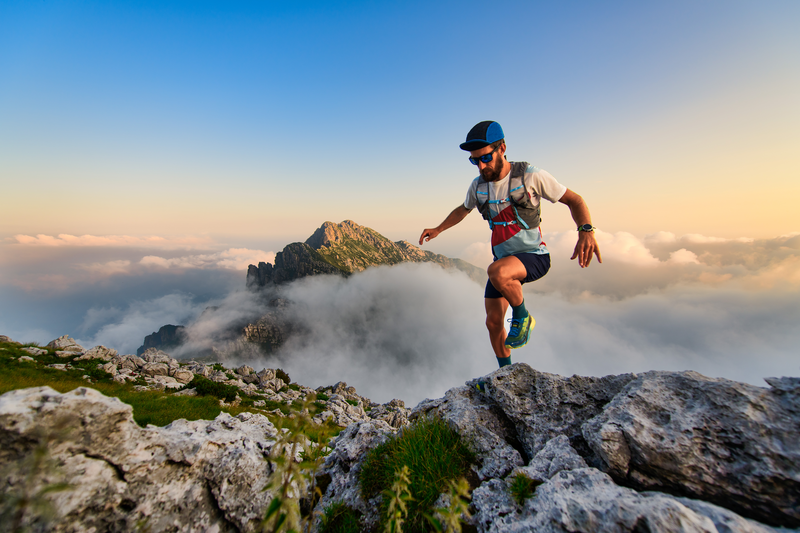
94% of researchers rate our articles as excellent or good
Learn more about the work of our research integrity team to safeguard the quality of each article we publish.
Find out more
ORIGINAL RESEARCH article
Front. Trop. Dis.
Sec. Emerging Tropical Diseases
Volume 6 - 2025 | doi: 10.3389/fitd.2025.1565116
The final, formatted version of the article will be published soon.
You have multiple emails registered with Frontiers:
Please enter your email address:
If you already have an account, please login
You don't have a Frontiers account ? You can register here
We report that Aedes albopictus Paris collected in June 2023 were experimentally able to transmit at least three dengue virus (DENV) serotypes, from 14 days post-infection (dpi) for DENV-3 and DENV-4, and from 21 dpi for DENV-2. Given the growing number of dengue autochthonous cases reported in France, episodes of active transmission in Paris are expected as it was reported in 2023 in the greater Paris area.
Keywords: Aedes albopictus, vector competence, Dengue Virus, France, Risk Assessment
Received: 22 Jan 2025; Accepted: 06 Mar 2025.
Copyright: © 2025 BOHERS, VAZEILLE, PASCALIN, MEIGNAN, KARCH, DE LAMBALLERIE and FAILLOUX. This is an open-access article distributed under the terms of the Creative Commons Attribution License (CC BY). The use, distribution or reproduction in other forums is permitted, provided the original author(s) or licensor are credited and that the original publication in this journal is cited, in accordance with accepted academic practice. No use, distribution or reproduction is permitted which does not comply with these terms.
* Correspondence:
Anna-Bella FAILLOUX, Institut Pasteur, Paris, 75015, Île-de-France, France
Disclaimer: All claims expressed in this article are solely those of the authors and do not necessarily represent those of their affiliated organizations, or those of the publisher, the editors and the reviewers. Any product that may be evaluated in this article or claim that may be made by its manufacturer is not guaranteed or endorsed by the publisher.
Research integrity at Frontiers
Learn more about the work of our research integrity team to safeguard the quality of each article we publish.