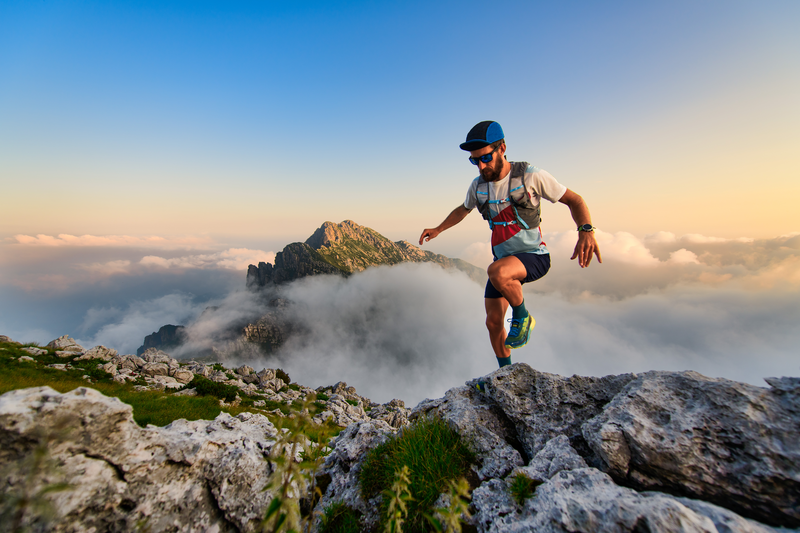
95% of researchers rate our articles as excellent or good
Learn more about the work of our research integrity team to safeguard the quality of each article we publish.
Find out more
ORIGINAL RESEARCH article
Front. Trop. Dis. , 01 November 2024
Sec. Major Tropical Diseases
Volume 5 - 2024 | https://doi.org/10.3389/fitd.2024.1443952
Background: Aedes aegypti vectors several important arboviruses including dengue and yellow fever. This vector mosquito is controlled mainly by using synthetic insecticides and repellents. Overusing these insecticides causes mosquito resistance, harms the environment, and affects human health. This report reevaluates the repellent activities of Cymbopogon nardus, Eucalyptus camaldulensis essential oils (EOs), and their mixtures against laboratory-reared adult Ae. aegypti.
Methods: The chemical composition of C. nardus, E. camaldulensis EOs, and their 1:1 combination was identified by gas chromatography-mass spectrometry (GC-MS). We evaluated the repellent activities of these oils against Ae. aegypti using a Y-maze olfactometer. The preference index (PI) was evaluated and compared with the binary data obtained from the olfactometer assay with samples that did not contain EOs (control) using an Exact Binomial test (α= 0.05)
Results: Several monoterpenes and sesquiterpene compounds were found in EOs and their mixture. The EOs of E. camaldulensis and the mixture of the two oils showed a repellent activity of 50%, whereas C. nardus was less active and attracted mosquitoes at 1 ppm.
Conclusion: We show that EOs from C. nardus and E. camaldulensis contain compounds that repel Ae. aegypti. Future studies will identify specific compounds with the highest repellent activities and use them to formulate in the future a potent repellent against Ae. aegypti for human protection.
Dengue, chikungunya, and zika viruses are transmitted by Aedes mosquitoes. Among these, dengue virus (DENV) is a significant global health concern, affecting millions of people annually (1, 2). Historically, dengue fever was more prevalent in South America than in other parts of the world (3). Over the past two decades, the reported cases of dengue have increased many folds, and the World Health Organization (WHO) reports a more than eight folds increase from 505,430 cases in 2000 to over 2.4 million in 2010 and 5.2 million cases in 2019 (4). Following a slight decline in cases between 2020 and 2022 mainly due to lower reporting during the COVID-19 pandemic and a global upsurge in dengue cases was observed in 2023 (5). Outbreaks have been reported in 15 of the 47 surveyed African countries, including Benin, Burkina Faso, Cape Verde, Chad, Côte d’Ivoire, Ethiopia, Ghana, Guinea, Mali, Mauritius, Niger, Nigeria, São Tomé and Principe, Senegal, and Togo (5). Burkina Faso was the most affected country in the sub-Saharan Africa region in 2023 with a cumulative total of 154,867 suspected cases and 709 recorded deaths (6). This situation is explained by the presence of discarded car tires in the cities and the water-based handwashing stations introduced into public places to reduce or prevent transmission of the SARS-CoV-2 virus (7).
The primary vector responsible for transmitting DENV is female Aedes aegypti, that predominantly inhabits urban areas and breeds in human-made containers. Ae. aegypti exhibits diurnal biting behavior, with peak activity observed during early mornings and evenings before sunset (4, 8). Current strategies for disease control involve the use of insecticides to reduce mosquito populations and the application of chemical repellents to protect humans from mosquito bites (9, 10). The widespread use of synthetic insecticides, however, has led to a surge in insecticide resistance among mosquito populations (11–14) contributing to environmental toxicity that adversely impacts biodiversity and human health (15, 16). It has been shown that Aedes resistant to DDT and pyrethroid are less sensitive to the effects of repellents such as N, N-diethyl-3-methylbenzamide (DEET), ethyl 3-[acetyl(butyl)amino] propanoate (IR3535), and 2-undecanone (17). Similarly, conventional topical repellents, such as DEET, IR3535, and picaridin are used to protect against mosquito bites (18, 19). However, using repellents such as DEET has raised significant health concerns including allergic reactions and skin irritation, and brain disease such as encephalopathy, especially for children (20, 21). Additionally, DEET has limitations, including high production costs, plasticizing effects on polymers, limited efficacy against specific insect species, and nontarget effects (22–24). Keeping in mind the problems associated with chemical insecticides, synthetic repellents, and diseases spread by Ae. aegypti, there is a dire need to find natural chemical ingredient to develop new plant-based mosquito repellents.
Plant-derived oils and extracts are safe and eco-friendly alternatives. Several plant essential oils (EOs) are known to have insecticidal, repellent, and growth-reducing properties (25–29). EOs from the leaves of Lantana camara L., Hyptis suaveolens Poit., Hyptis spicigera Lam, and Ocimum canum Sims show strong oviposition deterrence, excito-repellent and blood-feeding inhibitory activities against An. coluzzii and An. gambiae (28). Various aromatic plant EOs, such as Zanthoxylum piperitum, Anethum graveolens, Kaempferia galanga (30), Lippia alba, L. origanoides, Eucalyptus citriodora, Cymbopogon citratus, Cymbopogon flexuosus, Citrus sinensis, Cananga odorata, Swinglea glutinosa, and Tagetes lucida (31), are known for their repellent effects against Ae. aegypti. Several EOs mixtures exhibit synergistic effects, eliciting stronger repellency when compared with single EOs. For instance, a mixture of Litsea cubeba and Litsea salicifolia oils are highly repellent and is comparable to DEET against Ae. aegypti, than each EO (32). This synergistic effect results from the interaction between specific EO compounds, such as monoterpenes and sesquiterpenes, which deter mosquitoes from landing on treated surfaces (33).
In this study we report the repellent activities of two EOs from local plant species: Eucalyptus camaldulensis and Cymbopogon nardus. The chemical compositions of these EOs were determined using gas chromatography-mass spectrometry and their repellent activity was quantified against female Ae. aegypti mosquitoes using an olfactometer. The repellent potential of these EOs, will contribute to the development of safe and natural mosquito repellents.
Plant leaves (Figure 1) were sampled at the “Institut de Recherche en Sciences Appliquées et Technologies” (IRSAT) in Ouagadougou, Burkina Faso. The leaves are the parts of the plant that contain the most essential oils. The taxonomic identification of the collected samples was carried out at the ‘Laboratoire de Biologie et d’Ecologie Végétales’ (Université joseph KI-ZERBO, Burkina Faso). Essential oils (EOs) were extracted from Eucalyptus camaldulensis (EC) and Cymbopogon nardus (CN) by hydro distillation at IRSAT. The EOs were distilled, dried over anhydrous sodium sulfate, and stored at 4°C.
Gas chromatography-mass spectrometry (GC-MS) analysis identified and quantitated the major and minor constituents of C. nardus, E. camaldulensis EOs alone, and their 1:1 combination. An aliquot (20 μL) was extracted from each EO sample using a micropipette, diluted at 1/5000 in hexane, and placed into a vial with an insert (VWR, Radnor, PA), allowing it to be injected into a GC-MS (Trace 1310, Thermo Fisher Scientific) equipped with a 30 m column (IDD 0.25 mm, #36096-1420, Thermo Fisher Scientific). Five microliters of the prepared samples were loaded into the GC-MS using an autosampler (TriPlus RSH, Thermo Fisher Scientific). Helium was used as the carrier gas at a constant flow of 1 cc/min1 cc/min. The oven temperature was set at 45°C, held for 4 minutes, followed by a heating gradient ramping to 230°C (10°C/min), and the 230°C temperature was maintained isothermally for 6 minutes (total run: 28.5 min.). Each sample was analyzed in triplicates. Chromatographic peaks were integrated using the Chromeleon software MS quantitative processing method (Thermo Fisher Scientific), and chemical compounds were identified using the online NIST library. Major peaks found with consistently high abundances across multiple samples for each EO were then recorded, and compared with all Eos.
The EOs were diluted in mineral oil, and the tested concentrations were obtained from a stock solution of 10,000 ppm (0.5 ml of oil diluted in 49.5 ml of ethanol or 0.25 ml in 24.75 ml of ethanol). A stock solution (10,000 ppm) of the two oil combinations (1:1) was prepared by mixing 0.25 ml of each EO with 49.5 ml of mineral oil from a stock solution (10,000 ppm) aliquots of 1, 10, and 100 µl were removed and added to 10 ml of mineral oil to obtain 1, 10, and 100 ppm dilutions, respectively [Sigma Life Science (M8410)].
Aedes aegypti (Rockefeller strain: MR-734, MR4, ATCC®, Manassas, VA, USA) mosquitoes were reared at Virginia Tech (Blacksburg, VA, USA) in an incubator at 26°C and 60% relative humidity under a 12:12 h (light: dark) cycle. Eggs were hatched in deionized water, and larvae were fed with fish food (Hikari Tropic First Bites, Petco, San Diego, CA, USA). After emergence, adult females were fed on 10% sucrose solution for five days. On day six, the sucrose solution was withdrawn for 24 hours, and female mosquitoes seeking response were determined using an olfactometer.
A Y-maze olfactometer [as described in (34)] was used to quantify the seeking or deterrence response of mosquitoes in the presence of single and mixed EOs at concentrations of 1, 10, and 100 ppm. The olfactometer, constructed using custom-cut acrylic sheets, comprises two choice arms connected to an entry arm via a central chamber (100 cm long, 10 cm internal diameter, with choice arms positioned at an angle of 120°). Two fans, mounted at the end of the choice arms, generated a constant airflow (Rosewill, Los Angeles, CA, USA, air speed ~30 cm.s-1), with incoming air filtered through a series of activated charcoal filters and honeycomb mesh (10 cm long) to create contaminant-free laminar airflow. Charcoal-filtered air (~3 cm.s-1) passed through scintillation vials containing either the test (EOs) or control odor (mineral oil) and was delivered as olfactory stimuli at the distal end of the olfactometer’s choice arms through divided circuits of polytetrafluoroethylene (PTFE) tubing (McMaster-Carr, Elmhurst, IL, USA). The position of the test and control olfactory stimuli were randomized between the two choice arms across experimental trials to eliminate bias (35). The behavior of individual mosquitoes in the olfactometer was observed for 5 minutes. Additionally, statistical analysis of mosquitoes’ behavioral preference toward two clean air currents (i.e., mineral oil-laden air delivered in both choice arms of the olfactometer) confirmed the absence of directional bias in the assay (n = 16; Binomial Exact test: P = 0.59) (35). For each EO concentration, assays evaluating the behavioral preference of individual females were spread across two to three experimental sessions to address reproducibility and block biased effects. For every EO concentration tested, 24-28 female mosquitoes (released individually into the olfactometer) that showed attraction or deterrence during the assay were included in the analysis of behavioral preference. The sample size that we used is sufficient to achieve a statistical power > 0.8. All assays were conducted in a well-ventilated room at a constant temperature (26 ± 1.5°C) and relative humidity (50–60%). DEET (98.11%) served as a positive control to demonstrate deterrence.
The olfactory preference of mosquitoes in the Y-maze olfactometer assay was quantified as a preference index (P). The preference index was calculated using the formula: P = (O - C)/(O + C), where O denotes the number of mosquitoes that preferred the odor arm, and C denotes the number of mosquitoes that preferred the control arm. The standard error for the preference index was computed using the formula √{[q(1-q)]/[O+C]}, where q denotes the proportion of active mosquitoes. The binary data quantified in the olfactometer assay were compared to chance using the Binomial Exact test (α = 0.05). The percentage repellency of the EOs and the proportion of active and decision-making mosquitoes were compared across treatments in the olfactometer assay using a Generalized Linear Model assuming binomially distributed errors (36). Post hoc comparisons reported in this study were performed using the Tukey’s HSD method and corrected for pairwise multiple comparisons. All analyses were performed using R software (version 4.2.2).
Thirty-seven compounds with concentrations higher than 0.1% were identified in C. nardus (Table 1). The main constituents of this oil were citronellal (28.41%), geranial (16.03%), cis-geraniol (10.30%), and elemol (7.39%). The remaining compounds were less than 5% in this oil. In E. camaldulensis, 25 compounds were identified (Table 1), with the major compounds being eucalyptol (48.59%), p-cymene (23.86%), γ-Terpinene (6.83%), and α-Phellandrene (5.13%). The blend (1:1) of C. nardus and E. camaldulensis contained a reduced number of compounds (21), with the primary constituents being eucalyptol (36.61%), citronellal (29.67%), nerol (9.5%), α-Pinene (7.19%), and p-Cymene (6.51%). Some compounds in the mixture were less abundant compared with the individual oil samples. Nerol, γ-Elemen, and β-Elemen were present in the mixture despite their initial absence in the individual oils.
In the Y-maze olfactometer, when given the choice between two clean air currents (negative control), the mosquitoes showed random orientation between the two arms, indicating no bias in the experimental setup (n=44, P > 0.05). At a 1 ppm concentration (Figure 2A), the EO from E. camaldulensis (EC) demonstrated significant repellent activity both alone (n=27, P = 0.026) and in combination with the EO from C. nardus (CN) (n=70, P = 0.021). The EO from CN at a 1 ppm concentration showed low levels of attraction that were not statistically significant (n=25, P > 0.05). At a 10 ppm concentration, the EOs from both EC and CN did not exhibit repellent activity (n=25 per EO, P > 0.05). The combination of EOs from EC and CN at a 10 ppm concentration did not show repellent activity (n=26, P > 0.05). At a 100 ppm concentration, the EO from EC showed the strongest repellent activity (n=25, P = 0.007) (Figure 2A). Additionally, the EOs from EC and CN, in combination at a 100 ppm concentration, displayed significant repellent activity (n=27, P = 0.026). However, when used alone at a 100 ppm concentration, the EO from CN did not demonstrate significant repellent activity (n=27, P > 0.05).
Figure 2. Olfactory responses of Ae. aegypti adult female mosquitoes: (A) Mosquito olfactory preference represented as a preference index. Asterisks denote responses that were significantly different from chance (Binomial Exact test, p < 0.05), (B) Proportion of active mosquitoes during bioassay, (C) Proportion of mosquitoes making an active choice between the two choice arms of the olfactometer; CN, Cymbopogon nardus; EC, Eucalyptus camaldulensis. The different lowercase letters show Statistical Significance of essential oil effect. Graphs with the same letters are not statistically different.
The activity levels of mosquitoes in the olfactometer assay (i.e., the proportions of mosquitoes initiating flight to move out of the release arm in the olfactometer) were consistently over 50% (Figure 2B). When presented with no odorant stimulus, i.e., negative control, 93.4% of the mosquitoes were active in the assay (n=57). In comparison, the EOs from CN and EC, both in isolation and in combination at 1 ppm concentration, elicited statistically comparable levels of flight initiation and activity (CN: 96.6%, n=56; EC: 98.2%, n=55, CN+EC: 98.9%, n=89; P > 0.05 for all comparisons). At 10 ppm concentration, the activity levels of mosquitoes exposed to the EO from CN were significantly lower when compared to the negative control (CN: 62.9%, n=44; P = 0.012). The EO from EC, in isolation and in combination with the EO from CN at 10 ppm concentration, elicited activity levels statistically comparable to the negative control (EC: 86.8%, n=66, CN+EC: 98.5%, n=64; P > 0.05 for all comparisons) (Figure 2B). The EOs, at 100 ppm concentration, did not affect the activity levels of mosquitoes in the olfactometer assay (CN: 83.5%, n=66; EC: 96.8%, n=60, CN+EC: 100%, n=48; P > 0.05 for all comparisons).
The analysis of the proportion of decision-making mosquitoes (i.e., the proportions of active mosquitoes that made a choice between control and test odors) revealed that 77.2% of the active mosquitoes, in the absence of an odorant stimulus i.e., negative control, made a choice in the olfactometer assay (n=44) (Figure 2C). The proportion of decision-making mosquitoes in the olfactometer assay across the EOs, both in isolation and in combination, as well as across the three concentrations, although slightly lower compared to the negative control, were statistically comparable.
GC-MS analyses revealed several compounds in the extracted plants’ essential oils (EOs) and their mixtures. Previous studies have shown variations in the chemical composition of EOs of E. camaldulensis (37, 38) and C. nardus (39, 40), exhibiting different chemotypes. For example, in the repellent study by Azeem et al., E. camaldulensis major compounds were limonene, trans-β-ocimene, and germacrene D, while in the larvicidal study by Manh et al. (38), the major compounds were 1,8-cineol, α-pinene, and citronellyl acetate. Citronellal and geraniol are consistently major compounds in C. nardus (41, 42). The composition of E. camaldulensis is typically varied, containing compounds such as 1,8 cineole, γ-terpinene, α-Phellandrene, and p-Cymene (43, 44). The use of 12 EOs of the Eucalyptus genus against Ae. aegypti highlighted major compounds like 1,8-cineole, α-pinene, α-phellandrene, β-phellandrene, γ-terpinene, 4-terpineol, α-terpineol, p-cymene, and spathulenol (45). In our study, eucalyptol and p-cymene appear to be specific to E. camaldulensis oil. The high concentration of eucalyptol in E. camaldulensis (48.59%) might be more influential in repellent activity compared to the lesser concentrations of other compounds.
Compounds such as α-Pinene, α-Terpinolene, Citronellal, α-Terpinyl propionate, Decanal, Geraniol, cis-Geraniol, α-Citral, Eugenol, Geranyl acetate, Germacrene D, Aromandendrene, Elemol, Thunbergol, α-Cadinol known to have mosquito repellent properties (46–53) are present in C. nardus and absent in E. camaldulensis. Previous studies in Burkina Faso showed the presence of only 1,8-cineole in large quantities in E. camadulensis while C. nardus mainly contained geraniol, citronelal, elemol and nerol, compounds known for their antifungal and insecticidal properties against Ae. aegypti. This justifies the repellent effect of and E. camaldulensis in this study. In the combination of oils, only four compounds (α-Pinene, Citronellal, Elemol, and α-Cadinol) recognized as good repellents were detected, explaining the weak repellent activity of the EO combination. Eucalyptol, p-cymene, and α-phellandrene, known for their repellent properties (54, 55), were found in large quantities in the oil of E. camaldulensis and could justify its strong repulsion against Ae. aegypti.
In the combination of the two oils, we detected fewer compounds than in each individual EO. Muturi et al., who studied the EOs of Origanum vulgare, Syzygium aromaticum, and Leptospermum scoparium against Ae. aegypti, reported similar findings (56). This could be attributed to the dilution of minor constituents into undetectable levels in the oil mixtures. Additionally, similar to other research on EOs combinations, we identified new compounds such as Nerol, γ-Elemen, and β-Elemen (29, 56). These new compounds may be linked to a different distribution based on solubility and mixing, which likely altered the composition of the original EOs (56). The variations in the composition of EOs between the two plants can be attributed to various factors, such as the age of the plant, the plant parts used in oil extraction, the extraction method, the seasonal sampling period, and environmental and climatic conditions (57). Moreover, ethanol which was used as a solvent may have an effect as well. It might be interesting to explore why some compounds, like nerol or γ-Elemen, appear in the blend but not in the individual oils.
Based on the results of the olfactometer test, the EOs repelled female Ae. aegypti at 1ppm and 100 ppm concentrations for EC. However, C. nardus at 1 ppm attracted mosquitoes. The activity was dose-dependent for EC and the EC + CN mixture at 1 and 100ppm concentrations. These results were similar to those obtained by Uniyal et al. (26) which showed the repulsive effect of 23 EOs against Ae. aegypti by olfactometry (26). The EOs of C. nardus were reported to act in a dose-dependent repellent activity against Ae. aegypti, especially when mixed with citral and myrcene (40). In previous studies, several EOs showed repellent properties against Ae. aegypti either through formulations (58–61) or through combinations of EOs (56, 62, 63) or by using simple oils (56, 64). E. camaldulensis showed weak repellent activity significantly different from the response to the EO at 1 ppm. Of the three types of oils used, E. camaldulensis was most active, followed by the CN + EC mixtures at 1 and 100ppm and C. nardus at 100 ppm however it was weakly attractive at 1 ppm. While the response at 10 ppm does not exhibit a straightforward dose-dependent trend, the increase in the preference index at 100 ppm highlights the complex, non-linear nature of mosquito behavior in response to essential oils (EOs). We speculate that this pattern can be explained by the dynamics of olfactory receptor adaptation, where intermediate concentrations like 10 ppm may temporarily saturate or desensitize receptors, leading to a plateau (65, 66). However, at higher concentrations such as 100 ppm, these effects can be overcome, potentially due to the activation of additional sensory pathways or cumulative receptor effects, resulting in a renewed and stronger behavioral response (67, 68). The non-linear dose-response relationships observed in insect behavior, including that of mosquitoes, are well-documented and suggest that behavioral responses can vary widely with concentration (69, 70). This underscores the importance of considering the intricate mechanisms at play in mosquito olfactory systems, reinforcing the validity of our findings despite the lack of a simple linear relationship (71, 72). Finally, the observed mosquito behavior at 10ppm leads us to further questions on mechanisms underlying the dose-dependent responses to repellents which could be addressed in future studies.The EOs contain citronellal, 1,8-cineole, carvacrol, geraniol, caryophyllene, p-cymene, pinene that were reported to act as repellents (73), similar to some of the EOs compositions in this study and are known to be mosquito repellents. In recent study, researchers show that the performance of the citronellal is comparable (95% protection for ≤3.5 h) with those of the most widespread synthetic repellents DEET and Picaridin, tested at a four-fold higher doses against Aedes albopictus and An. gambiae (74). Eucalyptol, the major component in the oil of E. camaldulensis in combination with CN + EC mixture, is an effective repellent. In some studies where pure compounds were tested, eucalyptol was more repellent than the other compounds against Rhodnius prolixus Ståhl and Ae. aegypti (73, 75). This mixture did not show any synergy between the two oils and this would be due to antagonism between certain compounds of the two plants, which would reduce the repellent potential of the mixture. Cases of antagonisms are reported when evaluating the repellent effects of oils against ticks and mosquitoes (73). These results using eucalyptus oils make it possible to use the eucalyptus leaves, in tropical zones, which are often discarded after the tree trunks are used in the construction of buildings. At low doses, C. nardus seems to attract mosquitoes, probably due to some of the volatile compounds in this oil mixture that would attract mosquitoes and therefore could be used in mosquito traps. Several compounds have been reported to attract mosquitoes like limonene, menthone, and hexanal, however, they are weak attractants as compared with 3-methyl indole (76). Screening of the individual compounds identified in C. nardus EOs could in the future identify repellent compounds and those that are attractants.
The EOs tested in this study contain several compounds that are potential repellents of Ae. aegypti. The EO of E. camaldulensis and strongly repelled Ae. aegypti than the EOs of C. nardus, which lost potency at high concentrations and exhibited weak attraction at 1 ppm. The mixture of C. nardus and E. camaldulensis EOs had a little repellent effect compared to E. camaldulensis oil. Future characterizations of these compounds could be used for vector control applications.
The raw data supporting the conclusions of this article will be made available by the authors, without undue reservation.
The manuscript presents research on animals that do not require ethical approval for their study.
DW: Conceptualization, Data curation, Funding acquisition, Methodology, Writing – original draft. KC: Data curation, Formal analysis, Methodology, Software, Visualization, Writing – review & editing. FU: Investigation, Writing – review & editing. DB: Supervision, Visualization, Writing – review & editing. IS: Supervision, Writing – review & editing. CV: Supervision, Validation, Writing – review & editing. AB: Writing – review & editing. SA: Supervision, Writing – review & editing. CL: Conceptualization, Supervision, Validation, Visualization, Writing – review & editing.
The author(s) declare financial support was received for the research, authorship, and/or publication of this article. DW was partly supported by the Fulbright Foreign Student Program, Grantee ID: PS00301440.
The authors are thankful to The Fralin Life Science Institute, Virginia Polytechnic Institute and State University, Blacksburg for facilitating DW’s Fulbright stay.
The authors declare that the research was conducted in the absence of any commercial or financial relationships that could be construed as a potential conflict of interest.
All claims expressed in this article are solely those of the authors and do not necessarily represent those of their affiliated organizations, or those of the publisher, the editors and the reviewers. Any product that may be evaluated in this article, or claim that may be made by its manufacturer, is not guaranteed or endorsed by the publisher.
1. Guzman MG, Halstead SB, Artsob H, Buchy P, Farrar J, Gubler DJ, et al. Dengue: a continuing global threat. Nat Rev Microbiol. (2010) 8:S7–S16. doi: 10.1038/nrmicro2460
2. Whitehorn J, Chau NVV, Truong NT, Tai LTH, Van Hao N, Hien TT, et al. Lovastatin for adult patients with dengue: protocol for a randomised controlled trial. Trials. (2012) 13:1–6. doi: 10.1186/1745-6215-13-203
3. Messina JP, Brady OJ, Scott TW, Zou C, Pigott DM, Duda KA, et al. Global spread of dengue virus types: mapping the 70 year history. Trends Microbiol. (2014) 22:138–46. doi: 10.1016/j.tim.2013.12.011
4. WHO. Dengue et dengue sévère. In: World Health Organisation report (2021) (Geneva: World Health Organization). Available at: https://www.who.int/fr/news-room/fact-sheets/detail/dengue-and-severe-dengue.
5. WHO. World health organization (21 december 2023). In: Disease Outbreak News; Dengue – Global situation (2023) (Geneva: World Health Organization). Available at: https://www.who.int/emergencies/disease-outbreak-news/item/2023-DON498.
6. Manigart O, Ouedraogo I, Ouedraogo HS, Sow A, Lokossou VK. Dengue epidemic in Burkina Faso: how can the response improve? Lancet. (2024) 403:434–5. doi: 10.1016/S0140-6736(23)02803-9
7. Ouédraogo WM, Zanré N, Rose NH, Zahouli JZ, Djogbenou LS, Viana M, et al. Dengue vector habitats in Ouagadougou, Burkina Fas : an unintended consequence of the installation of public handwashing stations for COVID-19 prevention. Lancet Global Health. (2024) 12:e199–200. doi: 10.1016/S2214-109X(23)00565-X
8. Baik LS, Nave C, Au DD, Guda T, Chevez JA, Ray A, et al. Circadian regulation of light-evoked attraction and avoidance behaviors in daytime-versus nighttime-biting mosquitoes. Curr Biol. (2020) 30:3252–3259. e3253. doi: 10.1016/j.cub.2020.06.010
9. Zaim M, Guillet P. Alternative insecticides: an urgent need. Trends Parasitol. (2002) 18:161–3. doi: 10.1016/S1471-4922(01)02220-6
10. Norris EJ, Coats JR. Current and future repellent technologies: the potential of spatial repellents and their place in mosquito-borne disease control. Int J Environ Res Public Health. (2017) 14:124. doi: 10.3390/ijerph14020124
11. Badolo A, Sombié A, Pignatelli PM, Sanon A, Yaméogo F, Wangrawa DW, et al. Insecticide resistance levels and mechanisms in Aedes aEgypti populations in and around Ouagadougou, Burkina Faso. PloS Negl Trop Dis. (2019) 13:e0007439. doi: 10.1371/journal.pntd.0007439
12. Djiappi-Tchamen B, Nana-Ndjangwo MS, Mavridis K, Talipouo A, Nchoutpouen E, Makoudjou I, et al. Analyses of Insecticide Resistance Genes in Aedes aEgypti and Aedes albopictus Mosquito Populations from Cameroon. Genes. (2021) 12:828. doi: 10.3390/genes12060828
13. Rahman RU, Cosme LV, Costa MM, Carrara L, Lima JBP, Martins AJ. Insecticide resistance and genetic structure of Aedes aEgypti populations from Rio de Janeiro State, Brazil. PloS Negl Trop Dis. (2021) 15:e0008492. doi: 10.1371/journal.pntd.0008492
14. Sombié A, Ouédraogo WM, Oté M, Saiki E, Sakurai T, Yaméogo F, et al. Association of 410 I and 1534C kdr mutations with pyrethroid resistance in Aedes aEgypti from Ouagadougou, Burkina Faso, and development of a one-step multiplex PCR method for the simultaneous detection of 1534C and 1016I kdr mutations. Parasites Vectors. (2023) 16:1–9. doi: 10.1186/s13071-023-05743-y
15. Koureas M, Tsakalof A, Tsatsakis A, Hadjichristodoulou C. Systematic review of biomonitoring studies to determine the association between exposure to organophosphorus and pyrethroid insecticides and human health outcomes. Toxicol Lett. (2012) 210:155–68. doi: 10.1016/j.toxlet.2011.10.007
16. Roy DN, Goswami R, Pal A. The insect repellents: A silent environmental chemical toxicant to the health. Environ Toxicol Pharmacol. (2017) 50:91–102. doi: 10.1016/j.etap.2017.01.019
17. Yang L, Norris EJ, Jiang S, Bernier UR, Linthicum KJ, Bloomquist JR. Reduced effectiveness of repellents in a pyrethroid-resistant strain of Aedes aEgypti (Diptera: culicidae) and its correlation with olfactory sensitivity. Pest Manage Sci. (2020) 76:118–24. doi: 10.1002/ps.v76.1
18. Badolo A, Ilboudo-Sanogo E, Ouédraogo AP, Costantini C. Evaluation of the sensitivity of Aedes aEgypti and Anopheles Gambiae complex mosquitoes to two insect repellents: DEET and KBR 3023. Trop Med Int Health. (2004) 9:330–4. doi: 10.1111/j.1365-3156.2004.01206.x
19. Costantini C, Badolo A, Ilboudo-Sanogo E. Field evaluation of the efficacy and persistence of insect repellents DEET, IR3535, and KBR 3023 against Anopheles Gambiae complex and other Afrotropical vector mosquitoes. Trans R Soc Trop Med Hygiene. (2004) 98:644–52. doi: 10.1016/j.trstmh.2003.12.015
20. Osimitz T, Murphy J, Fell L, Page B. Adverse events associated with the use of insect repellents containing N, N-diethyl-m-toluamide (DEET). Regul Toxicol Pharmacol. (2010) 56:93–9. doi: 10.1016/j.yrtph.2009.09.004
21. Chen-Hussey V, Behrens R, Logan JG. Assessment of methods used to determine the safety of the topical insect repellent N, N-diethyl-m-toluamide (DEET). Parasites Vectors. (2014) 7:1–7. doi: 10.1186/1756-3305-7-173
22. Oliferenko PV, Oliferenko AA, Poda GI, Osolodkin DI, Pillai GG, Bernier UR, et al. Promising Aedes aEgypti repellent chemotypes identified through integrated QSAR, virtual screening, synthesis, and bioassay. PloS One. (2013) 8:e64547. doi: 10.1371/journal.pone.0064547
23. Slaninova A, Modra H, Hostovsky M, Sisperova E, Blahova J, Matejova I, et al. Effects of subchronic exposure to N, N-diethyl-m-toluamide on selected biomarkers in common carp (Cyprinus carpio L.). BioMed Res Int 2014. (2014) 2014(1):828515. doi: 10.1155/2014/828515
24. Grant GG, Estrera RR, Pathak N, Hall CD, Tsikolia M, Linthicum KJ, et al. Interactions of DEET and novel repellents with mosquito odorant receptors. J Med entomology. (2020) 57:1032–40. doi: 10.1093/jme/tjaa010
25. Pavela R, Benelli G. Ethnobotanical knowledge on botanical repellents employed in the African region against mosquito vectors–a review. Exp Parasitol. (2016) 167:103–8. doi: 10.1016/j.exppara.2016.05.010
26. Uniyal A, Tikar SN, Mendki MJ, Singh R, Shukla SV, Agrawal OP, et al. Behavioral response of Aedes aEgypti mosquito towards essential oils using olfactometer. J arthropod-borne Dis. (2016) 10:370.
27. Chellappandian M, Vasantha-Srinivasan P, Senthil-Nathan S, Karthi S, Thanigaivel A, Ponsankar A, et al. Botanical essential oils and uses as mosquitocides and repellents against dengue. Environ Int. (2018) 113:214–30. doi: 10.1016/j.envint.2017.12.038
28. Wangrawa DW, Badolo A, Guelbéogo WM, Nebie RCH, Borovsky D, Sanon A. Larvicidal, oviposition-deterrence, and excito-repellency activities of four essential oils: an eco-friendly tool against malaria vectors Anopheles coluzzii and Anopheles Gambiae (Diptera: Culicidae). Int J Trop Insect Sci. (2021) 41:1771–81. doi: 10.1007/s42690-020-00390-7
29. Wangrawa DW, Ochomo E, Upshur F, Zanré N, Borovsky D, Lahondere C, et al. Essential oils and their binary combinations have synergistic and antagonistic insecticidal properties against Anopheles Gambiae sl (Diptera: Culicidae). Biocatalysis Agric Biotechnol. (2022) 42:102347. doi: 10.1016/j.bcab.2022.102347
30. Choochote W, Chaithong U, Kamsuk K, Jitpakdi A, Tippawangkosol P, Tuetun B, et al. Repellent activity of selected essential oils against Aedes aEgypti. Fitoterapia. (2007) 78:359–64. doi: 10.1016/j.fitote.2007.02.006
31. Castillo RM, Stashenko E, Duque JE. Insecticidal and repellent activity of several plant-derived essential oils against Aedes aEgypti. J Am Mosq Control Assoc. (2017) 33:25–35. doi: 10.2987/16-6585.1
32. Noosidum A, Chareonviriyaphap T, Chandrapatya A. Synergistic repellent and irritant effect of combined essential oils on Aedes aEgypti (L.) mosquitoes. J Vector Ecol. (2014) 39:298–305. doi: 10.1111/jvec.12104
33. Lee MY. Essential oils as repellents against arthropods. BioMed Res Int. (2018) 2018:6860271. doi: 10.1155/2018/6860271
34. Vinauger C, Lahondère C, Wolff GH, Locke LT, Liaw JE, Parrish JZ, et al. Modulation of host learning in Aedes aEgypti mosquitoes. Curr Biol. (2018) 28:333–344. e338. doi: 10.1016/j.cub.2017.12.015
35. Eilerts DF, VanderGiessen M, Bose EA, Broxton K, Vinauger C. Odor-specific daily rhythms in the olfactory sensitivity and behavior of Aedes aEgypti mosquitoes. Insects. (2018) 9:147. doi: 10.3390/insects9040147
36. Erler F, Ulug I, Yalcinkaya B. Repellent activity of five essential oils against Culex pipiens. Fitoterapia. (2006) 77:491–4. doi: 10.1016/j.fitote.2006.05.028
37. Azeem M, Zaman T, Tahir M, Haris A, Iqbal Z, Binyameen M, et al. Chemical composition and repellent activity of native plants essential oils against dengue mosquito, Aedes aEgypti. Ind Crops Products. (2019) 140:111609. doi: 10.1016/j.indcrop.2019.111609
38. Manh HD, Hue DT, Hieu NTT, Tuyen DTT, Tuyet OT. The Mosquito larvicidal activity of essential oils from Cymbopogon and Eucalyptus Species in Vietnam. Insects. (2020) 11:128. doi: 10.3390/insects11020128
39. Solomon B, Gebre-Mariam T, Asres K. Mosquito repellent actions of the essential oils of Cymbopogon citratus, Cymbopogon nardus and Eucalyptus citriodora: evaluation and formulation studies. J Essential Oil Bearing Plants. (2012) 15:766–73. doi: 10.1080/0972060X.2012.10644118
40. Hsu W-S, Yen J-H, Wang Y-S. Formulas of components of citronella oil against mosquitoes (Aedes aEgypti). J Environ Sci Health Part B. (2013) 48:1014–9. doi: 10.1080/03601234.2013.816613
41. Nakahara K, Alzoreky NS, Yoshihashi T, Nguyen HT, Trakoontivakorn G. Chemical composition and antifungal activity of essential oil from Cymbopogon nardus (citronella grass). Japan Agric Res Quarterly: JARQ. (2013) 37:249–52. doi: 10.6090/jarq.37.249
42. Kaur H, Bhardwaj U, Kaur R. Cymbopogon nardus essential oil: a comprehensive review on its chemistry and bioactivity. J Essential Oil Res. (2021) 33:205–20. doi: 10.1080/10412905.2021.1871976
43. Batish DR, Singh HP, Kohli RK, Kaur S. Eucalyptus essential oil as a natural pesticide. For Ecol Manage. (2008) 256:2166–74. doi: 10.1016/j.foreco.2008.08.008
44. Medhi SM, Reza S, Mahnaz K, Reza AM, Abbas H, Fatemeh M, et al. Phytochemistry and larvicidal activity of Eucalyptus camaldulensis against malaria vector, Anopheles stephensi. Asian Pacific J Trop Med. (2010) 3:841–5. doi: 10.1016/S1995-7645(10)60203-9
45. Lucia A, Licastro S, Zerba E, Masuh H. Yield, chemical composition, and bioactivity of essential oils from 12 species of Eucalyptus on Aedes aEgypti larvae. Entomologia experimentalis applicata. (2008) 129:107–14. doi: 10.1111/j.1570-7458.2008.00757.x
46. Schultz G, Peterson C, Coats J. Natural insect repellents: activity against mosquitoes and cockroaches. (2006) (Washington, DC, USA: American Chemical Society) 927:168–181.
47. Ravi Kiran S, Sita Devi P. Evaluation of mosquitocidal activity of essential oil and sesquiterpenes from leaves of Chloroxylon swietenia DC. Parasitol Res. (2007) 101:413–8. doi: 10.1007/s00436-007-0485-z
48. Alighiri D, Cahyono E, Eden WT, Kusuma E, Supardi KI. Study on the improvement of essential oil quality and its repellent activity of betel leaves oil (Piper betle l.) from Indonesia. Oriental J Chem. (2018) 34:2913. doi: 10.13005/ojc
49. Baker BP, Grant JA. Active ingredients eligible for minimum risk pesticide use: Overview of the profiles. (2018) (New York, NY, USA: Cornell University).
50. Yusufoglu HS, Tabanca N, Bernier UR, Li AY, Salkini MA, Alqasoumi SI, et al. Mosquito and tick repellency of two Anthemis essential oils from Saudi Arabia. Saudi Pharm J. (2018) 26:860–4. doi: 10.1016/j.jsps.2018.03.012
51. Portilla-Pulido JS, Castillo-Morales RM, Barón-Rodríguez MA, Duque JE, Mendez-Sanchez SC. Design of a repellent against Aedes aEgypti (Diptera: Culicidae) using in silico simulations with AaegOBP1 protein. J Med entomology. (2020) 57:463–76. doi: 10.1093/jme/tjz171
52. Liakakou A, Angelis A, Papachristos DP, Fokialakis N, Michaelakis A, Skaltsounis LA. Isolation of volatile compounds with repellent properties against Aedes albopictus (Diptera: Culicidae) using CPC technology. Molecules. (2021) 26:3072. doi: 10.3390/molecules26113072
53. İdris AA, Ishag O, Abdurahman N. Chemical composition and repellent activity of methyl cinnamate-rich basil (Ocimum basilicum) essential oil. J Turkish Chem Soc Section A: Chem. (2022) 9:1277–84. doi: 10.18596/jotcsa.1108807
54. Sukontason KL, Boonchu N, Sukontason K, Choochote W. Effects of eucalyptol on house fly (Diptera: Muscidae) and blow fly (Diptera: Calliphoridae). Rev do Instituto Medicina Trop São Paulo. (2004) 46:97–101. doi: 10.1590/S0036-46652004000200008
55. Sfara V, Zerba EN, Alzogaray RA. Fumigant insecticidal activity and repellent effect of five essential oils and seven monoterpenes on first-instar nymphs of Rhodnius prolixus. J Med entomology. (2014) 46:511–5. doi: 10.1603/033.046.0315
56. Muturi EJ, Ramirez JL, Doll KM, Bowman MJ. Combined toxicity of three essential oils against Aedes aEgypti (Diptera: Culicidae) larvae. J Med Entomology. (2017) 54:1684–91. doi: 10.1093/jme/tjx168
57. Bisht D, Chanotiya CS, Rana M, Semwal M. Variability in essential oil and bioactive chiral monoterpenoid compositions of Indian oregano (Origanum vulgare L.) populations from northwestern Himalaya and their chemotaxonomy. Ind Crops Products. (2009) 30:422–6. doi: 10.1016/j.indcrop.2009.07.014
58. Misni N, Nor ZM, Ahmad R. Repellent effect of microencapsulated essential oil in lotion formulation against mosquito bites. J Vector Borne Dis. (2017) 54:44. doi: 10.4103/0972-9062.203183
59. Arpiwi NL, MUksin IK, Kartini NL. Essential oil from Cymbopogon nardus and repellant activity against Aedes aEgypti. Biodiversitas J Biol Diversity. (2020) 21. doi: 10.13057/biodiv/d210857
60. Esmaili F, Sanei-Dehkordi A, Amoozegar F, Osanloo M. A review on the use of essential oil-based nanoformulations in control of mosquitoes. Biointerface Res Appl Chem. (2021) 11:12516–29. doi: 10.33263/BRIAC
61. Luker HA, Salas KR, Esmaeili D, Holguin FO, Bendzus-Mendoza H, Hansen IA. Repellent efficacy of 20 essential oils on Aedes aEgypti mosquitoes and Ixodes scapularis ticks in contact-repellency assays. Sci Rep. (2023) 13:1705. doi: 10.1038/s41598-023-28820-9
62. Harismah K, Vitasari D, Mirzaei M, Fuadi AM, Aryanto YH. (2017). Year. Published. Protection capacity of mosquito repellent ink from citronella (Cymbopogon nardus L.) and clove leaf oils (Syzygium aromaticum) againts Aedes aEgypti, in: AIP Conference Proceedings, . p. 020023. AIP Publishing LLC.
63. Peach DA, Almond M, Gries R, Gries G. Lemongrass and cinnamon bark: plant essential oil blend as a spatial repellent for mosquitoes in a field setting. J Med entomology. (2019) 56:1346–52. doi: 10.1093/jme/tjz078
64. Chattopadhyay P, Dhiman S, Borah S, Rabha B, Chaurasia AK, Veer V. Essential oil based polymeric patch development and evaluating its repellent activity against mosquitoes. Acta tropica. (2015) 147:45–53. doi: 10.1016/j.actatropica.2015.03.027
65. Clyne PJ, Warr CG, Carlson JR. Candidate taste receptors in Drosophila. Science. (2000) 287:1830–4. doi: 10.1126/science.287.5459.1830
66. Dekker T, Ibba I, Siju K, Stensmyr MC, Hansson BS. Olfactory shifts parallel superspecialism for toxic fruit in Drosophila melanogaster sibling, D. sechellia. Curr Biol. (2006) 16:101–9. doi: 10.1016/j.cub.2005.11.075
67. Hallem EA, Carlson JR. Coding of odors by a receptor repertoire. Cell. (2006) 125:143–60. doi: 10.1016/j.cell.2006.01.050
68. DeGennaro M, McBride CS, Seeholzer L, Nakagawa T, Dennis EJ, Goldman C, et al. orco mutant mosquitoes lose strong preference for humans and are not repelled by volatile DEET. Nature. (2013) 498:487–91. doi: 10.1038/nature12206
69. Ghaninia M, Larsson M, Hansson BS, Ignell R. Natural odor ligands for olfactory receptor neurons of the female mosquito Aedes aEgypti: use of gas chromatography-linked single sensillum recordings. J Exp Biol. (2008) 211:3020–7. doi: 10.1242/jeb.016360
70. Wang G, Carey AF, Carlson JR, Zwiebel LJ. Molecular basis of odor coding in the malaria vector mosquito Anopheles Gambiae. Proc Natl Acad Sci. (2010) 107:4418–23. doi: 10.1073/pnas.0913392107
71. Zwiebel L, Takken W. Olfactory regulation of mosquito–host interactions. Insect Biochem Mol Biol. (2004) 34:645–52. doi: 10.1016/j.ibmb.2004.03.017
72. Syed Z, Leal WS. Acute olfactory response of Culex mosquitoes to a human-and bird-derived attractant. Proc Natl Acad Sci. (2009) 106:18803–8. doi: 10.1073/pnas.0906932106
73. Benelli G, Pavela R. Beyond mosquitoes—Essential oil toxicity and repellency against bloodsucking insects. Ind Crops products. (2018) 117:382–92. doi: 10.1016/j.indcrop.2018.02.072
74. Iovinella I, Caputo B, Cobre P, Manica M, Mandoli A, Dani FR. Advances in mosquito repellents: effectiveness of citronellal derivatives in laboratory and field trials. Pest Manage Sci. (2022) 78:5106–12. doi: 10.1002/ps.v78.12
75. de Brito GA, Rocha de Oliveira PF, de Andrade Silva CM, de Araújo Neto MF, Leite FHA, Mesquita PRR, et al. Identification of bioactive compounds against Aedes aEgypti (Diptera: Culicidae) by bioassays and in silico assays. Chem Biodiversity. (2021) 18:e2100242. doi: 10.1002/cbdv.202100242
Keywords: essential oil, repellent, Aedes aegypti, mixture, GC-MS
Citation: Wangrawa DW, Chandrasegaran K, Upshur F, Borovsky D, Sharakhov IV, Vinauger C, Badolo A, Sanon A and Lahondère C (2024) Behavioral response of Aedes aegypti (Diptera: Culicidae) to essential oils of Cymbopogon nardus (L.) Eucalyptus camaldulensis (Dehnh) and their blend in Y-maze olfactometer. Front. Trop. Dis 5:1443952. doi: 10.3389/fitd.2024.1443952
Received: 04 June 2024; Accepted: 10 October 2024;
Published: 01 November 2024.
Edited by:
Ramesh C. Dhiman, National Institute of Malaria Research (ICMR), IndiaReviewed by:
Merid Negash Getahun, International Centre of Insect Physiology and Ecology (ICIPE), KenyaCopyright © 2024 Wangrawa, Chandrasegaran, Upshur, Borovsky, Sharakhov, Vinauger, Badolo, Sanon and Lahondère. This is an open-access article distributed under the terms of the Creative Commons Attribution License (CC BY). The use, distribution or reproduction in other forums is permitted, provided the original author(s) and the copyright owner(s) are credited and that the original publication in this journal is cited, in accordance with accepted academic practice. No use, distribution or reproduction is permitted which does not comply with these terms.
*Correspondence: Dimitri Wendgida Wangrawa, ZGltd2FuZzU2QGdtYWlsLmNvbQ==
†Present address: Karthikeyan Chandrasegaran, Department of Entomology, Entomology Museum, Riverside, CA, United States
Disclaimer: All claims expressed in this article are solely those of the authors and do not necessarily represent those of their affiliated organizations, or those of the publisher, the editors and the reviewers. Any product that may be evaluated in this article or claim that may be made by its manufacturer is not guaranteed or endorsed by the publisher.
Research integrity at Frontiers
Learn more about the work of our research integrity team to safeguard the quality of each article we publish.