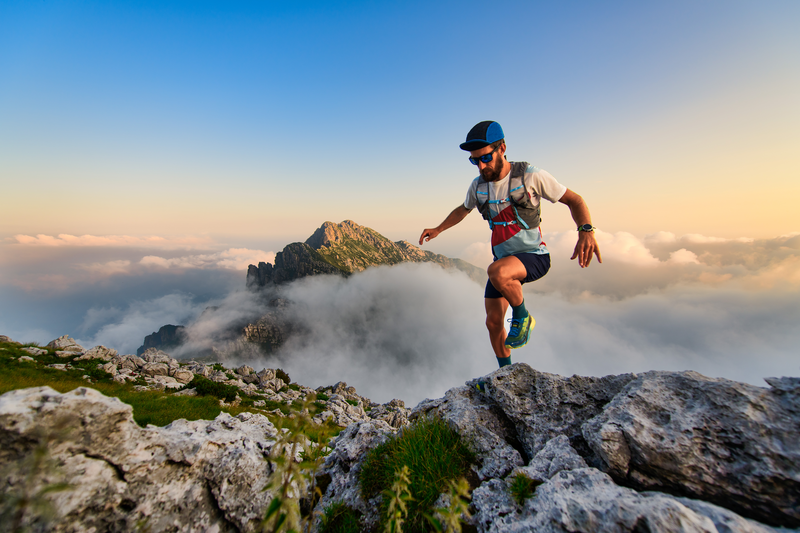
95% of researchers rate our articles as excellent or good
Learn more about the work of our research integrity team to safeguard the quality of each article we publish.
Find out more
REVIEW article
Front. Tuberc. , 24 December 2024
Sec. Epidemiology of Tuberculosis
Volume 2 - 2024 | https://doi.org/10.3389/ftubr.2024.1487518
Despite impacting mankind since ancient times, tuberculosis (TB) persists as the leading cause of death from an infectious disease. TB can remain latent and further research is required to understand activation risk and the risks vs. the benefits of treating latent infection. Drug resistance poses an escalating threat to treating active disease and achieving cure. Recent advances in molecular and epidemiological techniques facilitate early diagnosis, drug susceptibility testing and an opportunity to better understand transmission dynamics. Research is ongoing to develop safe, efficacious tolerable drug regimens and the challenges of antibiotic resistance have led to a resurgent interest in therapeutic alternatives. Vaccine development is challenged by the pathogen's genetic diversity, the heterogeneity of host susceptibility and the extreme complexities that occur across the interactions between TB and its host. Across all stages of TB pathogenesis, developments in artificial intelligence, geographic information systems, digital health technologies, renewable energy solutions and nano medicine are providing opportunities to improve TB control. Resource constraints however often challenge the opportunity to access these new technologies by those most in need. The societal inequalities in accessing new technologies further compound socio-economic and health related TB determinants Addressing these complex determinants which include malnutrition, HIV infection, diabetes, substance abuse, poor environmental conditions and multi-factorial barriers to health care access, will require political will, sufficient funding, and a holistic multisectoral response.
With respect to mortality and morbidity, tuberculosis (TB) is one of the most important infectious diseases that has affected mankind since ancient times. Although the genus Mycobacterium is hypothesized to have originated more than 150 million years ago (1), the disease still continues to take a huge toll on society and in 2023 was estimated to have resulted in the loss of 1.25 million lives (2). In 2019, 66 million disability life years (DALYs) were attributed to TB (3), but due to the exclusion of post-TB sequelae in the DALY calculation, the true burden of morbidity is likely to be significantly higher (4). The pathogen's ability to evade the hosts immune response and elicit effective transmission, and the inequitable nature of mankind have contributed to the disease eluding eradication (5, 6).
The Mycobacterium tuberculosis complex (MTBC) contains a group of genetically similar bacteria capable of causing TB across a range of mammalian hosts (7). M. tuberculosis (Mtb) is the main etiological agent of TB in humans, and M. africanum is also prevalent in Africa, with each of these species having different lineages that have distinct characteristics in terms of pathogenicity, transmissibility, drug resistance and geographic origin (8, 9).
Mtb is an obligate intracellular pathogen and although it has been established that transmission occurs by bioaerosol, the complexities of transmission are not fully understood and are considered a research priority (10). Mtb has evolved to infect, survive and replicate within human phagocytic cells and although the lungs are the primary site of infection, the bacillus are able to infect any organ in the body via hematogenous routes (10). The intracellular nature of the pathogen facilitates long-term latency which translates to active disease when host immunity is compromised (11). Host immunity is highly heterogenous and the spectrum of disease is hard to differentiate and identify, resulting in fundamental gaps in our knowledge of its features and implications (10, 12). The ability to correlate the spectrum of subclinical disease with symptoms, clinical signs and diagnostic tests is complicated in endemic settings, where exposure is difficult to identify and often multitudinous (12). Mtb encounters a range of host environments at different stages of infection and although our understanding of the host/pathogen determinants and mechanisms that promote a successful infection are poorly understood, the pathogens unique and dynamic cell envelope is thought to play a key role (13). The cell envelope is the target of most current and proposed therapies, but more work needs to be undertaken to understand the cell envelope composition across Mtb strains with different antibiotic resistance profiles (14). Antibiotic resistance is conferred by genomic mutations and propagated through replication of resistant Mtb and onward transmission (15). Mtb has acquired resistance to all the antibiotic drugs that have been used against it and there are concerns that current targeted genetic diagnostics could create their own selective pressures (15).
Although globally ubiquitous, the prevalence of infection shows wide variation by region and social context. TB is associated with low socio-economic status and is a disease that fuels the cycle of poverty with more than 80% of cases and deaths occurring in low- and middle-income countries (6). In addition to socio-economic development, TB is influenced by health-related risk factors including HIV infection, malnutrition, diabetes and drug and alcohol abuse (16). Although all age groups are at risk of infection, the disease has its greatest impact on adults in their productive years (6). In 2022, the greatest number of new cases occurred in the World Health Organization (WHO) South-East Asia Region (46%) followed by the African Region (23%), with 87% of cases occurring in 30 high TB burden countries and more than two thirds of the global total befalling eight countries (6).
Within the targets of the 2030 Sustainable Development Agenda, adopted by members of the United Nations (UN) in 2015, was a commitment to end the TB epidemic by 2030 (17). The WHO End TB Strategy builds upon and expands the scope of efforts in the context of the UN Sustainable Development Goals (SDG). The End TB Strategy has targets of a 90% reduction in TB deaths and 80% reduction in TB incidence by 2030, relative to a 2015 baseline (18). Progress toward the interim targets for the 2030 goals, is severely off track with 2015–2021 net reductions in TB incidence and death only one fifth and one-tenth of the way to the 2025 milestones respectively (19). The 2018–2022 WHO status report on TB treatment, preventative therapy and funding, also show progress to be significantly off-track (20). Examples of the shortfalls show only 19% of targeted children with multi-drug resistant TB (MDR-TB) received treatment, only 10% of targeted household contacts aged < 5 years received TB preventative treatment, and that funding for TB services only reached 44% of that targeted in 2022 (20). Modeling shows that if the 2030 mortality target is not achieved until 2045, there will be the loss of an additional 5.7 million lives and an estimated economic cost of $3.0 trillion (21).
If we are to achieve the End TB Strategy targets a holistic, multisectoral response will be required that optimizes current control program strategies, addresses social determinants, eliminates inequities and that invests in research and development (22) This review seeks to discuss the challenges and opportunities in achieving these targets and is structured to consider aspects relevant to the three End TB Strategy pillars. Pillar I: “integrated, patient-centered care and prevention,” pillar II: “bold policies and supportive systems” and pillar III: “intensified research and innovation” (22).
Efforts to combat the TB pandemic have focused on early diagnosis, appropriate treatment, active case finding and the administration of preventative therapy to people with latent infection who are at high risk of developing active disease (23). Delivering these strategies will require tools capable of detecting latent TB infection (LTBI), the ability to predict the risk of latent infection progressing to active disease, the capability to detect active disease and any drug resistance and the administration of the correct treatment regime (24).
The diagnosis of LTBI is indirect and reliant upon the measurement of an immune response to Mtb antigens (25). Currently there are no diagnostics available to differentiate between an immune response due to LTBI vs. active disease and the distinction is reliant upon clinical, bacteriological and radiological findings (25). Methods used to identify LTBI include the tuberculin skin test (TST), M. tuberculosis antigen skin test (TBST) and interferon gamma release assays (IGRAs) (25, 26). There is no recommended gold standard for LTBI testing and each of the available methods have their challenges, including the requirement for multiple appointments and a cold chain for the skin tests and cost and time sensitivity for the IGRAs (26). The global prevalence of LTBI is unknown but estimates of 25% have been made (27). Literature quotes the lifetime risk of LTBI developing into active disease to average 5%−10% but figures are based upon a single dated study (28), highlighting the need for comprehensive up-to-date research on the activation risk. Latency is not stable and bacterial replication can be intermittent, transient or progressive leading to incipient and subclinical infection prior to active disease (25). A number of approaches to estimating activation risk are being investigated including the use of gene signatures and transcriptional biomarkers, but further research is required (29, 30). The prophylactic treatment of patients with LTBI at risk of progressing to active disease is a key component of the End TB Strategy, but this needs balancing against the potential adverse effects of treatment (31). LTBI therapy is challenged by sub-optimal treatment adherence rates, the absence of a regime to treat latent MDR strains, and resource deficits as many countries prioritize programs that address active disease (32, 33). Amongst the key “at risk” populations prioritized for LTBI testing and treatment are people living with human immunodeficiency virus (PLHIV) and household contacts of bacteriologically confirmed TB cases (31) Additional high risk population groups include healthcare workers, immigrants from high TB burden countries, the homeless and illicit drug users (34). The diagnostic methods used to identify a ‘bacteriologically confirmed' case of TB include culture, sputum smear microscopy, molecular testing and lateral flow urine lipoarabinomannan (LF-LAM) assays (35). Bacteriological confirmation is required to facilitate drug susceptibility testing and in the absence of this confirmation, the case is classified as a clinical diagnosis (i.e., based on clinical symptoms, chest radiograph abnormalities) (35, 36). Culture is the gold standard diagnostic test with the capacity to detect drug resistance and new mutations, but it has the disadvantage of a long mean time to detection and for many resource limited settings sub-optimal laboratory facilities restrict its utility (37, 38). For many countries, culture is reserved for cases of treatment relapse (38). Microscopy is the primary method of diagnosis in resource constrained environments and although less sensitive than culture, it has the advantage of simplicity, reduced cost and rapidity (39). Although advances have been made in improving sensitivity (e.g., from traditional Ziehl-Neelsen staining to light-emitting diode microscopy) efficacy is operator dependant and the need for a large bacillary load limits its application for PLHIV, extrapulmonary and childhood diagnoses (40, 41). Microscopy also has the disadvantage of the inability to differentiate between MTBC and nontuberculous mycobacterium (NTB) and between live and dead bacilli (38, 42). Recent advances in molecular diagnostics include nucleic acid amplification tests (NAATs) which facilitate an early diagnosis of disease with high sensitivity and specificity (43). The WHO now recommend that rapid techniques be used as the initial diagnostic (44). NAATs have the advantage of detecting resistance to selected anti-TB drugs, the ability to test at point-of-care with a rapid turn-around and although they are not capable of differentiating between live and dead bacilli they have increased accuracy vs. microscopy especially in patients with paucibacillary disease and PLHIV (43–46). These molecular techniques are also able to utilize stool as an alternative specimen diagnostic which provides an opportunity to address the case detection gap in children, which is greatest in those under 5 years of age (47). Despite the advantages offered by NAATs, there are challenges to accessing these technologies in resource constrained environments including cost, complexity, the need for power, and reagent supply chain and storage considerations (37, 48). Another recent biomolecular development is the lateral flow urine lipoarabinomannan assay (LF-LAM) which detects lipopolysaccharide in the wall of metabolically active or degenerating mycobacterium cells (37, 49). LF-LAM has suboptimal sensitivity for general screening but has improved sensitivity in patients with human immunodeficiency virus (HIV)-TB coinfection (49). LF-LAM sensitivity has been shown to increase with decreasing CD4 cell counts and this method also has utility where sputum samples cannot be obtained (49). Another TB screening test of utility for PLHIV is the immunological marker C-reactive protein, especially in situations where other recommended screening tools such as chest X-ray are not readily available (44, 50).
Line probe assays (LPA) are molecular assays that can be used on smear-positive and culture isolates following DNA extraction and amplification to differentiate genetic mutations associated with drug resistance (46). In addition to the identification of drug resistance, LPAs can facilitate the identification of pre-extensively drug resistant (XDR) and XDR-TB in MDR-TB isolates but they are complex to perform and the geographical variation in mutation prevalence requires regional level sensitivity and specificity baseline assessment (46). Whole genome sequencing (WGS) provides an opportunity for genotypic drug susceptibility testing (DST), bacterial strain identification and the ability to differentiate relapse from re-infection thereby facilitating a better understanding of transmission dynamics which can inform improved contact tracing (51). Although these new technologies have numerous advantages, resource constraints make their extension to many jurisdictions prohibitive and there is a need to address financial constraints and implementation gaps (52).
In combating active disease, technological capability is only one part of the jigsaw and timely diagnosis is vital to interrupting transmission and optimizing treatment outcomes (53). There are an estimated three to four million active TB cases per year that are undiagnosed, with 75% of these cases occurring in 13 countries (54). To identify missing cases, the WHO recommend active case finding (ACF) in high risk populations (55). High risk populations include contacts of index cases and PLHIV, with other at-risk groups being situation specific depending upon the epidemiological, social and health system context (55). ACF interventions are context specific and are influenced by resource availability, physical geography and health system capability (56). For many low- and middle-income countries, resources limit the capacity to undertake ACF and diagnosis is dependent upon passive case finding (PCF) (57). For both ACF and PCF there are patient related (e.g., low TB literacy and education, cost- indirect and direct expenses, stigma and anxiety) and healthcare related (e.g., capacity, capability and resource) barriers to optimizing diagnosis (58). The barriers to patient health seeking behavior are complex and setting specific necessitating the need for local solutions (59). The interface between patient and healthcare barriers are also setting specific and often complicated by the mix of traditional, private, and public providers (59). Although global initiatives have been implemented [e.g., Global Laboratory Initiative (GLI)] to expand and accelerate access to quality assured laboratory services (60), provider specific delays (e.g., scarcity of healthcare professionals and infrastructure, low index of suspicion for TB and weak referral links) (61, 62) need addressing at a local level.
To be successful, effective diagnosis must be paired with correct and timely treatment and research is ongoing to develop safe, efficacious tolerable drug regimens capable of eradicating the disease and preventing long-term sequelae associated with the disease or resulting as an adverse effect of treatment (37). For both drug-susceptible TB (DS-TB) and drug-resistant TB (DR-TB), significant advances have been made in optimizing and re-purposing, identifying new compounds, and shortening treatment durations without adverse effect upon efficacy (63). In addition to efficacy, is the need to improve patient adherence and in parallel with shortened regimen durations, alternate routes of drug delivery are being evaluated (37). At August 2023 there were 28 TB drugs in the clinical trial pipeline, of which 18 are new chemical entities, seven are repurposed, two drugs (bedaquiline and delamanid) have received accelerated regulatory approval and one drug-pretomanid has received approval by the United States (US) Food and Drug Administration (64). Following these drug development advances, the WHO has endorsed new TB treatment regimen recommendations. In addition to the existing recommended regimen of 6 months of rifampicin, a new shorter 4-month course of isoniazid, rifapentine, moxifloxacin and pyrazinamide has been endorsed for DS-TB (65). Ethionamide has also been included in the essential medicine list for the treatment of DS-TB (66). Although the new regime has the advantage of a shorter treatment duration, it has the disadvantage of an increased pill burden and a substantially higher cost, due primarily to the inclusion of rifapentine (65). It is hoped that further developments will lead to reduced costs and a fixed dose combination tablet (65). For patients with MDR/rifampicin resistant (RR) TB a new all oral, shorter 6 month regimen of bedaquiline (B), pretomanid (Pa), linezolid (L) and moxifloxacin (M) collectively referred to as BPaLM has been endorsed (67). For patients with pre-XDR-TB the regimen can be applied without moxifloxacin (BPaL) (67). Although these new regimens offer benefit, they are complex and require clinical management especially in patients with comorbidities (68). New treatment regimens will require long-term appraisal for safety, tolerability, efficacy, and disease relapse rates, plus ongoing evaluation when administered with concurrent antiretroviral therapy (ART) (24). For these new regimens, further work is also required to improve genotypic-phenotypic concordance limits to facilitate the development of molecular DST (24).
Although the WHO have endorsed a number of new treatment regimens (66), certain population groups (e.g., children, PLHIV with a CD4 count < 100 cells/mm3, pregnant, breastfeeding and post-partum women) are excluded due to a lack of safety data, necessitating the need for further research (65, 68). Another limitation of the new recommendations is the lack of consideration for disease severity which can translate into undertreatment for patients with severe disease, and unnecessary treatment with potential toxic side effects for patients with a lower disease burden (69). Research is ongoing to identify methods to predict treatment response and the likelihood of relapse, so drug combinations and durations can be tailored with greater specificity for each patient (69).
The duration, complexity and side effects of treatment create a challenge to adherence. Poor treatment adherence leads to prolonged infectiousness, poor treatment outcomes and the development of drug resistance (70). Interventions that have been reported to be effective at increasing adherence include direct observation therapy (DOT), text reminders and education, drug box reminders, counseling and voucher interventions (71). Although interventions like DOT have been widely adopted, there is controversy regarding its reported effectiveness (70), which highlights the complex and dynamic nature of treatment taking behavior which in itself may change over time (72). Community involvement throughout the TB care cascade has been shown to be valuable in addressing communication and implementation gaps that often relate to complex cultural, social and economic interrelations (73).
Antimicrobial resistance (AMR) is one of the greatest threats to public health and development and one that will further exacerbate inequalities (74). Modeling shows AMR will result in an annual global economic shortfall of $1–$3.3 trillion by 2050 (75). DR-TB accounts for a disproportionately large amount of the global AMR burden and if left unchecked threatens to reduce the world economy by 0.63 per cent annually (76, 77). The extent of TB drug resistance- from mono (primarily isoniazid and rifampicin)- to extensive (isoniazid and rifampicin, a fluoroquinolone, and a second-line injectable or bedaquiline or linezolid) has been increasing over time (78, 79). The escalation in resistance brings an escalation in the challenges to achieving cure. Combating DR-TB requires more protracted treatment with increasingly complex, toxic, and costly regimens (80). In 2020 the direct cost of treating DS-TB in the U.S. was estimated to average $20,000/patient, a figure that escalates to $568,000 for extensively resistant TB, estimates that would be significantly higher if the indirect costs borne by patients were also considered (78).
DR-TB can arise through intra-patient evolution of resistance (acquired resistance) or through the direct transmission of genetically resistant bacteria (transmitted resistance) (76). Acquired resistance develops due to inadequate treatment and can be further amplified by the repeated use of short-course chemotherapy (81). A “One Health” approach to tackling drug resistance acknowledges the complexities of the issue and also provides an opportunity to consider the reservoir of infection and consequence of TB infection in other host species (82). Advances in molecular and phenotypic methods have revolutionized the diagnosis of drug resistance, but access to DST is still poor in many parts of the world (81). Universal DST is a key component of the End TB Strategy (18), and is fundamental to halting escalating resistance and retaining drug efficacy (83). To implement DST, policies within and between countries will need aligning and funding restraints and healthcare limitations will need addressing (84). To limit DR-TB transmission, active case finding, rapid diagnosis (including DST) and prompt effective treatment are key (76). Active case finding can employ a range of strategies from education campaigns and community mobilization through to systematic screening depending on resource availability (56). The WHO have recently recommended novel DR-TB regimes which have shorter treatment durations, lower toxicity and improved efficacy and should therefore improve treatment adherence and as a result the development of further resistance and continued transmission (68). Although these new treatment regimens offer significant benefits for patients and cost savings for their respective governments (85), more needs to be done to ensure pharmaceutical companies facilitate generic competition equitably and that they do not continue to price gouge (86).
Although a number of species within the MTBC have been demonstrated to cause zoonotic TB (zTB) including M. canetti, M. bovis, M. caprae, M. microti, M. pinnipedii, M. mungi, and M. orygis, current zTB estimates are solely based on M. bovis (87). In addition to the exclusion of many species, zTB estimates are challenged by the inability to clinically differentiate between M. bovis and M. tuberculosis infection and a lack of surveillance data in both human and animal populations, especially in countries where bovine TB is endemic and laboratory capacity is limited (88). Although the true burden of zTB is unknown, an estimated 140,000 cases (range 69,800–235,000) occurred globally in 2019 (89). The detrimental impact of infection goes beyond human health, with impacts on other species, livelihoods, and the conservation of wildlife (88). In addition to the large reservoir of infection across host species, is the challenge of reverse zoonoses with the concern that this includes the bi-directional transmission risk of DR-TB (87). M. bovis is naturally resistant to pyrazinamide and some isolates have also been reported as resistant to rifampicin and isoniazid (88).
The Stop TB Partnerships Global Plan to End TB 2016–2020 now identifies people in contact with livestock as a key at TB risk population and a “One Health” approach is being advocated which recognizes the interdependence of the health of people, livestock and the environment (87, 88). Recommendations to address zTB include expansion of appropriate diagnostic tools and capacity to identify zTB in people; development of appropriate sample collection methods (as many cases of zTB are extrapulmonary); expanded coverage of DST; improved diagnosis, surveillance, control and elimination programs in livestock and the consideration of wildlife as a reservoir of infection; improved food safety standards; improved surveillance and reporting; improved intersectoral and collaborative approaches to developing and implementing policy (88). Implementing these recommendation will require political will and adequate funding (88).
Vaccines have the potential to significantly reduce TB incidence, mortality, and societal costs whilst also mitigating AMR (90). At present however there is only one vaccine- Bacillus Calmette–Guérin (BCG)-that is licensed to combat TB (91). BGC was deployed in 1921 and despite its administration to more people in the world than any other vaccine, controversy exists regarding its efficacy (91–94). The vaccine provides protection against tuberculous meningitis and miliary TB in infants and young children and is usually administered as part of the routine newborn immunization schedule (95–97). In adults, the effectiveness of the vaccine against both the acquisition of infection and the development of active pulmonary disease, varies widely across studies (92, 96, 98, 99).
In the quest for a BCG replacement, three vaccination strategies have been proposed: one that prevents primary infection and disease progression following exposure; one that prevents the development of active disease from LTBI and an immunotherapeutic adjunct to accompany TB treatment in patients with active disease (100). To guide vaccine development the WHO has documented the preferred product characteristics for therapeutic and prophylactic vaccines (101, 102), and as of August 2023, there were 16 vaccine candidates in the clinical development pipeline (64). There are currently four novel vaccine candidates in phase III trials (64), including GamTBvac a recombinant subunit BGC booster vaccine aimed at preventing pulmonary TB in HIV negative adults aged 18–45 years (103). Mycobacterium indicus pranii (Mycobacterium w) (MIP) vaccine (also known as Immuvac), an immunotherapeutic and immunoprophylactic agent approved to treat leprosy, is also being evaluated for its utility in the treatment of TB (104). MTBVAC is the only vaccine candidate in phase III trials which is based upon an attenuated human clinical isolate of M. tuberculosis (105). MTBVAC aims to replace BCG as a preventative vaccine for newborns and also serve as a booster for adults and adolescents (106). The fourth phase III candidate is VPM1002, a recombinant BCG (rBCG) vaccine that is being evaluated for its efficacy in preventing infection in newborns and the reoccurrence of disease in individuals who have been successfully treated (107–109).
Vaccine efficacy is impacted by the extreme complexities that occur across the interactions between MTB and its host (109). Pathogen virulence and host immunity, and the interactions between these impact infection prevalence, disease progression and TB outcome (109). Although there have been significant advances in molecular biology and bioinformatics (110, 111), one of the greatest challenges is the lack of biomarkers which signal the prospective risk of developing disease or the correlates of protection (90). TB vaccine development is also limited by the lack of a suitable animal evaluation model (106). Findings from “successful” animal studies do not always extrapolate, as evidenced by the vaccine candidates that are terminated once they enter human trials (112). It is hoped that novel humanized transgenic animal models will play a future role in pre-clinical evaluations and that new technologies will facilitate new opportunities within existing models (112). Another challenge is the lack of emulated exposure variables- those employed in animal models (typically high dose, single exposure, single strain) differ from those experienced by the human host in natural conditions (typically low dose, multiple exposure, multiple strains) (106, 113). The discord between animal models, and clinical trial endpoint criteria, adds another complexity to candidate vaccine evaluation (106). In preclinical trials, efficacy is assessed in terms of improved bacterial counts, reduced histopathological damage and increased longevity, whereas TB incidence is used to determine vaccine effectiveness in clinical trials (106).
Vaccine development is also challenged by the complex and diverse heterogeneities of TB epidemiology. In addition to pathogen genetic diversity, and the heterogeneity of host susceptibility to infection, is the large variation in confounding variables between and within populations e.g., nutritional status, socio-environment determinants, co-infection/co-morbidities etc. (114). The infection background of a population is also heterogenous, and complicated by BCG vaccination and nontuberculous mycobacteria (NTM) infections (106).
Despite these challenges, the delivery of an effective vaccine for SARS-CoV-2 within 12 months of its discovery, demonstrates what can be achieved when there is collective political will and sufficient funding (115). Whilst genomic and pathogenicity differences between MTB and SARS-CoV-2 are acknowledged, would a vaccine solution be fast tracked if TB posed an imminent threat to the developed world? (116). Despite modeling studies showing novel TB vaccines to be impactful and cost effective in low- and middle-income countries, and investment cases demonstrating clear and significant returns on investment, there is a lack of political and funding momentum (90, 116, 117). To address this lack of impetus, the WHO has established a TB Vaccine Accelerator Council which aims to replicate the successes achieved in the SARS-CoV2 vaccination program whilst also acknowledging lessons learnt and the need to ensure vaccine equity (91, 118).
Social determinants of health (SDH) are the non-medical factors that influence health outcomes and include social and economic policy, social norms, development agendas, political systems and climate change (119). SDH are estimated to account for 30%−55% of health outcomes which highlights the need to employ a holistic approach to disease eradication (120, 121). SDH are drivers of health inequity and must to be addressed if global targets for TB eradication are to be achieved (16, 121). Communicable disease programs have made significant progress when SDH are addressed, as has been demonstrated for HIV/AIDS (120).
Social determinants are of significance across all stages of TB pathogenesis and impact exposure to infection, disease transmission and progression, timely and accurate diagnosis, treatment adherence and treatment outcomes (120). The population distribution of TB mirrors the distribution of these social determinants and highlights the inequities between and within societies (120, 122). For some populations e.g., migrants and refugees, the social determinants of TB are compounded, thereby exacerbating their vulnerability to disease and impacting their ability to access appropriate treatment (123, 124).
For TB, poverty is often the underlying cause of numerous risk factors including malnutrition, poor living and environmental conditions and is associated with poor health knowledge, limited access to healthcare, and a lack of empowerment (125). The lack of education and empowerment then leads to further exposure to additional risk factors such as HIV, drug, and alcohol abuse (125). It can be argued that poverty is a policy decision and that inequities are effectively established by the government of the day at levels they are happy to contend with (126, 127).
Addressing the complexities of poverty will require international commitment to short and long-term solutions (128). Although food assistance is critical to preventing malnutrition, it is only an interim solution and long-term investments in sustainable agriculture are required (129). Social protection and microfinance initiatives, offer an opportunity to provide short-term assistance whilst providing an opportunity for long-term human capital development (120). Universal health coverage (UHC) is considered a key component to achieving the End TB Strategy and is defined as universal access to healthcare without incurring financial hardship (130, 131). Although this is a recommended component of the End TB Strategy and improvements in UHC have been shown to be effective in improving other health outcomes e.g. child mortality (132), further research is required to understand its causal effect on TB morbidity and mortality (133). Over the last decade there have been improvements in health service coverage globally, but at the same time there has been an increasing trend in the number of people facing catastrophic expenditure on health (134). Barriers to accessing TB healthcare are not however solely economic, and geographic, social, cultural and health system barriers must also be overcome (135). Socio-cultural barriers include inadequate knowledge about TB, belief systems and traditional medicine, stigma, and cultural norms that result in gender based imbalances in accessing care (136). Education and the cultural competence of health care providers are key to addressing these socio-cultural barriers (137, 138). Health system barriers often reflect organizational capacity constraints in terms of infrastructure, resources, co-ordination, and workforce limitations, the latter of which is also impacted by stigma (139–141). However, an overarching barrier to all aspects of healthcare delivery is corruption, to which $560 billion per year is estimated to be lost (142, 143). Education, in conjunction with policies that reduce supply and demand, will play an important role in addressing behavioral TB risk factors such as drug and alcohol abuse (144). Implementing control policies in developing countries can however create an economic conflict, due to the significant value of for example tobacco exports (144). A possible solution to overcoming this conflict is to integrate tobacco cessation interventions into TB control programs (145). Vertically integrated TB control programs also provide an opportunity to address the latent synergies of HIV and diabetes (146).
The inequities that impact TB have been further exacerbated by the recent COVID-19 pandemic, conflict, and climate change (129, 147). There are many parallels between TB and COVID-19, the influence of misinformation and the failure of the world's major powers to collaborate resulted in a staggering death toll (148). Despite these failings, there are opportunities for learnings and the COVID-19 vaccine development response shows what can be achieved when there is political will and adequate resource allocation (149). Many of the technological advances and behavioral changes that were successful in tackling COVID-19, are of translatable value to combating TB e.g., technological developments in contact tracing, risk assessment and improved interconnected real time data, portable diagnostics, improved supply chain management, effective public health communication, and the application of non-pharmaceutical interventions (149–151). Increased resourcefulness is also required when tackling TB during conflict, which compounds the determinants of TB and the risk of drug resistance, whilst amplifying social inequities and vulnerabilities (152, 153). Conflict leads to the diversion of economic resources, food insecurity, patient delays in seeking care, healthcare disruption, and displacement (152, 154). During conflict, strong local leadership and community engagement are required as many stakeholders cannot actively work in the field due to security and logistical reasons (155, 156). Other innovations that have been implemented to address the additional complexities of conflict include self-administered therapy (SAT), emergency drug packs, use of technology to maintain communication and diagnostic investments (157–159). For innovations that are applied to mitigate climate change they must ensure that they do not exacerbate the inequities that climate change already creates (160). It is the populations who have contributed least to climate change that are the most vulnerable to its impact, and mitigation strategies that impact food and energy prices also have their greatest impact on these populations (160). Research shows climate change to be positively associated with a number of TB risk factors including poverty, undernutrition, HIV, diabetes and overcrowding (161). In addition to affecting environmental factors, climate change has been shown to impact TB epidemiology through its impact on both the host and the pathogen (162). Increasing temperatures are associated with increased bacterial growth and the collateral development of antibiotic resistance, and changes in ultra-violet radiation are associated with Vit D metabolism and BCG vaccine efficacy (162, 163).
In addition to the SDH, are a number of health and demographic factors that also need to be taken into consideration within the context of the TB pandemic. HIV infection is the greatest risk factor for LTBI progressing to active disease (15–22 times more likely) and TB is the major cause of HIV-related deaths (164, 165). Many of the SDH that increase vulnerability to TB, also increase vulnerability to HIV/AIDS, pandemic vulnerabilities that are amplified by COVID-19 and conflict (166–168). The COVID-19 pandemic highlighted the need to reorientate primary healthcare provision and the WHO now recommends an integrated rather than vertical approach (169), which has been shown to improve TB treatment outcomes and expediate ART initiation (170). Like HIV, diabetes has a synergistic role with TB, people with diabetes are at increased risk of developing TB and experiencing poor treatment outcomes, and TB and TB medications can impair glucose tolerance (171, 172). Diabetes is a growing health concern, estimated to impact 6.1% of the global population in 2021, and projected to affect 9.8% by 2050 (173). The disease has its greatest impact in low- and middle-income countries, which were estimated to carry 80% of the global burden in 2019 (174). The need to integrate TB and diabetes care is gaining increasing recognition (175, 176), and where co-management has been implemented, improved patient outcomes are being achieved (177). The most common form of diabetes is type-2 and like TB, its prevalence increases with age (178, 179). Many aspects of aging present challenges to TB control and these will become of increasing importance as the global demographic ages. By 2030, it is estimated that one in six people will be over 60 years of age and that by 2050, the population in this age group will have doubled (180). Aging is associated with immunosenescence, malnutrition, comorbidities, cognitive disorders, functional dependence, drug-drug interactions for those on polymedication and the possibility of poorer tolerance of TB drugs (179). Although multidisciplinary management is advocated for in geriatric health facilities (179, 181), these concepts are unlikely to be applicable in low-income settings where the elderly are cared for by family. Concepts, policies, and frameworks are also lacking to address post-TB sequelae across all patient populations (182). There is increasing evidence to show that TB has frequent and substantial morbidity consequences post microbiological cure (183), and there is a need to consider these sequelae in TB healthcare provision. Although some interventions have been shown to be effective at reducing the burden of post-TB sequelae, further research is urgently required as a significant population is impacted, with the number of TB survivors estimated to be alive in 2020 being more than 10 times the estimated annual disease incidence (182, 184, 185).
Addressing the TB epidemic will require, sustained, long-term, funding. The Global Plan to End TB, 2023–2030 (Global Plan) estimates that US$249.98 billion will be required over this time frame if TB is to be eradicated as a public health challenge by 2030 (186). The significantly higher funding needed in the current Global Plan, relative to the previous plan, is attributed to catching up on historic underfunding, mitigating the consequences of COVID-19 and fast tracking the development of new tools including the development of at least one new vaccine (186). However, significant under-funding continues to be a major barrier with political commitments not being upheld (187, 188). The World's response to the COVID-19 pandemic however, shows what can be achieved when there is significant political will and adequate resource allocation (186). The financing disparity between COVID-19 and TB reflects the global inequity in our approach to healthcare, despite TB modeling showing a 40:1 return on investment and the potential loss of US$1 trillion and 234 million DALYs between 2023–2030 if the status quo is maintained (186, 187).
The current levels of funding for TB research and development (R&D) are insufficient, with $1.03 billion invested in 2022 vs. an annual target of $2 billion as pledged by World leaders at the U.N. high level meeting on TB in 2018 (189). Over half of the 2022 TB R&D funding originated from two organizations- the United States National Institutes of Health and the Bill & Melinda Gates Foundation (189). In 2021, 79% of funding for TB prevention, diagnosis and treatment was derived from domestic sources with the split of funding (domestic vs. international) shown to be highly variable between countries (190). The Global Fund to fight HIV, TB, and malaria is a major source of funding for TB, with its contribution equating to 76% of international donations in 2021 (190). Proposed strategies to combat TB form the basis of funding applications to the Global Fund, which enables each country to tailor their response in consideration of their cultural, political, and epidemiological context (191).
Although patents are necessary to incentivize innovation and R&D investment, they can be exploited for financial gain to the detriment of the people the innovation is intended to serve (192). A pertinent example is Johnson and Johnson (J&J) profiteering from bedaquiline, and Otsuka profiteering from delamanid, with treatment regimens estimated to cost 13–18 times and three times more than the cost of production plus profit, respectively (193, 194). Both pharmaceutical companies are also exploiting their monopoly positions by enforcing secondary patents and utilizing opaque restrictive licenses that undermine competition (193, 194).
Similar corporate extortion, to the detriment of those most in need, occurs with TB diagnostic tools (195). Although Cepheid has made marginal reductions to the cost of its GeneXpert technology (196), prices remain at more than triple to cost of production and petitions continue to seek further price reductions (195, 197). The extent of corporate greed is exacerbated by the fact that significant public funding has supported the development of these technologies; Cepheid received $250 million to develop GeneXpert (195), and direct and indirect public contributions to the development of bedequiline are estimated at $455–747 million (198).
Artificial Intelligence (AI) is the simulation of human cognitive function using computer systems (199). AI techniques include machine learning that employs multiple algorithms to identify complex nonlinear relationships within large datasets, deep learning where multiple layers of processing are used to progressively extract higher level features, and generative AI that can create new content (199, 200). AI has revolutionized healthcare and can be applied to improve disease screening and diagnosis, optimize treatment regimens and advance precision medicine, support drug and vaccine development, optimize health systems management, support disease surveillance and refine disease risk predictions, assist clinical care and improve patient education (201, 202). AI tools can also improve accuracy and efficiency while reducing costs and providing a solution to the universal shortage of healthcare professionals (201, 203).
Although AI presents many opportunities, ethical, privacy, data security, algorithm biases, and legal considerations present complex challenges and further clinical trials are required to verify the relevance of AI models (201, 204). AI algorithm development is reliant upon large well annotated datasets which is challenged by the complexities of accessing sensitive personal data (205). The challenges of accessing sensitive personal data include the technological implications of data protection and definitions of confidentiality and other core tenets of medical ethics (206). AI algorithms may reflect human biases in decision making and the biases that are inherent in current healthcare delivery (206). The legal complexities regarding the application of this technology include the lack of universal definitions and guidelines for its use, and overlapping theories of liability across multiple stakeholders (207, 208). It is important to ensure that the business models used to develop, deploy and support these technologies do not embody typical political power relations that reproduce past inequities (209).
Despite these complexities, AI is providing a transformative role in TB control. In 2022, the WHO launched an operational research package to generate data on treatment decision algorithms for pulmonary TB in children (210). This operational research seeks to provide external validation of two decision algorithms designed to help clinicians determine whether to commence treatment (210). Computer-aided detection (CAD) software is being utilized to automate the interpretation of digital X-ray images and is now recommended as an alternative to human interpretation in WHO screening guidelines (211). WHO recommend the use of CAD for screening and triage when interpreting plain X-ray s from patients ≥15 years old (211). CAD has the potential to improve access to screening as it can operate in both portable and ultra-portable X-ray machines, but it has the disadvantage of requiring calibration with local data due to heterogeneity in the accuracy of threshold scores and can only be applied to frontal X-ray images for adults (212). AI provides an opportunity to increase the efficacy of resources which in many high TB burden countries are constrained. The application of this technology to patients on directly observed therapy (DOT) has been successful in reducing the time and cost burden on both patients and healthcare workers (213, 214). The application of AI to monitor treatment efficacy and predict prognosis is being explored (215). AI is successfully predicting treatment duration, adverse reactions, drug resistance, and treatment outcomes which paves the way for personalized care and improved treatment outcomes (204, 216).
Advances in geographic information systems (GIS) have played a pivotal role in analyzing and visualizing complex health data to inform evidence-based decision making (217). GIS applications provide an opportunity to evaluate the intricacies between disease and environmental, socio-economic and demographic factors (218). Although there are challenges (e.g., data quality, data privacy, and resource constraints) emerging technologies and improved spatial resolution hold promise for advances in this field (218).
Digital health technologies have a number of innovative approaches that the WHO recommend in support of the End TB Strategy (219). Technologies to improve patient engagement and treatment adherence include telemedicine, electronic medication monitors, video supported treatment and mobile phone messaging (219). Electronic data systems are of increasing importance for patient care, TB program management and surveillance systems (219). Online technologies are an effective education tool and have potential to support mental health interventions (220, 221).
Drones have been used to provide cost-effective bi-directional transport of sputum samples and TB medications between remote communities and diagnostic/treatment facilities (222). Drones provide an opportunity to support TB control programs in resource limited settings that often have limited laboratory and transportation infrastructure, limited healthcare system coverage, a paucity of healthcare professionals and the challenge remoteness and vulnerability to natural disasters (222, 223).
The use of renewable energy as facilitated access to modern medical technologies for populations without access to electricity. The installation of solar photovoltaic systems within health systems as part of the United Nations Development Programme (UNDP) Solar for Health is proving to be fundamental to the success of many TB programs (224–226). Advances in battery technology have also made significant contributions to the feasibility of utilizing renewable energy solutions in healthcare (227). Battery solutions in conjunction with the development of mobile technologies e.g. portable X-rays, enable remote communities to access TB healthcare (228, 229).
Nano medicine has a number of potential applications including mycobacterial strain identification and efficient medication delivery (230). Nanoparticles are being evaluated for their ability to diagnose both latent and active infection and discriminate specific mutations associated with antibiotic resistance (230, 231). Nanomedicine has the potential to deliver targeted, long-lasting antibiotics within the therapeutic range, thereby avoiding the toxicity and non-compliance issues arising from conventional therapy (232).
An alternative approach to conventional therapies is Host-Directed Therapy (HDT), which uses agents to modulate host immunity and enhance the efficacy of anti-tubercular drugs (233). Potential HDT agents include antimicrobial peptides, micronutrients, and re-purposed drugs but further clinical trials are required to evaluate their safety and efficacy alone and in combination with anti-TB drugs (233).
The increasing health security threat of antibiotic resistance, and advances in molecular biology, have led to a resurgent interest in the therapeutic use of bacteriophages (phages) (234). Phages are viruses that infect bacteria and kill their host cells in the lytic replication phase of their lifecycle (235, 236). Phages are host specific, with the specificity of some limited to strains within a bacteria species (237).
Mycobacteriophages are phages of the genus Mycobacterium and were first isolated in 1954 (236). Although phages are ubiquitous and considered to be one of the most abundant biological agents on earth, the slow growth rate of MTB creates technical challenges to their isolation (237, 238), and to date the efficacy of phages against MTB infection in humans has not been evaluated (239), Conversely, the fast-growing non-pathogenic mycobacterium M. smegmatis, has provided an ideal host platform from which >10,000 phages have been isolated and of these >2,000 have been sequenced (238). Despite the significant number of isolates, there is very limited data on their potential role in the treatment of infection (240), and phages have only been used on compassionate grounds for patients with highly antibiotic-refractory NTM infections (235). While these NTM cases were limited in number, and outcomes confounded by complex clinical conditions, it is hoped that they can inform future trails (238).
Future trials will need to evaluate phage efficacy, further elucidate their strengths and limitations and determine optimal doses, methods of administration, potential antibiotic interactions and the impact of antibiotic resistant hosts (235, 239). Phages have the advantage of high host specificity, the capacity to replicate and although data is limited, reported safety profiles are high and adverse reactions rare (235). High host specificity can however be a double edged sword, by limiting the development of generalized treatments and necessitating the need for patient specific treatments (235). Phages also have the disadvantage of being potential targets of the human immune system, and their large size relative to antimicrobial drugs, can impact how and where they can travel in the body (235, 237). Although phages can access extracellular bacteria, their ability to infect intracellular bacteria or bacteria within granulomas may be limited (235). In mice, phage aerosols have been shown to reduce MTB burdens prior to macrophage uptake and granuloma formation, leading to the suggestion that they may also be utilized as a prophylactic agent to minimize transmission in high-risk settings (241). Although phages have mechanisms to evade bacteria host defense mechanisms and selection pressure favors their fitness, the same selection pressures apply to the host cells, making phage resistance a future consideration (238). Combined phage-antibiotic therapies present a synergistic challenge to the bacteria host cell that maybe beneficial to combating infection (242). To counter the development of phage resistance when used as the sole therapeutic agent, phage cocktails or sequential treatments have been proposed (242).
Although TB continues to elude eradication, many new technologies and applied applications offer exciting prospects in working toward the End TB Strategy targets. Although these new technologies offer promise, one of the greatest challenges will be ensuring that those in need have equitable access. Addressing underlying inequities, which also result in the social determinants of the disease, will be one of the biggest challenges to overcoming the TB pandemic.
BG: Writing – original draft, Writing – review & editing. KA: Writing – review & editing.
The author(s) declare that no financial support was received for the research, authorship, and/or publication of this article.
The authors declare that the research was conducted in the absence of any commercial or financial relationships that could be construed as a potential conflict of interest.
All claims expressed in this article are solely those of the authors and do not necessarily represent those of their affiliated organizations, or those of the publisher, the editors and the reviewers. Any product that may be evaluated in this article, or claim that may be made by its manufacturer, is not guaranteed or endorsed by the publisher.
1. Barberis I, Bragazzi NL, Galluzzo L, Martini M. The history of tuberculosis: from the first historical records to the isolation of Koch's bacillus. J Prev Med Hyg. (2017) 58:E9.
3. World Health Organization. Global health estimates: Leading causes of DALY. Available at: https://www.who.int/data/gho/data/themes/mortality-and-global-health-estimates/global-health-estimates-leading-causes-of-dalys (accessed May 20, 2024).
4. Tomeny EM, Nightingale R, Chinoko B, Nikolaidis GF, Madan JJ, Worrall E, et al. TB morbidity estimates overlook the contribution of post-TB disability: evidence from urban Malawi. BMJ Global Health. (2022) 7:e007643. doi: 10.1136/bmjgh-2021-007643
5. Chandra P, Grigsby SJ, Philips JA. Immune evasion and provocation by Mycobacterium tuberculosis. Nat Rev Microbiol. (2022) 20:750–66. doi: 10.1038/s41579-022-00763-4
6. World Health Organization. Tuberculosis Key Facts. (2023). Available at: https://www.who.int/news-room/fact-sheets/detail/tuberculosis#:~:text=Over%2080%25%20of%20cases%20and,the%20Western%20Pacific%20(18%25) (accessed May 20, 2024).
7. Zhang H, Liu M, Fan W, Sun S, Fan X. The impact of Mycobacterium tuberculosis complex in the environment on one health approach. Front Public Health. (2022) 10:994745. doi: 10.3389/fpubh.2022.994745
8. Thwaites G, Caws M, Chau TTH, D'Sa A, Lan NTN, Huyen MNT, et al. Relationship between Mycobacterium tuberculosis genotype and the clinical phenotype of pulmonary and meningeal tuberculosis. J Clin Microbiol. (2008) 46:1363–8. doi: 10.1128/JCM.02180-07
9. Gehre F, Kumar S, Kendall L, Ejo M, Secka O, Ofori-Anyinam B, et al. A mycobacterial perspective on tuberculosis in West Africa: significant geographical variation of M. africanum and other M tuberculosis complex lineages. PLoS Negl Trop Dis. (2016) 10:e0004408. doi: 10.1371/journal.pntd.0004408
10. Scriba TJ, Dinkele R, Warner DF, Mizrahi V. Challenges in TB research. J Exp Med. (2022) 219:e20221334. doi: 10.1084/jem.20221334
11. Chai Q, Zhang Y, Liu CH. Mycobacterium tuberculosis: an adaptable pathogen associated with multiple human diseases. Front Cell Infect Microbiol. (2018) 8:158. doi: 10.3389/fcimb.2018.00158
12. Wong EB. It is Time to Focus on Asymptomatic Tuberculosis. Oxford: Oxford University Press (2021), p. e1044–6. doi: 10.1093/cid/ciaa1827
13. Allué-Guardia A, García JI, Torrelles JB. Evolution of drug-resistant Mycobacterium tuberculosis strains and their adaptation to the human lung environment. Front Microbiol. (2021) 12:612675. doi: 10.3389/fmicb.2021.612675
14. Garcia-Vilanova A, Chan J, Torrelles JB. Underestimated manipulative roles of Mycobacterium tuberculosis cell envelope glycolipids during infection. Front Immunol. (2019) 10:494578. doi: 10.3389/fimmu.2019.02909
15. Nimmo C, Millard J, Faulkner V, Monteserin J, Pugh H, Johnson EO. Evolution of Mycobacterium tuberculosis drug resistance in the genomic era. Front Cell Infect Microbiol. (2022) 12:954074. doi: 10.3389/fcimb.2022.954074
16. World Health Organization. TB determinants. (2022). Available at: https://www.who.int/teams/global-tuberculosis-programme/tb-reports/global-tuberculosis-report-2022/uhc-tb-determinants/6-3-tb-determinants (accessed April 16, 2024).
17. United Nations. Transforming our world: the 2030 Agenda for Sustainable Development. New York, NY: United Nations (2015).
19. United Nations. The Sustainable Development Goals Report 2023 Special Edition. New York, NY: United Nations (2023).
20. World Health Organization. Status Update: Reaching the Targets in the Political Declaration of the United Nations General Assembly High-Level Meeting on the Fight against Tuberculosis. Geneva: WHO (2023).
21. Silva S, Arinaminpathy N, Atun R, Goosby E, Reid M. Economic impact of tuberculosis mortality in 120 countries and the cost of not achieving the Sustainable Development Goals tuberculosis targets: a full-income analysis. Lancet Glob Health. (2021) 9:e1372–e9. doi: 10.1016/S2214-109X(21)00299-0
22. The World Health Organization. Implementing the end TB strategy: the essentials, 2022 update. Geneva: WHO (2022).
23. Thorpe J, Sawaengdee W, Ward D, Campos M, Wichukchinda N, Chaiyasirinroje B, et al. Multi-platform whole genome sequencing for tuberculosis clinical and surveillance applications. Sci Rep. (2024) 14:5201. doi: 10.1038/s41598-024-55865-1
24. Naidoo K, Perumal R. Advances in tuberculosis control during the past decade. Lancet Respir Medi. (2023) 11:311–3. doi: 10.1016/S2213-2600(23)00090-5
25. Zellweger J-P, Sotgiu G, Corradi M, Durando P. The diagnosis of latent tuberculosis infection (LTBI): currently available tests, future developments, and perspectives to eliminate tuberculosis (TB). Med Lav. (2020) 111:170. doi: 10.23749/mdl.v111i3.9983
26. World Health Organization. WHO Operational Handbook on Tuberculosis. Module 1: Prevention - Tuberculosis Preventive Treatment, 2nd Edn. Geneva: WHO (2024).
27. Cohen A, Mathiasen VD, Schön T, Wejse C. The global prevalence of latent tuberculosis: a systematic review and meta-analysis. Eur Respir J. (2019) 54. doi: 10.1183/13993003.00655-2019
28. Comstock GW, Livesay VT, Woolpert SF. The prognosis of a positive tuberculin reaction in childhood and adolescence. Am J Epidemiol. (1974) 99:131–8. doi: 10.1093/oxfordjournals.aje.a121593
29. Warsinske H, Vashisht R, Khatri P. Host-response-based gene signatures for tuberculosis diagnosis: A systematic comparison of 16 signatures. PLoS Med. (2019) 16:e1002786. doi: 10.1371/journal.pmed.1002786
30. Esmail H, Cobelens F, Goletti D. Transcriptional biomarkers for predicting development of tuberculosis: progress and clinical considerations. Eur Respir J. (2020) 55. doi: 10.1183/13993003.01957-2019
31. World Health Organization. Latent Tuberculosis Infection: Updated and Consolidated Guidelines for Programmatic Management. Geneva: World Health Organization (2018).
32. Dobler CC, Batbayar O, Wright CM. Practical challenges and solutions to TB control in a lower–middle-income country: experiences from Mongolia. Eur Respir Soc. (2018) 180–3. doi: 10.1183/20734735.020218
33. Chin KL, Anibarro L, Sarmiento ME, Acosta A. Challenges and the way forward in diagnosis and treatment of tuberculosis infection. Trop Med Infect Dis. (2023) 8:89. doi: 10.3390/tropicalmed8020089
34. World Health Organization. WHO Operational Handbook on Tuberculosis. Module 3: Diagnosis Tests for Tuberculosis Infection. Geneva: WHO (2022).
35. World Health Organization. Diagnostic Testing. (2023). Available at: https://www.who.int/teams/global-tuberculosis-programme/tb-reports/global-tuberculosis-report-2023/tb-diagnosis—treatment/2.2-diagnostic-testing-for-tb
36. Centers for Disease Control and Prevention. Diagnosis of Tuberculosis Disease Fact Sheet. Atlanta, GA: Centers for Disease Control and Prevention (2016).
37. Gill CM, Dolan L, Piggott LM, McLaughlin AM. New developments in tuberculosis diagnosis and treatment. Breathe. (2022) 18. doi: 10.1183/20734735.0149-2021
38. Shaozae CK, Das D, Kumar M. Diagnosis of tuberculosis in low-resource settings: overcoming challenges within laboratory practice. Eur Med J. (2023). doi: 10.33590/emj/10302558
39. Singhal R, Myneedu VP. Microscopy as a diagnostic tool in pulmonary tuberculosis. Int J Mycobacteriol. (2015) 4:1–6. doi: 10.1016/j.ijmyco.2014.12.006
40. Global Laboratory Initiative. Laboratory Diagnosis of Tuberculosis by Sputum Microscopy: The Handbook Global Edition (Adelaide, SA: Partnership ST, editor). (2013).
41. World Health Organization. Fluorescent Light-Emitting Diode (LED) Microscopy for Diagnosis of Tuberculosis: Policy Statement. Geneva: World Health Organization (2011).
42. Shuaib YA, Khalil EA, Schaible UE, Wieler LH, Bakheit MA, Mohamed-Noor SE, et al. Smear microscopy for diagnosis of pulmonary tuberculosis in Eastern Sudan. Tuberc Res Treat. (2018) 2018:8038137. doi: 10.1155/2018/8038137
43. World Health Organization. WHO Consolidated Guidelines on Tuberculosis: Rapid Diagnostics for Tuberculosis Detection. Module 3. Geneva: World Health Organization (2020).
44. World Health Organization. WHO Operational Handbook on Tuberculosis Module 3: Diagnosis. Rapid Diagnostics for Tuberculosis Detection, 3rd Edn. Geneva: World Health Organization (2024).
45. MacLean E, Kohli M, Weber SF, Suresh A, Schumacher SG, Denkinger CM, et al. Advances in molecular diagnosis of tuberculosis. J Clin Microbiol. (2020) 58. doi: 10.1128/JCM.01582-19
46. Oommen S, Banaji N. Laboratory diagnosis of tuberculosis: advances in technology and drug susceptibility testing. Indian J Med Microbiol. (2017) 35:323–31. doi: 10.4103/ijmm.IJMM_16_204
47. World Health Organization. Practical Manual of Processing Stool Samples for Diagnosis of Childhood TB. Geneva: World Health Organization (2022).
48. Hong JM, Lee H, Menon NV, Lim CT, Lee LP, Ong CW. Point-of-care diagnostic tests for tuberculosis disease. Sci Transl Med. (2022) 14:eabj4124. doi: 10.1126/scitranslmed.abj4124
49. World Health Organization. The Use of Lateral Flow Urine Lipoarabinomannan Assay (LF-LAM) for the Diagnosis and Screening of Active Tuberculosis in People Living with HIV: Policy Guidance. Geneva: World Health Organization (2015). Report No.: 9241509635.
50. Ruperez M, Shanaube K, Mureithi L, Wapamesa C, Burnett JM, Kosloff B, et al. Use of point-of-care C-reactive protein testing for screening of tuberculosis in the community in high-burden settings: a prospective, cross-sectional study in Zambia and South Africa. Lancet Glob Health. (2023) 11:e704–e14. doi: 10.1016/S2214-109X(23)00113-4
51. Donnan EJ, Marais BJ, Coulter C, Waring J, Bastian I, Williamson DA, et al. The use of whole genome sequencing for tuberculosis public health activities in Australia: a joint statement of the National Tuberculosis Advisory Committee and Communicable Diseases Genomics Network. Commun Dis Intell. (2023) 47:1–13. doi: 10.33321/cdi.2023.47.8
52. Pai M, Furin J. Tuberculosis innovations mean little if they cannot save lives. Elife. (2017) 6:e25956. doi: 10.7554/eLife.25956
53. Long R. Making a timely diagnosis of pulmonary tuberculosis. Can Respir J. (2015) 22:317–21. doi: 10.1155/2015/826035
54. Stop TB Partnership. The Missing TB Millions. (2019). Available at: https://www.stoptb.org/global-drug-resistant-tb-initiative/missing-tb-millions (accessed May 9, 2024).
55. World Health Organization. Systematic Screening for Active Tuberculosis: An Operational Guide. Geneva: WHO (2015).
56. Burke RM, Nliwasa M, Feasey HR, Chaisson LH, Golub JE, Naufal F, et al. Community-based active case-finding interventions for tuberculosis: a systematic review. Lancet Public Health. (2021) 6:e283–99. doi: 10.1016/S2468-2667(21)00033-5
57. Fenta MD, Ogundijo OA, Warsame AAA, Belay AG. Facilitators and barriers to tuberculosis active case findings in low-and middle-income countries: a systematic review of qualitative research. BMC Infect Dis. (2023) 23:515. doi: 10.1186/s12879-023-08502-7
58. Dixit K, Biermann O, Rai B, Aryal TP, Mishra G, de Siqueira-Filha NT, et al. Barriers and facilitators to accessing tuberculosis care in Nepal: a qualitative study to inform the design of a socioeconomic support intervention. BMJ Open. (2021) 11:e049900. doi: 10.1136/bmjopen-2021-049900
59. Lestari BW, McAllister S, Hadisoemarto PF, Afifah N, Jani ID, Murray M, et al. Patient pathways and delays to diagnosis and treatment of tuberculosis in an urban setting in Indonesia. Lancet Reg Health West Pac. (2020) 5:100059. doi: 10.1016/j.lanwpc.2020.100059
60. Stop TB Partnership. Welcome to the website of the Global Laboratory Initiative (GLI). Available at: https://stoptb.org/wg/gli/ (accessed May 17, 2024).
61. Feyisa JW, Lemu JC, Hunde MD, Berhanu RD, Jaleta DD, Abdisa DK, et al. Why health system diagnosis delay among tuberculosis patients in Illubabor, Oromia region, South West Ethiopia? A qualitative study. PLoS ONE. (2022) 17:e0278592. doi: 10.1371/journal.pone.0278592
62. World Health Organization. Diagnostic and treatment delay in tuberculosis. An in-depth analysis of the health-seeking behaviour of patients and health system response in seven countries of the Eastern Mediterranean Region. Geneva: WHO (2006).
63. Motta I, Boeree M, Chesov D, Dheda K, Günther G, Horsburgh Jr CR, et al. Recent advances in the treatment of tuberculosis. Clin Microbiol Infect. (2023). doi: 10.1016/j.cmi.2023.07.013
64. World Health Organization. TB Research and Innovation. (2023). Available at: https://www.who.int/teams/global-tuberculosis-programme/tb-reports/global-tuberculosis-report-2023/tb-research-and-innovation#:~:text=TB%20vaccines,of%20treatment%20for%20TB%20disease (accessed February 15, 2024).
65. World Health Organization. WHO consolidated guidelines on tuberculosis. Module 4: treatment-drug-susceptible tuberculosis treatment. Geneva: World Health Organization. (2022).
66. World Health Organization. What's new in the TB section of the 2023 WHO Model Lists of Essential Medicines. (2023). Available at: https://www.who.int/news/item/09-08-2023-what-s-new-in-the-tb-section-of-the-2023-who-model-lists-of-essential-medicines (accessed May 9, 2024).
67. World Health Organization. New treatment for drug-resistant tuberculosis. (2023). Available at: https://www.who.int/teams/global-tuberculosis-programme/tb-reports/global-tuberculosis-report-2023/featured-topics/new-treatment-tb (accessed May 14, 2024).
68. World Health Organization. WHO Consolidated Guidelines on Tuberculosis. Module 4: Treatment-Drug-Resistant Tuberculosis Treatment, 2022 Update. Geneva: World Health Organization (2022).
69. Imperial MZ, Nahid P, Phillips PP, Davies GR, Fielding K, Hanna D, et al. A patient-level pooled analysis of treatment-shortening regimens for drug-susceptible pulmonary tuberculosis. Nat Med. (2018) 24:1708–15. doi: 10.1038/s41591-018-0224-2
70. Volmink J, Garner P. Directly observed therapy for treating tuberculosis. Cochrane Database Syst Rev. (2007). doi: 10.1002/14651858.CD003343.pub3
71. Pradipta IS, Houtsma D, van Boven JF, Alffenaar J-WC, Hak E. Interventions to improve medication adherence in tuberculosis patients: a systematic review of randomized controlled studies. NPJ Prim Care Respira Med. (2020) 30:21. doi: 10.1038/s41533-020-0179-x
72. Munro SA, Lewin SA, Smith HJ, Engel ME, Fretheim A, Volmink J. Patient adherence to tuberculosis treatment: a systematic review of qualitative research. PLoS Med. (2007) 4:e238. doi: 10.1371/journal.pmed.0040238
73. Chavez-Rimache L, Ugarte-Gil C, Brunette MJ. The community as an active part in the implementation of interventions for the prevention and care of tuberculosis: a scoping review. PLOS Glob Public Health. (2023) 3:e0001482. doi: 10.1371/journal.pgph.0001482
74. World Health Organization. Antimicrobial Resistance (2023). Available at: https://www.who.int/news-room/fact-sheets/detail/antimicrobial-resistance (accessed May 6, 2024).
75. Jonas OB, Irwin A, Berthe FCJ, Le Gall FG, Marquez PV. Drug-Resistant Infections: A Threat to Our Economic Future. Washington, DC: World Bank Group (2017).
76. Liebenberg D, Gordhan BG, Kana BD. Drug resistant tuberculosis: implications for transmission, diagnosis, and disease management. Front Cell Infect Microbiol. (2022) 12:943545. doi: 10.3389/fcimb.2022.943545
77. Zweynert A. Drug-Resistant TB Threatens to Kill 75 Million People by 2050, Cost $16.7 Trillion. London: Reuters (2015). Available at: https://www.reuters.com/article/us-health-tuberculosis-economy-idUSKBN0MK00520150324/ (accessed May 6, 2024).
78. Centers for Disease Control and Prevention. Drug Resistant TB. Available at: https://www.cdc.gov/tb/topic/drtb/default.htm#:~:text=Drug%2Dresistant%20TB%20is%20caused,)%20and%20rifampin%20(RIF) (accessed October 13, 2022).
79. Yusoof KA, García JI, Schami A, Garcia-Vilanova A, Kelley HV, Wang S-H, et al. Tuberculosis phenotypic and genotypic drug susceptibility testing and immunodiagnostics: a review. Front Immunol. (2022) 13:870768. doi: 10.3389/fimmu.2022.870768
80. TB Alliance. Drug Resistance, A response to antimicrobial resistance includes tackling TB. Available at: https://www.tballiance.org/why-new-tb-drugs/antimicrobial-resistance (accessed May 6, 2024).
81. Seung KJ, Keshavjee S, Rich ML. Multidrug-resistant tuberculosis and extensively drug-resistant tuberculosis. Cold Spring Harb Perspect Med. (2015) 5:a017863. doi: 10.1101/cshperspect.a017863
82. World Health Organization. Antimicrobial Resistance: Global Report on Surveillance. Geneva: World Health Organization (2014).
83. Bhattarai MD. Universal drug-susceptibility testing of first-line drugs to preserve their efficacy: an essential strategy to defeat tuberculosis. J Clin Tuberc Other Mycobact Dis. (2023) 33:100394. doi: 10.1016/j.jctube.2023.100394
84. Svadzian A, Sulis G, Gore G, Pai M, Denkinger CM. Differential yield of universal versus selective drug susceptibility testing of patients with tuberculosis in high-burden countries: a systematic review and meta-analysis. BMJ Global Health. (2020) 5:e003438. doi: 10.1136/bmjgh-2020-003438
85. TB Alliance. New Study: Recently Approved Drug-Resistant TB Treatments Can Save National Governments up to US$740 Million Annually. (2016). Available at: https://www.tballiance.org.za/news/study-plos-global-public-health (accessed May 7, 2024).
86. Wadman M. Major drug company bends in battle over access to key TB treatment. (2023). Available at: https://www.science.org/content/article/major-drug-company-bends-battle-over-access-key-tb-treatment (accessed May 7, 2024).
87. Kock R, Michel AL, Yeboah-Manu D, Azhar EI, Torrelles JB, Cadmus SI, et al. Zoonotic tuberculosis–the changing landscape. Int J Infect Dis. (2021) 113:S68–72. doi: 10.1016/j.ijid.2021.02.091
90. World Health Organization. An Investment Case for New Tuberculosis Vaccines. Geneva: World Health Organization (2022).
91. World Health Organization. Investing in new TB vaccines: It's time to end the century-long wait! (2021). Available at: https://www.who.int/news/item/16-07-2021-investing-in-new-tb-vaccines (accessed February 14, 2024).
92. Fine PE. Variation in protection by BCG: implications of and for heterologous immunity. Lancet. (1995) 346:1339–45. doi: 10.1016/S0140-6736(95)92348-9
93. Vaudry W. “To BCG or not to BCG, that is the question!”. The challenge of BCG vaccination: Why can't we get it right? Paediatr Child Health. (2003) 8:141–4. doi: 10.1093/pch/8.3.141
94. Ottenhoff TH, Kaufmann SH. Vaccines against tuberculosis: where are we and where do we need to go? PLoS Pathog. (2012) 8:e1002607. doi: 10.1371/journal.ppat.1002607
95. Okafor CN, Rewane A, Momodu II. Bacillus Calmette Guerin. Treasure Island, FL: StatPearls (2019).
96. Mangtani P, Abubakar I, Ariti C, Beynon R, Pimpin L, Fine PE, et al. Protection by BCG vaccine against tuberculosis: a systematic review of randomized controlled trials. Clin Infect Dis. (2014) 58:470–80. doi: 10.1093/cid/cit790
97. Trunz BB, Fine P, Dye C. Effect of BCG vaccination on childhood tuberculous meningitis and miliary tuberculosis worldwide: a meta-analysis and assessment of cost-effectiveness. Lancet. (2006) 367:1173–80. doi: 10.1016/S0140-6736(06)68507-3
98. Trollfors B, Sigurdsson V, Dahlgren-Aronsson A. Prevalence of latent TB and effectiveness of BCG vaccination against latent tuberculosis: an observational study. Int J Infect Dis. (2021) 109:279–82. doi: 10.1016/j.ijid.2021.06.045
99. Katelaris AL, Jackson C, Southern J, Gupta RK, Drobniewski F, Lalvani A, et al. Effectiveness of BCG vaccination against Mycobacterium tuberculosis infection in adults: a cross-sectional analysis of a UK-based cohort. J Infect Dis. (2020) 221:146–55. doi: 10.1093/infdis/jiz430
101. World Health Organization. Preferred product characteristics for therapeutic vaccines to improve tuberculosis treatment outcomes. Geneva: World Health Organization (2019.)
102. World Health Organization. WHO preferred product characteristics for new tuberculosis vaccines. Geneva: World Health Organization (2018).
103. U.S. Department of Health and Human Services. Study of the Safety and Efficacy of the Subunit Recombinant Tuberculosis Vaccine GamTBvac ref.NCT04975737. Available at: clinicaltrials.gov/study/NCT04975737 (accessed November 30, 2022).
104. Talwar GP, Gupta JC, Mustafa AS, Kar HK, Katoch K, Parida SK, et al. Development of a potent invigorator of immune responses endowed with both preventive and therapeutic properties. Biologics. (2017) 11:55–63. doi: 10.2147/BTT.S128308
105. Pérez I, Uranga S, Sayes F, Frigui W, Samper S, Arbués A, et al. Live attenuated TB vaccines representing the three modern Mycobacterium tuberculosis lineages reveal that the Euro–American genetic background confers optimal vaccine potential. EBioMedicine. (2020) 55:102761. doi: 10.1016/j.ebiom.2020.102761
106. Li J, Zhao A, Tang J, Wang G, Shi Y, Zhan L, et al. Tuberculosis vaccine development: from classic to clinical candidates. Eur J Clin Microbiol Infect Dis. (2020) 39:1405–25. doi: 10.1007/s10096-020-03843-6
107. United Sates Government National Institute of Health. Study to Check the Efficacy and Safety of Recombinant BCG Vaccine in Prevention of TB Recurrence ref. NCT03152903. Available at: clinicaltrials.gov/study/NCT03152903 (accessed December 4, 2023).
108. United Sates Government National Institute of Health. Evaluation of Efficacy and Safety of VPM1002 in Comparison to BCG in Prevention of TB Infection in Infants (VPM1002) ref. NCT04351685. Available at: clinicaltrials.gov/study/NCT04351685 (accessed December 20, 2022).
109. Zhuang L, Ye Z, Li L, Yang L, Gong W. Next-generation TB vaccines: progress, challenges, and prospects. Vaccines. (2023) 11:1304. doi: 10.3390/Vaccines11081304
110. Manzoni C, Kia DA, Vandrovcova J, Hardy J, Wood NW, Lewis PA, et al. Genome, transcriptome and proteome: the rise of omics data and their integration in biomedical sciences. Brief Bioinform. (2018) 19:286–302. doi: 10.1093/bib/bbw114
111. Romano M, Squeglia F, Kramarska E, Barra G, Choi H-G, Kim H-J, et al. A structural view at vaccine development against M. tuberculosis. Cells. (2023) 12:317. doi: 10.3390/cells12020317
112. Gong W, Liang Y, Wu X. Animal models of tuberculosis vaccine research: an important component in the fight against tuberculosis. BioMed Res Int. (2020) 2020:4263079. doi: 10.1155/2020/4263079
113. Fennelly KP, Jones-López EC, Ayakaka I, Kim S, Menyha H, Kirenga B, et al. Variability of infectious aerosols produced during coughing by patients with pulmonary tuberculosis. Am J Respir Crit Care Med. (2012) 186:450–7. doi: 10.1164/rccm.201203-0444OC
114. Trauer JM, Dodd PJ, Gomes MGM, Gomez GB, Houben RM, McBryde ES, et al. The importance of heterogeneity to the epidemiology of tuberculosis. Clin Infect Dis. (2019) 69:159–66. doi: 10.1093/cid/ciy938
115. United Sates Government National Institute of Health. Decades in the Making: mRNA COVID-19 Vaccines. Available at: https://covid19.nih.gov/nih-strategic-response-covid-19/decades-making-mrna-covid-19-vaccines (accessed January 10, 2023).
116. Dockrell HM, McShane H. Tuberculosis vaccines in the era of COVID-19–what is taking us so long? EBioMedicine. (2022) 79:103993. doi: 10.1016/j.ebiom.2022.103993
117. Portnoy A, Clark RA, Quaife M, Weerasuriya CK, Mukandavire C, Bakker R, et al. The cost and cost-effectiveness of novel tuberculosis vaccines in low-and middle-income countries: a modeling study. PLoS Med. (2023) 20:e1004155. doi: 10.1371/journal.pmed.1004155
118. World Health Organization. Tuberculosis Vaccine Accelerator Council. Available at: https://www.who.int/initiatives/tuberculosis-vaccine-accelerator-council (accessed February 15, 2024).
119. Centers for Disease Control and Prevention. Social Determinants of Health at CDC. (2022). Available at: https://www.cdc.gov/about/sdoh/index.html (accessed April 18, 2024).
120. Hargreaves JR, Boccia D, Evans CA, Adato M, Petticrew M, Porter JD. The social determinants of tuberculosis: from evidence to action. Am J Public Health. (2011) 101:654–62. doi: 10.2105/AJPH.2010.199505
121. World Health Organization. Social Determinants of Health. Available at: https://www.who.int/health-topics/social-determinants-of-health#tab=tab_1 (accessed April 18, 2024).
122. Khazaei S, Rezaeian S, Baigi V, Saatchi M, Molaeipoor L, Khazaei Z, et al. Incidence and pattern of tuberculosis treatment success rates in different levels of the human development index: a global perspective. S Afr J Infect Dis. (2017) 32:100–4. doi: 10.1080/23120053.2017.1321243
123. Stop TB Partnership. Mobile Populations. Key Populations Brief . Available at: https://stoptb.org/assets/documents/resources/publications/acsm/kp_mobile_spreads.pdf (accessed April 18, 2024).
124. Boyarinova G. Management of TB in Refugee Populations. (2012). Available at: https://med.virginia.edu/family-medicine/wp-content/uploads/sites/285/2017/02/Galina-Boyarinova-TB-Management_Web.pdf (accessed April 12, 2024).
125. World Health Organization. Social determinants. Available at: https://www.who.int/teams/global-tuberculosis-programme/populations-comorbidities/social-determinants#:~:text=Crowded%20and%20poorly%20ventilated%20living,factor%20for%20developing%20active%20disease (accessed April 16, 2024).
126. Anglicare Southern Queensland. Senate Community Affairs References Committee: The extent and nature of poverty in Australia. (2023). Available at: https://anglicaresq.org.au/wp-content/uploads/2023/03/20230203_Inquiry-into-poverty-in-Australia_Anglicare-Sthn-Qld_v1.1.pdf (accessed April 16, 2024).
127. Jericho G. The pandemic showed us that poverty is a policy choice- we must do better. New York, NY: The Guardian (2022).
128. The World Bank. Ending Poverty and Ensuring Dignity for All. (2023). Available at: https://www.worldbank.org/en/news/feature/2023/10/16/end-poverty-and-ensure-dignity-for-all (accessed April 18, 2024).
129. World Food Programme. 6 solutions to end hunger and malnutrition: WFP Appeal to G7 leaders in Hiroshima. (2023). Available at: https://www.wfp.org/stories/6-solutions-end-hunger-and-malnutrition-wfp-appeal-g7-leaders-hiroshima#:~:text=Immediate%20impact&text=Food%20assistance%20is%20crucial%20to,and%20increasing%20sustainable%20agricultural%20production (accessed April 21, 2024).
130. World Health Organization and International Bank for Reconstruction and Development/The World Bank. Tracking Universal Health Coverage: 2017 Global Monitoring Report. Geneva: WHO (2017). Report No.: ISBN 978-92-4-151355-5.
131. World Health Organization. Universal Health Coverage. Available at: https://www.who.int/publications/digital/global-tuberculosis-report-2021/uhc-tb-determinants/universal-health-coverage (accessed April 19, 2024).
132. Bastos ML, Menzies D, Hone T, Dehghani K, Trajman A. The impact of the Brazilian family health on selected primary care sensitive conditions: a systematic review. PLoS ONE. (2017) 12:e0182336. doi: 10.1371/journal.pone.0182336
133. Pelissari DM, Oliveira PB. Universal health coverage: an effective intervention for a tuberculosis-free world. Lancet Glob Health. (2022) 10:e309–10. doi: 10.1016/S2214-109X(21)00564-7
134. World Health Organization. Universal Health Coverage and TB determinants. Available at: https://www.who.int/teams/global-tuberculosis-programme/tb-reports/global-tuberculosis-report-2022/uhc-tb-determinants/6.1-universal-health-coverage (accessed April 19, 2024).
135. World Health Organization. Equity of and access to services for prevention of tuberculosis and treatment of patients. In: Implementing the WHO stop TB strategy. (2008). Available at: https://www.ncbi.nlm.nih.gov/books/NBK310763/ (accessed April 19, 2024).
136. Msoka EF, Orina F, Sanga ES, Miheso B, Mwanyonga S, Meme H, et al. Qualitative assessment of the impact of socioeconomic and cultural barriers on uptake and utilisation of tuberculosis diagnostic and treatment tools in East Africa: a cross-sectional study. BMJ Open. (2021) 11:e050911. doi: 10.1136/bmjopen-2021-050911
137. McArthur E, Bali S, Khan AA. Socio-cultural and knowledge-based barriers to tuberculosis diagnosis for women in Bhopal, India. Indian J Community Med. (2016) 41:62–4. doi: 10.4103/0970-0218.170990
138. New Jersey Medical School Global Tuberculosis Institute. Cultural Competency and Tuberculosis Care: A Guide for Self-Study and Self-Assessment. Geneva: WHO (2008).
139. Pradipta IS, Idrus LR, Probandari A, Puspitasari IM, Santoso P, Alffenaar J-WC, et al. Barriers to optimal tuberculosis treatment services at community health centers: a qualitative study from a high prevalent tuberculosis country. Front Pharmacol. (2022) 13:857783. doi: 10.3389/fphar.2022.857783
140. Zawedde-Muyanja S, Manabe YC, Cattamanchi A, Castelnuovo B, Katamba A. Patient and health system level barriers to and facilitators for tuberculosis treatment initiation in Uganda: a qualitative study. BMC Health Serv Res. (2022) 22:831. doi: 10.1186/s12913-022-08213-w
141. Jops P, Cowan J, Kupul M, Trumb RN, Graham SM, Bauri M, et al. Beyond patient delay, navigating structural health system barriers to timely care and treatment in a high burden TB setting in Papua New Guinea. Glob Public Health. (2023) 18:2184482. doi: 10.1080/17441692.2023.2184482
142. Transparency International Global Health. Anti-corruption, the missing link in global health security and the attainment of universal health coverage. (2023). Available at: https://ti-health.org/content/anti-corruption-the-missing-link-in-global-health-security-and-the-attainment-of-universal-health-coverage/ (accessed April 24, 2024).
143. García PJ. Corruption: possibly the biggest threat to health care. Lancet Reg Health Am. (2024) 32:100744. doi: 10.1016/j.lana.2024.100744
144. Abdullah A, Husten C. Promotion of smoking cessation in developing countries: a framework for urgent public health interventions. Thorax. (2004) 59:623–30. doi: 10.1136/thx.2003.018820
145. Siddiqi K, Khan A, Ahmad M. An intervention to stop smoking among patients suspected of TB-evaluation of an integrated approach. BMC Public Health. (2010) 10:1–13. doi: 10.1186/1471-2458-10-160
146. Bates M, Marais BJ, Zumla A. Tuberculosis comorbidity with communicable and noncommunicable diseases. Cold Spring Harb Perspect Med. (2015) 5:a017889. doi: 10.1101/cshperspect.a017889
147. The World Bank. Measuring Poverty. (2023). Available at: https://www.worldbank.org/en/topic/measuringpoverty (accessed April 18, 2024).
148. Sachs JD, Karim SSA, Aknin L, Allen J, Brosbøl K, Colombo F, et al. The Lancet Commission on lessons for the future from the COVID-19 pandemic. Lancet. (2022) 400:1224–80. doi: 10.1016/S0140-6736(22)01585-9
149. Sheikh JA, Malik AA, Quadir N, Ehtesham NZ, Hasnain SE. Learning from COVID-19 to tackle TB pandemic: from despair to hope. Lancet Reg Health Southeast Asia. (2022) 2:100015. doi: 10.1016/j.lansea.2022.05.004
150. Management Sciences for Health. Tackling TB: Three Lessons the COVID-19 Pandemic Taught Us. (2022). Available at: https://msh.org/story/tackling-tb-three-lessons-the-covid-19-pandemic-taught-us/
151. Oga-Omenka C, Tseja-Akinrin A, Boffa J, Heitkamp P, Pai M, Zarowsky C. Commentary: lessons from the COVID-19 global health response to inform TB case finding. Healthcare. (2021) 9:100487. doi: 10.1016/j.hjdsi.2020.100487
152. London School of Hygiene & Tropical Medicine. Will the war in Ukraine lead to a spike in tuberculosis cases? (2022). Available at: https://www.lshtm.ac.uk/research/centres/health-humanitarian-crises-centre/news/343596/will-war-ukraine-lead-spike-tuberculosis-cases (accessed April 27, 2024).
153. World Health Organization. Fighting TB amidst the fighting in Syria. (2020). Available at: https://www.who.int/europe/news-room/photo-stories/item/fighting-tb-amidst-the-fighting-in-syria (accessed April 27, 2024).
154. Gele AA, Bjune GA. Armed conflicts have an impact on the spread of tuberculosis: the case of the Somali Regional State of Ethiopia. Confl Health. (2010) 4:1–6. doi: 10.1186/1752-1505-4-1
155. Seddiq K, Enarson DA, Shah K, Haq Z, Khan WM. Implementing a successful tuberculosis programme within primary care services in a conflict area using the stop TB strategy: Afghanistan case study. Confl Health. (2014) 8:1–9. doi: 10.1186/1752-1505-8-3
156. Health MSf. Tackling TB in Conflict Settings: The Value of Community-Based Interventions in Afghanistan. (2023). Available at: https://msh.org/story/tackling-tb-in-conflict-settings-the-value-of-community-based-interventions-in-afghanistan/ (accessed April 29, 2024).
157. PATH. TB prevention and treatment in Ukraine: Keeping momentum through conflict. (2023). Available at: https://www.path.org/our-impact/articles/tb-prevention-and-treatment-in-ukraine-keeping-momentum-through-conflict/ (accessed April 29, 2024).
158. Das M, Isaakidis P, Armstrong E, Gundipudi NR, Babu RB, Qureshi IA, et al. Directly-observed and self-administered tuberculosis treatment in a chronic, low-intensity conflict setting in India. PLoS ONE. (2014) 9:e92131. doi: 10.1371/journal.pone.0092131
159. Liddle KF, Elema R, Thi SS, Greig J, Venis S. TB treatment in a chronic complex emergency: treatment outcomes and experiences in Somalia. Trans R Soc Trop Med Hyg. (2013) 107:690–8. doi: 10.1093/trstmh/trt090
160. International Monetary Fund. Linking climate change and inequality. (2021). Available at: https://www.imf.org/en/Publications/fandd/issues/2021/09/climate-change-and-inequality-guivarch-mejean-taconet (accessed April 29, 2024).
161. Kharwadkar S, Attanayake V, Duncan J, Navaratne N, Benson J. The impact of climate change on the risk factors for tuberculosis: a systematic review. Environ Res. (2022) 212:113436. doi: 10.1016/j.envres.2022.113436
162. Maharjan B, Gopali RS, Zhang Y. A scoping review on climate change and tuberculosis. Int J Biometeorol. (2021) 65:1579–95. doi: 10.1007/s00484-021-02117-w
163. Rodríguez-Verdugo A, Lozano-Huntelman N, Cruz-Loya M, Savage V, Yeh P. Compounding effects of climate warming and antibiotic resistance. IScience. (2020) 23:101024. doi: 10.1016/j.isci.2020.101024
164. World Health Organization. TB & HIV. Available at: https://www.who.int/teams/global-tuberculosis-programme/populations-comorbidities/hiv (accessed April 30, 2024).
165. Centers for Disease Control and Prevention. TB and HIV Coinfection. (2016). Available at: https://www.cdc.gov/tb/topic/basics/tbhivcoinfection.htm (accessed April 30, 2024).
166. Lua I, Silva AF, Guimarães NS, Magno L, Pescarini J, Anderle RV, et al. The effects of social determinants of health on acquired immune deficiency syndrome in a low-income population of Brazil: a retrospective cohort study of 28.3 million individuals. Lancet Reg Health–Am. (2023) 24:100554. doi: 10.1016/j.lana.2023.100554
167. Andrade LA, de França Amorim T, da Paz WS, do Rosário Souza MS, Camargo EL, dos Santos Tavares D, et al. Reduced HIV/AIDS diagnosis rates and increased AIDS mortality due to late diagnosis in Brazil during the COVID-19 pandemic. Sci Rep. (2023) 13:23003. doi: 10.1038/s41598-023-50359-y
168. UNAIDS. Focus–AIDS and conflict: a growing problem worldwide. 2004 Report on the AIDS Global Epidemic. Geneva: UNAIDS (2004), p. 75–81.
169. World Health Organization. World Health Organization guidance on integrating the prevention and control of noncommunicable diseases in HIV/AIDS, tuberculosis, and sexual and reproductive health programmes. (2023). Available at: https://www.who.int/news/item/05-04-2023-world-health-organization-guidance-on-integrating-the-prevention-and-control-of-noncommunicable-diseases-in-hiv-aids-tuberculosis-and-sexual-and-reproductive-health-programmes (accessed April 30, 2024).
170. Hermans SM, Castelnuovo B, Katabira C, Mbidde P, Lange JM, Hoepelman AI, et al. Integration of HIV and TB services results in improved TB treatment outcomes and earlier prioritized ART initiation in a large urban HIV clinic in Uganda. J Acquir Immune Defic Syndr. (2012) 60:e29–35. doi: 10.1097/QAI.0b013e318251aeb4
171. Yorke E, Atiase Y, Akpalu J, Sarfo-Kantanka O, Boima V, Dey ID. The bidirectional relationship between tuberculosis and diabetes. T uberc Res Treat. (2017) 2017:1702578. doi: 10.1155/2017/1702578
172. Niazi AK, Kalra S. Diabetes and tuberculosis: a review of the role of optimal glycemic control. J Diabetes Metab Disord. (2012) 11:1–4. doi: 10.1186/2251-6581-11-28
173. Ong KL, Stafford LK, McLaughlin SA, Boyko EJ, Vollset SE, Smith AE, et al. Global, regional, and national burden of diabetes from 1990 to 2021, with projections of prevalence to 2050: a systematic analysis for the Global Burden of Disease Study 2021. Lancet. (2023) 402:203–34. doi: 10.1016/S0140-6736(23)01301-6
174. Chan JC, Lim L-L, Wareham NJ, Shaw JE, Orchard TJ, Zhang P, et al. The Lancet Commission on diabetes: using data to transform diabetes care and patient lives. Lancet. (2020) 396:2019–82. doi: 10.1016/S0140-6736(20)32374-6
175. World Health Organization. Collaborative framework for care and control of tuberculosis and diabetes. Geneva: World Health Organization (2011). Report No.: 9241502258.
176. World Health Organization regional Office for South-East Asia. Integrated care for tuberculosis (TB) and diabetes mellitus (DM) comorbidity in Asian countries: health system challenges and opportunities. In: Policies APOoHSa. Geneva: WHO (2022).
177. Milice DM, Macicame IL, Peñalvo J. The collaborative framework for the management of tuberculosis and type 2 diabetes syndemic in low-and middle-income countries: a rapid review. BMC Public Health. (2024) 24:738. doi: 10.1186/s12889-024-18256-9
178. Australian Bureau of Statistics. Diabetes: Key statistics. (2023). Available at: https://www.abs.gov.au/statistics/health/health-conditions-and-risks/diabetes/latest-release (accessed May 1, 2024).
179. Caraux-Paz P, Diamantis S, de Wazières B, Gallien S. Tuberculosis in the elderly. J Clin Med. (2021) 10:5888. doi: 10.3390/jcm10245888
180. World Health Organization. Ageing and Health. (2022). Available at: https://www.who.int/news-room/fact-sheets/detail/ageing-and-health#:~:text=At%20this%20time%20the%20share,2050%20to%20reach%20426%20million (accessed May 1, 2024).
181. Teo AKJ, Morishita F, Islam T, Viney K, Ong CW, Kato S, et al. Tuberculosis in older adults: challenges and best practices in the Western Pacific Region. Lancet Reg Health West Pac. (2023) 36:100770. doi: 10.1016/j.lanwpc.2023.100770
182. Alene KA, Hertzog L, Gilmour B, Clements AC, Murray MB. Interventions to prevent post-tuberculosis sequelae: a systematic review and meta-analysis. EClinicalMedicine. (2024) 70:102511. doi: 10.1016/j.eclinm.2024.102511
183. Rachow A, Ivanova O, Wallis R, Charalambous S, Jani I, Bhatt N, et al. TB sequel: incidence, pathogenesis and risk factors of long-term medical and social sequelae of pulmonary TB–a study protocol. BMC Pulm Med. (2019) 19:1–9. doi: 10.1186/s12890-018-0777-3
184. van Kampen SC, Wanner A, Edwards M, Harries AD, Kirenga BJ, Chakaya J, et al. International research and guidelines on post-tuberculosis chronic lung disorders: a systematic scoping review. BMJ Glob Health. (2018) 3:e000745. doi: 10.1136/bmjgh-2018-000745
185. Dodd PJ, Yuen CM, Jayasooriya SM, van der Zalm MM, Seddon JA. Quantifying the global number of tuberculosis survivors: a modelling study. Lancet Infect Dis. (2021) 21:984–92. doi: 10.1016/S1473-3099(20)30919-1
187. Venkatesan P. Worrying lack of funding for tuberculosis. Lancet Infect Dis. (2022) 22:318. doi: 10.1016/S1473-3099(22)00073-1
188. Ijaz RK, Biswas RG, Bland S, Figueroa P, Hopkins D, Lammie P, et al. Weekly epidemiological record. In: 33rd Meeting of the International Task Force for Disease Eradication, 14-15 March 2022, Vol. 97. World Health Organization (2022). p. 325–336.
189. The Stop TB Partnership. New report by TAG and STOP TB: TB R&D Funding Perilously Off Track of UN Commitments to End TB by 2030. (2023). Available at: https://www.stoptb.org/news/new-report-tag-and-stop-tb-tb-rd-funding-perilously-off-track-of-un-commitments-to-end-tb-2030#:~:text=Global%20funding%20for%20TB%20R%26D,cases%20of%20TB%20each%20year (accessed April 22, 2024).
190. World Health Organization. Financing for TB prevention, diagnostic and treatment services. Available at: https://www.who.int/teams/global-tuberculosis-programme/tb-reports/global-tuberculosis-report-2022/financing-for-tb (accessed April 22, 2024).
191. The Global Fund. About the Global Fund. (2024). Available at: https://www.theglobalfund.org/en/about-the-global-fund/
192. Yadav S, Rawal G, Jeyaraman M. India's decision to deny an extension of patent for bedaquiline: a public health imperative. Cureus. (2023) 15:49542. doi: 10.7759/cureus.49542
193. Medicins Sans Frontieres. MSF calls for increasing access to lifesaving TB drug. (2023). Available at: https://msf.org.au/article/statements-opinion/msf-calls-increasing-access-lifesaving-tb-drug (accessed April 24, 2024).
194. Reliefweb. DR-TB Drugs Under the Microscope (8th edition): Pricing and patent landscape of medicines for adults and children. United Nations Office for the Coordination of Humanitarian Affairs (OCHA). (2022). Available at: https://reliefweb.int/report/world/dr-tb-drugs-under-microscope-8th-edition-pricing-and-patent-landscape-medicines-adults-and-children (accessed April 24, 2024).
195. Reliefweb. TB test price reduction by Cepheid and Danaher is an important step in the right direction. (2023). Available at: https://reliefweb.int/report/world/tb-test-price-reduction-cepheid-and-danaher-important-step-right-direction (accessed April 24, 2024).
196. The Global Fund. New Pricing for Cepheid GeneXpert Tuberculosis Testing. (2023). Available at: https://www.theglobalfund.org/media/13442/operational_2023-10-cepheid-genexpert-tb-testing_briefingnote_en.pdf (accessed April 24, 2024).
197. Medicins Sans Frontieres. Campaign Time for $5. Available at: https://www.msfaccess.org/time-for-5 (accessed April 24, 2024).
198. Gotham D, McKenna L, Frick M, Lessem E. Public investments in the clinical development of bedaquiline. PLoS ONE. (2020) 15:e0239118. doi: 10.1371/journal.pone.0239118
199. McKinsey & Company. What is AI (artificial intelligence)? (2024). Available at: https://www.mckinsey.com/featured-insights/mckinsey-explainers/what-is-ai (accessed April 10, 2024).
200. Miller DD, Brown EW. Artificial intelligence in medical practice: the question to the answer? Am J Med. (2018) 131:129–33. doi: 10.1016/j.amjmed.2017.10.035
201. Alowais SA, Alghamdi SS, Alsuhebany N, Alqahtani T, Alshaya AI, Almohareb SN, et al. Revolutionizing healthcare: the role of artificial intelligence in clinical practice. BMC Med Educ. (2023) 23:689. doi: 10.1186/s12909-023-04698-z
202. World Health Organization. WHO issues first global report on Artificial Intelligence (AI) in health and six guiding principles for its design and use. (2021). Available at: https://wwwwhoint/news/item/28-06-2021 (accessed April 10, 2024).
203. World Health Organization. Health workforce. Available at: https://www.who.int/health-topics/health-workforce#tab=tab_1 (accessed April 21, 2024).
204. Liang S, Ma J, Wang G, Shao J, Li J, Deng H, et al. The application of artificial intelligence in the diagnosis and drug resistance prediction of pulmonary tuberculosis. Frontiers in medicine. (2022) 9:935080. doi: 10.3389/fmed.2022.935080
205. Khan B, Fatima H, Qureshi A, Kumar S, Hanan A, Hussain J, et al. Drawbacks of artificial intelligence and their potential solutions in the healthcare sector. Biomed Mater Devices. (2023) 1:731–8. doi: 10.1007/s44174-023-00063-2
206. Pesapane F, Volonté C, Codari M, Sardanelli F. Artificial intelligence as a medical device in radiology: ethical and regulatory issues in Europe and the United States. Insights Imaging. (2018) 9:745–53. doi: 10.1007/s13244-018-0645-y
207. Maliha G, Gerke S, Cohen IG, Parikh RB. Artificial intelligence and liability in medicine: balancing safety and innovation. Milbank Q. (2021) 99:629. doi: 10.1111/1468-0009.12504
208. Jiang L, Wu Z, Xu X, Zhan Y, Jin X, Wang L, et al. Opportunities and challenges of artificial intelligence in the medical field: current application, emerging problems, and problem-solving strategies. J Int Med Res. (2021) 49:03000605211000157. doi: 10.1177/03000605211000157
209. David P-M, Onno J, Keshavjee S, Khan FA. Conditions required for the artificial-intelligence-based computer-aided detection of tuberculosis to attain its global health potential. Lancet Digit Health. (2022) 4:e702–4. doi: 10.1016/S2589-7500(22)00172-8
210. World Health Organization. TDR and WHO announce TDA4Child, an operational research package to generate data on treatment decision algorithms for pulmonary tuberculosis in children. (2023). Available at: https://wwwwhoint/news/item/15-05-2023 (accessed April 11, 2024).
211. World Health Organization. WHO consolidated guidelines on tuberculosis. Module 2: screening-systematic screening for tuberculosis disease. Geneva: World Health Organization (2021).
212. Geric C, Qin Z, Denkinger C, Kik S, Marais B, Anjos A, et al. The rise of artificial intelligence reading of chest X-rays for enhanced TB diagnosis and elimination. Int J Tuberc Lung Dis. (2023) 27:367–72. doi: 10.5588/ijtld.22.0687
213. University of Geogia College of Public Health. Artificial intelligence could reduce barriers to TB care. (2023). Available at: https://publichealth.uga.edu/artificial-intelligence-could-reduce-barriers-to-tb-care/ (accessed April 12, 2024).
214. Salcedo J, Rosales M, Kim JS, Nuno D. Suen S-c, Chang AH. Cost-effectiveness of artificial intelligence monitoring for active tuberculosis treatment: a modeling study. PLoS ONE. (2021) 16:e0254950. doi: 10.1371/journal.pone.0254950
215. The Conversation. AI can help predict whether a patient will respond to specific tuberculosis treatments, paving way for personalized care. (2024). Available at: https://theconversation.com/ai-can-help-predict-whether-a-patient-will-respond-to-specific-tuberculosis-treatments-paving-way-for-personalized-care-225637 (accessed April 12, 2024).
216. Zhang F, Zhang F, Li L, Pang Y. Clinical utilization of artificial intelligence in predicting therapeutic efficacy in pulmonary tuberculosis. J Infect Public Health. (2024). doi: 10.1016/j.jiph.2024.02.012
217. GIS People. GIS in Public Health: Disease Mapping. Available at: https://www.gispeople.com.au/gis-in-public-health-disease-mapping/ (accessed May 17, 2024).
218. Biu PW, Nwasike CN, Tula OA, Ezeigweneme CA, Gidiagba JO. A review of GIS applications in public health surveillance. World J. Adv. Res. Rev. (2024) 21:30–039. doi: 10.30574/wjarr.2024.21.1.2684
219. World Health Organization. Digital Resources for Tuberculosis. Available at: https://www.who.int/tools/digital-resources-for-tuberculosis (accessed April 15, 2024).
220. World Health Organization. Mental health - a human right for people affected by tuberculosis. (2023). Available at: https://www.who.int/news/item/09-10-2023-mental-health-a-human-right-for-people-affected-by-tuberculosis#:~:text=People%20affected%20by%20TB%20have,suffered%20by%20people%20with%20TB (accessed April 15, 2024).
221. The Royal Australian and New Zealand College of Psychiatrists. Benefits of e-mental health treatments and interventions. (2023). Available from: https://www.ranzcp.org/clinical-guidelines-publications/clinical-guidelines-publications-library/benefits-of-e-mental-health-treatments-and-interventions (accessed April 15, 2024).
222. Bahrainwala L, Knoblauch AM, Andriamiadanarivo A, Diab MM, McKinney J, Small PM, et al. Drones and digital adherence monitoring for community-based tuberculosis control in remote Madagascar: a cost-effectiveness analysis. PLoS ONE. (2020) 15:e0235572. doi: 10.1371/journal.pone.0235572
223. World Economic Forum. How drones are transforming access to healthcare in India. (2023). Available at: https://www.weforum.org/impact/drones-delivering-vaccines/ (accessed April 12, 2024).
224. United Nations. Solar for Health: Five Ways Solar Power Can Make Universal Healthcare a Reality. Available at: https://www.un.org/en/un-chronicle/solar-health-five-ways-solar-power-can-make-universal-healthcare-reality (accessed April 16, 2024).
225. The Union. The Union's TB Care Project Lights up TB-HIV Clinics in Zimbabwe with Solar Power. (2014). Available at: https://theunion.org/news/the-union%E2%80%99s-tb-care-project-lights-up-tb-hiv-clinics-in-zimbabwe-with-solar-power (accessed April 16, 2024).
226. United Nations. Renewable energy proves essential to service continuation of the National HIV and TB Centre in Beirut. Available at: https://unhabitat.org/news/12-oct-2021/renewable-energy-proves-essential-to-service-continuation-of-the-national-hiv-and (accessed April 16, 2024).
227. Power Sonic Corporation. Why Energy Storage is Vital for Healthcare. Available at: https://www.power-sonic.com/blog/batteries/why-energy-storage-is-vital-for-healthcare/ (accessed April 16, 2024).
228. Lohum. Lithium Battery Technology in Medicine: Powering Medical Devices and Health Infrastructure. (2023). Available at: https://lohum.com/media/blog/lithium-battery-technology-in-medicine-powering-medical-devices-and-health-infrastructure/ (accessed April 16, 2024).
229. Solutions for Tomorrow. Expanding Healthcare Accessibility With A Mobile X-Ray Van. Available at: https://solutionsfortomorrow.se/expanding-healthcare-accessibility-with-a-mobile-x-ray-van/ (accessed April 16, 2024).
230. Chopra H, Mohanta YK, Rauta PR, Ahmed R, Mahanta S, Mishra PK, et al. An insight into advances in developing nanotechnology based therapeutics, drug delivery, diagnostics and vaccines: multidimensional applications in tuberculosis disease management. Pharmaceuticals. (2023) 16:581. doi: 10.3390/ph16040581
231. Muthukrishnan L. Multidrug resistant tuberculosis–diagnostic challenges and its conquering by nanotechnology approach–an overview. Chem Biol Interact. (2021) 337:109397. doi: 10.1016/j.cbi.2021.109397
232. Macêdo dos Santos DC, Cavalcanti IDL, de Souza JB, Nogueira MCdBL, Cavalcanti IMF. Nanotechnology and tuberculosis: an old disease with new treatment strategies. Tuberculosis. (2022) 135:102208. doi: 10.1016/j.tube.2022.102208
233. Ahmed S, Raqib R, Guð*mundsson GH, Bergman P, Agerberth B, Rekha RS. Host-directed therapy as a novel treatment strategy to overcome tuberculosis: targeting immune modulation. Antibiotics. (2020) 9:21. doi: 10.3390/antibiotics9010021
234. Ouyang X, Li X, Li Z, Song N. Mycobacteriophages in diagnosis and alternative treatment of mycobacterial infections. Front Microbiol. (2023) 14:1277178. doi: 10.3389/fmicb.2023.1277178
235. Hatfull GF. Exploring the possibilities of bacteriophages for tuberculosis. (2023). Available at: https://www.openaccessgovernment.org/article/possibilities-bacteriophages-tuberculosis-tb/159096/ (accessed March 11, 2024).
236. Kelishomi Zeynali F, Khanjani S, Fardsanei F, Saghi Sarabi H, Nikkhahi F, Dehghani B. Bacteriophages of Mycobacterium tuberculosis, their diversity, and potential therapeutic uses: a review. BMC Infect Dis. (2022) 22:957. doi: 10.1186/s12879-022-07944-9
238. Hatfull GF. Mycobacteriophages: from petri dish to patient. PLoS Pathog. (2022) 18:e1010602. doi: 10.1371/journal.ppat.1010602
239. Diacon AH, Guerrero-Bustamante CA, Rosenkranz B, Rubio Pomar FJ, Vanker N, Hatfull GF. Mycobacteriophages to treat tuberculosis: dream or delusion? Respiration. (2022) 101:1–15. doi: 10.1159/000519870
240. Azimi T, Mosadegh M, Nasiri MJ, Sabour S, Karimaei S, Nasser A. Phage therapy as a renewed therapeutic approach to mycobacterial infections: a comprehensive review. Infect Drug Resist. (2019) 294–59. doi: 10.2147/IDR.S218638
241. Carrigy NB, Larsen SE, Reese V, Pecor T, Harrison M, Kuehl PJ, et al. Prophylaxis of Mycobacterium tuberculosis H37Rv infection in a preclinical mouse model via inhalation of nebulized bacteriophage D29. Antimicrob Agents Chemother. (2019) 63:10.1128/aac. 00871-19. doi: 10.1128/AAC.00871-19
Keywords: tuberculosis, challenges, opportunities, elimination, SDG
Citation: Gilmour B and Alene KA (2024) Ending tuberculosis: challenges and opportunities. Front. Tuberc. 2:1487518. doi: 10.3389/ftubr.2024.1487518
Received: 28 August 2024; Accepted: 02 December 2024;
Published: 24 December 2024.
Edited by:
Fraser Wares, KNCV TB Foundation, NetherlandsReviewed by:
Peter Kerndt, United States Agency for International Development, United StatesCopyright © 2024 Gilmour and Alene. This is an open-access article distributed under the terms of the Creative Commons Attribution License (CC BY). The use, distribution or reproduction in other forums is permitted, provided the original author(s) and the copyright owner(s) are credited and that the original publication in this journal is cited, in accordance with accepted academic practice. No use, distribution or reproduction is permitted which does not comply with these terms.
*Correspondence: Beth Gilmour, YmV0aC5naWxtb3VyQGN1cnRpbi5lZHUuYXU=
Disclaimer: All claims expressed in this article are solely those of the authors and do not necessarily represent those of their affiliated organizations, or those of the publisher, the editors and the reviewers. Any product that may be evaluated in this article or claim that may be made by its manufacturer is not guaranteed or endorsed by the publisher.
Research integrity at Frontiers
Learn more about the work of our research integrity team to safeguard the quality of each article we publish.