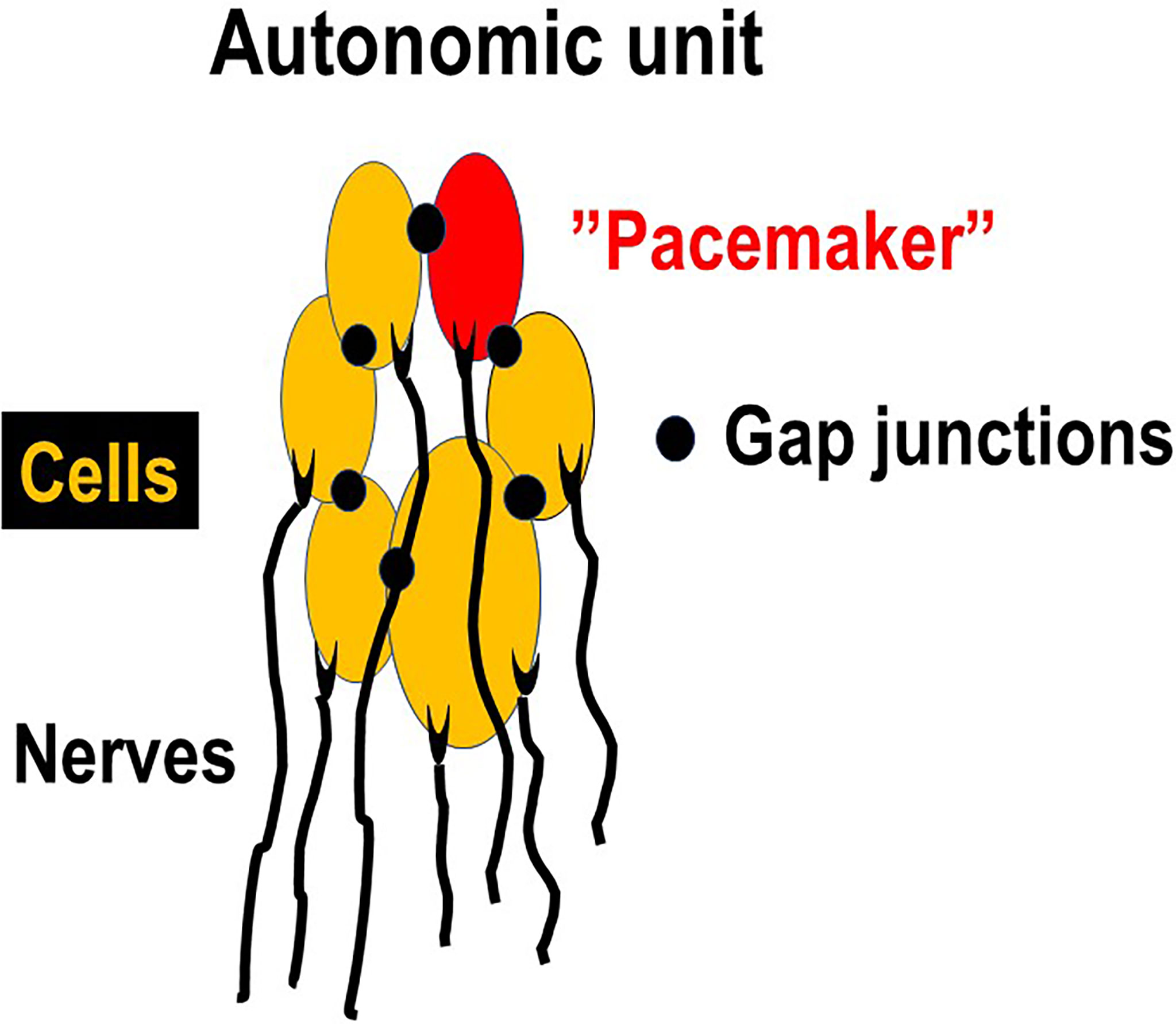
Figure 1 Autonomic unit. Each cell has at least one nerve connection (afferent and/or motor), but the motor nerves are not active during the bladder storage phase. However, one of the cells may act as a “pacemaker”, activating the others and generating contraction of the unit and afferent activity. When several units are contracting simultaneously this can lead to increases in bladder pressure (micromotions) leading to sensation of bladder filling.
The contractile activity of such an autonomous unit requires intercellular communication. Detrusor myocytes are electrically coupled (27) - electrophysiologic data clearly indicate that current injected into one cell flows into neighbouring cells (28, 29). Structural connections between cells can be observed in the de-obstructed bladder (e.g., finger-like processes (30)). However, even by electron microscopy classical gap junctions have been difficult to demonstrate both in the normal and the obstructed and de-obstructed bladders (30). However, the presence of small junctional plaques between myocytes comprising connexins (Cxs) have been demonstrated by different techniques (27). Following 6 weeks of outlet obstruction in a rat model, a dramatic increase of Cx43 mRNA levels associated with bladder hypertrophy and overactivity was demonstrated (31).
The rat detrusor is richly innervated, and each myocyte has at least one neuromuscular junction forming an innervated unit (Figure 2). Afferent fibres in the musculature are present in each muscle bundle and are aligned with the muscle cells (32, 33). The cells are also richly innervated by motor nerves. A detrusor muscle cell can be innervated by more than one motor nerve (34) and belong to more than one motor unit. In the obstructed bladder it has been well demonstrated that some areas of the bladder wall may be denervated – “patchy denervation” (Figure 2) (35, 36). It is reasonable to expect that spontaneous action potentials might appear in detrusor smooth muscle cells in areas of denervation. The action potentials would then spread to both denervated and innervated cells activating the latter (no activity in motor nerves). The bigger the unit, the greater number of cells would contract with consequent greater effect on wall tension and (according to the law of Laplace) on bladder pressure. If the contraction is spread to innervated areas, these could be a source of afferent activity leading to LUTS (27). Afferent activity is a key factor for the generation LUTS and among the approximately 16 000 axons that reach the bladder at least half are sensory (37). Activity in afferent nerves is generated during the filling phase (“afferent noise”) and may mediate sensation of bladder filling and urgency (38).
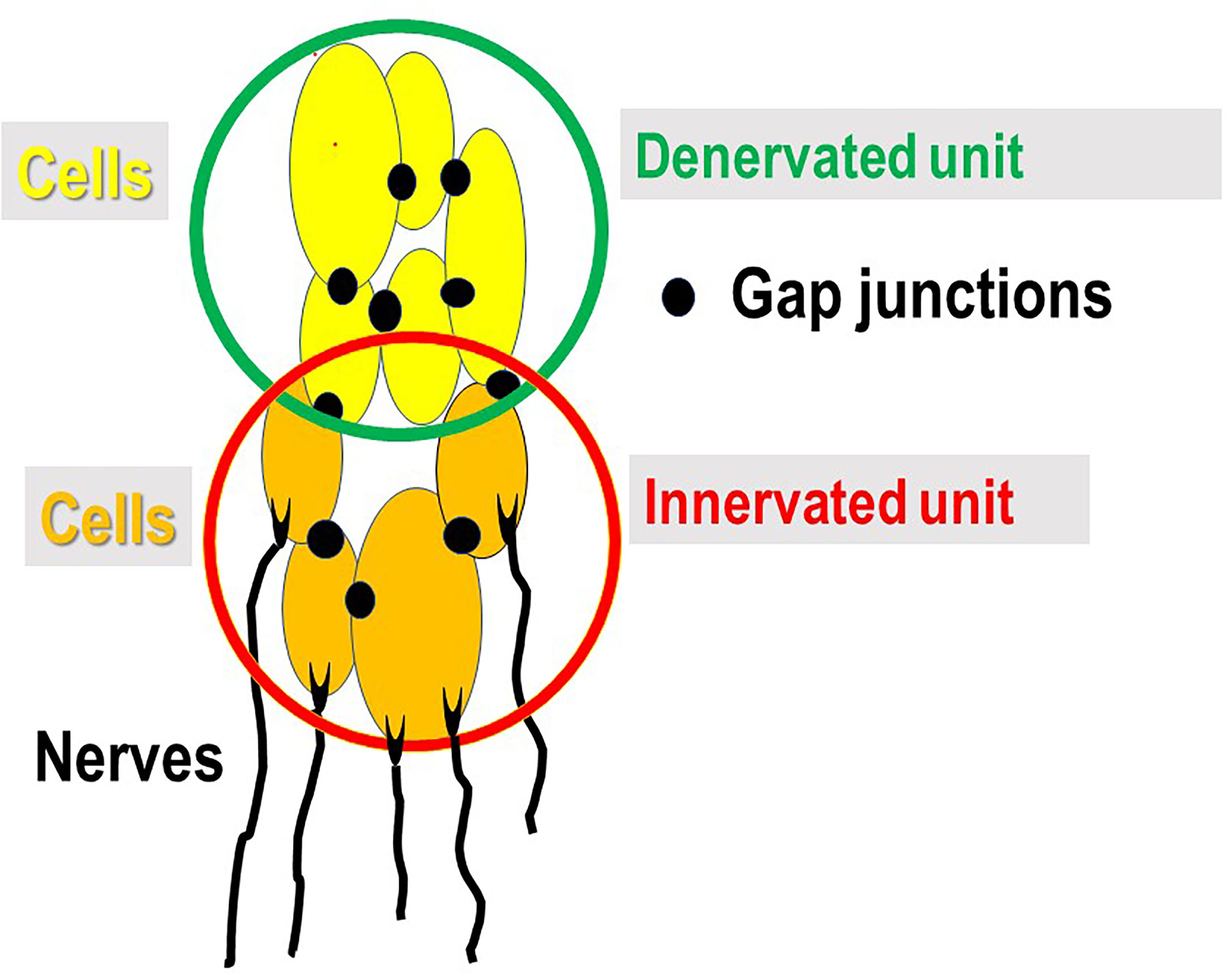
Figure 2 Innervated (motor and sensory) and denervated units. In areas of patchy denervation (denervated unit) of the bladder wall, spontaneous contractile activity of the cells within unit can, via gap junctions, spread to the innervated unit with consequent contraction and activity in afferent nerves (no activity in motor nerves) mediating sensations of bladder filling and urgency.
Structural changes in obstruction and de-obstruction
The changes occurring in the bladder following partial bladder outflow obstruction (BOO) have been well studied. Studies comprise investigations on bladder structure, blood flow, metabolism, receptors and nerves. As studied in a commonly used rat model of BOO (18, 39), the bladder increases in weight and the detrusor muscle responds to the obstruction by hypertrophy-hyperplasia (40). The relative volume of smooth muscle in the bladder wall increases (41) with corresponding increases in contractile and cytoskeletal proteins (42). Total bladder collagen increases, but the increased relative amount of smooth muscle leads to a decrease in collagen concentration (43). Concomitant with the hypertrophy/hyperplasia the pelvic ganglionic neurons innervating the bladder become enlarged (43, 44).
After de-obstruction most of these changes are reversed (12, 13, 22) Bladder weight is almost normalized after 6 weeks, but despite the loss of muscle mass, there seemed to be no degeneration of muscle cells or nerve endings, but the detrusor muscle will contain a large amount of intercellular material, mainly collagen (13). However, structural normalization of the muscle is far from complete (Figure 3). The muscle cells are still larger than in controls and have very irregular shapes with long processes, corrugations, deep laminar indentations and long tubular invaginations. Many small cell contacts are formed (13). In the pelvic ganglionic neurons innervating the bladder the average neuronal size reversed to values very close to controls (45), suggesting that the number of synapses per postganglionic motor axon has normalized in the de-obstructed detrusor.
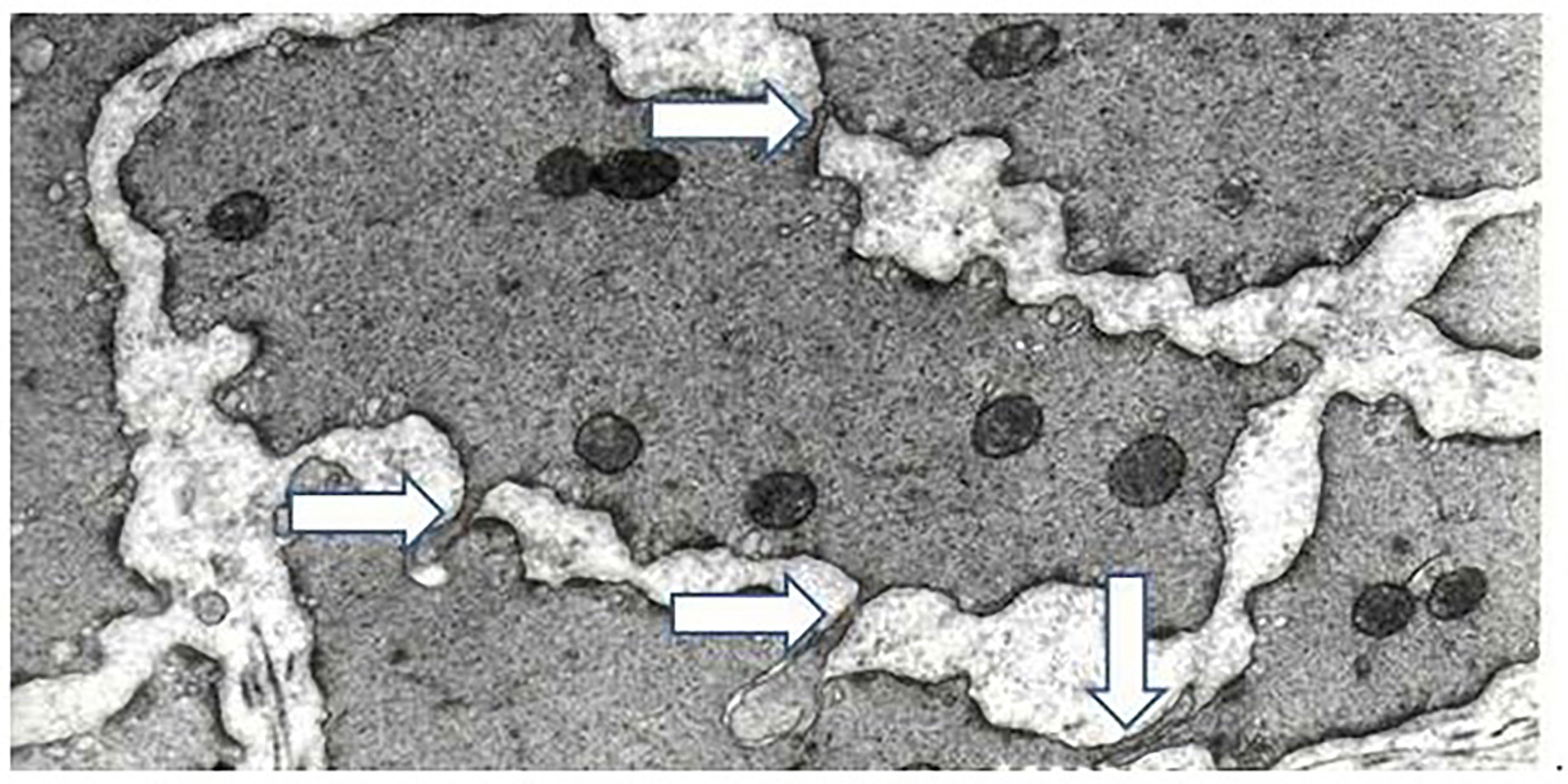
Figure 3 Arrows show finger-like processes from adjacent muscle cells. Detrusor muscle, six weeks of obstruction, followed by six weeks of de-obstruction. Base of micrograph corresponds to 5um. Modified from Uvelius and Andersson, Int J Mol Sci 2022, 23, 11330.
Molecular changes in obstruction and de-obstruction
The microarrays revealed a wide range of changes after de-obstruction. Compared with the obstructed the de-obstructed bladders had a significant difference for 1059 mRNAs (7.3%). For 857 (5.9%) mRNAs the de-obstructed value was significantly higher, and for 202 (1.4%) significantly lower than in the obstructed bladder. Lee et al. (21) analyzed in female rats the patterns of gene expression after bladder outflow obstruction, and de-obstruction. They further analyzed the expression profiles in de-obstructed rats with and without bladder overactivity. They found that in the de-obstructed bladders without overactivity the expression pattern was far from normalized, with 3,472 upregulated and 4,025 down-regulated genes. However, in those with persistent overactivity only 145 and 72 genes were up- or down-regulated, and they speculated that the overactivity may play a protective role against the stress to the bladder induced by outflow obstruction and de-obstruction as a form of adaptive neuroplasticity. Our data on the expression profile after de-obstruction are in general agreement with those of Lee et al. (21). Since our data did not allow a comparison between the de-obstructed groups with and without overactivity, a confirmation and further analysis of the results of Lee et al. (21) would be desirable since they may give clues to changes that can be targets for treatment.
Lee et al. (21) commented on the gene expression profiles in different functional categories but did not discuss their potential for translation into targets for therapeutic intervention. Below a number of the mRNA changes in our material believed to reflect protein changes in the obstructed and de-obstructed bladders with such a potential have been selected for further discussion.
Connexins
In the microarrays it was found that mRNA for this protein increased to 1.79 in the obstructed bladders but normalized (0.91) following de-obstruction. In denervated bladders, connexin 43 mRNA increased 3.30 fold in comparison with the controls, suggesting that cell to cell contacts increase in the absence of nerves. Connexins are the principal protein component of the gap junction (GJ), responsible for the functional generation of gap junction channels (46). GJs arise from the interaction between 2 hemichannels (HCs) of neighboring cells, which in turn are composed of 6 connexin (Cx) proteins (47). There are 12 different subunits in the connexin family, and their diversity is still poorly understood. Several connexins, including Cx40, Cx43, and Cx45, are confirmed to be in the detrusor myocytes, and Cx43 is the main one (45). These connexins are assumed to be essential for the cell-cell communication observed between detrusor cells. In the human bladder Cx43 interstitial cells are the major site for the gap junction protein Cx43, which would allow propagation of electrical and Ca2+ signals (48, 49).
The microarrays revealed that mRNA for Cx43 increased in the obstructed bladders but normalized following de-obstruction. Denervated rat bladders have high expression of connexin 43 mRNA, and this is associated with a high spontaneous contractile activity. Thus, detrusor muscle from denervated bladders produce in vitro spontaneous phasic contractions with amplitudes up to 40% of maximal activation (50).
It is unclear whether Cxs can be reasonable targets for drugs meant for de-obstruction LUTS. Cx antagonists have been used experimentally with some success (51–53). Kim et al. (53) found an upregulation of Cx43 and Cx26 after BOO in rat bladder, and that connexin inhibitors glycyrrhetinic acid and oleamide relieved BOO-induced detrusor overactivity. However, the many Cx inhibitors available do not always strictly distinguish between gap junctions, connexin hemichannels and pannexin channels, and may have effects on other targets as well (54). An exception lies with mimetic peptides, which reproduce specific amino acid sequences in connexin or pannexin primary protein structure. The effects of such peptides have been explored in several organs/tissues (54) but, to the best of our knowledge, not in the urinary bladder.
Membrane receptors
Following BOO in rats there are non-uniform changes in bladder membrane receptors (55). Some receptors are up-regulated and can be associated with increased agonist responses whereas other can be down-regulated leading to decreased responses. Some of these BOO-induced changes remain after de-obstruction. Although the functional consequences of these changes are not known they can be targets for drugs. At present it has not been established whether agonists or antagonists of the receptors would inhibit the persistent OAB after de-obstruction.
Endothelins
In the microarrays endothelin-1 mRNA was significantly increased in the obstructed bladders and stayed so after de-obstruction. Endothelin receptor typ A mRNA increased significantly (2.05) in obstructed bladders. The mRNA level remained significantly increased during de-obstruction. Bladder denervation induced an even higher level (2.51) of endothelin A receptor mRNA. In the normal bladder endothelins (ET-1, ET-2, ET-3) and endothelin receptors (ETA, ETB) have been demonstrated (56). Through their receptors, ETs can initiate both short-term (contraction) and long-term (mitogenesis) events in targets cells in the bladder and urethra. In the obstructed rat bladder, we found that ET-1 mRNA was significantly increased, and it remained elevated also after de-obstruction. ETA mRNA increased significantly in obstructed bladders and the level remained significantly increased during de-obstruction. Bladder denervation induced an even higher level of endothelin A receptor mRNA.
Saenz de Tejada et al. (57) suggested that ETs may act as an autocrine hormone in the regulation of bladder wall structure and smooth muscle tone, and that it may regulate cholinergic neurotransmission by a paracrine mechanism. The contractile effect of ETs seems to be mediated mainly by the ETA receptor (58), and the molecular mechanism by which ET-1 causes contraction involves the ROK pathway (59).
The functional role of ETs in the detrusor has not been established. However, in the rat bladder Donoso et al. (60) found that ET-1 potentiated the contractions evoked by both transmural nerve stimulation (ACh and ATP) and applications of ATP at peptide concentrations 10-fold below those needed to produce an increase in bladder tone. Locally generated ET-1 may thus not only exert a direct contractile effect on the myocyte, but also indirectly increase the effects of other mediators that influence myogenic activity.
The ETA selective receptor antagonist, YM598, dose-dependently reduced the frequency of NVCs in BOO rats without effects on other voiding parameters (61). Further, inhibition of the endothelin-converting enzyme, which metabolizes big endothelin to ET-1, decreased bladder overactivity in BOO rats (62). Several ET receptor antagonists have been tested in humans (mostly in cancer treatment) which means that they are available for human use. Since ET-1 receptor antagonists can reduce spontaneous activity in the detrusor (61) and decrease DO in animal models (63), they have a promising profile for treatment of bladder disorders, but their effect on LUT dysfunction in humans remains to be established.
Purinergic receptors
Among purinergic receptors, the P2x1 receptor mRNA level was unaffected by obstruction or de-obstruction. On the other hand, the P2x5 receptor mRNA was significantly increased in both obstructed and de-obstructed bladders. P2y1, P2y2, P2y4, and P2y6 mRNAs were not affected by obstruction or de-obstruction.
ATP is released from the umbrella cells when the urothelium is stretched during bladder filling. P2X3 and several other P2X receptors are expressed in the lamina propria, urothelium and detrusor smooth muscle of rat and human bladder. ATP stimulates purinergic receptors on suburothelial sensory nerves, leading to the sensation of bladder filling and urgency but also to initiation of the voiding reflex (64). In the rat microarrays the P2x1 receptor mRNA level was unaffected by obstruction or de-obstruction. On the other hand, the P2x5 receptor mRNA was significantly increased in both obstructed and de-obstructed bladders. P2y1, P2y2, P2y4, and P2y6 mRNAs were not affected by obstruction or de-obstruction. The adenosine A2B receptor mRNA was similar in control and obstructed bladder, but significantly higher in de-obstructed bladders. Adenosine relaxes agonist-induced detrusor contractions (65) and attenuates stretch-activated urothelial ATP release (66). These effects are exerted partly via A2B receptors (67).
The purinergic signaling in the bladder may be changed in different LUT disorders. In the diseased human detrusor P2X3 receptors seem to mediate the non-adrenergic, non-cholinergic (NANC) contraction (68, 69), and for decades P2X3 receptors have been the target for drugs intended for treatment of LUT disorders. Selective P2X3 antagonists, such as A-317491, and AF-353 were shown to be effective in several animal models of bladder dysfunction, but not taken into clinical use. The new selective P2X3 antagonists, gefapixant reduced urinary urgency and a positive trend in the improvement of pain in patients with IC/BPS (70). Also eliapixant (BAY 1817080) is a highly potent and selective P2X3 receptor antagonist that has promising effects on disorders associated with hypersensitive nerve fibers in different relevant rodent models (71). This would make these drugs, particularly eliapixant, of interest for treatment of different types of bladder disorders, e.g., OAB and LUTS (including de-obstruction LUTS) and painful bladder syndrome/interstitial cystitis (PBS/IC). However, such clinical studies are still to be performed.
β3-Adrenoceptors
The β3-adrenoreceptor mRNA was significantly decreased (10 days 0.39, 6 weeks 0.56) in the obstructed bladders. The de-obstructed bladders were not (0.76) significantly lower in mRNA compared with the control bladders. The urinary bladder expresses mRNA for all three β‐adrenoceptor subtypes (72), but the predictive value of β3‐adrenoceptor mRNA for the presence of functional receptor protein remains uncertain (73). In the microarrays of the obstructed rat bladder, β‐adrenoceptor mRNA was significantly decreased. Compared with the mRNA in control bladders the mRNA in the de-obstructed bladders was also reduced (0.762). Zeng et al. (58) found that the β3‐adrenoceptor CL316243 caused a concentration- dependent relaxation in control rat bladder strips but had little effect in strips from obstructed bladders This is in agreement with the decrease in β‐adrenoceptor mRNA in the obstructed bladder and, if valid also in humans, may explain why the storage symptoms improvement was less in patients with BOO than in those without (74). Since there was a decrease (although n.s.) in β‐adrenoceptor mRNA also in the de-obstructed bladder, it may be expected that the effect on symptoms persisting after prostatectomy may be small.
Arginine vasopressin
The arginine vasopressin mRNA level was significantly decreased (0.53) in the obstructed bladders but had normalized in the de-obstructed bladders.
The biological effects of AVP are mediated by three receptor subtypes: the V1a and V1b receptors and the V2 receptor (72). The V2 receptor is located mainly in the collecting ducts and distal tubule and facilitates water reabsorption but has also been demonstrated in the bladder mucosa (73). The V1 vasopressin receptors are present in the rat and rabbit bladder (75, 76), and mediates the contractile effects of AVP on the detrusor. A decreased contractile effect of AVP has been reported in the obstructed rat bladder (77). Zeng et al. (58) demonstrated that this was associated with a reduced level of V1a receptor mRNA and protein. mRNA for AVP on the other hand was unchanged, arguing that vasopressin is synthesized in the rat bladder but that its synthesis is unchanged after obstruction. After de-obstruction the AVP mRNA levels are normalized.
Ikeda et al. (78) found a direct role for VRs in regulating detrusor tone with V1Rs causing contraction and V2Rs relaxation. They also found that in aged bladders, the relaxation response to V2R activation is attenuated by lack of release of a mucosal factor that contributes to V2R-induced relaxation and speculated that administering an exogenous V2R agonist (e.g., desmopressin) could counteract this defect and potentially increase nighttime bladder capacity through detrusor relaxation. Together with the V2R receptor decrease in urine production this could contribute to reduced nocturnal voiding frequency.
The RhoA/Rho-kinase pathway
Rho is a family of small signaling G proteins. ROCK1 and ROCK2 are major downstream effectors of the small GTPase RhoA. Both ROCK1 and ROCK 2 isozymes have been demonstrated in the rat bladder but the complete functional differences between the ROCK1 and ROCK2 isoforms have not been elucidated (59).
Rho-kinase and the RhoA/ROCK pathway appears to play a critical role in normal bladder function. This pathway is important for the maintenance of basal detrusor muscle tone and also serves as a common final pathway of various contractile stimuli in animals as well as humans by acting as a “calcium sensitizer”. The RhoA/Rho-kinase pathway can be activated by e.g, muscarinic acetylcholine receptors, endothelin, angiotensin, P2-type purinoceptors, lysophosphatidic acid, and prostaglandin F2a (59, 79, 80).
Our microarrays showed a significant upregulation of ROCK1 both after 10 days (1.532) and 6 weeks (1.566) obstruction; after de-obstruction the increase (1.361) was non-significant. ROCK2 showed a non-significant increase 10 days and 6 weeks after BOO, but a significant increase (1.225) after de-obstruction. Similarly, Takahashi et al. (81) found an increased expression of RhoA, ROCK1 (ROKa), and ROCK2 (ROKb) after 4 weeks of BOO in the male rat. In the obstructed male rabbit bladder, Guven et al. (82) found that ROKa was upregulated but there was a simultaneous significant decrease in the expression of ROKb. The importance of this is unclear.
Inhibitors of Rho kinase such as Y 27,632, GSK 269,962 and fasudil inhibit bladder contraction in in vitro preparations from multiple species and Rho-kinase would be an interesting target for drugs aimed at treatment of LUTS/OAB (80, 83). Fasudil has been approved in some countries for clinical use in indications unrelated to the bladder. It seems that the drug (or the principle of Rho-kinase inhibition) has a therapeutic potential in the management of LUTD, including symptoms persisting after de-obstruction. However, no clinical studies of Rho kinase inhibitors in LUTD seem to have been performed and such studies are urgently needed to prove the principle.
Conclusions
Whole bladder arrays is a method to find possible changes of mRNA expression simultaneously for a huge number of proteins in the obstructed and de-obstructed bladders. The arrays may help identifying cellular mechanisms that could then be targets for drugs meant for treatment of persistent DO and LUTS after de-obstruction. Based on available array information it seems worthwhile to re-assess the development potential for e.g., endothelin antagonists, purinergic receptor antagonists and Rho-kinase inhibitors.
Author contributions
All authors listed have made a substantial, direct, and intellectual contribution to the work and approved it for publication.
Acknowledgments
We acknowledge the input on the morphological data made by Professor Giorgio Gabella.
Conflict of interest
The authors declare that the research was conducted in the absence of any commercial or financial relationships that could be construed as a potential conflict of interest.
Publisher’s note
All claims expressed in this article are solely those of the authors and do not necessarily represent those of their affiliated organizations, or those of the publisher, the editors and the reviewers. Any product that may be evaluated in this article, or claim that may be made by its manufacturer, is not guaranteed or endorsed by the publisher.
References
1. Andersson KE. Storage and voiding symptoms: pathophysiologic aspects. Urology (2003) 62(5 Suppl 2):3–10. doi: 10.1016/j.urology.2003.09.030
2. Abrams PH, Farrar DJ, Turner-Warwick RT, Whiteside CG, Feneley RC. The results of prostatectomy: a symptomatic and urodynamic analysis of 152 patients. J Urol (1979) 121(5):640–2. doi: 10.1016/s0022-5347(17)56918-9
3. Madersbacher S, Marberger M. Is transurethral resection of the prostate still justified? BJU Int (1999) 83(3):227–37. doi: 10.1046/j.1464-410x.1999.00908.x
4. Yang Q, Peters TJ, Donovan JL, Wilt TJ, Abrams P. Transurethral incision compared with transurethral resection of the prostate for bladder outlet obstruction: A systematic review and meta-analysis of randomized controlled trials. J Urol (2001) 165(5):1526–32. doi: 10.1016/S0022-5347(05)66342-2
5. Tubaro A, Carter S, Hind A, Vicentini C, Miano L. A prospective study of the safety and efficacy of suprapubic transvesical prostatectomy in patients with benign prostatic hyperplasia. J Urol (2001) 166(1):172–6. doi: 10.1016/S0022-5347(05)66102-2
6. Antunes AA, Iscaife A, Reis ST, Albertini A, Nunes MA, Lucon AM, et al. Can we predict which patients will experience resolution of detrusor overactivity after transurethral resection of the prostate? J Urol (2015) 193(6):2028–32. doi: 10.1016/j.juro.2014.12.095
7. Chai TC, Gemalmaz H, Andersson KE, Tuttle JB, Steers WD. Persistently increased voiding frequency despite relief of bladder outlet obstruction. J Urol (1999) 161(5):1689–93.
8. Seaman EK, Jacobs BZ, Blaivas JG, Kaplan SA. Persistence or recurrence of symptoms after transurethral resection of the prostate: a urodynamic assessment. J Urol (1994) 152(3):935–7. doi: 10.1016/s0022-5347(17)32614-9
9. Cumming JA, Chisholm GD. Changes in detrusor innervation with relief of outflow tract obstruction. Br J Urol (1992) 69(1):7–11. doi: 10.1111/j.1464-410x.1992.tb15448.x
10. Speakman MJ, Brading AF, Dixon JS, Gilpin SA, Gilpin CJ, Gosling JA. Cystometric, physiological and morphological studies after relief of bladder outflow obstruction in the pig. Br J Urol (1991) 68(3):243–7. doi: 10.1111/j.1464-410x.1991.tb15315.x
11. Jin LH, Andersson KE, Han JU, Kwon YH, Park CS, Shin HY, et al. Persistent detrusor overactivity in rats after relief of partial urethral obstruction. Am J Physiol Regul Integr Comp Physiol (2011) 301(4):R896–904. doi: 10.1152/ajpregu.00046.2011
12. Uvelius B, Lindner P, Mattiasson A. Collagen content in the rat urinary bladder following removal of an experimental infravesical outlet obstruction. Urol Int (1991) 47(4):245–9. doi: 10.1159/000282230
13. Gabella G, Uvelius B. Reversal of muscle hypertrophy in the rat urinary bladder after removal of urethral obstruction. Cell Tissue Res (1994) 277(2):333–9. doi: 10.1007/BF00327781
14. Kudo T, Shimoda N, Nisizawa O, Kato T. Function and nerve growth factor of the rat urinary bladder during urethral obstruction and its relief]. Nihon Hinyokika Gakkai Zasshi (1999) 90(7):651–6. doi: 10.5980/jpnjurol1989.90.651
15. Kim JC, Yoon JY, Seo SI, Hwang TK, Park YH. Effects of partial bladder outlet obstruction and its relief on types I and III collagen and detrusor contractility in the rat. Neurourol Urodyn (2000) 19(1):29–42. doi: 10.1002/(sici)1520-6777(2000)19:1<29:aid-nau5>3.0.co;2-
16. Lee SR, Hong CH, Choi YD, Kim JH. Increased urinary nerve growth factor as a predictor of persistent detrusor overactivity after bladder outlet obstruction relief in a rat model. J Urol (2010) 183(6):2440–4. doi: 10.1016/j.juro.2010.02.003
17. Schröder A, Aitken KJ, Jiang JX, Sidler M, Tölg C, Siebenaller A, et al. Persistent myopathy despite release of partial obstruction: in vivo reversal of dysfunction and transcriptional responses using rapamycin. FASEB J (2020) 34(3):3594–615. doi: 10.1096/fj.201900547RR
18. Malmgren A, Sjögren C, Uvelius B, Mattiasson A, Andersson KE, Andersson PO. Cystometrical evaluation of bladder instability in rats with infravesical outflow obstruction. J Urol (1987) 137(6):1291–4. doi: 10.1016/s0022-5347(17)44485-5
19. Saito M, Longhurst PA, Tammela TL, Wein AJ, Levin RM. Effects of partial outlet obstruction of the rat urinary bladder on micturition characteristics, DNA synthesis and the contractile response to field stimulation and pharmacological agents. J Urol (1993) 150(3):1045–51. doi: 10.1016/s0022-5347(17)35683-5
20. Saito M, Ohmura M, Kondo A. Effects of long-term partial outflow obstruction on bladder function in the rat. Neurourol Urodyn (1996) 15(2):157–65. doi: 10.1002/(SICI)1520-6777(1996)15:2<157::AID-NAU5>3.0.CO;2-E
21. Lee T, Lim US, Kang DH, Jung HD, Kim H, Choi BH, et al. Near-normalized gene expression profiles in bladder with detrusor overactivity in rats with bladder outlet obstruction after deobstruction. Int Neurourol J (2017) 21(4):247–58. doi: 10.5213/inj.1732774.387
22. Malmgren A, Uvelius B, Andersson KE, Andersson PO. On the reversibility of functional bladder changes induced by infravesical outflow obstruction in the rat. J Urol (1990) 143(5):1026–31. doi: 10.1016/s0022-5347(17)40176-5
23. Ozturk H, Ozturk H, Guneli E, Yagmur Y, Buyukbayram H. Expression of CD44 and e-cadherin cell adhesion molecules in hypertrophied bladders during chronic partial urethral obstruction and after release of partial obstruction in rats. Urology (2005) 65(5):1013–8. doi: 10.1016/j.urology.2004.12.006
24. Fry CH, McCloskey KD. Spontaneous activity and the urinary bladder. Adv Exp Med Biol (2019) 1124:121–47. doi: 10.1007/978-981-13-5895-1_5
25. Drake MJ, Hedlund P, Harvey IJ, Pandita RK, Andersson KE. Gillespie JI. partial outlet obstruction enhances modular autonomous activity in the isolated rat bladder. J Urol (2003) 170(1):276–9. doi: 10.1097/01.ju.0000069722.35137.e0
26. Fatoyinbo HO, Brown RG, Simpson DJW, van Brunt B. Pattern formation in a spatially extended model of pacemaker dynamics in smooth muscle cells. Bull Math Biol (2022) 84(8):86. doi: 10.1007/s11538-022-01043-1
27. Andersson KE. Detrusor myocyte activity and afferent signaling. Neurourol Urodyn (2010) 29(1):97–106. doi: 10.1002/nau.20784
28. Bramich NJ, Brading AF. Electrical properties of smooth muscle in the guinea-pig urinary bladder. J Physiol (1996) 492(Pt 1):185–98. doi: 10.1113/jphysiol.1996.sp021300
29. Hashitani H, Brading AF. Electrical properties of detrusor smooth muscles from the pig and human urinary bladder. Br J Pharmacol (2003) 140(1):146–58. doi: 10.1038/sj.bjp.0705319
30. Gabella G, Uvelius B. Urinary bladder of rat: fine structure of normal and hypertrophic musculature. Cell Tissue Res (1990) 262(1):67–79. doi: 10.1007/BF00327747
31. Li L, Jiang C, Hao P, Li W, Song C, Song B. Changes of gap junctional cell-cell communication in overactive detrusor in rats. Am J Physiol Cell Physiol (2007) 293(5):C1627–35. doi: 10.1152/ajpcell.00122.2007
32. Gabella G, Davis C. Distribution of afferent axons in the bladder of rats. J Neurocytol (1998) 27(3):141–55. doi: 10.1023/a:1006903507321
33. Gabella G. Afferent nerve fibres in the wall of the rat urinary bladder. Cell Tissue Res (2019) 376(1):25–35. doi: 10.1007/s00441-018-2965-0
34. Gabella G. The structural relations between nerve fibres and muscle cells in the urinary bladder of the rat. J Neurocytol (1995) 24(3):159–87. doi: 10.1007/BF01181533
35. Turner WH, Brading AF. Smooth muscle of the bladder in the normal and the diseased state: pathophysiology, diagnosis and treatment. Pharmacol Ther (1997) 75(2):77–110. doi: 10.1016/s0163-7258(97)00038-7
36. Pandita RK, Fujiwara M, Alm P, Andersson KE. Cystometric evaluation of bladder function in non-anesthetized mice with and without bladder outlet obstruction. J Urol (2000) 164(4):1385–9.
37. Gabella G. Structure of the intramural nerves of the rat bladder. J Neurocytol (1999) 28(8):615–37. doi: 10.1023/a:1007084130642
38. Gillespie JI, van Koeveringe GA, de Wachter SG, de Vente J. On the origins of the sensory output from the bladder: the concept of afferent noise. BJU Int (2009) 103(10):1324–33. doi: 10.1111/j.1464-410X.2009.08377.x
39. Mattiasson A, Uvelius B. Changes in contractile properties in hypertrophic rat urinary bladder. J Urol (1982) 128(6):1340–2. doi: 10.1016/s0022-5347(17)53503-x
40. Uvelius B, Persson L, Mattiasson A. Smooth muscle cell hypertrophy and hyperplasia in the rat detrusor after short-time infravesical outflow obstruction. J Urol (1984) 131(1):173–6. doi: 10.1016/s0022-5347(17)50253-0
41. Berggren T, Uvelius B, Arner A. Denervation and outlet obstruction induce a net synthesis of contractile and cytoskeletal proteins in the urinary bladder of the male rat. Urol Res (1996) 24(3):135–40. doi: 10.1007/BF00304076
42. Uvelius B, Mattiasson A. Collagen content in the rat urinary bladder subjected to infravesical outflow obstruction. J Urol (1984) 132(3):587–90. doi: 10.1016/s0022-5347(17)49753-9
43. Gabella G, Berggren T, Uvelius B. Hypertrophy and reversal of hypertrophy in rat pelvic ganglion neurons. J Neurocytol (1992) 21(9):649–62. doi: 10.1007/BF01191726
44. Steers WD, Ciambotti J, Erdman S, de Groat WC. Morphological plasticity in efferent pathways to the urinary bladder of the rat following urethral obstruction. J Neurosci (1990) 10(6):1943–51. doi: 10.1523/JNEUROSCI.10-06-01943.1990
45. Wang HZ, Brink PR, Christ GJ. Gap junction channel activity in short-term cultured human detrusor myocyte cell pairs: gating and unitary conductances. Am J Physiol Cell Physiol (2006) 291(6):C1366–76. doi: 10.1152/ajpcell.00027.2006
46. Goodenough DA, Paul DL. Gap junctions. Cold Spring Harb Perspect Biol (2009) 1(1):a002576. doi: 10.1101/cshperspect.a002576
47. Nielsen MS, Axelsen LN, Sorgen PL, Verma V, Delmar M, Holstein-Rathlou NH. Gap junctions. Compr Physiol (2012) 2(3):1981–2035. doi: 10.1002/cphy.c110051
48. Sui GP, Rothery S, Dupont E, Fry CH, Severs NJ. Gap junctions and connexin expression in human suburothelial interstitial cells. BJU Int (2002) 90(1):118–29. doi: 10.1046/j.1464-410x.2002.02834.x
49. Heinrich M, Oberbach A, Schlichting N, Stolzenburg JU, Neuhaus J. Cytokine effects on gap junction communication and connexin expression in human bladder smooth muscle cells and suburothelial myofibroblasts. PloS One (2011) 6(6):e20792. doi: 10.1371/journal.pone.0020792
50. Ekström J, Uvelius B. Length-tension relations of smooth muscle from normal and denervated rat urinary bladders. Acta Physiol Scand (1981) 112(4):443–7. doi: 10.1111/j.1748-1716.1981.tb06842.x
51. Lagaud G, Davies KP, Venkateswarlu K, Christ GJ. The physiology, pathophysiology and therapeutic potential of gap junctions in smooth muscle. Curr Drug Targets (20022003) 3(6):427–40. doi: 10.2174/1389450023347399
52. Christ GJ, Day NS, Day M, Zhao W, Persson K, Pandita RK, et al. Increased connexin43-mediated intercellular communication in a rat model of bladder overactivity in vivo. Am J Physiol Regul Integr Comp Physiol (2003) 284(5):R1241–8. doi: 10.1152/ajpregu.00030.2002
53. Kim SJ, Park EY, Hwang TK, Kim JC. Therapeutic effects of connexin inhibitors on detrusor overactivity induced by bladder outlet obstruction in rats. Urology (2011) 78(2):475.e1–7. doi: 10.1016/j.urology.2011.03.029
54. Willebrords J, Maes M, Crespo Yanguas S, Vinken M. Inhibitors of connexin and pannexin channels as potential therapeutics. Pharmacol Ther (2017) 180:144–60. doi: 10.1016/j.pharmthera.2017.07.001
55. Zeng J, Ekman M, Jiang C, Uvelius B, Swärd K. Non-uniform changes in membrane receptors in the rat urinary bladder following outlet obstruction. Eur J Pharmacol (2015) 762:82–8. doi: 10.1016/j.ejphar.2015.05.037
56. Andersson KE, Arner A. Urinary bladder contraction and relaxation: physiology and pathophysiology. Physiol Rev (2004) 84(3):935–86. doi: 10.1152/physrev.00038.2003
57. Saenz de Tejada I, Mueller JD, de Las Morenas A, Machado M, Moreland RB, Krane RJ, et al. Endothelin in the urinary bladder. i. synthesis of endothelin-1 by epithelia, muscle and fibroblasts suggests autocrine and paracrine cellular regulation. J Urol (1992) 148(4):1290–8. doi: 10.1016/s0022-5347(17)36895-7
58. Traish A, Moran E, Krane RJ, Saenz de Tejada I. Endothelin in the urinary bladder. II. characterization of endothelin receptor subtypes. J Urol (1992) 148(4):1299–306. doi: 10.1016/s0022-5347(17)36896-9
59. Zhang X, DiSanto ME. Rho-kinase, a common final path of various contractile bladder and ureter stimuli. Handb Exp Pharmacol (2011) 202):543–68. doi: 10.1007/978-3-642-16499-6_24
60. Donoso MV, Salas C, Sepúlveda G, Lewin J, Fournier A, Huidobro-Toro JP. Involvement of ETA receptors in the facilitation by endothelin-1 of non-adrenergic non-cholinergic transmission in the rat urinary bladder. Br J Pharmacol (1994) 111(2):473–82. doi: 10.1111/j.1476-5381.1994.tb14761.x
61. Ukai M, Yuyama H, Fujimori A, Koakutsu A, Sanagi M, Ohtake A, et al. In vitro and in vivo effects of endothelin-1 and YM598, a selective endothelin ET a receptor antagonist, on the lower urinary tract. Eur J Pharmacol (2008) 580(3):394–400. doi: 10.1016/j.ejphar.2007.11.021
62. Schröder A, Tajimi M, Matsumoto H, Schröder C, Brands M, Andersson KE. Protective effect of an oral endothelin converting enzyme inhibitor on rat detrusor function after outlet obstruction. J Urol (2004) 172(3):1171–4. doi: 10.1097/01.ju.0000133561.32285.23
63. Ogawa T, Sasatomi K, Hiragata S, Seki S, Nishizawa O, Chermansky CJ, et al. Therapeutic effects of endothelin-a receptor antagonist on bladder overactivity in rats with chronic spinal cord injury. Urology (2008) 71(2):341–5. doi: 10.1016/j.urology.2007.10.025
64. Fry CH, McCloskey KD. Purinergic signalling in the urinary bladder - when function becomes dysfunction. Auton Neurosci (2021) 235:102852. doi: 10.1016/j.autneu.2021.102852
65. Rubinstein R, Shalev M, Nissenkorn I, Cohen S. Effect of exogenous adenosine and its monophosphate on the contractile response to acetylcholine in the human isolated detrusor muscle strips. J Auton Pharmacol (1998) 18(2):99–104. doi: 10.1046/j.1365-2680.1998.1820099.x
66. Dunning-Davies BM, Fry CH, Mansour D, Ferguson DR. The regulation of ATP release from the urothelium by adenosine and transepithelial potential. BJU Int (2013) 111(3):505–13. doi: 10.1111/j.1464-410X.2012.11421.x
67. Pakzad M, Ikeda Y, McCarthy C, Kitney DG, Jabr RI, Fry CH. Contractile effects and receptor analysis of adenosine-receptors in human detrusor muscle from stable and neuropathic bladders. Naunyn Schmiedebergs Arch Pharmacol (2016) 389(8):921–9. doi: 10.1007/s00210-016-1255-1
68. Sjögren C, Andersson KE, Husted S, Mattiasson A, Moller-Madsen B. Atropine resistance of transmurally stimulated isolated human bladder muscle. J Urol (1982) 128(6):1368–71. doi: 10.1016/s0022-5347(17)53509-0
69. Bayliss M, Wu C, Newgreen D, Mundy AR, Fry CH. A quantitative study of atropine-resistant contractile responses in human detrusor smooth muscle, from stable, unstable and obstructed bladders. J Urol (1999) 162(5):1833–9.
70. Hanno PM. Afferent clinical data for lead candidate, AF-219, demonstrate improvements in pain and urgency in interstitial cystitis/bladder pain syndrome (IC/BPS). Afferent Pharm. http://www.businesswire.com/news/home/20150226005187/en/Afferent-Clinical-Data-Lead-Candidate-AF-219-Demonstrate.
71. Davenport AJ, Neagoe I, Bräuer N, Koch M, Rotgeri A, Nagel J, et al. Eliapixant is a selective P2X3 receptor antagonist for the treatment of disorders associated with hypersensitive nerve fibers. Sci Rep (2021) 11(1):19877. doi: 10.1038/s41598-021-99177-0
72. Birnbaumer M. Vasopressin receptors. Trends Endocrinol Metab (2000) 11(10):406–10. doi: 10.1016/s1043-2760(00)00304-0
73. Birder LA, Wolf-Johnston AS, Jackson EK, Wein AJ, Dmochowski R. Aging increases the expression of vasopressin receptors in both the kidney and urinary bladder. Neurourol Urodyn (2019) 38(1):393–7. doi: 10.1002/nau.23830
74. Liao CH, Kuo HC. Mirabegron 25 mg monotherapy is safe but less effective in Male patients with overactive bladder and bladder outlet obstruction. Urology (2018) 117:115–9. doi: 10.1016/j.urology.2018.03.038
75. Uvelius B, Lundin S, Andersson KE. Content and contractile effect of arginine vasopressin in rat urinary bladder. Eur J Pharmacol (1990) 182(3):549–54. doi: 10.1016/0014-2999(90)90054-a
76. Holmquist F, Lundin S, Larsson B, Hedlund H, Andersson KE. Studies on binding sites, contents, and effects of AVP in isolated bladder and urethra from rabbits and humans. Am J Physiol (1991) 261(4 Pt 2):R865–74. doi: 10.1152/ajpregu.1991.261.4.R865
77. Berggren T, Andersson KE, Lundin S, Uvelius B. Effect and content of arginine vasopressin in normal and obstructed rat urinary bladder: an in vivo and in vitro investigation. J Urol (1993) 150(5 Pt 1):1540–3. doi: 10.1016/s0022-5347(17)35837-8
78. Ikeda Y, Zabbarova I, de Rijk M, Kanai A, Wolf-Johnston A, Weiss JP, et al. Effects of vasopressin receptor agonists on detrusor smooth muscle tone in young and aged bladders: Implications for nocturia treatment. Continence (Amst) (2022) 2:100032. doi: 10.1016/j.cont.2022.100032
79. Peters SL, Schmidt M, Michel MC. Rho kinase: a target for treating urinary bladder dysfunction? Trends Pharmacol Sci (2006) 27(9):492–7. doi: 10.1016/j.tips.2006.07.002
80. Christ GJ, Andersson KE. Rho-kinase and effects of rho-kinase inhibition on the lower urinary tract. Neurourol Urodyn (2007) 26(6 Suppl):948–54. doi: 10.1002/nau.20475
81. Takahashi N, Shiomi H, Kushida N, Liu F, Ishibashi K, Yanagida T, et al. Obstruction alters muscarinic receptor-coupled RhoA/Rho-kinase pathway in the urinary bladder of the rat. Neurourol Urodyn (2009) 28(3):257–62. doi: 10.1002/nau.20625
82. Guven A, Onal B, Kalorin C, Whitbeck C, Chichester P, Kogan B, et al. Long term partial bladder outlet obstruction induced contractile dysfunction in male rabbits: a role for rho-kinase. Neurourol Urodyn (2007) 26(7):1043–9. doi: 10.1002/nau.20435
Keywords: rat model, bladder, bladder outflow obstruction, microarrays, cystometry, electron microscopy
Citation: Andersson K-E and Uvelius B (2022) Changes in the rat urinary bladder after the relief of outflow obstruction – tracing targets for treatment of persistent symptoms in patients. Front. Urol. 2:1027086. doi: 10.3389/fruro.2022.1027086
Received: 24 August 2022; Accepted: 10 October 2022;
Published: 20 October 2022.
Edited by:
Naoki Yoshimura, University of Pittsburgh, United StatesReviewed by:
Ana Charrua, University of Porto, PortugalTack Lee, Inha University Hospital, South Korea
Copyright © 2022 Andersson and Uvelius. This is an open-access article distributed under the terms of the Creative Commons Attribution License (CC BY). The use, distribution or reproduction in other forums is permitted, provided the original author(s) and the copyright owner(s) are credited and that the original publication in this journal is cited, in accordance with accepted academic practice. No use, distribution or reproduction is permitted which does not comply with these terms.
*Correspondence: Karl-Erik Andersson, a2FybC1lcmlrLmFuZGVyc3NvbkBtZWQubHUuc2U=