- 1Bio-Manguinhos Institute, Oswaldo Cruz Foundation, Rio de Janeiro, Brazil
- 2Department of Infectious Diseases and Microbiology, University of, Pittsburgh, PA, United States
- 3Aggeu Magalhães Institute, Oswaldo Cruz Foundation, Recife, Pernambuco, Brazil
- 4Vaccine Analytics and Formulation Center, Department of Pharmaceutical Chemistry, University of Kansas, Lawrence, KS, United States
- 5Director General Office, International Vaccine Institute, Seoul, Republic of Korea
- 6College of Natural Sciences, Seoul National University, Seoul, Republic of Korea
Introduction
The scientific debate on the need for pandemic preparedness and an evolutionary approach to infectious disease emergence, from disease evolution and eco-social conceptual frameworks (1), gained visibility in the 1990s. This was promoted by academic concerns over the spread and dislocation of vectors and pathogenic agents from the Tropics to other regions of the globe consequent to climate change, global warming and El Niño.
This debate on eco-social conditions favoring the emergence and resurgence of neglected pathogens and diseases, promoted by the Harvard New Diseases Group, resulted in publications in the mid-1990s with pioneer recommendations for epidemic/pandemic anticipation and response (1, 2). Recently, this eco-social perspective to accelerate pandemic anticipation and response gained visibility, in a new era of scientific and technological breakthroughs, especially new insights from immunomics, genomics and artificial intelligence.
This academic agenda included, from this perspective, concerns with the dissemination of cross-species transmissions of emerging and re-emerging zoonosis, such as Yellow Fever, Oropouche Fever and Mayaro Fever. Yellow Fever, an arboviral disease under the risk of reurbanization in Brazil (3, 4), emerged as an African mosquito-borne disease of human and non-human primates, transmitted by forest-dwelling primatophilic Sabethes and Haemagogus mosquitoes and in urban areas by Aedes aegypti and Aedes albopictus, has disseminated worldwide. Oropouche Fever, caused by Oropouche virus, was first identified in Vega de Oropouche, Trinidad and Tobago and emerged and disseminated in Brazil from predominant vector C. paraensis feeding on humans, other mammals and possibly birds. Mayaro Fever, caused by Mayaro virus, was first isolated in Mayaro County, Trinidad and Tobago, disseminated in Brazil and other South and Central American countries around the Amazon basin transmitted by Haemagogus spp mosquitoes also feeding on humans and non-human primates.
The 21st century added urgency to the implementation of systems to predict emerging threats, early detection, and rapid responses (5, 6). In addition to those infectious diseases exacerbated by climate change, the emergence in different regions of the globe of SARS-1, H1N1, Avian influenza, and West Nile also contributed for the sense of urgency of the need for preparedness and helped to catalyze the mission of BARDA in the US.
Scientists have also alerted to the recent aggravation of this scenario. Climate change, with extreme weather events in several regions of the world, is contributing to accelerate infectious disease emergence and resurgence, heavily impacting on the health of populations. The ongoing severe Dengue outbreak in Brazil and other Latin-American and Caribbean countries (7, 8) illustrates well this scenario and the urgent need of public policies to anticipate and minimize the consequences of global warming and extreme weather events.
Even with all these alerts, the translation of science into timely epidemic/pandemic anticipation and response for global security is a complex governance issue and has so far proved to be a difficult task, with policy makers even discussing its feasibility in tropical regions. The COVID-19 pandemic evidenced this governance complexity. Lack of integrated surveillance and effective spillover prevention, among other factors, have proved to be key issues and provide important lessons for future pandemic prepared- ness and response.
Our article aims thus to contribute to an integrated approach to the three main factors impacting on anticipation and response to a possible future Disease X pandemic: an active and functional epidemiological surveillance, supported by genomic, antigenic, epizootic, laboratory and participatory surveillance; an acceleration of spillover prevention, supported by strategic monitoring of high-risk pathogens and reservoirs, increasing the global capacity to respond to newly emerging zoonotic diseases; finally, vaccine preparedness, discussing the main initiatives at the international level to accelerate vaccine development, such as CEPI´s 100 days mission initiative.
Pandemic preparedness
Pandemic preparedness, particularly for emerging diseases in the Tropics, has so far deserved very low investment priority from the G-7 and G-20 leading economies and international agencies, severely threatening global health (6). It requires huge and sustained long-term investments, based on One Health perspective, providing access to innovative technologies and support to accelerate global exchange of information and materials.
The lack of investments, particularly in more vulnerable developing countries, contrasts with the fact that zoonotic diseases are estimated to be responsible for 60% of epidemics worldwide (5). These epidemics have been favored by the aggravation of several eco-social conditions: market, trade, intensive land use in agriculture and mining, deforestation and climate change that have contributed to exponentially increase exposure of humans to the animal reservoirs of the pathogen increasing the risk of spillovers (Figure 1). Even though SARS-1, SARS-CoV-2, and MERS are related to human exposure to animal reservoirs for food, recreation, or farming, spillover prevention has been largely sidelined in the post-Covid 19 debates by international agencies, governments and stakeholders on global approaches to pandemics (9, 10).
The previous reemergence of SARS in 2003 and emergence of MERS in 2012 also provided important alerts and lessons for epidemic/pandemic preparedness and illustrate well the need for integrated surveillance and an effective spillover prevention (10). SARS resurged in 2003 in Asia as an epidemic spreading to more than two dozen countries. MERS epidemics emerged in Saudi Arabia in 2012 linked to dromedary possibly infected by bats, with cases reported to WHO by several countries in Africa, Asia, Europe and the Americas.
The COVID-19 pandemic, which likely emerged in humans in 2020, dramatically evidenced this global neglect and the implications of environmental and eco-social change for infectious disease emergence. The viral spillover in Wuhan, a sub-tropical city in Hubei province of Central China, was facilitated by the increased human exposure to bats and animal reservoirs and for the local conditions favoring zoonotic cross-overs due to wet markets. This scenario of increased human vulnerability to infectious disease emergence in tropical and sub-tropical regions underscores the need for comprehensive epidemic/pandemic preparedness.
The lesson for spillover prevention and preparedness is that the impacts of the COVID-19 pandemic could have been minimized if early warnings being translated to preventive action (10). In 2002, an initial SARS outbreak emerged, and after its decline, the topic was neglected, in spite of alerts from the scientific community. In 2007, scientists warned again on the presence of “large reservoirs of SARS-CoV-like viruses in horseshoe bats in Southern China and that civets are the amplification hosts”, alerting for the issue of “biosecurity in farms and wet markets” (11). Nevertheless, despite worldwide scientific recognition of this prediction and many citations in the literature, the topic was again neglected.
International agencies and governments were once again surprised when COVID-19 pandemic emerged 12 years after this 2007 warning due to lack of a proactive approach to global management of potentially epidemic/pandemic infectious diseases (10, 12).
Fortunately, based on previous developments for Zika vaccines engendered from the 2015/16 outbreak and the use of molecular dynamics simulations to engineer a stabilized spike proteins based on the studies that came out of RSV and HIV research, the US Warp Speed Operation, in collaboration with WHO, GAVI and other international organizations succeeded in developing COVID-19 vaccines in less than six months, largely distributed but heavily concentrated in developed nations.
This rapid development of COVID-19 vaccines has certainly been an extraordinary breakthrough and a landmark in the history of science. However, it is important to alert the global community that when Disease X emerges possibly this scenario of immediate availability of COVID-19 vaccines´ might not be repeated due to immunological challenges imposed by the pathogen X, which could result in a global disaster. Vaccines might not be available if effective novel international governance strategies for pandemic preparedness are not immediately put in place. Consequently, the countries, international agencies, and policymakers could once again be surprised by an unexpected pandemic.
The next disease X pandemic: tropical emergence, high lethality and no vaccines?
According to WHO, Disease X could be 20 times more lethal than COVID-19, with very high costs, possibly no vaccines, and enormous social and economic impacts. It is important to underline that direct and indirect costs of COVID-19, a disease with relatively low lethality and vaccine available, were estimated just for one country, the US, in U$ 14 trillion by the end of 2023 (13).
Possibly, the next Disease X pandemic will emerge or resurge as a zoonotic disease in tropical or sub-tropical regions of the globe in spillover from wildlife animals to humans, followed by mutations favoring human-to-human transmission in complex eco-social environments (Figure 2). We should also consider that the emergence of novel pathogens might result from the melting of the permafrost associated with increased extraction of natural resources in sub-polar areas.
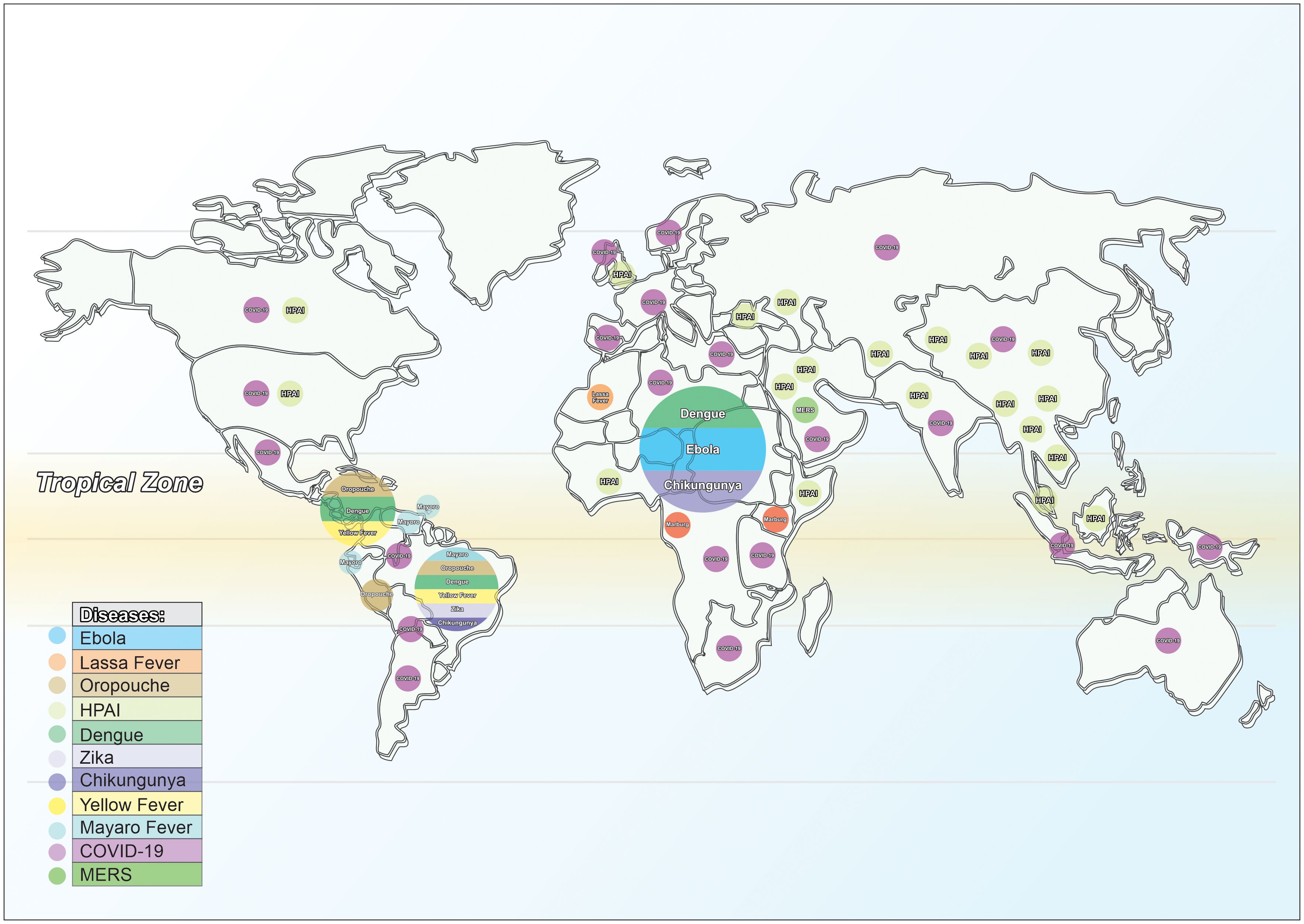
Figure 2 Pathogens/diseases: future Disease X emergence in the Tropics. Source: elaborated by the authors.
Disease X might emerge from pathogens originating in tropical and sub-tropical areas, such as Ebola, Lassa Fever, Marburg, Mayaro, Oropouche and MERS. Highly Pathogenic Avian Influenza (HPAI) has also been a great global concern, despite absence of human-to-human transmission so far. A recent publication has highlighted the bottlenecks in vaccine development for HPAI, further emphasizing the need for preparedness (14).
Concerning these bottlenecks, it is important to underline that the “valley of death” concerning vaccine development, particularly for neglected tropical diseases, has been the main barrier limiting translation from basic research to final products. This constraint requires new global governance strategies and increased investment in science and technology, aiming to strengthen technological development, which requires GMP infrastructure for laboratory activity, trained human resources and high-quality inputs for laboratory work and other issues.
In addition, neglected arboviral diseases such as Dengue, Zika, Chikungunya and Yellow Fever have received very low attention and insufficient funding for research and vaccine development, with important consequences for the populations in the Tropics (Figure 2). The emergence of scrub typhus in the United States is another example of vector-borne disease, spread by travel, and becoming established in a new locale (15).
It is important to note that spread of infectious agents favoring dissemination of these emerging diseases to other regions of the globe is, together with climate change and other determinants, mainly a result of a globalization processes related to populational mobility and international travel, as previously indicated in Figure 1. Zika is a good example of the impacts this global spread can cause.
The recent severe Dengue epidemic in Brazil and other Latin American countries (7, 8) that resulted from El Niño and record high temperatures in Brazil, has led several Brazilian states to declare an emergency for a disease that has been globally neglected for nearly three decades, for which only partially efficacious vaccines are available (16). A combination of determinants -very high temperatures, heavy rains and lack of basic sanitation in the country, with only 50% of Brazilian houses and 40% of Brazilian schools with access to treated water and adequate sewage collection (17) resulted in the hatching of Aedes aegypti eggs and accelerated dengue dissemination due to low heard immunity against serotypes 1 and 2.
Although there are two dengue vaccines approved for use in Brazil, Dengvaxia and Qdenga, the rollout of this vaccines has been slow due to some limitation of these vaccines. “Dengvaxia is only recommended for use in people that have laboratory confirmed prior dengue infection, that maybe useful for the elderly population; and Qdenga has demonstrated for be efficacious against dengue serotypes 1 and 2, however has no efficacy against dengue serotype 3. This scenario is further aggravated by the reduced availability of dengue vaccines in the international pharmaceutical market, as informed by the country´s Ministry of Health. For a population of 203 million, Brazil could just buy 5.2 million doses of the two-doses Takeda´s Qdenga vaccine, available for delivery this year” (7, 8), too late to have impact on the epidemic. In addition, there are still poorly understood interactions between dengue and Zika antibodies that need to be better clarified.
An additional nine million doses will be delivered only in 2025, with a donation from Takeda of additional 1.3 million, indicating the severity of the global vaccine scarcity scenario. There are no other vaccine alternatives in the market: Sanofi´s Dengvaxia has not been incorporated into the Brazilian National Health System (SUS) and is not indicated for individuals not previously infected by the virus. A promising vaccine by the Butantan Institute in São Paulo is still in the final stage of development. As the Qdenga vaccine, it is tetravalent, however still has not demonstrated protection against all four viral subtypes due to epidemiological reasons. In addition, it is still not clear what is the production capacity of the Butantan Dengue vaccine.
Research institutes and companies should conceive creative partnerships to develop better dengue vaccines using newer and more immediately scalable technologies. Moreover, it is essential to build strategic stockpiles of these dengue vaccines.
Finally, it is important to highlight that strategic vaccine stockpiling for potentially epidemic/pandemic diseases is a key issue for accelerating innovative prototype vaccine development, in support to CEPI´s 100 days mission.
Vaccine preparedness: accelerating prototype vaccines in 100 days mission
Vaccine Preparedness for Disease X is a critical issue and a novel global governance strategy should build on the innovative CEPI´s 100 days mission initiative (18, 19). A thorough overview of the challenging issues for accelerating vaccine development for emerging infectious diseases has been recently provided (20).
Prototype vaccines would be based on common or few technologies and vaccine factures aiming to start massive vaccine production immediately. Vaccines against medium or high-risk pathogens should be subject to a strategic stockpile. For Dengue vaccines, for example, there is a discussion: should a stockpile of existing vaccines be within easy reach, despite their current limitations, or should scientists accelerate their work on a vaccine that is more immediately available?
Rapid-response platforms are critical in this prototype vaccine strategy. Researchers will be able to address in advance the main features of a given viral family, providing an innovative strategy to develop vaccines against related emerging pathogens. Such prototype vaccines would examine the protection levels in animal challenge models and determine the immune correlates of protection followed by clinical studies of safety and immunogenicity (19). This novel Vaccine Preparedness model should assure emergency manufacturing, procurement and supply chain management without compromising safety or quality.
The contribution of the Human Immunome Project (HIP) (21, 22) can also be key to consolidating CEPI´s 100 days mission, especially by support in accelerating the discovery of the immune correlates of protection and predicting the vaccine efficacy based on immunogenicity studies (23, 24). HIP has been involved in sequencing the immunome and in developing universal vaccines against COVID-19 and Influenza, that could end the next pandemic before it starts. This innovative strategy, accelerating the development of universal vaccines against all viral variants, could contribute in a possible future Disease X pandemic, to avoid “as in COVID-19 pandemic, a continuous vaccine redesign for each viral variant, delaying vaccine response “ (25).
Finally, to be successful, this prototype vaccine strategy will require building laboratory competence supported by pathogen libraries to accelerate identification of emerging pathogens.
Pathogen libraries: building laboratory competences for pandemic preparedness
Laboratory competence has been a critical issue for preparedness, with gaps and bottlenecks evidenced in COVID-19 pandemic. Recent advancements aiming to overcome these constraints have facilitated rapid and precise identification and characterization of emerging pathogens, with important applications in global outbreak surveillance (26).
Several successful initiatives have emerged in different countries to overcome these constraints, such as the PREMISE Program at the US NIAID Vaccine Research Center and the SciLifeLab Program in Sweden, aiming to strengthen laboratory capacity for pandemic preparedness.
To promote pandemic preparedness and response, PREMISE (Pandemic Response Repository via Microbial and Immune Surveillance and Epidemiology) was conceived as a translational tool to incorporate cellular immunological and serologic discoveries aimed at a wide range of pathogens into product development. PREMISE uses sequencing to find novel and reemerging viruses in human and animal reservoir samples, searching to find monoclonal antibodies and immunogens for vaccine and drug development and to produce reagent and data for early detection and diagnosis (27).
The Swedish government is supporting SciLifeLab in a program conceived to increase national laboratory capacity for future pandemics and has recently made strong investments in laboratory design and infra-structure. The program includes: research on emerging pathogens; competence and technological development, including sequencing, genetic analysis, immunological technique and big data management as well as maximization of operations at national and regional levels (28).
Similar innovative programs should be urgently implemented in vulnerable developing countries, aiming to strengthen laboratory competence for pandemic preparedness.
Spillover prevention: environmental protection to reduce human exposure
The need for minimizing eco-social conditions favoring emergence and resurgence of potentially pandemic zoonoses and for environmental protection has been long debated (1, 29). Evidence provided by several studies indicate that limiting human exposure to reservoirs is crucial and could substantially reduce zoonotic spillover, contributing to pandemic preparedness (30).
Zoonotic host diversity rises in habitats where human populations predominate, according to a recent study. The results indicate that habitat degradation in human populated areas has significantly increased reservoir populations (bats, rodents and birds), which may have accelerated viral spillover to humans (31).
Cities are expanding worldwide their urban areas into fields and forests, increasing human exposure to sylvatic vectors (Figure 3). Thus, protecting the environment and providing vector control are necessary conditions to minimize human exposure to zoonotic spillover.
Evidence from another study also indicates that the cost of environmental and eco-social strategies directed to the prevention of zoonotic spillover is very low when compared with the exponential increase in global expenditure and long-term economic and social impacts from a pandemic such as COVID-19 (32).
We support refocusing international efforts and resources on developing a cutting-edge global pandemic preparedness model, that combines eco-social foresight with the ability to respond to newly emerging viruses. This model could offer a quick, early reaction to these risks, reducing or eliminating the spread of these infections into humans.
Artificial intelligence: powerful tool for integrating spillover prevention, surveillance and vaccine preparedness
It is of utmost urgency the adequate implementation of an innovative pandemic anticipation/response system rooted in the One Health paradigm, based on forecast models, risk prediction and integrated surveillance - genomic, antigenic, epizootic, laboratory and participatory (Figure 4).
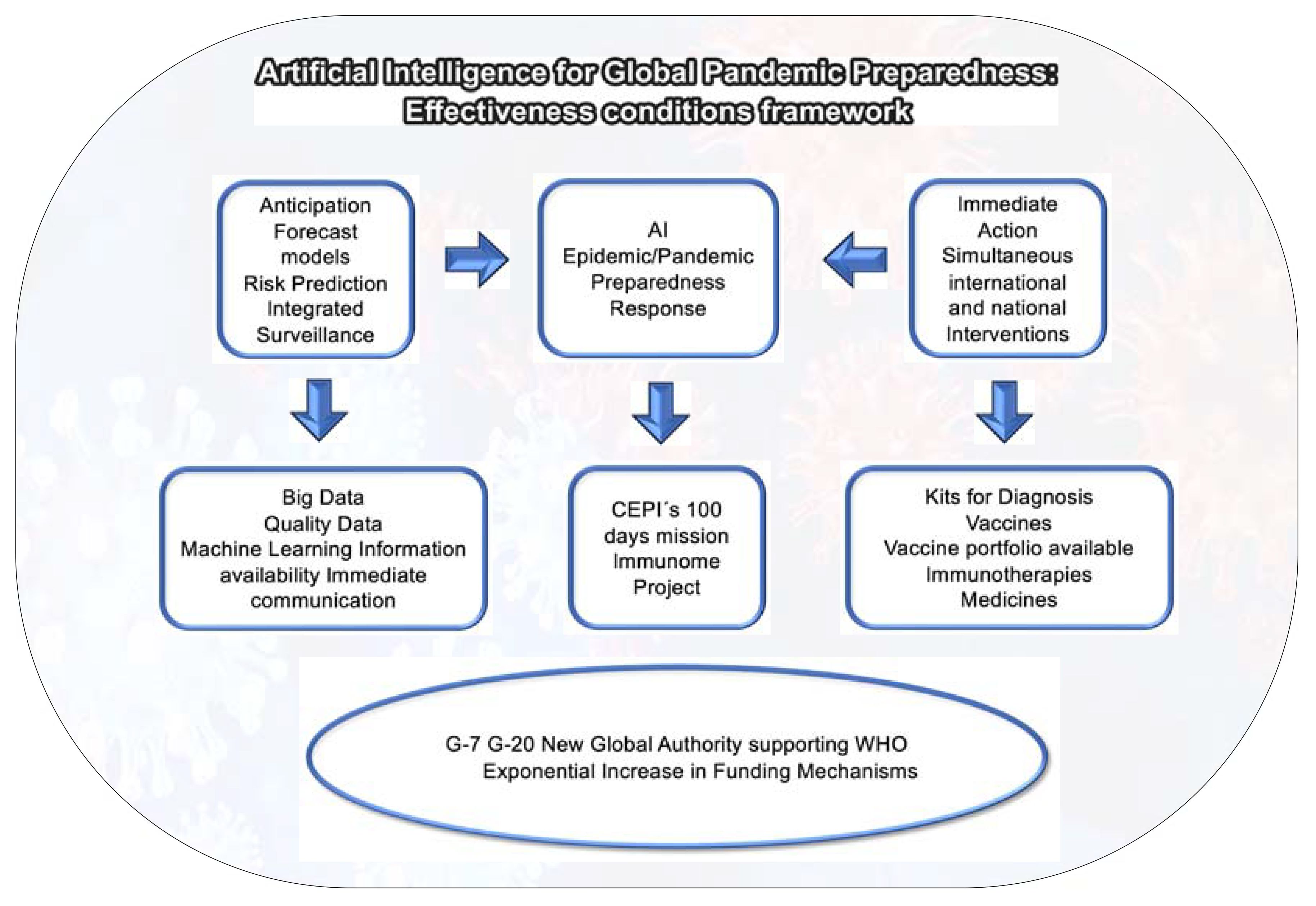
Figure 4 Artificial Intelligence: Disease X Preparedness and Response. Source: elaborated by the authors.
Supported by a new approach integrating Artificial Intelligence, Machine Learning and Big Data, this system should be conceived to anticipate the impact of outbreaks. It can become a critical tool in our arsenal to prevent future pandemics and its implementation will support policy makers in their decision-making processes.
While Artificial Intelligence can play a pivotal role in Disease X Pandemic Preparedness, it is necessary to stress that Big Data alone is insufficient. The effectiveness of AI systems is directly linked to the quality of data they use. Large amounts of data alone do not help if most of the data is wrong or irrelevant.
Benefiting from pioneer AI advancements in pandemic foresight, the Canadian AI start-up BlueDot anticipated the COVID-19 outbreak in China ahead of governments and international organizations by utilizing initial advancements in AI strategies. BlueDot began notifying government and private sector clients in December 2019, warning about the risks of ´unusual pneumonia´ cases near a market in Wuhan, China (33).
Similar AI projects are being carried out by other enterprises: BioSymetrics, Biovista and the Azure ML. BioSymetrics has created, in association with Janssen and Sema4, novel COVID-19 prediction methods (34). Biovista has designed a new AI strategy focused on identifying bioactive chemicals and developing pandemic healthcare scenarios (35). The Azure ML Strategy, developed in India in 2020, has been conceived as an innovative AI and machine learning forecast model (36).
Despite recent advancements, AI solutions have not yet been able to effectively assist policymakers in developing and implementing a prompt and targeted strategy for COVID-19 and other potential pandemics (37). The data collection systems are very outdated and require substantial expenses for maintenance. Implementing new low-cost technologies necessitates substantial capital inputs. Furthermore, it is important to underline that the range of AI strategies available for preparing and responding to epidemics/pandemics is currently restricted. Available high-quality data on widely recognized viruses, like coronaviruses and other respiratory viruses, should be more efficiently integrated into these novel AI systems.
These constraints can be attributed to two primary obstacles: inadequate data and an excessive volume of data (37). It is thus unclear to what extent it will be possible to accurately anticipate the occurrence of new potentially pandemic viruses in the near future.
Some important initiatives have recently been conceived in an attempt to reverse this scenario: SPILLOVER, PREDICT and CEPI´s 100 days mission, in collaboration with Houston Methodist Research Institute-led consortium.
SpillOver, an innovative tool created by the Global Virome Project, calculates risk scores for viruses originating from wildlife. A recent study compared zoonotic viruses with unknown potential for spillover to humans with those already known to be capable of infecting humans, identifying 887 wildlife viruses with spillover risk (38).
In the same direction, the PREDICT Project, a remarkable AI strategy developed by the One Health Institute, University of California, Davis, evidences the potential of novel developments in pandemic foresight. The study has analyzed samples from almost 56,000 wild animals and concluded that 800 recently found zoonotic viruses possess the ability to infect humans (39).
Finally, pioneer initiatives for using AI in accelerating Vaccine Preparedness for the Pandemic, such as CEPI´s 100 days mission, in collaboration with Houston Methodist Research Institute-led consortium, are recent and in initial development (40).
In order to strengthen the role AI can play in knowledge governance and in accelerating vaccine development for Disease X Preparedness, G-7 and G-20 countries should create a Global Authority supporting WHO in pandemic anticipation and response, as indicated in Figure 4.
Conclusion
The potential lack of vaccines in a future Disease X outbreak or pandemic could lead to a catastrophic global disaster, with profound social and economic implications. This crisis would disproportionately affect developing countries, many of which are located in the Tropics.
Pandemic preparedness is a multifaceted challenge that extends well beyond scientific breakthroughs. It necessitates different levels of interventions and a knowledge governance model that can coordinate complex, simultaneous processes. This new governance strategy, bolstered by Artificial Intelligence for its effective and timely implementation, should be able to integrate a broad range of interventions: spillover prevention, epidemic/pandemic anticipation, more sensitive and integrated surveillance (genomic, antigenic, epizootic and epidemiological), along with the availability of suitable pre-clinical animal models and a fully prepared vaccine bank for manufacture and clinical trials.
It will also be necessary to conceive and organize multipurpose laboratories with capacity to develop viral, bacterial, fungal and parasitic vaccines and adequate conditions for timely Disease X vaccine production. In addition, this innovative governance model should assure laboratory biosafety, emergency manufacturing, procurement and supply chain management.
This strategy will require, based on One Health Paradigm and on CEPI´s 100 days vaccine mission, an exponential increase in sustainable funding from multiple stakeholders, including WHO, IMF, the World Bank, G-7, G-20. An International Pandemic Preparedness Authority supporting WHO should be created with these new funding mechanisms for immediate intervention when a new pathogen or emerging disease is identified, avoiding global dissemination. This new Authority supported by a Pandemic Preparedness and Innovation Fund created by international agencies, governments, donors and stakeholders should be able to strengthen and integrate a broad range of scientific and technological collaborations.
While these strategies require substantial global long-term investments, the return on such investments to global health, economy and society will be enormous. Artificial Intelligence will be key for this new global governance model, catalyzing the acceleration of spillover prevention, integrated into One Health surveillance, and the scaling-up of rapid-response vaccine platforms and clinical trials.
Author contributions
CP: Conceptualization, Investigation, Writing – original draft, Writing – review & editing. EM: Conceptualization, Writing – original draft, Writing – review & editing. SK: Writing – review & editing. PK: Writing – review & editing. JK: Writing – review & editing. AH: Conceptualization, Writing – original draft, Writing – review & editing.
Funding
The author(s) declare financial support was received for the research, authorship, and/or publication of this article. Thanks to Frontiers for funding the APC for this article. Thanks to Bio-Manguinhos/Oswaldo Cruz Foundation for the support for this research.
Acknowledgments
The authors thank José Viña for technical support in the design of the Figures.
Conflict of interest
The authors declare that the research was conducted in the absence of any commercial or financial relationships that could be construed as a potential conflict of interest.
The author(s) declared that they were an editorial board member of Frontiers, at the time of submission. This had no impact on the peer review process and the final decision.
Publisher’s note
All claims expressed in this article are solely those of the authors and do not necessarily represent those of their affiliated organizations, or those of the publisher, the editors and the reviewers. Any product that may be evaluated in this article, or claim that may be made by its manufacturer, is not guaranteed or endorsed by the publisher.
References
1. Wilson ME, Levins R, Spielman A. Disease in evolution: global changes and emergence of infectious diseases. Ann New York Acad Sci. (1994) v. 740.
2. Levins R, Awerbuch T, Brinkmann U, Eckardt I, Epstein P, Makhoul N, et al. The emergence of new diseases. Am Sci. (1994) 82:52–60.
3. Possas C, Martins RR, Lourenço-de-Oliveira R, Homma A. Urgent call for action: avoiding spread and reurbanisation of yellow fever in Brazil. Memórias do Instituto Oswaldo Cruz. (2017) 113:1–2.
4. Possas C, Lourenço-de-Oliveira R, Tauil PL, Pinheiro FD, Pissinatti A, Cunha RV, et al. Yellow fever outbreak in Brazil: the puzzle of rapid viral spread and challenges for immunisation. Memórias do Instituto Oswaldo Cruz. (2018) 113. doi: 10.1590/0074-02760180278
5. Jones KE, Patel NG, Levy MA, Storeygard A, Balk D, Gittleman JL, et al. Global trends in emerging infectious diseases. Nature. (2008) 451:990–3. doi: 10.1038/nature06536
6. Patel J, Sridhar D. Toward better pandemic preparedness (2021). Washington DC: International Monetary Fund, IMF. Available online at: https://www.imf.org/en/Publications/fandd/issues/2021/12/Pandemic-preparedness-Patel-Sridhar (Accessed May 29 2024).
7. PAHO/WHO Epidemiological Alert. Increase in dengue cases in the Region of the Americas (2024). Available online at: https://www.paho.org/en/documents/epidemiological-alert-increase-dengue-cases-region-americas-16-february-2024 (Accessed February 18 2024).
8. Nolen S. Brazil Has a Dengue Emergency, Portending a Health Crisis for the Americas (2024). New York Times. Available online at: https://www.nytimes.com/2024/02/10/health/dengue-Brazil-americas.html (Accessed February 27 2024).
9. Vora NM, Hannah L, Walzer C, Vale MM, Lieberman S, Emerson A, et al. Interventions to reduce risk for pathogen spillover and early disease spread to prevent outbreaks, epidemics, and pandemics. Emerg Infect Dis. (2023) 29:1–9. doi: 10.3201/eid2903.221079
10. Morens DM, Breman JG, Calisher CH, Doherty PC, Hahn BH, Keusch GT, et al. The origin of COVID-19 and why it matters. Am J Trop Med Hyg. (2020) 103:955–9. doi: 10.4269/ajtmh.20-0849
11. Cheng VC, Lau SK, Woo PC, Yuen KY. Severe acute respiratory syndrome coronavirus as an agent of emerging and reemerging infection. Clin Microbiol Rev. (2007) 20:660–94. doi: 10.1128/CMR.00023-07
12. Taubenberger JK, Kash JC, Morens DM. The 1918 influenza pandemic: 100 years of questions answered and unanswered. Sci Trans Med. (2019) 11. doi: 10.1126/scitranslmed.aau5485
13. Hlávka J, Rose A. COVID-19’s total cost to the US economy will reach $14 trillion by end of 2023. In: The Evidence Base: Informing Policy in Health, Economics and Well Being. California, USA: Leonard D. Schaeffer Center for Health Policy and Economics, University of Southern California (2023).
14. Possas C, Marques ET, Oliveira A, Schumacher S, Mendes F, Siqueira MM, et al. Highly Pathogenic Avian Influenza Vaccines: challenges for innovation, technological development and pandemic preparedness. medRxiv. (2023). doi: 10.1101/2023.05.31.23290790
15. Khairkar M, Vagha S, Deotale V. Review on scrub typhus: an important etiology of acute undifferentiated fever illness. Cureus. (2023) 15. doi: 10.7759/cureus.47290
16. Ooi EE, Kalimuddin S. Insights into dengue immunity from vaccine trials. Sci Trans Med. (2023) 15. doi: 10.1126/scitranslmed.adh3067
17. Trata Brasil Institute. Ranking of Sanitation 2024 based on the National Sanitation Information System of the Brazilian government 2022 (SNIS). São Paulo, 20 march 2024.
18. Gouglas D, Christodoulou M, Hatchett R. The 100 days mission - 2022 global pandemic preparedness summit. Emerg Infect Dis. (2023) 29(3).
19. Global Pandemic Preparedness Summit - Recording . Available online at: https://100days.cepi.net (Accessed 28 04 2024).
20. Excler JL, Saville M, Berkley S, Kim JH. Vaccine development for emerging infectious diseases. Nat Med. (2021) 27(4):591–600.
21. Koff WC, Burton DR, Johnson PR, Walker BD, King CR, Nabel GJ, et al. Accelerating next-generation vaccine development for global disease prevention. Science. 340:1232910.
23. De Sousa KP, Doolan DL. Immunomics: a 21st century approach to vaccine development for complex pathogens. Parasitology. 143:236–44.
24. Leslie M. Megaproject will chart human immune diversity: Human Immunome Project’s survey aims to improve drugs and vaccines. Science. (2024) 383(6678):13–14. doi: 10.1126/science.adn8379
25. Possas C, Marques ET, Risi JB Jr., Homma A. COVID-19 and future disease X in circular economy transition: redesigning pandemic preparedness to prevent a global disaster. Circular Economy Sustainability. (2021) 1:1463–78.15. doi: 10.1007/s43615-021-00060-x
26. Vashisht V, Vashisht A, Mondal AK, Farmaha J, Alptekin A, Singh H, et al. Genomics for emerging pathogen identification and monitoring: Prospects and obstacles. BioMedInformatics. (2023) 3(4):1145–77.
27. NIAID, NIH. PREMISE Research 2024 . Available online at: https://www.niaid.nih.gov/research/premise (Accessed April 8 2024).
28. SciLifeLab. Pandemic Laboratory Preparedness (PLP) Program (2022). Sweden. Available online at: https://www.pathogens.se/plp-program-background/#progress-to-date (Accessed April 4 2024).
29. Morse SS, Mazet JAK, Woolhouse M, Parrish CR, Carroll D, Karesh WB, et al. Prediction and prevention of the next pandemic zoonosis. Lancet. (2012) 380:1956–65. doi: 10.1016/S0140-6736(12)61684-5
30. Nature Ecology and Evolution. Three-pronged pandemic prevention. Editorial Nat Ecol Evol. (2020) 4:1149. doi: 10.1038/s41559-020-01304-z
31. Gibb R, Redding DW, Chin KQ, Donnelly CA, Blackburn TM, Newbold T, et al. Zoonotic host diversity increases in human-dominated ecosystems. Nature. (2020) 584:398–02. doi: 10.1038/s41586-020-2562-8
32. Dobson AP, Pimm SL, Hannah L, Kaufman L, Ahumada JA, Ando AW, et al. Ecology and economics for pandemic prevention. Science. (2020) 369:379–81. doi: 10.1126/science.abc3189
33. Bowles J. How Canadian AI start-up BlueDot spotted coronavirus before anyone else had a clue. London, UK: Diginomica (2020) p. 1–2.
34. BioSymetrics. BioSymetrics collaborates with Janssen and Sema4 to predict the onset of COVID-19 (2020). Available online at: http://wwwprnewswirecom/news-releases/biosymetrics-collaborates-with-janssen-and-sema4-to-predict-the-onset-of-covid-19-301114823html (Accessed January 8 2024).
35. Biovista. Biovista harnesses new kinds of augmented and artificial intelligence to address covid-19 (2020). Available online at: https://www.prnewswire.com/news-releases/biovista-harnesses-new-kinds-of-augmented-and-artificial-intelligence-to-address-covid-19-322 (Accessed January 8 2024).
36. Raja V. Building End to End COVID-19 Forecast Model using Azure ML, June 8 2020 (2020). Available online at: https://vivekraja98.medium.com/building-end-to-end-covid-19-forecast-model-using-azure-ml-16da338864b3 (Accessed March 10 2024).
37. Naudé W. Artificial intelligence vs COVID-19: limitations, constraints and pitfalls. AI Soc. (2020) 35:761–5. doi: 10.1007/s00146-020-00978-0
38. Grange ZL, Goldstein T, Johnson CK, Anthony S, Gilardi K, Daszak P, et al. Ranking the risk of animal-to-human spillover for newly discovered viruses. Proc Natl Acad Sci. (2021) 118. doi: 10.1073/pnas.2115409118
40. CEPI. Using AI to speed up vaccine development against Disease X (2023). Available online at: https://cepi.net/using-ai-speed-vaccine-development-against-disease-x (Accessed April 18 2021).
Keywords: disease X, pandemic, preparedness, COVID-19, spillover prevention, vaccines, artificial intelligence
Citation: Possas C, Marques ETA, Kuchipudi SV, Kumar P, Kim JH and Homma A (2024) Disease X in the Tropics, preventing the next pandemic: how to accelerate spillover prevention and vaccine preparedness?. Front. Trop. Dis 5:1417065. doi: 10.3389/fitd.2024.1417065
Received: 13 April 2024; Accepted: 12 June 2024;
Published: 04 July 2024.
Edited by:
Malcolm Scott Duthie, HDT Biotech Corporation, United StatesReviewed by:
Hai Yen Lee, University of Malaya, MalaysiaCopyright © 2024 Possas, Marques, Kuchipudi, Kumar, Kim and Homma. This is an open-access article distributed under the terms of the Creative Commons Attribution License (CC BY). The use, distribution or reproduction in other forums is permitted, provided the original author(s) and the copyright owner(s) are credited and that the original publication in this journal is cited, in accordance with accepted academic practice. No use, distribution or reproduction is permitted which does not comply with these terms.
*Correspondence: Cristina Possas, cG9zc2FzLmNyaXN0aW5hMUBnbWFpbC5jb20=; Ernesto T. A. Marques, bWFycXVlc0BwaXR0LmVkdQ==