- 1Department of Veterinary Integrative Biosciences, College of Veterinary Medicine and Biomedical Sciences, Texas A&M University, College Station, TX, United States
- 2Department of Epidemiology and Biostatistics, School of Public Health, Texas A&M University, College Station, TX, United States
Introduction: Chagas disease, a zoonotic infection transmitted by triatomine bugs, poses serious public health risks in endemic areas. As dogs are important reservoirs in the disease’s spread, developing a canine vaccine could be transformative for controlling disease transmission to dogs and humans.
Methods: We developed a compartmental Susceptible-Infected model to simulate the transmission dynamics of Trypanosoma cruzi, considering interactions among dogs, humans, cats, rodents, and triatomine vectors. We used the model to assess the direct and indirect impacts of two vaccine mechanisms—all-or-nothing and leaky—on disease incidence across different host populations. The sensitivity of the model’s outcomes to changes in input parameters was analyzed using univariate sensitivity analysis.
Results: Our model showed that with a 90% vaccine efficacy, an all-or-nothing vaccine could reduce the cumulative incidence of T. cruzi in dogs by 91.3% over five years. The 60% and 30% vaccine efficacies would result in reductions of 63.47% and 33%, respectively, over 5 years. Similarly, the leaky vaccine achieved a 92.62% reduction in dog infections over 5 years with 90% efficacy. The indirect effects on human T. cruzi infection were notable; the all-or-nothing vaccine reduced human disease incidence by 14.37% at 90% efficacy, while the leaky vaccine achieved a 32.15% reduction over 5 years. Both vaccine mechanisms may substantially reduce T. cruzi incidence among dogs, and generate indirect benefit to other hosts, such as humans, by reducing their infection risk. The indirect benefits of vaccination were heavily influenced by the proportion of triatomine bugs blood meals taken from dogs.
Discussion: The study highlights the potential of targeted canine vaccination in controlling Chagas disease transmission and burden in endemic countries. It provides additional evidence for pursuing the development of a canine vaccine as a valuable tool for Chagas disease elimination.
Introduction
Vector-borne disease research has focused heavily on vaccine development as a pivotal tool for disease management and control. Identifying efficient vaccine targets is a key feature of the vaccine development process. Vector-borne infections, such as Chagas disease, canine leishmaniasis, yellow fever, and rabies are caused by several pathogens that present unique biological properties such as variability in genetic structure, antigenic diversity, and immune evasion potential. These properties can hinder the choice of the best vaccine targets (1–5). Understanding the mechanisms of protective immunity and identifying conserved antigens that can elicit robust and long-lasting immune responses are important aspects of vaccine development for vector-borne diseases. Vaccination could impact population demographics and life history traits of parasite populations, which may lead to diverse demographic and evolutionary outcomes. Vaccination aims to protect individuals from disease; in that sense, vaccine-mediated protection may greatly impact the prevalence of parasitic diseases. However, it could lead to disease elimination (6).
Recent progress in Chagas disease vaccine research has shown promising results, indicating a positive step towards a potential vaccine. However, the development of a vaccine for neglected tropical diseases such as Chagas disease still faces obstacles, particularly in securing political support and financial resources. Dogs have been identified as a key host for the Chagas parasite (Trypanosoma cruzi), playing a significant role in pathogen transmission to humans in various countries and epidemiological settings (7–11). Therefore, a vaccine that can prevent or treat T. cruzi infection in dogs may ultimately reduce or eliminate canine Chagas disease in endemic settings. This could have a significant impact on controlling the spread of the disease to humans and potentially other animal hosts. Despite ongoing efforts to develop vaccines for Chagas disease in dogs, no vaccine has yet been approved for commercial use (12–14).
Vaccines play a pivotal role in controlling vector-borne canine diseases, as demonstrated by the effectiveness of Leishmune and CaniLeish vaccines against canine leishmaniasis (1, 15). Vaccination serves as a vital tool for reducing disease transmission and infection in the case of Leishmania, offering an alternative to drastic measures like culling (3, 4, 16). Beyond leishmaniasis, host-targeted interventions, such as insecticide use, have proven effective in controlling diseases like plague, showcasing the importance of various approaches in disease control (16). Successful vaccination campaigns have also been observed for vector-borne human diseases such as yellow fever with vaccination significantly reducing its global burden (1, 2).
Mathematical modeling plays an important role in assessing the potential impact of vaccination for reducing the spread and burden of infectious diseases. Vaccines can generally be categorized into two types: leaky vaccine and all-or-nothing vaccine. Leaky vaccines are those for which vaccine-induced protection might reduce infection rates on a pre-exposure basis (17). These vaccines aim to save lives by reducing disease severity rather than achieving complete protection from infections. These vaccines are common for animal diseases (18). All-or-nothing vaccines are those for which vaccine-induced protection prevents infections. This describes a vaccine that either succeeds entirely or fails (17). Through simulated vaccine mechanisms, researchers can estimate the resulting impact of vaccination on disease burden and gauge the effectiveness and cost-effectiveness of vaccination programs.
In this paper, we developed a comprehensive model to assess the impact of potential canine vaccine mechanisms in reducing both human and animal cases of Chagas disease. This model is built on a previously published Chagas disease model (19). We used a compartmental Susceptible-Infected framework of T. cruzi transmission dynamics, incorporating interactions between triatomine bugs and host species: humans, dogs, cats, rodents, and avians. Drawing from established principles of vector-borne disease modeling, we integrate real-world data on host populations and triatomine bug behavior to estimate feeding rates and parasite transmission within the various hosts. We used the model to evaluate the impact of two vaccine mechanisms (leaky and all-or-nothing vaccine) for reducing T. cruzi infection incidence in a Chagas endemic setting. Through this modeling approach, we seek to shed light on the potential impact of a dog vaccine for controlling Chagas disease in humans and other non-human hosts.
Methods
We outlined the standard vector-borne modeling principles for SI (Susceptible-Infected) models as Susceptible for human, dog, cat, rodent, and avian respectively, and infected individuals of the human, dog, cat, and rodent populations of which they experience natural mortality at a rate respectively, and the decline in host population is offset by the entry of susceptible individuals at a constant rate for humans, dogs, cats, rodents and avians respectively. The death rate of each host species was calculated as the inverse of its average lifetime expectancy with values for human, dogs, avian, rodents and cats set to 70, 3, 0.5, 2 and 4 years, respectively. The birth rate for each species was computed under the assumption that each population had a constant population size. Susceptible and infected triatomine bugs undergo natural mortality at a per-capita rate . We incorporate a consistent recruitment rate that signifies triatomine bugs immigration in our model, encompassing susceptible and infected triatomine bugs. The count of triatomine bugs is estimated as the product of the fertility rate per blood meal and a per- triatomine bugs per-capita blood-feeding rate , accounting for competition among the triatomine bugs aiming to feed on humans, dogs, cats, rodents and avians. The rivalry among triatomine bugs to seek nourishment from their host species causes a decrease in the per capita blood-feeding rate of triatomine bugs as the proportion of triatomine bugs to host density rises. This adverse interaction has been elucidated by using a conventional density-dependent function (20): where represents the maximal triatomine bugs feeding rate, describes the intensity of the density-dependent regulation, and represents the ‘effective’ size of the host community. This ‘effective’ size of the community is defined as the sum of all host species (humans, dogs, cats, rodents, and avians) abundance weighted by triatomine bugs blood-feeding rates i.e., . The rates at which parasites are transmitted were also established based on the interaction patterns between triatomine bugs and hosts (humans, dogs, cats, and rodents), denoted respectively. The relative blood-feeding rates were estimated from the link between the proportion of blood-meals on each host type () and the host type population sizes . values are derived by solving following linear system of equations with (19). Additionally, the probabilities of parasite transmission were considered for each potential infectious interaction. This includes the probability of transmission per contact between a susceptible host and an infectious triatomine bugs, referred to as as well as the probability of transmission between a susceptible triatomine bugs and an infectious host, referred to as . The values of were estimated through model fitting to disease prevalence data (19). The model description through a flow diagram is presented in Figure 1.
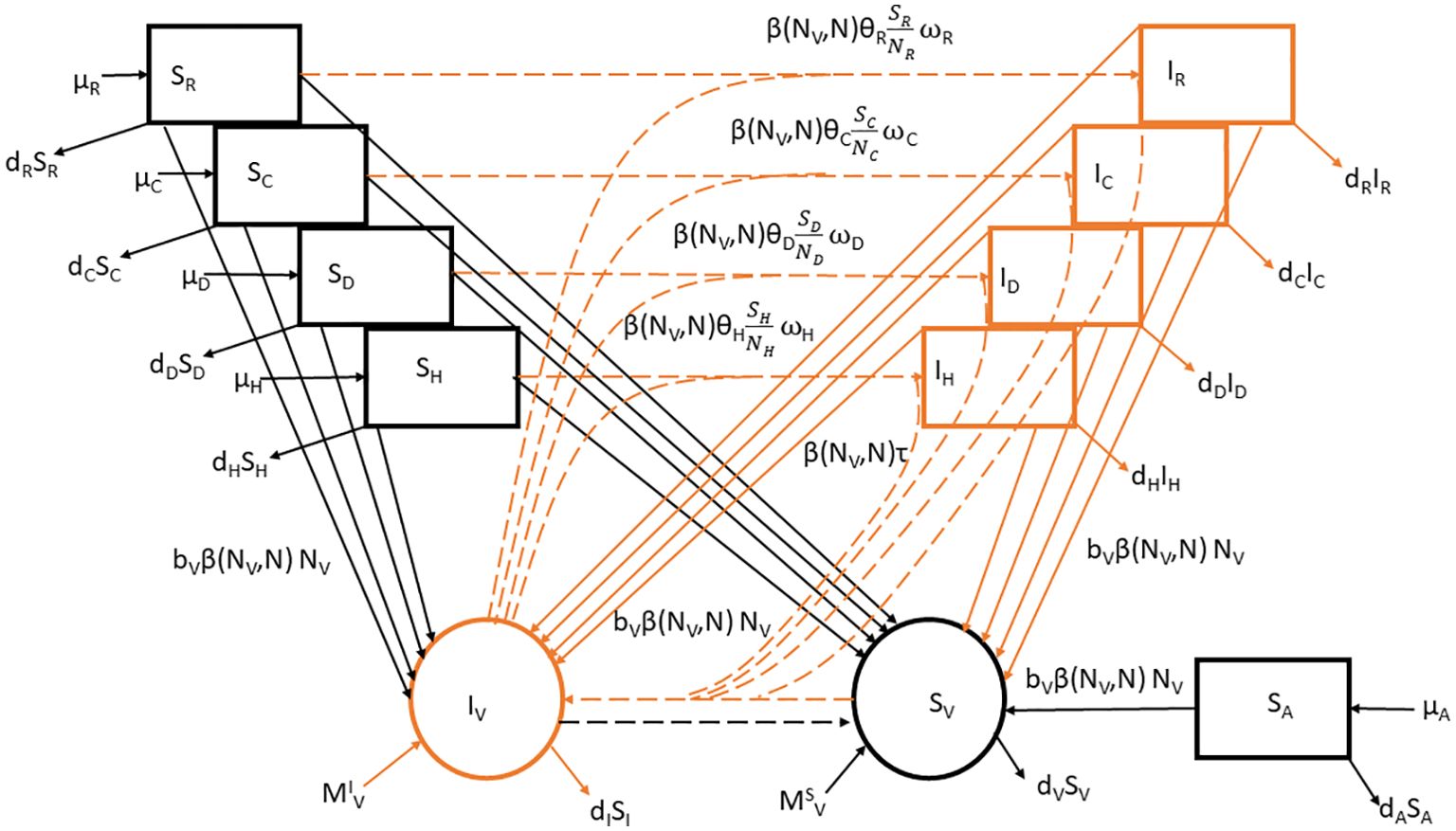
Figure 1. Flowchart for the SI model of T.cruzi transmission in hosts (humans, dogs, cats, rodents, and avian). The model comprises susceptible (black) and infected (orange) individuals. Arrows represent birth, death, migration, and infection processes according to the parameters in Table 1. The continuous and dotted lines correspond to demographic and T. cruzi transmission processes. Where . Species i= H, D, C, and R represent humans, dogs, cats, and rodents and ς is the set belonging to competent host species.
Base model:
Vaccine mechanisms
To assess the potential impact of a dog vaccine on Chagas disease, we explore two vaccine mechanisms: the all-or-nothing and leaky vaccines. An all-or-nothing vaccine confers complete protection against a specific disease or infection to a subset of vaccinated dogs, granting them full immunity while leaving the remainder susceptible to the disease as if they had not been vaccinated. In contrast, a leaky vaccine provides partial protection to all vaccinated dogs, reducing the risk of infection or the severity of the disease in case of infection. Unlike all-or-nothing vaccines, leaky vaccines do not establish a clear division between fully protected and entirely susceptible individuals. Instead, they provide degrees of protection to all vaccinated dogs.
Scenario 1: All-or-nothing vaccine
We used a straightforward transmission model to examine T. cruzi infections in domestic dogs and their indirect impacts on humans and other non-human hosts species. The dog population is grouped into Susceptible, Infectious, and Vaccinated groups. Dogs enter this population at a rate , with a fraction of the entering dogs effectively vaccinated. The fraction represents the vaccine efficacy. Vaccinated dogs become immune to infection and are assigned to the category, while the remaining fraction of entering dogs remain susceptible and are placed in the category. For simplification, we assume that all dogs are vaccinated. This yields the following differential equations.
Here ϵ is the efficacy of the vaccine. is the compartment that obtains full immunity protection from the vaccine.
Scenario 2: Leaky vaccine
We assume that vaccines reduce the susceptibility and infectivity of vaccinated dogs to T. cruzi infection. Therefore, if vaccinated dogs become infected, their capacity to transmit the pathogen will be diminished. The impact of the vaccine mechanism is reflected in the adjustment of susceptibility and infectivity among vaccinated dogs within the epidemiological framework. Here, vaccination has the potential to mitigate T. cruzi transmission by either reducing the susceptibility of dogs to T. cruzi infection denoted by parameter , or by decreasing the infectivity of infected dogs to transmitting T. cruzi, indicated by parameter . The progression of vaccinated dogs across distinct epidemiological categories under the influence of leaky vaccine mechanism can be elucidated through the following system of differential equations.
Endemic values of T. cruzi among triatomine bugs and hosts
Our parameterized model generated the following endemic prevalence of T. cruzi within the studied population. With triatomine bugs showing a prevalence of 30%, dogs at 25%, cats at 24%, rodents at 13%, and humans at 7%, these findings highlight the significant presence of T. cruzi across multiple host species in the research area. The varying disease prevalence among the host populations underscores the complex interactions between host species and triatomine bugs. This baseline prevalence serves as a benchmark against which the impact of vaccine intervention strategies was evaluated. By comparing the incidence of T. cruzi among hosts before and after the implementation of vaccination mechanisms, we aim to assess the potential impact of these interventions in reducing T. cruzi incidence and ultimately mitigating the burden of Chagas disease within the studied population.
Sensitivity analysis
To investigate the effect of input parameters on the potential impact of a dog vaccine for reducing Chagas disease incidence, we conducted univariate sensitivity analysis on key epidemiological parameters derived from published literature: the proportion of triatomine bugs’ blood meals in dogs, triatomine bug migration, maximal vector feeding rate, density-dependent regulation of triatomine feeding, probability of vector infection from host, triatomine fertility per blood-meal, triatomine bugs death rate. For each parameter we assumed a fixed percentage increase or decrease of the default parameter value. Regarding the proportion of triatomine bugs’ blood meal on dogs, we only alter the proportion of triatomine bugs’ blood meals on dogs and humans, while keeping the proportion for other host species unchanged. This decision was made for simplicity. This was done by increasing/reducing the blood meal proportions on dogs and adjusting the proportion of blood meal on humans accordingly to maintain overall balance. For a given percentage change (reduction/increase) in the proportion of triatomine bugs’ blood meal on dogs (*(percentage change)), the proportion of blood meal on humans was adjusted as follows: (*(percentage change)).
Results
All-or-nothing vaccine mechanism
In Figure 2, we explored the impact of varying vaccine efficacy on reducing disease incidence in host populations (humans, dogs, cats, and rodents) through continuous vaccination of dogs. We observed a substantial reduction in disease incidence across the host populations, with higher vaccine efficacy leading to more incidence reductions, particularly in dog populations. For a 90% vaccine efficacy, continuous vaccination over 5 years results in a 91.3% reduction in cumulative T. cruzi infection incidence among dogs (Figure 2). However, with a 60% and 30% vaccine efficacy, the cumulative incidence of T. cruzi infection among dogs is reduced by 63.47% and 33.00%, respectively, over 5 years (Figure 2). Similar qualitative results are observed for the reduction of T. cruzi infection incidence among humans, cats, and rodents (Figure 2). At 90% vaccine efficacy, the cumulative incidence in humans is reduced by 14.37%, and declines to 9.60% and 4.80% at 60% and 30% vaccine efficacy, respectively. For rodents, the cumulative incidence of T. cruzi infection is reduced by 13.62%, 9.05%, and 4.5% at efficacy levels of 90%, 60%, and 30%, respectively. For cats, the cumulative incidence of T. cruzi infection is reduced by 13.39%, 8.88%, and 4.41% at efficacy levels of 90%, 60%, and 30%, respectively. However, the impact of dog vaccination for reducing T. cruzi infection incidence among humans, cats, and rodents was observed to increase substantially with the duration of the vaccination program (Figure 2).
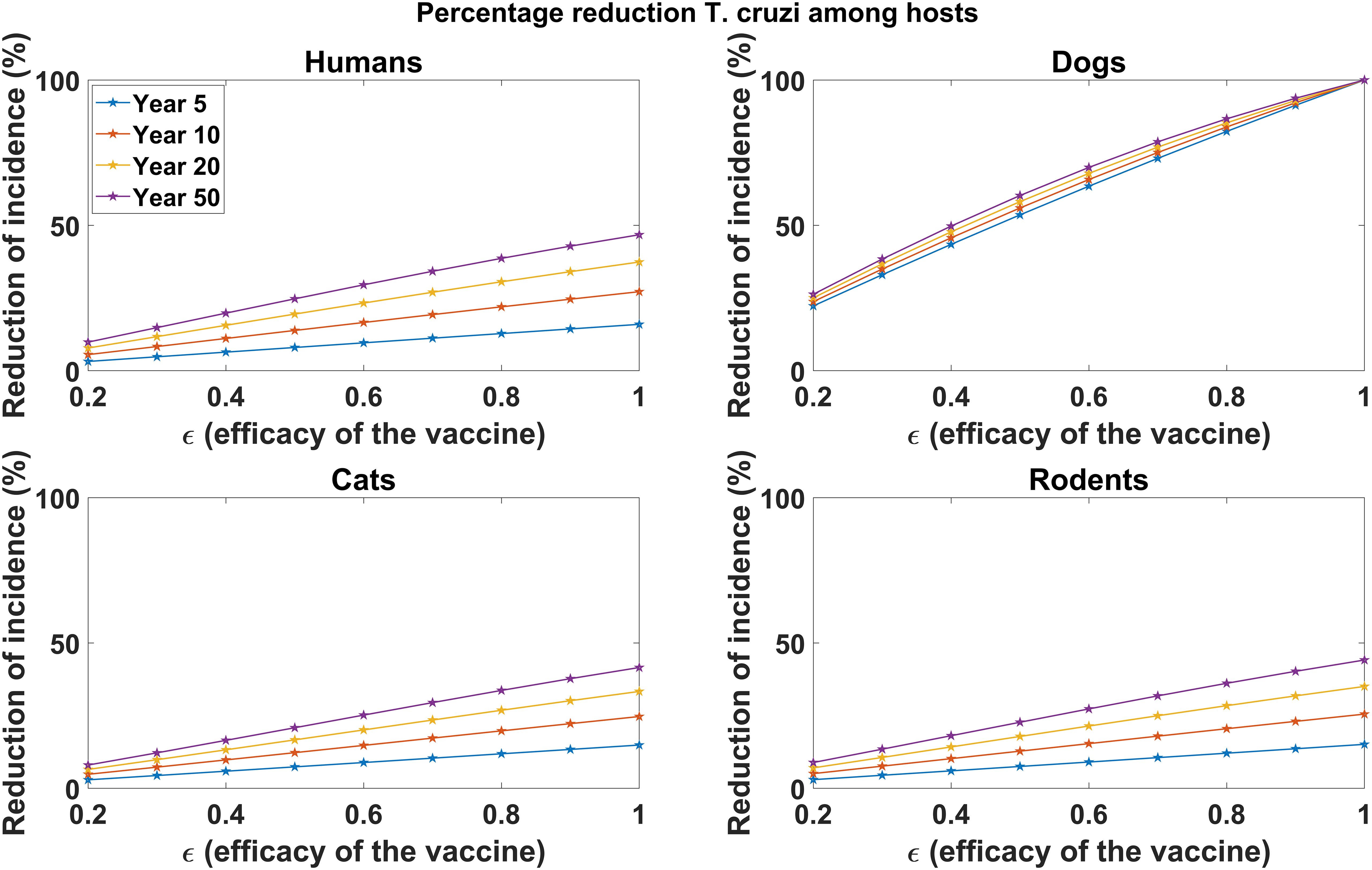
Figure 2. Impact of vaccine efficacy on T. cruzi incidence reduction across host populations. The impact of the vaccine for varying efficacy levels was measured as the proportional reduction of cumulative infection incidence in each host population through continuous vaccination of dogs over 5, 10, 20, and 50 years.
Leaky vaccine mechanism
In Figure 3, we explored the effect of varying vaccine efficacy on reducing T. cruzi infection incidence in host populations (humans, dogs, cats, and rodents). Our analysis showed considerable reductions in disease incidence across all host populations, with higher vaccine efficacy leading to greater reductions, particularly among dog populations. For instance, a 90% vaccine efficacy yields a 92.62% reduction in cumulative T. cruzi infection incidence among dogs over a 5-year vaccination program (Figure 3). For 60% and 30% vaccine efficacy, the cumulative incidence of T. cruzi infection among dogs is reduced by 65.92% and 35.08%, respectively, over the same 5-year period (Figure 3). Similar qualitative results are observed for the reduction of T. cruzi infection incidence among humans, cats, and rodents (Figure 3). At 90% vaccine efficacy, the cumulative incidence in humans is reduced by 32.15%, with reductions declining to 23.47% and 12.62% at 60% and 30% vaccine efficacy, respectively. Among rodents, the cumulative incidence of T. cruzi infection is reduced by 30.31%, 12.97%, and 11.69% at efficacy levels of 90%, 60%, and 30%, respectively. Likewise, for cats, the cumulative incidence of T. cruzi infection sees reductions of 30.01%, 21.73%, and 11.55% at efficacy levels of 90%, 60%, and 30%, respectively. As observed with the all-or-nothing vaccine mechanism, the impact of dog vaccination in reducing T. cruzi infection incidence among humans, cats, and rodents was shown to increase with the duration of the vaccination program (Figure 3).
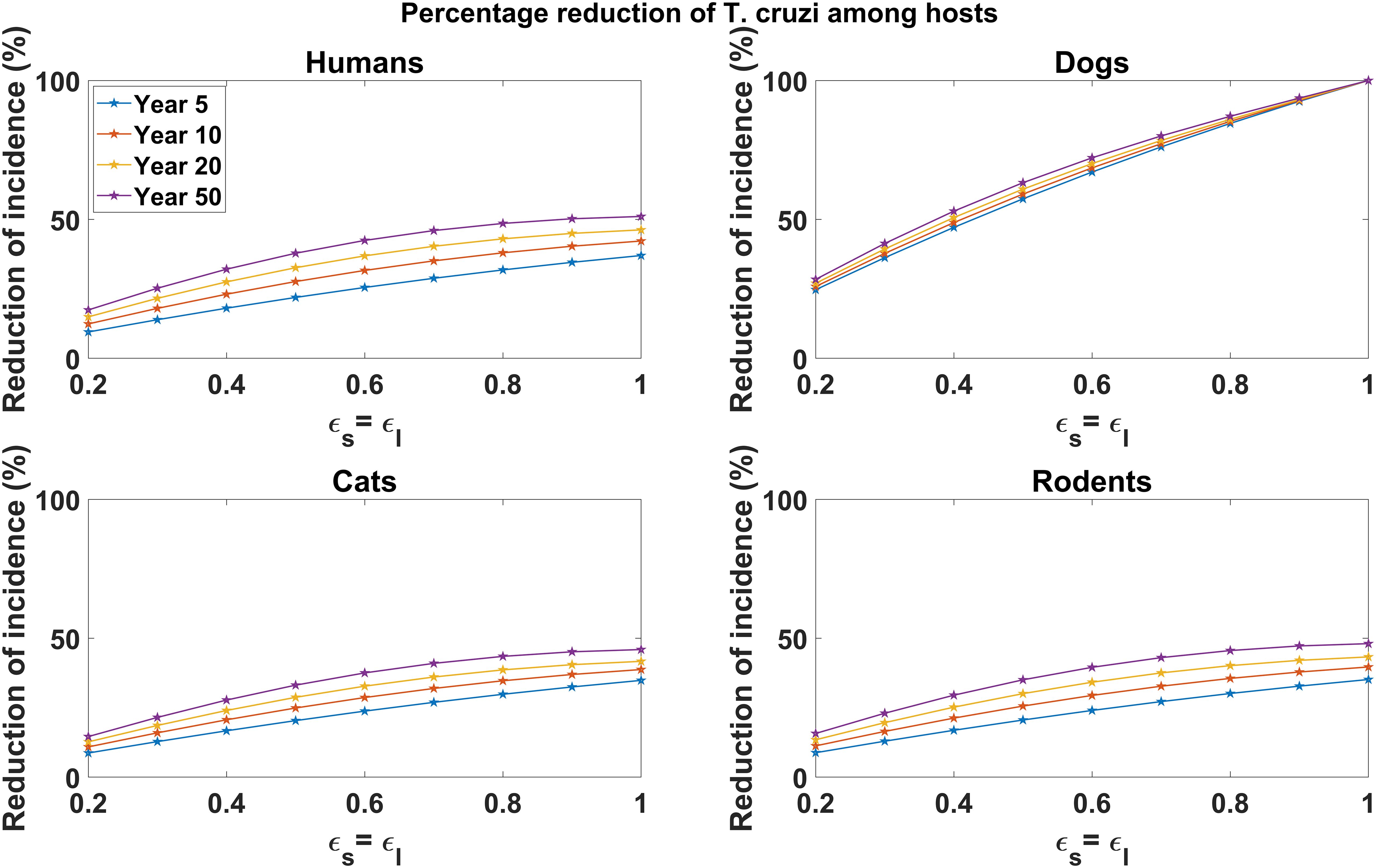
Figure 3. Impact of leaky vaccine efficacy on T. cruzi incidence reduction across host populations. The impact of the vaccine for varying efficacy levels was measured as the proportional reduction of cumulative infection incidence in each host population through continuous vaccination of dogs over 5, 10, 20, and 50 years. For simplicity, we set equal values for vaccine efficacy for reducing susceptibility and efficacy for reducing infectivity.
Sensitivity analysis
We investigated the effect of the proportion of triatomine bugs’ blood meal on dogs on the impact of dog vaccination for reducing T. cruzi infection among the different hosts. For the all-or-nothing vaccine mechanism, we showed that changes in the proportion of triatomine bugs’ blood meal on dogs have minimal effect on reducing T. cruzi infection among dogs (Figure 4). For each host species (humans, cats, and rodents), we showed that an increase/decrease in the proportion of triatomine bugs’ blood meal on dogs would increase/decrease the potential impact of dog vaccination on reducing T. cruzi infection, respectively (Figure 4). The magnitude of this impact was shown to increase with vaccine efficacy. For example, for a 60% vaccine efficacy, a 50% increase/decrease in the proportion of triatomine bugs’ blood meal on dogs increases/decreases the potential impact of vaccination on reducing cumulative T. cruzi infection incidence among humans over 10 years from 16.59% to 22.79%/5.98%, respectively (Figure 4). For a 90% vaccine efficacy, a 50% increase/decrease in the proportion of triatomine bugs’ blood meal on dogs increases/decreases the potential impact of vaccination on reducing cumulative T. cruzi infection incidence among humans over 10 years from 24.61% to 34.58%/8.91%, respectively (Figure 4).
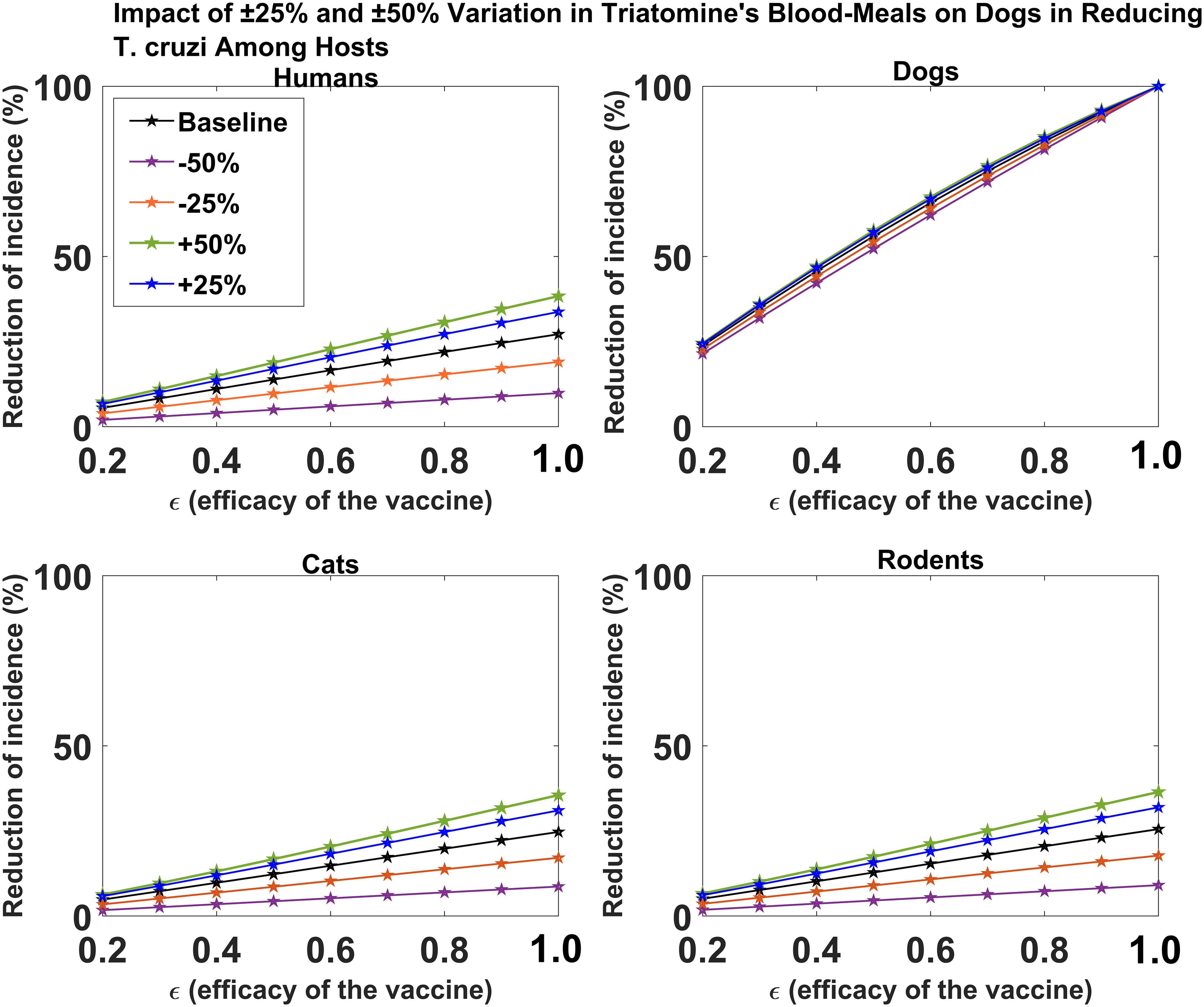
Figure 4. Impact of vaccine efficacy on T. cruzi incidence reduction across host populations for varying proportions of triatomine blood meal on dogs. The impact of the vaccine for varying efficacy levels was measured as the proportional reduction of cumulative infection incidence in each host population through continuous vaccination of dogs over 10 years.
For the leaky vaccine mechanism, we showed that changes in the proportion of triatomine bugs’ blood meal on dogs have minimal effect on reducing T. cruzi infection among dogs (Figure 5). For each host species (humans, cats, and rodents), we showed that an increase/decrease in the proportion of triatomine bugs’ blood meal on dogs would increase/decrease the potential impact of dog vaccination on reducing T. cruzi infection, respectively (Figure 5). The magnitude of this impact was shown to increase with vaccine efficacy. For example, for a 60% vaccine efficacy, a 50% increase/decrease in the proportion of triatomine bugs’ blood meal on dogs increases/decreases the potential impact of vaccination on reducing cumulative T. cruzi infection incidence among humans over 10 years from 31.64% to 44.54%/7.90%, respectively (Figure 5). For a 90% vaccine efficacy, a 50% increase/decrease in the proportion of triatomine bugs’ blood meal on dogs increases/decreases the potential impact of vaccination on reducing cumulative T. cruzi infection incidence among humans over 10 years from 40.36% to 58.01%/10.26%, respectively (Figure 5).
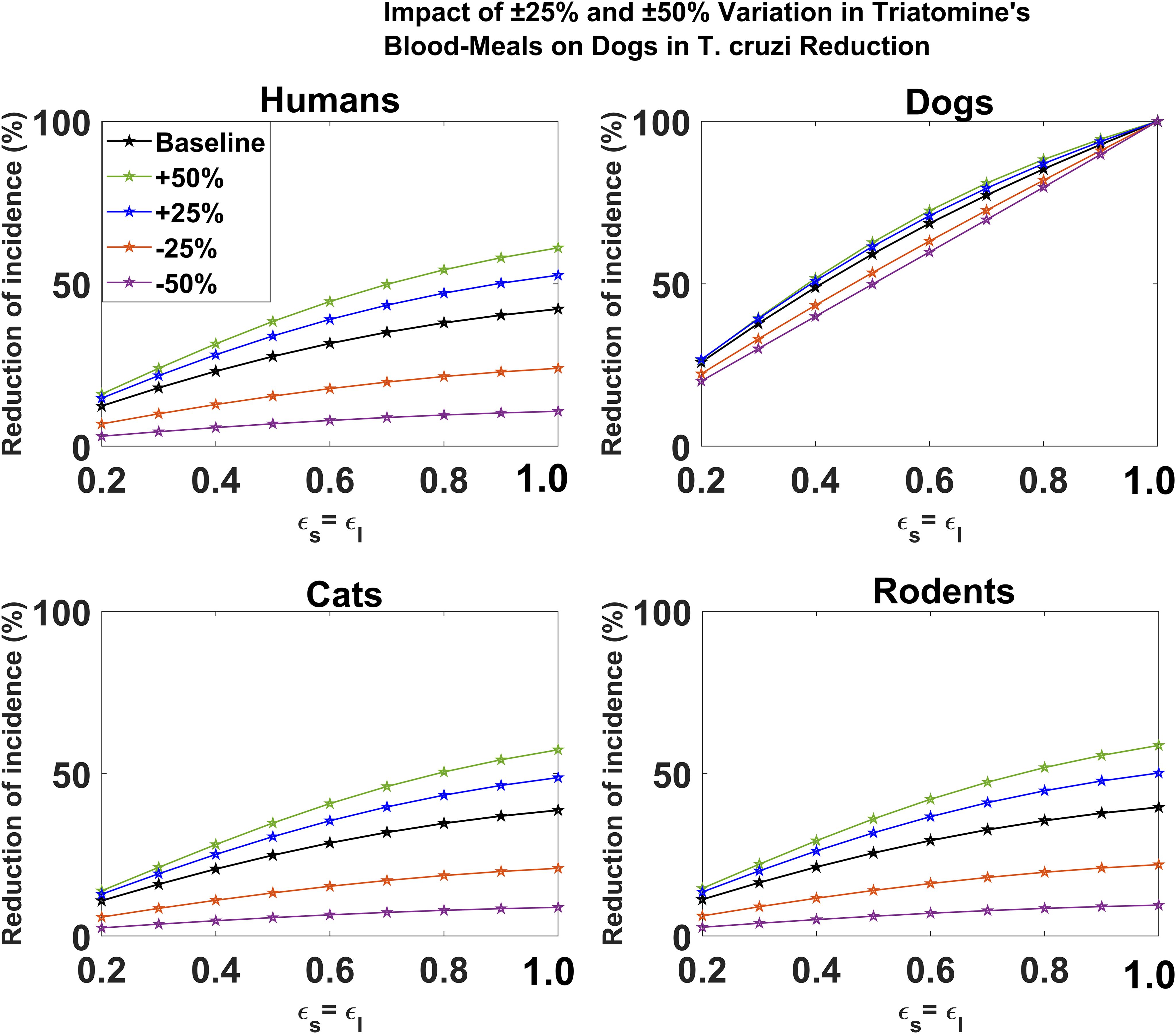
Figure 5. Impact of leaky vaccine efficacy on T. cruzi incidence reduction across host populations for varying proportions of triatomine blood meal on dogs. The impact of the vaccine for varying efficacy levels was measured as the proportional reduction of cumulative infection incidence in each host population through continuous vaccination of dogs over 10 years. For simplicity, we set equal values for vaccine efficacy for reducing susceptibility and efficacy for reducing infectivity.
We further investigated the effect of variations in critical epidemiological transmission parameters on the impact of vaccination strategies for reducing T. cruzi infection in the human population. For both the all-or-nothing and leaky vaccine mechanisms, we found that changes in these parameters had varying effects on the reduction of T. cruzi infection, depending on the parameter and the vaccine efficacy level, as outlined below.
Maximal vector feeding rate
Variations in the maximal vector feeding rate have a significant impact on the impact of vaccines in reducing T. cruzi transmission. For the all-or-nothing vaccine mechanism, at 60% vaccine efficacy, a 50% increase/decrease in the maximal vector feeding rate reduces the potential impact of vaccination on cumulative T. cruzi infection incidence among humans over 10 years from 16.6% to 12.5%/14.8%, respectively (Figure 6). Similarly, at 90% vaccine efficacy, a 50% increase or decrease in the feeding rate reduces the impact from 24.6% to 19.3%/21.2%, respectively. These results are consistent with those observed for the leaky vaccine mechanism (Figure 7).
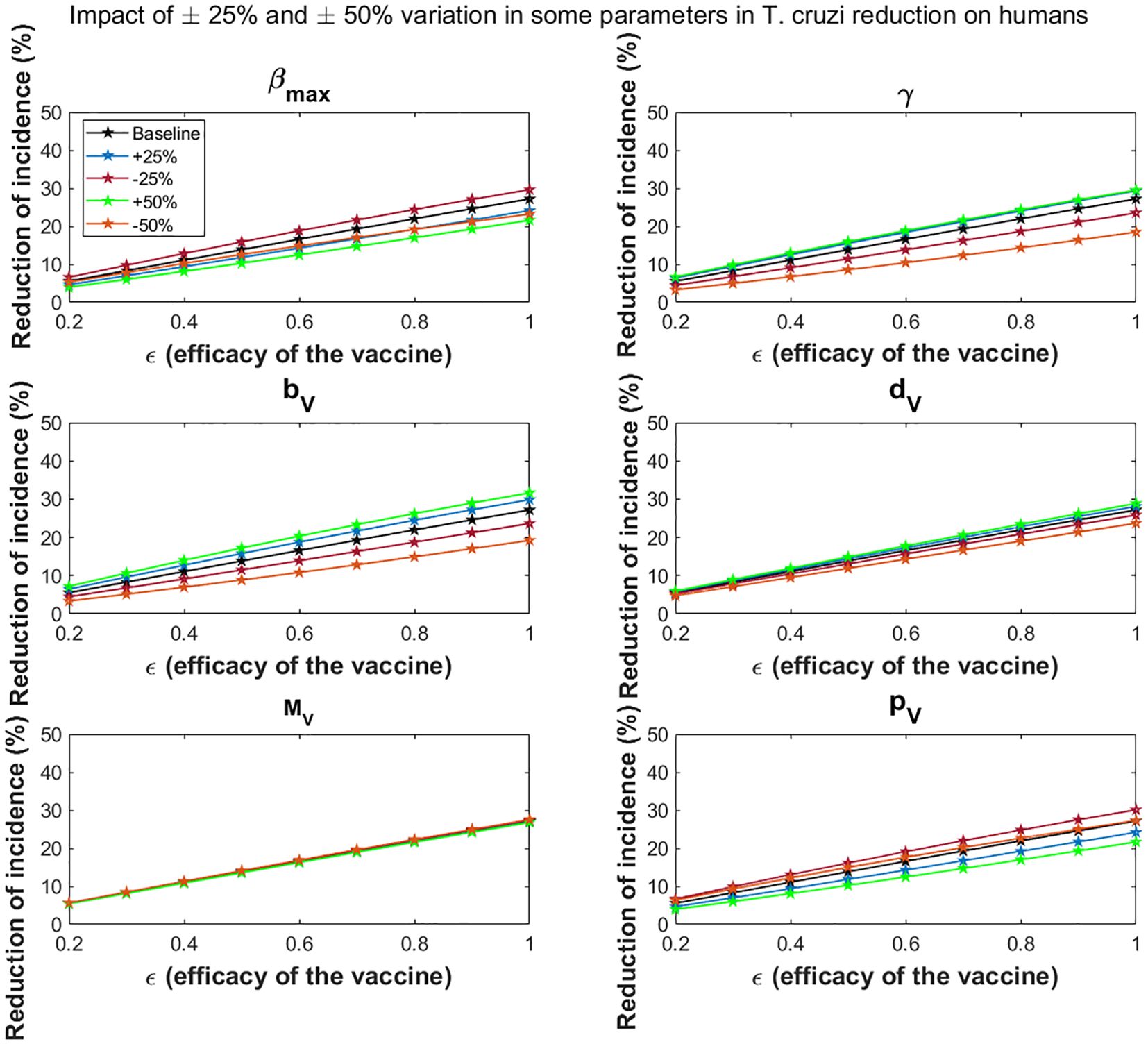
Figure 6. Impact of all-or- nothing vaccine efficacy on T. cruzi incidence reduction in populations for varying some key parameters. The impact of the vaccine for varying efficacy levels was measured as the proportional reduction of cumulative infection incidence in the human population through continuous vaccination of dogs over 10 years. For simplicity, we set equal values for vaccine efficacy for reducing susceptibility and efficacy for reducing infectivity.
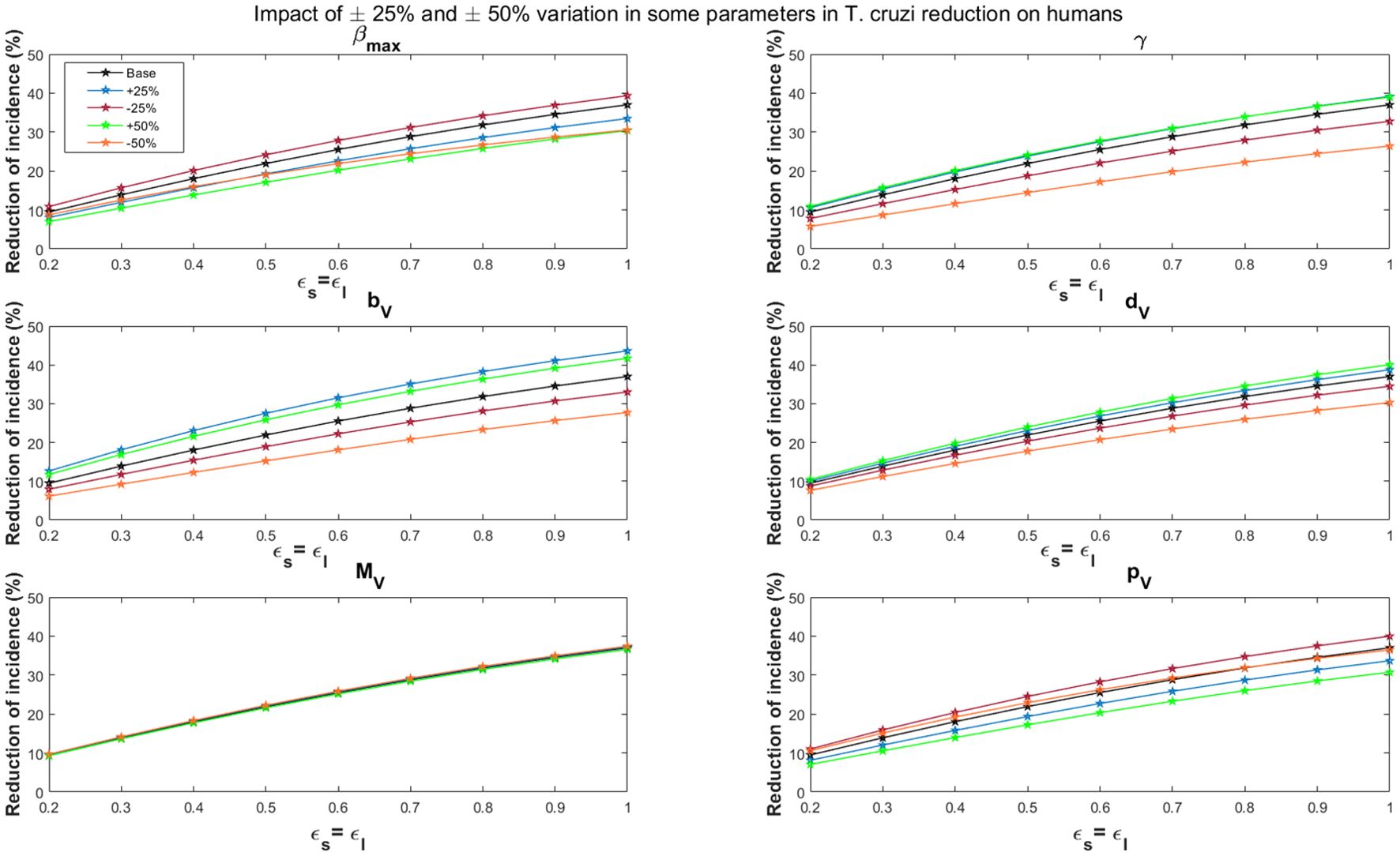
Figure 7. Impact of leaky vaccine efficacy on T. cruzi incidence reduction in populations for varying some key parameters. The impact of the vaccine for varying efficacy levels was measured as the proportional reduction of cumulative infection incidence in the human population through continuous vaccination of dogs over 10 years. For simplicity, we set equal values for vaccine efficacy for reducing susceptibility and efficacy for reducing infectivity.
Dependent regulation of triatomine feeding (γ)
Changes in density-dependent regulation of triatomine feeding significantly affect the vaccine’s impact, particularly as vaccine efficacy increases. For a 60% vaccine efficacy, a 50% increase or decrease in this parameter raises or lowers the potential impact of vaccination on cumulative T. cruzi infection incidence among humans over 10 years from 16.6% to 18.9%/10.4%, respectively (Figure 6). At 90% efficacy, a 50% increase or decrease alters the impact from 24.6% to 27.0%/16.4%, respectively. This trend is similarly observed for the leaky vaccine mechanism (Figure 7).
Triatomine fertility per blood meal
The fertility rate of triatomines per blood meal plays a critical role in the overall impact of vaccines. The impact of vaccination on T. cruzi infection becomes more pronounced with changes in triatomine fertility rates as vaccine efficacy increases. For a 60% vaccine efficacy, a 50% increase/decrease in fertility rates changes the cumulative infection reduction from 16.6% to 20.4%/10.9%, respectively (Figure 6). With a 90% vaccine efficacy, a similar increase/decrease alters the impact from 24.6% to 29.0%/17.1%, respectively. The leaky vaccine mechanism shows similar sensitivity to changes in fertility (Figure 7).
Triatomine bug death rate
Variations in the death rate of triatomine bugs affect the impact of vaccines, especially as vaccine efficacy increases. For a 60% vaccine efficacy, a 50% increase or decrease in the death rate changes the cumulative T. cruzi infection incidence reduction from 16.6% to 17.8% and 14.3%, respectively (Figure 6). At a 90% vaccine efficacy, the impact adjusts from 24.6% to 26.2%/21.4% with a 50% increase or decrease in the death rate. This pattern is consistent across the leaky vaccine mechanism (Figure 7).
Triatomine bug migration
Changes in triatomine bug migration have a relatively minor effect on the overall impact of vaccination. For a 60% vaccine efficacy, a 50% increase/decrease in triatomine bug migration results in slight changes in the vaccination’s effectiveness, with cumulative T. cruzi infection incidence among humans varying from 16.6% to 16.3%/17.0%, respectively (Figure 6). At 90% vaccine efficacy, the impact decreases to 24.2%/increases to 25.0% with a 50% change in migration rates. This trend was also observed for the leaky vaccine mechanism (Figure 7).
Probability of vector infection from host
The probability of vector infection from hosts plays a crucial role in determining the vaccine’s impact on reducing T. cruzi transmission. For a 60% vaccine efficacy, a 50% increase/decrease in the probability of vector infection decreases/increases the cumulative infection incidence reduction from 16.6% to 12.5%/17.7%, respectively (Figure 6). At 90% vaccine efficacy, a 50% change in this probability reduces the impact to 19.3%/increases it to 25.0%. These results mirror those found with the leaky vaccine mechanism (Figure 7).
Discussion
In this study, a dynamical model for T. cruzi transmission was developed to evaluate the potential impact of a canine vaccine in controlling Chagas disease in endemic settings. The model considers the interactions among multiple host species and the triatomine bugs. The findings emphasize the role of dogs as significant reservoirs in the disease ecology, making them an ideal target for vaccination. This dog-targeted control approach builds on the concept of using a reservoir-targeted measure to control zoonotic diseases. Similar strategies have been employed for other diseases, such as rabies and canine leishmaniasis, where vaccination of animal reservoirs led to decreased disease incidence in human populations (4, 27).
To evaluate the impact of a canine vaccine on Chagas disease, the study considered two vaccine mechanisms: all-or-nothing and leaky vaccine mechanisms. For each vaccine mechanism, the study evaluated varying levels of vaccine efficacy. In addition, the study evaluated how triatomine bugs’ feeding preference could impact vaccination outcomes. For the all-or-nothing vaccine mechanism, the study showed a robust potential to reduce the transmission of T. cruzi among dogs, especially under high vaccine efficacy. Dog vaccination also provides indirect protection for other hosts by reducing the infection pressure from triatomine. However, the benefit of this indirect protection substantially increases with the duration of the vaccination program and vaccine efficacy. The effect of this indirect benefit was shown to be highly dependent on the vaccine efficacy rate and the proportion of triatomine bugs’ blood meals on dogs. On the other hand, the leaky vaccine mechanism demonstrated a more consistent reduction in disease transmission among hosts for vaccine efficacy above 70%. Its indirect benefit was shown to be less sensitive to the duration of the vaccination program.
Sensitivity analysis was used to investigate the effect of the proportion of triatomine bugs’ blood meals on dogs on the potential impact of canine vaccine for Chagas disease control. It provided critical insights into the interdependencies within the transmission dynamics of T. cruzi among host species. The study findings demonstrate that the potential impact of canine vaccines for reducing T. cruzi infection among humans is highly dependent on the triatomine bugs’ feeding patterns on hosts, especially dogs. For instance, increasing the proportion of triatomine bugs’ blood meals on dogs generally enhances the impact of the canine vaccine on reducing T. cruzi infection across all hosts, including humans, cats, and rodents. So, settings with high triatomine bugs’ feeding rates on dogs would experience a higher interruption of T. cruzi transmission cycles than settings with lower feeding rates. Conversely, decreasing the proportion of triatomine blood meals on dogs decreases the potential vaccine’s impact by dispersing the pathogen’s transmission routes more broadly among other host populations, thus reducing the canine vaccine’s protective impact on other hosts. However, the direct benefit of dog vaccination for reducing infection among the dog population was marginally affected by triatomine bug feeding preference. Our sensitivity analysis underscores the importance of integrated approaches in managing Chagas disease. The potential impact of dog vaccination in reducing T. cruzi infection in humans is influenced by various epidemiological parameters. All-or-nothing and leaky vaccine mechanisms are affected by changes in triatomine behaviors and host interactions. The decrease in vaccination’s impact with increasing triatomine feeding rates (28) and changes in density-dependent regulation (29) suggest that vector-host dynamics substantially influence transmission. This emphasizes the importance of considering triatomines behaviors in vaccination strategies and integrating vector population control measures to enhance vaccination efficacy (30). The results also show that high triatomine fertility and triatomine survival rates intensify transmission dynamics, highlighting the potential benefits of interventions targeting triatomine populations. Additionally, the relatively minor effects of triatomine migration on transmission underscore the significance of localized vector control efforts (31). Finally, variations in the probability of triatomine infection from hosts underscore the complexity of transmission in multi-host environments. Reducing infection rates in key reservoir hosts like dogs can have a cascading effect on human infection reduction (32). Overall, the study aligns with the need for integrated approaches in managing Chagas disease, as emphasized in previous literature (33).
It is important to acknowledge that while the model simplifies complex interactions among hosts and triatomine bugs and assumes a degree of homogeneity in host susceptibility and triatomine bugs behavior, these limitations do not undermine the valuable insights provided by this Chagas disease vaccine mechanism modeling. A substantial limitation is that the model needs to account for the potential role of other host species, such as goats, pigs and wildlife, which serve as essential reservoirs in various transmission settings. In regions like Argentina, Brazil, and Peru, the contribution of synanthropic rodents, guinea pigs, and sylvatic-domestic cycles—where dogs hunt infected wildlife and become infected via sylvatic vectors—is a critical factor that could affect transmission dynamics. The assumptions of constant epidemiological parameters’ values, although not capturing the full variability of real-world dynamics, are necessary to maintain computational feasibility and focus the analysis on the potential impacts of different vaccine mechanisms. Additionally, by not incorporating some economic and social factors, the analysis remains focused on the epidemiological impact of vaccination. These simplifications enable the model to provide clear, actionable insights into how different vaccine mechanisms could potentially reduce the transmission of T. cruzi, despite its inherent constraints. The models provide a foundational tool for guiding initial public health decisions and pinpointing crucial areas for further research.
Conclusion
The study evaluates the potential impact of a canine vaccine against Chagas disease transmission in endemic settings, using mathematical modeling to simulate an all-or-nothing and leaky vaccine mechanism. Findings show that both vaccine mechanisms can substantially reduce T. cruzi transmission in dogs and other hosts. The indirect benefit of dog vaccination for reducing infection in other hosts, such as humans, was shown to vary substantially with vaccine efficacy and triatomine bugs’ feeding patterns. These results highlight the critical role of the ecological and behavioral dynamics of triatomine bugs on Chagas disease transmission and control. Although the benefit of a canine vaccine against human Chagas disease may vary greatly depending on the vaccine mechanism and the setting, the study findings provide critical insights into the potential impact of a canine vaccine on reducing Chagas disease. However, vaccinating only dogs reduces the T. cruzi burden but may have limited effects if triatomines switch to other hosts. Since triatomines may change their feeding preferences when vaccinated dogs become less suitable hosts, control programs should target multiple reservoir species, including other domestic animals, to maximize the reduction in human infections.
Data availability statement
The original contributions presented in the study are included in the article/supplementary material, further inquiries can be directed to the corresponding author/s.
Author contributions
EF: Formal analysis, Investigation, Software, Writing – original draft, Writing – review & editing. AD: Methodology, Software, Writing – original draft, Writing – review & editing. MN-M: Conceptualization, Funding acquisition, Methodology, Software, Supervision, Validation, Writing – original draft, Writing – review & editing.
Funding
The author(s) declare financial support was received for the research, authorship, and/or publication of this article. The authors declare financial support was received for the research, authorship, and/or publication of this article. This work is funded through a grant awarded to MLNM from Texas A&M AgriLife Research and the United States Department of Agriculture (APHIS-USDA AP23OA000000C013).
Conflict of interest
The authors declare that the research was conducted in the absence of any commercial or financial relationships that could be construed as a potential conflict of interest. The author's declared that they were an editorial board member of Frontiers, at the time of submission. This had no impact on the peer review process and the final decision.
Publisher’s note
All claims expressed in this article are solely those of the authors and do not necessarily represent those of their affiliated organizations, or those of the publisher, the editors and the reviewers. Any product that may be evaluated in this article, or claim that may be made by its manufacturer, is not guaranteed or endorsed by the publisher.
References
1. Palatnik-de-Sousa CB. Vaccines for leishmaniasis in the fore coming 25 years. Vaccine. (2008) 26:1709–24. doi: 10.1016/j.vaccine.2008.01.023
2. Monath TP. Yellow fever vaccine. Expert Rev Vaccines. (2005) 4:553–74. doi: 10.1586/14760584.4.4.553
3. Alvar J, Cañavate C, Molina R, Moreno J, Nieto J. Canine leishmaniasis. Adv Parasitol. (2004) 57:1–88. doi: 10.1016/S0065-308X(04)57001-X.
4. Costa DNCC, Codeço CT, Silva MA, Werneck GL. Culling dogs in scenarios of imperfect control: realistic impact on the prevalence of canine visceral leishmaniasis. PloS Negl Trop Dis. (2013) 7:e2355. doi: 10.1371/journal.pntd.0002355
5. Halloran ME, Longini IM Jr. Emerging, evolving, and established infectious diseases and interventions. Science. (2014) 345:1292–4. doi: 10.1126/science.1254166
6. Fenner F, Henderson DA, Arita I, Jezek Z, Ladnyi ID. Smallpox and its eradication. Geneva, Switzerland: World Health Organization (1988).
7. Tenney TD, Curtis-Robles R, Snowden KF, Hamer SA. Shelter Dogs as Sentinels for Trypanosoma cruzi Transmission across Texas. Emerging Infect Dis J. (2014). 20(8):1323–6. doi: 10.3201/eid2008.131843
8. Mott KE, Mota EA, Sherlock I, Hoff R, Muniz TM, Oliveira TS, et al. Trypanosoma cruzi infection in dogs and cats and household seroreactivity to T. Cruzi in a rural community in northeast Brazil. Am J Trop Med Hyg. (1978) 27:1123–7. doi: 10.4269/ajtmh.1978.27.1123
9. Gürtler RE, Cecere MC, Lauricella MA, Cardinal MV, Kitron U, Cohen JE. Domestic dogs and cats as sources of Trypanosoma cruzi infection in rural northwestern Argentina. Parasitology. (2007) 134:69–82. doi: 10.1017/S0031182006001259
10. Montenegro VM, Jiménez M, Dias JCP, Zeledón R. Chagas disease in dogs from endemic areas of Costa Rica. Mem Inst Oswaldo Cruz. (2002) 97:491–4. doi: 10.1590/S0074-02762002000400006
11. Díaz ML, Leal S, Mantilla JC, Molina-Berríos A, López-Muñoz R, Solari A, et al. Acute chagas outbreaks: molecular and biological features of Trypanosoma cruzi isolates, and clinical aspects of acute cases in Santander, Colombia. Parasit Vectors. (2015) 8:1–14. doi: 10.1186/s13071-015-1218-2
12. Basombrio MA, Segura MA, Mora MC, Gomez L. Field trial of vaccination against american trypanosomiasis (Chagas’ Disease) in dogs. Am J Trop Med Hyg. (1993) 49:143–51. doi: 10.4269/ajtmh.1993.49.143
13. Quijano-Hernández IA, Castro-Barcena A, Vázquez-Chagoyán JC, Bolio-González ME, Ortega-López J, Dumonteil E. Preventive and therapeutic DNA vaccination partially protect dogs against an infectious challenge with Trypanosoma cruzi. Vaccine. (2013) 31:2246–52. doi: 10.1016/j.vaccine.2013.03.005
14. Travi BL. Considering dogs as complementary targets of chagas disease control. Vector Borne Zoonotic Dis. (2019) 19:90–4. doi: 10.1089/vbz.2018.2325
15. Costa DNCC, Codeço CT, Silva MA, Werneck GL. Culling dogs in scenarios of imperfect control: realistic impact on the prevalence of canine visceral leishmaniasis. PloS Negl Trop Dis. (2013) 7:e2355. doi: 10.1371/journal.pntd.0002355
16. Palatnik-de-Sousa CB. Vaccines for canine leishmaniasis. Front Immunol. (2012) 3:69. doi: 10.3389/fimmu.2012.00069
17. Halloran ME, Haber M, Longini IM. Interpretation and estimation of vaccine efficacy under heterogeneity. Am J Epidemiol. (1992) 136:328–43. doi: 10.1093/oxfordjournals.aje.a116498
18. Kupferschmidt K. Risk of “leaky” vaccines debated. Science. (2015) 349:461–2. doi: 10.1126/science.349.6247.461
19. Flores-Ferrer A, Waleckx E, Rascalou G, Dumonteil E, Gourbière S. Trypanosoma cruzi transmission dynamics in a synanthropic and domesticated host community. PloS Negl Trop Dis. (2019) 13:e0007902. doi: 10.1371/journal.pntd.0007902
20. Maynard Smith J, Slatkin M. The stability of predator-prey systems. In: Ecology, (1973) 54:384–91.
21. Dumonteil E, Nouvellet P, Rosecrans K, Ramirez-Sierra MJ, Gamboa-León R, Cruz-Chan V, et al. Eco-bio-social determinants for house infestation by non-domiciliated triatoma dimidiata in the yucatan peninsula, Mexico. PloS Negl Trop Dis. (2013) 7:e2466. doi: 10.1371/journal.pntd.0002466
22. Rascalou G, Pontier D, Menu F, Gourbière S. Emergence and prevalence of human vector-borne diseases in sink vector populations. PloS One. (2012) 7:e36858. doi: 10.1371/journal.pone.0036858
23. Barbu C, Dumonteil E, Gourbière S. Characterization of the dispersal of non-domiciliated Triatoma dimidiata through the selection of spatially explicit models. PloS Negl Trop Dis. (2010) 4:e777. doi: 10.1371/journal.pntd.0000777
24. Moo-Millan JI, Arnal A, Pérez-Carrillo S, Hernandez-Andrade A, Ramírez-Sierra M-J, Rosado-Vallado M, et al. Disentangling Trypanosoma cruzi transmission cycle dynamics through the identification of blood meal sources of natural populations of Triatoma dimidiata in Yucatán, Mexico. Parasit Vectors. (2019) 12:1–11. doi: 10.1186/s13071-019-3819-7
25. Dumonteil E, Ramirez-Sierra M-J, Pérez-Carrillo S, Teh-Poot C, Herrera C, Gourbière S, et al. Detailed ecological associations of triatomines revealed by metabarcoding and next-generation sequencing: implications for triatomine behavior and Trypanosoma cruzi transmission cycles. Sci Rep. (2018) 8:4140. doi: 10.1038/s41598-018-22455-x
26. Zeledón R. El triatoma dimidiata (Latreille, 1811) y su relación con la enfermedad de Chagas (2024). Available online at: https://bdu3.siu.edu.ar/bdu/Record/B-28-OMD001829 (Accessed July 20, 2024).
27. Sevá AP, Ovallos FG, Amaku M, Carrillo E, Moreno J, Galati EAB, et al. Canine-based strategies for prevention and control of visceral leishmaniasis in Brazil. PloS One. (2016) 11:e0160058. doi: 10.1371/journal.pone.0160058
28. Rosenberg R. Vital signs: trends in reported vectorborne disease cases — United states and territories, 2004–2016. MMWR Morb Mortal Wkly Rep. (2018) 67. doi: 10.15585/mmwr.mm6717e1
29. Gürtler RE, Ceballos LA, Ordóñez-Krasnowski P, Lanati LA, Stariolo R, Kitron U. Strong host-feeding preferences of the vector Triatoma infestans modified by vector density: implications for the epidemiology of Chagas disease. PloS Negl Trop Dis. (2009) 3:e447. doi: 10.1371/journal.pntd.0000447
30. Chagas disease (also known as American trypanosomiasis) (2024). Available online at: https://www.who.int/news-room/fact-sheets/detail/chagas-disease-(american-trypanosomiasis) (Accessed July 20, 2024).
31. Abad-Franch F, Celeste Vega M, Rolón MS, Santos WS, de Arias AR. Community participation in chagas disease vector surveillance: systematic review. PloS Negl Trop Dis. (2011) 5:e1207. doi: 10.1371/journal.pntd.0001207
32. Lloyd-Smith JO, Cross PC, Briggs CJ, Daugherty M, Getz WM, Latto J, et al. Should we expect population thresholds for wildlife disease? Trends Ecol Evol. (2005) 20:511–9. doi: 10.1016/j.tree.2005.07.004
Keywords: canine vaccine, Chagas Disease, mathematical modeling, vaccination, vector-borne disease
Citation: Fiatsonu E, Deka A and Ndeffo-Mbah ML (2024) Assessing the impact of a potential canine vaccine for the control of Chagas disease: a mathematical modeling study. Front. Trop. Dis 5:1421669. doi: 10.3389/fitd.2024.1421669
Received: 22 April 2024; Accepted: 07 October 2024;
Published: 25 October 2024.
Edited by:
Allassane Foungoye Ouattara, University of Nangui Abrogoua, Côte d’IvoireReviewed by:
Aldemir Branco de Oliveira Filho, Federal University of Pará, BrazilRicardo Castillo-Neyra, University of Pennsylvania, United States
Copyright © 2024 Fiatsonu, Deka and Ndeffo-Mbah. This is an open-access article distributed under the terms of the Creative Commons Attribution License (CC BY). The use, distribution or reproduction in other forums is permitted, provided the original author(s) and the copyright owner(s) are credited and that the original publication in this journal is cited, in accordance with accepted academic practice. No use, distribution or reproduction is permitted which does not comply with these terms.
*Correspondence: Edem Fiatsonu, e.fiatsonu@tamu.edu