- 1Department of Geography, University of Namur, Namur, Belgium
- 2Institute of Life, Earth, and Environment (ILEE), University of Namur, Namur, Belgium
- 3Namur Research Institute for Life Sciences (NARILIS), University of Namur, Namur, Belgium
- 4Jomo Kenyatta University of Agriculture and Technology, Nairobi, Kenya
- 5Department of Public Health, Institute of Tropical Medicine, Antwerp, Belgium
- 6Population & Health Impact Surveillance Group, Kenya Medical Research Institute-Wellcome Trust Research Programme, Nairobi, Kenya
- 7Centre for Health Informatics, Computing, and Statistics, Lancaster Medical School, Lancaster University, Lancaster, United Kingdom
- 8Centre for Tropical Medicine and Global Health, Nuffield Department of Medicine, University of Oxford, Oxford, United Kingdom
Background: Growing concerns about mosquito-borne diseases (MBDs) in urban settings have prompted renewed urgency for collaborative and active mosquito surveillance programs. This literature review collates entomological data from five East African Community (EAC) countries, studying the definitions of urban settings, co-occurrence of Anopheles, Aedes and Culex mosquito genera and their infection rates to provide a wholistic understanding of MBDs in urban EAC.
Methods: A literature search following the PRISMA-ScR guidelines was conducted in EMBASE, MEDLINE, PubMed, Web of Science and Scopus databases to identify entomological studies conducted in urban and peri-urban settings since 2000. Data on urban and peri-urban settings definitions, presence records and infection rates of Aedes, Culex and Anopheles mosquitoes were compiled and presented.
Results: A hundred and five articles were included in the review. Diverse definitions of urban landscapes emerged, emphasizing (i) distinct characteristics for dichotomous delineation from rural settings (e.g., population density) and (ii) urban-peri-urban continuum integrating spatial variations in urban features associated with MBDs (e.g., planned/unplanned neighbourhoods). Mosquito ecology data derived from 88 sites, comprising 45 urban, 25 peri-urban, 14 mixed settings and four slums, reported 91 mosquito species, including 32% Culex, 31% Aedes, and 25% Anopheles genera. Other co-occurring genera, such as Coquillettidia and Mansonia, represented 12% between 2000 and 2024. Intricate co-occurrence patterns among Aedes, Culex and Anopheles genera linked to habitat preference and climatic conditions (temperature and precipitation) were observed. The average infection rates were estimated as 0.8% for yellow fever, 0.9% and 1.1% for urban and peri-urban P. falciparum sporozoites respectfully, 2.7% for Dengue, 5.3% for Chikungunya and 6.02% for flavivirus, indicating co-circulation of arboviruses among mosquito population.
Conclusion: Our synthesis presents an overview of the complexities of urban MBD research over the past two decades. Integrated assessment of MBDs dynamics in rapidly evolving urban environments is crucial to achieving healthier urban environments in East Africa.
Systematic review registration: https://osf.io/a6s9j/.
Highlights
What is already known?
● Despite the increasing public health concerns of mosquito-borne diseases (MBDs) such as spread of arboviruses by mosquitoes adapted to urban environments in East Africa, a comprehensive review of the classification of ‘urban’ settings in MBDs research to inform adequate characterization of MBDs in urban settings for comparable intra-urban risk analyses and assessments of co-occurrence of mosquito genera (Aedes, Anopheles, and Culex) does not exist.
What are the new findings?
● Our literature synthesis consolidates context-specific characteristics utilized in urban MBD research within East Africa to define urban and peri-urban settings providing a basis of meaningful intra-urban differences in MBD.
● We showed the intricate variations in mosquito genera co-occurrence dynamics that highlight distinct Aedes, Anopheles, and Culex mosquito distribution patterns within urban landscapes and co-circulation of MBD infection rates.
● Critical geographical research gaps especially in Burundi, Rwanda, and Uganda in addition to entomological surveillance research gaps remain due to the limited assessments of the role Culex mosquitoes in urban MBD transmission dynamics.
What do the new findings imply?
● A pressing need for nuanced classification of ‘urban’ environments in MBD research to support effective intra-urban intervention strategies.
● Implementation of joint-mosquito genera surveillance, adaptive research methodologies including the application of robust entomological surveillance approaches such as citizen/community-based science and interdisciplinary approaches are essential to strengthen MBD surveillance in the rapidly evolving EA urban setting.
Background
Mosquito-borne diseases (MBDs) pose significant public health burden globally, with urbanization emerging as a critical factor in shaping disease dynamics (1–3). Urbanization, broadly defined as the process that involves more people moving to and living in urban areas (cities and towns) results in social, economic, and infrastructural alterations (4, 5) whose interplay with environmental changes such as climate change poses substantial and often complex impacts on various MBDs (6, 7). For example, urban centres, though pivotal for economic growth, often exacerbate poverty and inequality, particularly in developing countries resulting in the proliferation of overcrowded slums with poor infrastructure and inadequate public health services (8, 9). Further, the ecological disturbances produced by anthropogenic landscape transformation processes during urbanization including poor waste management, insufficient drainage systems, unplanned construction sites and reinforcement of environmental stressors such as urban heat islands can potentially enhance the proliferation of mosquito species, causing epidemics linked to substantial human morbidity and mortality (6, 10, 11). Current evidence indicates an increasing spread of arboviruses such as dengue, chikungunya, yellow fever, and Zika virus, transmitted by mosquito species adapted to urban environments (12, 13). Notably, more than 3.5 billion people are at risk of dengue virus infection in over 100 countries with approximately 390 million infections every year, of which 100 million cause clinical symptoms (14), while outbreaks of chikungunya occur frequently worldwide with the number of cases reaching hundreds of thousands (15). Simultaneously, several studies assert the increasing burden of urban malaria globally with major shifts expected in temperate regions (16–19). However, considerable uncertainty in urban malaria estimates in Sub-Saharan Africa, one of the most malaria-endemic region persist, with one study estimating a 6-28% contribution to the annual disease incidence (20), while another study provides a lower estimate (17). The discrepancy in the observed estimates is likely in part due to inconsistent definitions of ‘urban’ used in malaria epidemiology.
The East African Community (EAC) region is particularly vulnerable to MBDs due to its ecological, socioeconomic and topographically diverse tropical/subtropical microclimates which harbour a large diversity of mosquito species of public health importance (21–26). The region is significantly impacted by diseases transmitted by three major mosquito genera: Anopheles, Aedes and Culex. The Anopheles mosquitoes, primarily the Anopheles gambiae sensu lato (s.l.) (Anopheles gambiae and Anopheles arabiensis) which recently display adaptation pattern to breeding in polluted waters in urban cities and human-made habitats including artificial containers such as water tanks and tyre tracks (27) are efficient vectors for malaria transmission, one of the leading causes of morbidity and mortality in the region (19, 28). Aedes mosquitoes, particularly Aedes aegypti are vectors for emerging threats such as dengue fever, chikungunya, yellow fever, and Zika virus associated with several outbreaks reported in the last decade in EAC urban settings (29) while Culex mosquitoes, such as Culex quinquefasciatus, are known vectors of lymphatic filariasis and play a probable role in chikungunya virus transmission (30). The overlapping mosquito species and transmission cycles pose significant public health challenges and highlight the importance of understanding the co-occurrence of mosquito species in urban EAC. However, previous studies primarily focused on a singular mosquito genus, with the most studied genera being either the Anopheles or Aedes mosquitoes (16, 24, 31, 32), neglecting the wholistic urban context that shapes transmission patterns of multiple MBDs. Further, the recent detection and spread of invasive mosquito species, particularly Anopheles stephensi in Kenya and Sudan (33–35) and Aedes albopictus in Tanzania (36) that thrive in urban environments, can potentially lead to a resurgence and increased disease burden despite previous MBD control efforts in these countries (37, 38). Importantly, rapid urbanization may significantly increase the population at risk within urban EAC, raising concerns about urban MBDs. The region consists of heterogeneous urban settings encompassing sprawling metropolitan cities, peri-urban areas, and small towns and despite being predominantly rural with approximately 132 million rural residents, it experienced the fastest urban growth rates in the last decade (>4%), adding 15 million new urban residents (39, 40). Compounding this threat is the potential for further dispersal of these mosquito species and their associated pathogens to new territories within EAC facilitated by a shared regional passport, enabling freedom of transport, trade, and tourism (31, 35, 41) if proper mitigation strategies are not implemented.
Recent efforts to address these complex dynamics of MBDs in EAC have emphasized urban-specific entomological surveillance and cross-country collaborative initiatives, fostering the exchange of entomological data and insights across national boundaries to identify shared challenges and emerging threats in the region (42–44). However, existing platforms, such as the East African Integrated Vector Management Centre of Excellence (45), that aim to compile regional entomological surveillance data continue to face challenges including inconsistent definitions of ‘urban’ environments, unstandardized surveillance methodologies that limit direct comparisons, resource disparities across countries, and rapidly changing urban landscapes that complicate mosquito population tracking. These setbacks underscore the need to strengthen urban surveillance systems across the region. A comprehensive review is essential to identify research gaps in understudied urban areas where MBD risks may be underestimated. By compiling data from individual studies, the review highlights geographical and methodological disparities that obstruct a full understanding of mosquito dynamics in urban EAC, ultimately guiding more effective and standardized urban surveillance strategies.
In this context, we conducted a comprehensive scoping review to provide evidence on the distributions and the transmission indices of all prevalent mosquito populations associated with human disease in the urban EAC region from 2000 to 2024. Specifically, we: (i) evaluate the definition of ‘urban’ typology within the context of MBD studies in EAC, (ii) assess the urban/peri-urban distribution and co-occurrence of mosquito species with a focus on Anopheles, Aedes and Culex genera and, (iii) examine mosquito infection rates to provide an overview of the variations in entomological risk of MBD transmission in urban EAC. Findings from this review are useful for informing the formulation of joint mosquito surveillance and control programs for future MBD epidemics in urban areas across the EAC.
Methods
Review protocol and registration
A review protocol was developed and registered with the Open Scientific Framework registry (https://osf.io/a6s9j/), to ensure transparency.
Literature search
A scoping literature review was conducted following the PRISMA extension for scoping reviews (PRISMA-ScR) guidelines (46–48), incorporating the methodology framework outlined by Arksey and O’Malley (49) and subsequently refined by Levac et al. (50). Searches were performed in five electronic databases that index peer-reviewed literature: EMBASE, MEDLINE, PubMed, Web of Science and Scopus, ensuring the reliability and credibility of our findings from sources that undergo rigorous evaluation by experts. However, we acknowledge potential biases, including publication bias and geographical gaps, which may limit the generalizability of our findings to the entire EAC. Consequently, in this review we focused on five EAC member states namely: Kenya, Tanzania, Uganda (joined EAC in 2000) and, Rwanda, Burundi (joined in 2007) (51), with robust data and more homogeneous ecological and climatic conditions to provide insights into disease transmission patterns and control strategies relevant to the majority of the EAC member states (52–56).
The literature searches used relevant terminologies related to MBDs transmitted by mosquito species of public health importance in urban EAC as outlined in the introduction section, the respective disease-transmitting mosquito genus, mosquito infection measures and the names of the EAC countries (Table 1). The databases were searched for studies published from 1st January 2000 to 13th July 2024. The year 2000 was chosen as a starting point for our search to reflect the timing of increased interest in mosquito urban research on the potential public health impact of mosquito-borne diseases in urban settings in the EAC region (57, 58). A language restriction was applied to include articles only written in English and French. Full search strategies used for each database are provided in the review protocol (https://osf.io/a6s9j/).
Eligibility criteria
Studies were eligible for inclusion in the review if they met the following inclusion criteria: (i) articles reporting data published between 2000 and 2024 in EAC (Kenya, Uganda, Mainland Tanzania, Rwanda, and Burundi); (ii) articles that included information on any of the following outcomes of interest: the distribution, abundance, density and urban ecology adaptations of mosquito population, mosquito species composition, feeding and breeding preferences, and mosquito infection rates in urban and/or peri-urban settings; (iii) studies reporting information on mosquito species responsible for a subset of MBDs (Malaria, Dengue, Chikungunya, Yellow fever, Rift valley fever, Zika and Lymphatic filariasis) prevalent in urban EAC settings and (iv) articles published in English or French. We excluded studies that (i) reported entomological outcomes in rural settings; (ii) provided modelled entomological outcomes such as the suitability indices of mosquito habitats or other vector outcomes such as insecticide resistance and molecular or genetic data of vector species; (iii) reported infection rates (seroprevalences) on humans and animals and (iv) evaluated arbovirus vectors other than mosquitoes such as flies and ticks.
Literature screening procedures
All articles obtained from the searches were downloaded by one review author (NKJ) to EndNote 20 (Clarivate Analytics, PA, USA), and duplicates removed. After de-duplication, title- and-abstract screening and full-text screening were performed using the Rayyan systematic review open-source software (https://www.rayyan.ai/), that facilitates collaborative screening of articles while ensuring double-blinded article selection, reducing article selection bias. In addition, the software enables logs of reasons for exclusion and lists disagreements between reviewers. This ensures transparency and helps solve review discrepancies efficiently (59).
Three authors (NKJ, EM, English articles and CM, French articles) independently screened the titles and abstracts of all articles to filter studies that met the basic eligibility criteria. Eligible studies were selected through a questionnaire that specified the inclusion criteria as outlined in the review protocol (https://osf.io/a6s9j/). Two reviewers, NKJ and EM conducted full-text screening of the retained articles to ensure compliance with all inclusion criteria. Notably, at the full-text screening stage we reviewed the methods section describing the study sites to ascertain the urban/peri-urban focus of all the retrieved articles that may not be explicitly defined in the title and abstract. For example, when screening studies specifying a broader area, such as an administrative location or a major city as the study area in the title or abstract, we examined the methods section to identify if the sampled sites included were located within the vicinity of the city centre (urban strata), or peri-urban or rural settings. Citations of the included articles after full-text screening were manually screened to identify additional studies. Discrepancies concerning eligibility throughout the whole screening process were resolved through discussion among the reviewers.
Data extraction
All articles selected for inclusion were synthesised using a standardized Microsoft Excel spreadsheet data extraction form developed by NKJ and endorsed by other authors: PMM, CL and RWS. The Microsoft Excel form included the following data categories: study details (authorship, year of publication, study country, study location, geographical coordinates of sampled sites, study period), urbanicity (urban/peri-urban, key urban/peri urban characteristics linked to mosquito population), entomological surveillance metadata (mosquito collection techniques, species identification, indoor and/or outdoor sampling, mosquito life stage collected), mosquito-borne disease of study focus, transmission indices (disease-specific infection rates among mosquito populations sampled and human biting/blood meal rates), vector species involved in the transmission, vector abundance/density and vector bionomics (biting, feeding, and breeding preferences). Data extraction was limited to the information provided in the published work; authors were not contacted for further clarification or provision of missing information. NKJ extracted and recorded data manually to the Microsoft excel form including each listed variable for all selected articles and a second review author (EM), validated 20% of the extracted data. Finally, a geo-positioning entry matching the sampled sites of the selected studies was done by EM using electronic sources such as Google Earth to obtain the geographical coordinates of sampled sites when not provided.
Data analysis
Data extracted from the selected articles were thematically categorized to facilitate analysis while accounting for heterogeneity in various studies in terms of study scope and outcomes of interest. The thematic categories used included: i) ecological studies describing the different mosquito genera distributions and species compositions in urban/peri-urban study sites), ii) descriptive (studies providing the bionomic assessments of mosquito population such as mosquito genera co-occurrence, adaptations and preferred breeding sites), iii) transmission measures (studies reporting disease specific mosquito infectivity rates in urban/peri-urban contexts in EAC and iv) multiple scope category (consisted at least two categories previously mentioned).
Empirical data on disease-specific infection rates were pre-processed to obtain summaries following entomological adjustments from previous research (16) that account for variations in sampling methods (e.g., indoor/outdoor surveillance) and frequency of surveillance used in the studies reviewed. The following adjustments were applied: i) when multiple disease-specific infection rates were reported for the same site (infection rates corresponding to sub-locations within an urban/peri-urban region) at a particular point in time, the estimates were averaged; ii) when both indoor and outdoor disease-specific infection rates were reported, the rates were averaged iii) when data was available for two different seasons the infection rates corresponding to peak transmission seasons were considered and, iv) when data are available for different points in time but same season, the most recent rate was reported.
Overall, we summarized and presented data related to ‘urban’ definitions used in each study drawing converging and divergent definitions, a tally of the mosquito genera and species composition reported in each study site, the mosquito surveillance methods used, and the average disease infection rates in mosquito population using tables or figures to provide an overview of the urban typology in MBD research and mosquito population in EAC’s urban contexts. Summary tables and figures were produced using R version (4.3.2), and maps were produced using ArcGIS Pro version (3.1).
Results
Description of included studies
The search strategy yielded a total of 12,241 articles, with 5,577 articles remaining after removal of duplicate studies. The articles’ titles and abstracts were screened, and irrelevant studies excluded, resulting in 212 studies that were eligible for full-text screening. Two full texts were not accessible and hence excluded. A full-text screening of 210 articles was conducted and 108 articles with irrelevant information excluded, while 102 articles met the inclusion criteria. An additional three relevant articles were identified and included from the reference list of selected articles. Ultimately, a total of 105 articles were synthesised in the scoping review (Supplementary File 1). A PRISMA flowchart summarizing the stages of the screening process is presented Figure 1.
Most of the studies were conducted in Kenya (n=61; 58%) followed by Tanzania (n=31; 30%), Uganda (n=10, 10%) and Rwanda (n=3; 3%) (Supplementary File 2: Figure 1A). No studies were identified from urban Burundi. The thematic categories of articles included were distributed as follows: descriptive studies on mosquito genera bionomics, co-occurrence, and adaptation (n=51; 49%), transmission measures of disease-specific mosquito infection rates (n=23; 22%) and ecological studies highlighting mosquito genera distribution and species composition across countries (n=16; 15%), while 15 (14%) articles tackled multiple scopes of indicators of interest of this review (Supplementary File 2: Figure 1B).
Dynamic definitions of urban settings for MBD studies in EAC
Data presented were derived from 88 unique study sites conducted in urban (n=45), peri-urban (n=25), mixed (i.e., urban, and peri-urban) (n=14) and urban informal settlement (n=4) based on the authors’ descriptions across EAC. Among all the 88 unique sites, Kisumu city in Kenya had the most studies (n=23), followed by Dar es salaam in Tanzania (n=20) and Malindi town (n=14) in Kenya (Figure 2).
Varied definitions of urban/peri-urban settings emerged within the context of mosquito population research. The majority of studies, 89.5% (n=94) reviewed, relied on conventional definitions to define an ‘urban’-rural dichotomy based on various metrics, including population counts or density thresholds, administrative definitions (e.g., capital city of a country), functional characteristics (e.g., type of economic activity such as the extent of trade and agriculture in a specific geographical area) and economic development indicators (e.g., type of infrastructure such as road networks). In contrast, 11 studies conducted in Tanzania (Dar es Salaam and Ifakara: n=4) and Kenya (Malindi, Ukunda and Kisumu; n=7) applied an urban-gradient definition indicating spatial variations of context-specific factors associated with mosquito population to distinguish urban centres and peri-urban areas within a city, shifting from the ‘urban’-rural dichotomous definition to an urban-peri-urban continuum. For example, urban areas were typically described based on development dynamics exhibiting higher population densities, intra-urban connectivity based on transport routes, and improved housing conditions, while peri-urban areas often demonstrated intermediate characteristics between urban and rural environments with varying levels of housing infrastructure development often sheltered by vegetation or agricultural land. Conversely, suburbs/informal settlements were characterized as rapidly expanding unplanned neighbourhoods with poor housing and socioeconomic factors such as unreliable water supply (water storage), unreliable sewer and waste management systems. In addition, topographical factors influencing the types of mosquito habitat proximity (natural habitats such as river valleys, lakes and swamps and artificial habitats such as flood plains) was highlighted as important site characteristic across all urban typologies in MBD research (Supplementary File 2: Table 1).
Mosquito surveillance in urban EAC
Mosquito surveillance methods varied widely based on the different study designs tailored to account for the heterogeneity of each study location (Table 2). Most of the studies, 45% (n=49) focused on outdoor mosquito collections followed by a substantial portion targeting both indoor and outdoor collections 41% (n=40), with a smaller proportion concentrating solely on indoor collections 14% (n=12). Regarding mosquito life stage, adult mosquitoes were the predominant target, with 47% (n=44) of studies focusing exclusively on this stage, 37% (n=34) targeting immature stages (reared into adults), and 13% (n=15) addressing both life stages. Various mosquito capture methods were used, with the CDC-light traps used in 23%(n=24) studies, followed by human landing catches in 20%(n=21) for adult mosquito catches while larval dipping 76% (n=35) was the most common method for mosquito immature collections. Morphological methods were the most preferred methods for genus identification utilized in 61%(n=41) studies, while molecular (e.g., Polymerase Chain Reaction) or a combination of molecular and morphological identification methods were used in the remaining studies 39%(n=26).
Among the studies reviewed, 11%(n=10) compared mosquito collection methods in urban EAC (Supplementary File 2: Table 1). Nine of the ten studies assessed Anopheles species collection methods focusing mainly on An. gambiae s.l while one study evaluated the performance of three gravid traps [Sticky ovitraps (SO), MosquiTraps (MQT) and the CDC Gravid trap (CDC-GT)] to capture culex and aedes mosquitoes. These studies reported varied efficacy of various mosquito collection methods based on factors such as mosquito species diversity, habitat and biting preferences, and geographical context. For example, mosquito electrocuting grid traps, odour-baited CDC light traps and the Ifakara Tent Trap (ITT) were reported as sensitive tools to quantify epidemiologically relevant metrics such as mosquito biting densities, behaviours and human exposure distribution for An. gambiae s.l (An. Arabiensis, An. gambiae s.s. and An. Merus) that were statistically indistinguishable compared to Human landing catch (HLC) and BG-Sentinel traps. In addition, gravid traps were reported to be significantly efficient for Ae. aegypti and Cx. Quinquefasciatus collection (Supplementary File 2: Table 2).
Composition & co-occurrence patterns of mosquito genera
Collectively, 91 mosquito species were reported across urban and peri-urban EAC settings in studies published between the years 2000-2024. Species in the Culex, Aedes and Anopheles genera constituted 33% (n=30), 30% (n=27), 25%(n=23), respectively while others reported alongside the study focus genera were Coquillettidia (4%; n=4), Mansonia (2%; n=2) and other genera such as Lutzia, Eretmapodites, Toxorhynchites, Ficalbia (5%; n=5) (Figure 3A). The diversity of the mosquito species in the Aedes and Culex genera was greater in urban settings compared to peri-urban settings, while the diversity of mosquito species of the Anopheles genus was greater in peri-urban areas compared to urban settings (Figure 3B).
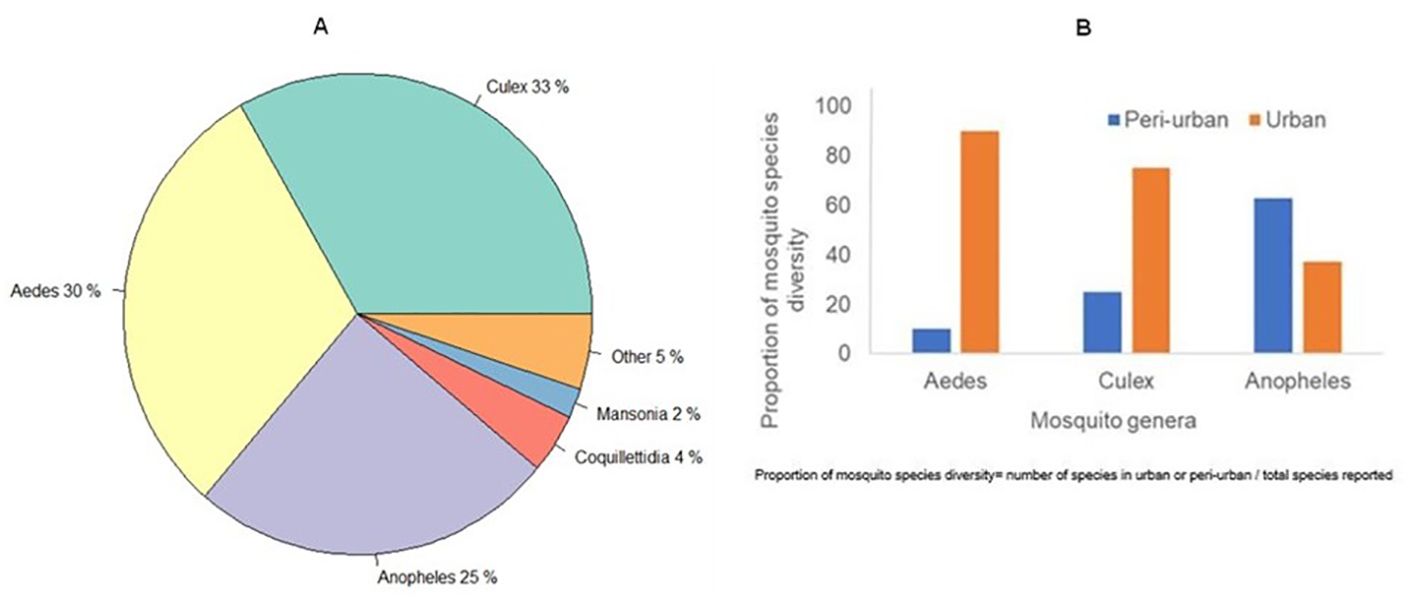
Figure 3. Mosquito population distribution. (A) Mosquito genera composition in urban EAC. (B) Mosquito species diversity by urban class in EAC.
Within the Anopheles gambiae complex, An. gambiae sensu stricto and An. Arabiensis were prevalent in both urban and peri-urban settings. However, An. merus was predominantly found in urban areas, indicating a potential adaptation to urban environments. In addition, within the Aedes and Culex genera, Ae. aegypti and Cx.pipiens s.l especially Cx. quinquefasciatus were common in both urban and peri-urban settings (Supplementary File 2: Figure 2). Seven studies highlighted varied adaptations of existing species to urban settings including changes in blood-seeking activities (biting), preference to outdoor breeding and species composition (Supplementary File 2: Table 3). Of the 105 studies analysed, 44% (n=41) investigated factors associated to the co-occurrence of multiple mosquito genera in urban environments. Preference of breeding habitats and climatic variations (temperature and precipitation) were highlighted as contributing factors to the co-existence of Aedes, Anopheles and Culex mosquitoes. Aedes and Culex mosquitoes were commonly found to coexist in urban environments rich in artificial containers, small water bodies and discarded receptacles while Anopheles and Culex mosquitoes often overlap, predominantly in natural settings characterized by stagnant ponds and vegetated areas within urban landscapes (Supplementary File 2: Table 4).
Mosquito infection rates
Thirty-two studies isolated pathogens from collected mosquitoes to assess the infection rates of varied MBDs prevalent in humans. Twenty-seven studies reported infection rates in field-caught mosquitoes while five studies (excluded from the infection rates analysis) were semi-field experimental assessments of vector competency for transmission. Studies to incriminate malaria vectors by detection of Plasmodium falciparum sporozoites were spread across the study period while those of other MBDs of interest were published post 2015 in the urban EAC. Consequently, many of the studies (n=18) evaluated P. falciparum sporozoites linked to malaria infections while both Dengue and Chikungunya virus isolates were reported in six and seven studies respectively. Other arboviruses namely Yellow Fever (n=5), Rift Valley Fever (n=1) and Zika viruses (n=1) were also reported in the EAC region for years post-2015. Three studies conducted in Kenya reported positive flavivirus isolates representing potential for co-infection of arboviruses in field-caught mosquitoes (Supplementary File 2: Table 5).
The average infection rates in field-caught mosquito populations varied, ranging from 0.8% for Yellow Fever virus to 5.3% for Chikungunya virus (Figure 4). Only studies on P. falciparum sporozoites infections evaluated infection rates in both urban and peri-urban contexts with no significant differences. Urban strata reported P. falciparum infection rates (n=4) ranging from 0.46% in 2009 to 1.14% in 2016 in Dar es salaam, Tanzania, while peri-urban strata reported infection rates (n=11) ranging from 0.02% in Jinja, Uganda to 3.92% in Kakamega, Kenya.
Extreme values were reported for Zika virus (67.1%) in Entebbe, Uganda, flavivirus (11.7%) and Rift valley fever virus (22%) in Sogan-Godud town in Kenya in studies that considered both domestic and zoonotic forest forms (Aedes) of mosquito vectors found in forests/national parks within the periphery of urban settings (Supplementary File 2: Table 5). However, these extreme values were likely a result of testing small mosquito samples for pathogens, which may not accurately represent the broader mosquito population and its infection rates.
Discussion
Research on the multifaceted nature of MBDs in urban landscapes is essential for developing targeted and effective intervention strategies, particularly in an era witnessing rapid urban growth (60–65). The growing diversity of mosquito species and pathogens in urban settings, combined with gaps in the definition of ‘urban’ and the limited understanding of mosquito species co-occurrence, poses significant challenges in managing the trajectories of urban MBDs in EAC. The current literature synthesis provides insights into the characterization of urban/peri-urban settings, co-occurrence of multiple mosquito genera (Aedes, Culex and Anopheles), and the infection rates of mosquito population across urban EAC.
Most of the studies included (n=94) use urban-rural dichotomy definitions based on various socio-economic and demographic metrics, reflecting the historical perception of ‘urban’ areas in EAC having better health outcomes for MBDs compared to rural areas, attributed to disparities in determinants of health such as adequate infrastructure and improved access to healthcare services (17, 66–69). However, there is a growing interest in incorporating multi-dimensional urban definitions that account for shifts in urban gradients to reveal disparities in mosquito genera population within urban areas. For example, the divergences highlighted in defining urban landscapes based on urbanization dynamics (rapid unplanned urban growth), limited access to water and sanitation amenities and poor housing conditions signify a potential focal point for MBD control strategy in suburbs/informal settlements within major cities. In addition, several studies (n=54; 51%) provided geographical coordinates of the study sites, suggesting an increasing scientific interest of precise mapping, critical in identifying mosquito habitats, disease hotspots and spatial distribution of vectors in urban EAC. Acknowledging and quantifying the complexities of intra-urban typologies is crucial for addressing emerging MBD challenges in EAC to account for the diminishing or even reversal of the urban advantage associated with rapid urbanization often characterized by poor planning and informal settlements that have significant public-health implications (66, 68, 70).
The co-existence of various mosquito genera, Aedes, Culex and Anopheles, marked by the presence of diverse indigenous species was reported in most studies in urban EAC. Aedes and Culex genera exhibited the highest species diversity overall, particularly in Kenya where the literature on urban MBD ecology is more extensive, spanning varied urban settings to include large cities and upcoming town centres. These studies also suggest predominance of Ae. aegypti, An. gambiae complex and cx. pipiens s.l, in urban settings while An. gambiae complex, An. funestus and cx. pipiens s.l. were commonly reported in peri-urban settings in EAC. The overlapping habitat usage in different urban typologies increases the potential for co-infection and makes vector control more complex (24, 32). However, adequate characterization of mosquito species composition, habitat preferences, and human-vector interactions, may vary depending on the effectiveness of sampling design used. Studies that integrated multiple surveillance methods and both indoor and outdoor mosquito collections provided extensive insights into mosquito abundance, genera co-occurrence, species diversity, and mosquito behaviour within urban landscapes compared to those using singular surveillance methods. However, there is a need for further research to address the current data limitations in assessing key aspects of mosquito bioecology such as such as endophilic versus exophilic tendencies, indoor and outdoor feeding behaviours, and preferred breeding habitats. Such research is essential for understanding the transmission dynamics of multiple in urban EAC. In addition, future entomological studies in urban EAC could benefit from prioritizing multi-dimensional and cost-effective research methodologies such as citizen science, community engagement and interdisciplinary approaches to understand better and mitigate urban mosquito challenges such as the emergence and spread of invasive species such as An. stephensi and Ae. albopictus, that pose new challenges to disease control and public health efforts in EAC (34, 36, 71).
The literature also highlights varied mosquito infection rates with substantial uncertainty across studies in urban EAC. Notably, the mosquito infection rates recorded were consistently low in urban EAC settings and are likely linked to small mosquito capture and varying methodologies used across studies. This uncertainty complicates efforts to draw meaningful conclusions on the potential impacts of urbanization on MBDs in EAC. Various studies reported the need for robust entomological surveillance such as citizen/community-based science may strengthen entomological surveillance to inform mosquito control efforts. In EAC, the urban malaria control program (UMCP) in Dar es Salaam, a rapidly urbanizing city in Tanzania integrates community-based mosquito surveillance to capture spatial and temporal trends of MBDs (42, 72, 73). Although, such initiatives are gaining importance in entomological surveillance, their benefits are limited by availability of cost-effective mosquito collection tools suitable for urban mosquitoes (74, 75). For example, 10 (11%) studies synthesized reported varied sensitivity of the mosquito collection methods used. Nonetheless, co-circulation of mosquito-borne pathogens, particularly Dengue and Chikungunya, were reported among the sampled mosquitoes, emphasizing the urgency of understanding the implications of mosquito species co-occurrence on MBD transmission dynamics in urban settings. Only two studies reported infection rates assessments of Culex mosquitoes (2 studies) (30, 76) in urban EAC while the infection rates of Aedes and Anopheles mosquitoes were extensively reported. This disparity underscores the need for research focusing on Culex mosquitoes to ascertain their contribution to the spread of MBDs in urban EAC. In addition, while countries like Kenya and Tanzania have more comprehensive data and better surveillance systems, others, especially Burundi, Rwanda and Uganda are still lagging, resulting in an incomplete picture of MBD urban ecology in EAC.
Conclusion
This study offers a comprehensive overview of various knowledge gaps in mosquito ecology research conducted in urban and peri-urban settings across East African Community (EAC) countries over the past two decades. The diverse definitions of urban settings emphasize the need for targeted interventions considering the different urbanization stages or urban ecosystems. Collectively, the assessment on factors influencing mosquito genera co-occurrence and their infection rates present a crucial link to ecological niche modelling, providing predictive insights into mosquito distributions, disease transmission potential and adaptation to varying urban landscapes. The development of comprehensive surveillance and integrated control approaches are paramount to achieving healthier urban environments in East Africa.
Data availability statement
The original contributions presented in the study are included in the article/Supplementary Material. Further inquiries can be directed to the corresponding author.
Author contributions
NKJ: Conceptualization, Data curation, Formal analysis, Investigation, Methodology, Visualization, Writing – original draft, Writing – review & editing. EM: Data curation, Validation, Visualization, Writing – review & editing. CM: Formal analysis, Validation, Writing – review & editing. PMM: Writing – review & editing. RWS: Conceptualization, Writing – review & editing. CL: Conceptualization, Funding acquisition, Writing – review & editing.
Funding
The author(s) declare financial support was received for the research, authorship, and/or publication of this article. NKJ is supported by the F.R.S-FNRS, PDR (40008711). RWS is supported as a Wellcome Trust Principal Research Fellow (212176/Z/18/Z) and is grateful for the support from the Wellcome Trust to the Core Award for the East Africa Major Overseas Programme (203077/Z/16/Z). PMM is supported by the Institute of Tropical Medicine’s Departement Economie, Wetenschap en Innovatie (EWI). CM is a Research Fellow funded by the Fonds de la Recherche Scientifique (F.R.S.-FNRS).
Acknowledgments
We are grateful to Dr. Charlotte Beaudart from the Clinical Pharmacology and Toxicology Research Unit, University of Namur for providing guidance during the review protocol development and feedback on the search strategy, Steven Wambua of the University of Birmingham and Dr. Florence De Longueville of the University of Namur for facilitating access to databases, actively supporting, and enabling full article accessibility during the literature search.
Conflict of interest
The authors declare that the research was conducted in the absence of any commercial or financial relationships that could be construed as a potential conflict of interest.
Publisher’s note
All claims expressed in this article are solely those of the authors and do not necessarily represent those of their affiliated organizations, or those of the publisher, the editors and the reviewers. Any product that may be evaluated in this article, or claim that may be made by its manufacturer, is not guaranteed or endorsed by the publisher.
Author disclaimer
The views expressed in this publication are those of the authors and not necessarily those of the funders of the study. In addition, the funders had no role in study design, data collection, data analysis, data interpretation, or writing of the report.
Supplementary material
The Supplementary Material for this article can be found online at: https://www.frontiersin.org/articles/10.3389/fitd.2024.1499520/full#supplementary-material
References
1. Flückiger M, Ludwig M. Malaria suitability, urbanization and subnational development in sub-Saharan Africa. J Urban Econ. (2020) 120:103279. doi: 10.1016/j.jue.2020.103279
2. Hotez PJ. Global urbanization and the neglected tropical diseases. PLoS Negl Trop Dis. (2017). doi: 10.1371/journal.pntd.0005308
3. Fu G. Epidemiological patterns of mosquito-borne diseases globally. J Mosq Res. (2024) 14(3):111–23. doi: 10.5376/jmr.2024.14.0012
4. United Nations. World urbanization prospects the 2018 revision. New York: Department of Economic and Social Affairs (2019). Population Division.
5. Montgomery MR, Stren R, Cohen B, Reed HE. Cities transformed: demographic change and its implications in the developing world. Routledge (2013). doi: 10.4324/9781315065700
6. Wilke AB, Beier JC, Benelli G. Complexity of the relationship between global warming and urbanization–an obscure future for predicting increases in vector-borne infectious diseases. Curr Opin Insect sci. (2019) 35:1–9. doi: 10.1016/j.cois.2019.06.002
7. Robert MA, Stewart-Ibarra AM, Estallo EL. Climate change and viral emergence: evidence from Aedes-borne arboviruses. Curr Opin virol. (2020) 40:41–7. doi: 10.1016/j.coviro.2020.05.001
8. Chewe M, Hangoma P. Drivers of health in sub-Saharan Africa: a dynamic panel analysis. Health Policy Open. (2020) 1:100013. doi: 10.1016/j.hpopen.2020.100013
9. Zerbo A, Delgado RC, González PA. Vulnerability and everyday health risks of urban informal settlements in Sub-Saharan Africa. Global Health J. (2020) 4:46–50. doi: 10.1016/j.glohj.2020.04.003
10. Duval P, Antonelli P, Aschan-Leygonie C, Valiente Moro C. Impact of human activities on disease-spreading mosquitoes in urban areas. J Urban Health. (2023) 100: 591–611. doi: 10.1007/s11524-023-00732-z
11. LaDeau SL, Allan BF, Leisnham PT, Levy MZ. The ecological foundations of transmission potential and vector-borne disease in urban landscapes. Funct ecol. (2015) 29:889–901. doi: 10.1111/fec.2015.29.issue-7
12. Zerbo A, Delgado RC, González PA. Aedes-borne viral infections and risk of emergence/resurgence in Sub-Saharan African urban areas. J Biosafety Biosecurity. (2020) 2:58–63. doi: 10.1016/j.jobb.2020.10.002
13. Gould E, Pettersson J, Higgs S, Charrel R, De Lamballerie X. Emerging arboviruses: why today? One Health. (2017) 4:1–13. doi: 10.1016/j.onehlt.2017.06.001
14. Bhatt S, Gething PW, Brady OJ, Messina JP, Farlow AW, Moyes CL, et al. The global distribution and burden of dengue. Nature. (2013) 496:504–7. doi: 10.1038/nature12060
15. World Health Organization. Chikungunya 2024 . Available online at: https://www.who.int/health-topics/chikungunyatab=tab_1 (Accessed August 28, 2024).
16. Doumbe-Belisse P, Kopya E, Ngadjeu C, Sonhafouo-Chiana N, Talipouo A, Djamouko-Djonkam L, et al. Urban malaria in sub-Saharan Africa: dynamic of the vectorial system and the entomological inoculation rate. Malaria J. (2021) 20:1–18. doi: 10.1186/s12936-021-03891-z
17. Hay SI, Guerra CA, Tatem AJ, Atkinson PM, Snow RW. Urbanization, malaria transmission and disease burden in Africa. Nat Rev Microbiol. (2005) 3:81–90. doi: 10.1038/nrmicro1069
18. Wilson ML, Krogstad DJ, Arinaitwe E, Arevalo-Herrera M, Chery L, Ferreira MU, et al. Urban malaria: understanding its epidemiology, ecology, and transmission across seven diverse ICEMR network sites. Am J Trop Med Hyg. (2015) 93:110–23. doi: 10.4269/ajtmh.14-0834
19. World Health Organization. World malaria report 2023. World Health Organization (2023). https://www.who.int/publications/i/item/9789240086173.
20. Keiser J, Utzinger J, De Castro MC, Smith TA, Tanner M, Singer BH. Urbanization in sub-saharan Africa and implication for malaria control. The Intolerable Burden of Malaria II: What’s New, What’s Needed. Am J Trop Med Hygiene. (2004) 71(2 Suppl):118–27. doi: 10.4269/ajtmh.2004.71.118
21. Harbach RE. Mosquito taxonomic inventory. (2013). http://mosquito-taxonomicinventory.info/sites/mosquito-taxonomicinventory.info/files/Valid.2020;20 (Accessed [August 11, 2024]).
22. Karungu S, Atoni E, Ogalo J, Mwaliko C, Agwanda B, Yuan Z, et al. Mosquitoes of etiological concern in Kenya and possible control strategies. Insects. (2019) 10:173. doi: 10.3390/insects10060173
23. Mutebi J-P, Crabtree M, Kading R, Powers A, Lutwama J, Miller B. Mosquitoes of western Uganda. J Med entomol. (2014) 49:1289–306. doi: 10.1603/ME12111
24. Philbert A, Majalija S, Lyantagaye SL. Arbovirus Vectors of Epidemiological Concern in East Africa: A systematic review of entomological studies (1940-2020). Afr J Health Sci. (2022) 35:496–520. https://ojs.ajhsjournal.or.ke/index.php/home/article/view/260.
25. Wiebe A, Longbottom J, Gleave K, Shearer FM, Sinka ME, Massey NC, et al. Geographical distributions of African malaria vector sibling species and evidence for insecticide resistance. Malaria J. (2017) 16:1–10. doi: 10.1186/s12936-017-1734-y
26. Irish SR, Kyalo D, Snow RW, Coetzee M. Updated list of Anopheles species (Diptera: Culicidae) by country in the Afrotropical Region and associated islands. Zootaxa. (2020) 4747(3). doi: 10.11646/zootaxa.4747.3.1
27. Djamouko-Djonkam L, Mounchili-Ndam S, Kala-Chouakeu N, Nana-Ndjangwo SM, Kopya E, Sonhafouo-Chiana N, et al. Spatial distribution of Anopheles Gambiae sensu lato larvae in the urban environment of Yaoundé, Cameroon. Infect Dis Poverty. (2019) 8:1–15. doi: 10.1186/s40249-019-0597-6
28. World Health Organization. Malaria threat map Geneva 2022. Available online at: https://apps.who.int/malaria/maps/threats//stories?theme=invasive (Accessed August 22, 2024).
29. Dahmana H, Mediannikov O. Mosquito-borne diseases emergence/resurgence and how to effectively control it biologically. Pathogens. (2020) 9:310. doi: 10.3390/pathogens9040310
30. Lutomiah J, Mulwa F, Mutisya J, Koskei E, Langat S, Nyunja A, et al. Probable contribution of Culex quinquefasciatus mosquitoes to the circulation of chikungunya virus during an outbreak in Mombasa County, Kenya, 2017-2018. Parasit Vectors. (2021) 14:138. doi: 10.1186/s13071-021-04632-6
31. Nyaruaba R, Mwaliko C, Mwau M, Mousa S, Wei H. Arboviruses in the East African Community partner states: a review of medically important mosquito-borne Arboviruses. Pathog Global Health. (2019) 113:209–28. doi: 10.1080/20477724.2019.1678939
32. Eder M, Cortes F, Teixeira de Siqueira Filha N, Araújo de França GV, Degroote S, Braga C, et al. Scoping review on vector-borne diseases in urban areas: transmission dynamics, vectorial capacity and co-infection. Infect Dis poverty. (2018) 7:1–24. doi: 10.1186/s40249-018-0475-7
33. Ahmed A, Pignatelli P, Elaagip A, Hamid MMA, Alrahman OF, Weetman D. Invasive malaria vector Anopheles stephensi mosquitoes in Sudan, 2016–2018. Emerging Infect diseases. (2021) 27:2952. doi: 10.3201/eid2711.210040
34. Ochomo EO, Milanoi S, Abong’o B, Onyango B, Muchoki M, Omoke D, et al. Molecular surveillance leads to the first detection of Anopheles stephensi in Kenya. Emerg Infect Dis. (2023) 29(12):2498–508. doi: 10.21203/rs.3.rs-2498485/v1
35. Ogola FO, Njenga GN, Mhando PC, Kiggundu MN. A profile of the East African community. Afr J Manage. (2015) 1:333–64. doi: 10.1080/23322373.2015.1106719
36. Longbottom J, Walekhwa AW, Mwingira V, Kijanga O, Mramba F, Lord JS. Aedes albopictus invasion across Africa: the time is now for cross-country collaboration and control. Lancet Global Health. (2023) 11:e623–e8. doi: 10.1016/S2214-109X(23)00046-3
37. Emiru T, Getachew D, Murphy M, Sedda L, Ejigu LA, Bulto MG, et al. Evidence for a role of Anopheles stephensi in the spread of drug-and diagnosis-resistant malaria in Africa. Nat Med. (2023) 29:3203–11. doi: 10.1038/s41591-023-02641-9
38. Samarasekera U. A missed opportunity? Anopheles stephensi in Africa. Lancet. (2022) 400:1914–5. doi: 10.1016/S0140-6736(22)02483-7
39. Saghir J, Santoro J eds. Urbanization in Sub-Saharan Africa. Meeting Challenges by Bridging Stakeholders. Washington, DC, USA: Centre for Strategic & International Studies (2018).
40. World Bank. Sub-Saharan Africa; Urban Population Growth Rate. (2017). Available online at: https://data.worldbank.org/indicator/SP.URB.GROW?locations=ZG. Accessed (September, 05, 2024).
41. Sinka M, Pironon S, Massey N, Longbottom J, Hemingway J, Moyes C, et al. A new malaria vector in Africa: predicting the expansion range of Anopheles stephensi and identifying the urban populations at risk. Proc Natl Acad Sci. (2020) 117:24900–8. doi: 10.1073/pnas.2003976117
42. De Castro MC, Yamagata Y, Mtasiwa D, Tanner M, Utzinger J, Keiser J, et al. Integrated urban malaria control: a case study in Dar es Salaam, Tanzania. The Intolerable Burden of Malaria II: What’s New, What’s Needed. Am J Trop Med Hygiene. (2004) 71(2 Suppl):103–17. doi: 10.4269/ajtmh.2004.71.103
43. Impoinvil DE, Ahmad S, Troyo A, Keating J, Githeko AK, Mbogo CM, et al. Comparison of mosquito control programs in seven urban sites in Africa, the Middle East, and the Americas. Health Policy. (2007) 83:196–212. doi: 10.1016/j.healthpol.2007.01.009
44. Africa Centre for Disease Control and Prevention. Regional coordination centres 2024. Available online at: https://africacdc.org/regional-collaborating-centres/ (Accessed August 22, 2024).
45. Mboera L, Rumisha S, Senkoro K, Kamugisha M, Kitua A. East African Integrated Disease Surveillance Network. (2002). Report submitted to the Rockefeller Foundation.
46. Tricco AC, Lillie E, Zarin W, O’Brien KK, Colquhoun H, Levac D, et al. PRISMA extension for scoping reviews (PRISMA-ScR): checklist and explanation. Ann Internal Med. (2018) 169:467–73. doi: 10.7326/M18-0850
47. Peters MD, Godfrey CM, Khalil H, McInerney P, Parker D, Soares CB. Guidance for conducting systematic scoping reviews. JBI Evidence Implement. (2015) 13:141–6. doi: 10.1097/XEB.0000000000000050
48. Colquhoun HL, Levac D, O’Brien KK, Straus S, Tricco AC, Perrier L, et al. Scoping reviews: time for clarity in definition, methods, and reporting. J Clin Epidemiol. (2014) 67:1291–4. doi: 10.1016/j.jclinepi.2014.03.013
49. Arksey H, O’Malley L. Scoping studies: towards a methodological framework. Int J Soc Res methodol. (2005) 8:19–32. doi: 10.1080/1364557032000119616
50. Levac D, Colquhoun H, O’brien KK. Scoping studies: advancing the methodology. Implement sci. (2010) 5:1–9. doi: 10.1186/1748-5908-5-69
51. East Africa Community. East African Community (EAC) . Available online at: https://au.int/en/recs/eac:~:text=The%20East%20African%20Community%20(EAC,its%20headquarters%20in%20Arusha%2C%20Tanzania (Accessed October 15, 2024).
52. Mbanzulu KM, Mboera LE, Luzolo FK, Wumba R, Misinzo G, Kimera SI. Mosquito-borne viral diseases in the Democratic Republic of the Congo: a review. Parasites Vectors. (2020) 13:1–11. doi: 10.1186/s13071-020-3985-7
53. Mio J, Mohamed M, Osman Y, Abukar M, Karama N, Nurani O. Mapping of main mosquitoes in Mogadishu-Somalia. Indiana J Agric Life Sci. (2022) 2:8–19. doi: 10.5281/zenodo.7032146
54. Panzi EK, Okenge LN, Kabali EH, Tshimungu F, Dilu AK, Mulangu F, et al. Geo-climatic factors of malaria morbidity in the democratic Republic of Congo from 2001 to 2019. Int J Environ Res Public Health. (2022) 19:3811. doi: 10.3390/ijerph19073811
55. Suhr F, Steinert JI. Epidemiology of floods in sub-Saharan Africa: a systematic review of health outcomes. BMC Public Health. (2022) 22:268. doi: 10.1186/s12889-022-12584-4
56. World Health Organization. Fragile and conflict-affected states: health and WHO: country presence profile. World Health Organization (2017). https://iris.who.int/handle/10665/255801.
57. Fenollar F, Mediannikov O. Emerging infectious diseases in Africa in the 21st century. New Microbes New Infect. (2018) 26:S10–S8. doi: 10.1016/j.nmni.2018.09.004
58. World Health Organization. A global brief on vector-borne diseases. (2014) https://iris.who.int/handle/10665/111008 (Accessed [August 11, 2024]).
59. Ouzzani M, Hammady H, Fedorowicz Z, Elmagarmid A. Rayyan—a web and mobile app for systematic reviews. System Rev. (2016) 5:1–10. doi: 10.1186/s13643-016-0384-4
60. Kache PA, Santos-Vega M, Stewart-Ibarra AM, Cook EM, Seto KC, Diuk-Wasser MA. Bridging landscape ecology and urban science to respond to the rising threat of mosquito-borne diseases. Nat Ecol Evol. (2022) 6:1601–16. doi: 10.1038/s41559-022-01876-y
61. Acevedo MA, Prosper O, Lopiano K, Ruktanonchai N, Caughlin TT, Martcheva M, et al. Spatial heterogeneity, host movement and mosquito-borne disease transmission. PloS One. (2015) 10:e0127552. doi: 10.1371/journal.pone.0127552
62. Egid BR, Jaramillo MH, Lindsay TC, Lopez Villegas CI, Mohan K, Ozano K, et al. Integrating city resilience and mosquito-borne diseases–a multi-site case study from the Resilient Cities Network. Cities Health. (2023) 7:348–62. doi: 10.1080/23748834.2022.2127626
63. Evans MV. An integrative approach to mosquito-borne disease in urban areas. University of Georgia (2020). doi: 10.1101/2021.09.06.459057
64. Massaro E, Kondor D, Ratti C. Assessing the interplay between human mobility and mosquito borne diseases in urban environments. Sci Rep. (2019) 9:16911. doi: 10.1038/s41598-019-53127-z
65. Shenton FC, Addissie A, Alabaster G, Baziwe D, Carrasco Tenezaca M, Chinula D, et al. Research agenda for preventing mosquito-transmitted diseases through improving the built environment in sub-Saharan Africa. Cities Health. (2022) 6:72–80. doi: 10.1080/23748834.2019.1684771
66. Dymitrow M, Stenseke M. Rural-urban blurring and the subjectivity within Vol. 3. Rural landscapes: Society, environment, history (2016). doi: 10.16993/rl.1
67. Hugo G. New forms of urbanization: beyond the urban-rural dichotomy. Routledge (2017). doi: 10.4324/9781315248073
68. Menashe-Oren A, Masquelier B. The shifting rural–urban gap in mortality over the life course in low-and middle-income countries. Popul Stud. (2022) 76:37–61. doi: 10.1080/00324728.2021.2020326
69. Vlahov D, Galea S. Urbanization, urbanicity, and health. J Urban Health. (2002) 79:S1–S12. doi: 10.1093/jurban/79.suppl_1.S1
70. Cyril S, Oldroyd JC, Renzaho A. Urbanisation, urbanicity, and health: a systematic review of the reliability and validity of urbanicity scales. BMC Public Health. (2013) 13:1–11. doi: 10.1186/1471-2458-13-513
71. Owino EA. Why Kenya should worry about Anopheles stephensi. Asian Pacific J Trop Med. (2023) 16:99–101. doi: 10.4103/1995-7645.372287
72. Chaki PP, Kannady K, Mtasiwa D, Tanner M, Mshinda H, Kelly AH, et al. Institutional evolution of a community-based programme for malaria control through larval source management in Dar es Salaam, United Republic of Tanzania. Malaria J. (2014) 13:1–13. doi: 10.1186/1475-2875-13-245
73. Maheu-Giroux M, Castro MC. Attribution of reductions in malaria prevalence in Dar es Salaam, Tanzania. Lancet Planet Health. (2019) 3:e246. doi: 10.1016/S2542-5196(19)30071-3
74. Freeman EA, Carlton EJ, Paull S, Dadzie S, Buchwald A. Utilizing citizen science to model the distribution of Aedes aEgypti in West Africa. J Vector Ecol. (2022) 47:117–27. doi: 10.52707/1081-1710-47.1.117
75. Murindahabi MM, Asingizwe D, Poortvliet PM, van Vliet AJ, Hakizimana E, Mutesa L, et al. A citizen science approach for malaria mosquito surveillance and control in Rwanda. NJAS-Wageningen J Life Sci. (2018) 86:101–10. doi: 10.1016/j.njas.2018.07.005
Keywords: urbanization, East Africa, mosquitoes, infection rates, Peri-urban, entomology, scoping review
Citation: Joseph NK, Mumo E, Morlighem C, Macharia PM, Snow RW and Linard C (2024) Mosquito-borne diseases in urban East African Community region: a scoping review of urban typology research and mosquito genera overlap, 2000-2024. Front. Trop. Dis 5:1499520. doi: 10.3389/fitd.2024.1499520
Received: 21 September 2024; Accepted: 13 November 2024;
Published: 04 December 2024.
Edited by:
Falgunee K Parekh, EpiPointe LLC, United StatesReviewed by:
Lara Ferrero Gómez, Universidade Jean Piaget de Cabo, Cabo VerdeNatarajan Gopalan, Central University of Tamil Nadu, India
Copyright © 2024 Joseph, Mumo, Morlighem, Macharia, Snow and Linard. This is an open-access article distributed under the terms of the Creative Commons Attribution License (CC BY). The use, distribution or reproduction in other forums is permitted, provided the original author(s) and the copyright owner(s) are credited and that the original publication in this journal is cited, in accordance with accepted academic practice. No use, distribution or reproduction is permitted which does not comply with these terms.
*Correspondence: Noel K. Joseph, bm9lbC1rYW5pbmkuam9zZXBoQHVuYW11ci5iZQ==
‡ORCID: Noel K. Joseph, orcid.org/0000-0002-0509-1373
Peter M. Macharia, orcid.org/0000-0003-3410-1881
Camille Morlighem, orcid.org/0000-0001-9085-0591
Catherine Linard, orcid.org/0000-0002-0819-7755