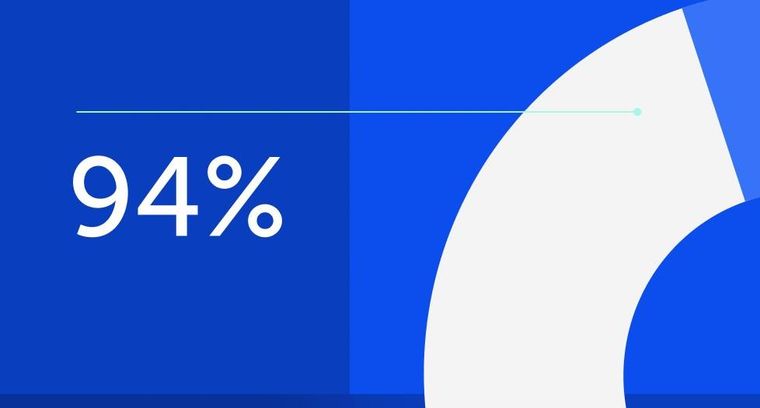
94% of researchers rate our articles as excellent or good
Learn more about the work of our research integrity team to safeguard the quality of each article we publish.
Find out more
ORIGINAL RESEARCH article
Front. Trop. Dis., 10 December 2024
Sec. Neglected Tropical Diseases
Volume 5 - 2024 | https://doi.org/10.3389/fitd.2024.1391823
This article is part of the Research TopicInnovative Strategies for Overcoming Drug Resistance in Tropical DiseasesView all articles
Background: Mansonella perstans is transmitted by Culicoides species and affects hundred millions of inhabitants in about 33 countries in sub-Saharan Africa. It is known that Mansonellosis due to Mansonella perstans do not result in a clear clinical picture, but down-regulates the immunity of patients predisposing them to other diseases like tuberculosis, HIV and malaria or damping vaccine efficacy. However, research about novel drugs against this filarial nematode is missing because of the lack of parasite material. Previous studies have developed in vitro culture systems using infective stage 3 larvae (L3), but these life stages are difficult to obtain and thus the performance of in vitro cultures is restricted and does not allow large-scale testing of drugs or even infection experiments in animal models. Therefore, we aim to establish a platform for the large-scale production of M. perstans infective larvae from engorged Culicoides milnei.
Methods: Culicoides species were caught in Yangom (Yabassi Health District) in the Littoral Region of Cameroon following a blood meal on six microfilariae-positive donors with different microfilaraemic loads over one year. Engorged midges were reared in the insectarium for up to 14 days and L3 were isolated from the different body parts.
Result: In summary, 13,658 engorged Culicoides were collected and reared in the laboratory. We observed an overall predicted survival of 78.5%. Out of the 8,123 survived midges, 7,086 midges belong to C. milnei, from which 2,335 were infected leading to a recovery of 6,310 L3. Moreover, we found the highest survival rates of midges during the early dry season in December with moderate temperatures (23-25°C) and low (2-4mm) or no rainfall. In addition, we observed that midges that fed on donors with high microfilarial loads showed increased mortality.
Conclusion: We revealed suitable conditions for the collection and maintenance of engorged Culicoides midges allowing the large-scale production of M. perstans L3. This procedure will provide a platform to produce sufficient parasite material that will facilitate in vitro cultures and the establishment of a murine model of M. perstans, which is important for in-depth investigation of the filarial biology and screening of novel drugs that are effective against this ivermectin-resistant nematode.
Mansonellosis is caused by Mansonella perstans, M. ozzardi, M. streptocerca and M. sp. DEUX (1, 2), but zoonotic species like M. rhodhaini have been described as well (3). In contrast to lymphatic filariasis or onchocerciasis, no severe clinical symptoms are associated with mansonellosis, but subcutaneous swellings, skin rashes, pericarditis and pleuritis, keratitis and eosinophilia have been reported (4). It is suggested that the lack of clinical symptoms is associated with a strong immunomodulatory capacity (2, 5), which has led to a shortfall of knowledge about this parasite, but is also associated with increased susceptibility and worsened disease course of concomitant infections (6–8) accompanied with lowered vaccination efficacy (9). Thus, knowledge about this filarial nematode, its biology and evasion tactics are needed to develop treatment strategies. Most of the knowledge about mansonellosis is obtained from M. perstans infections, which is widespread in Sub-Saharan Africa, Central and South America and was even considered as the most prevalent parasite of man in tropical Africa (10). In spite of this, very few studies have carried out its epidemiology and the associated health consequences in endemic populations, and no simple and effective drug therapy for treatment and control of the infection has been identified. A study in Cameroon revealed that M. perstans is mainly prevalent in equatorial rainforest and that ivermectin mass drug administration is not effective (11), whereas doxycycline can reduce microfilariae (mf; the worm’s offspring) in the peripheral blood (12, 13) confirming that M. perstans harbors Wolbachia endosymbionts (14, 15).
M. perstans is transmitted by Culicoides and their abundance and seasonal occurrence depends on habitats close to streams, ponds and marshes (16, 17). Recently, we revealed that C. milnei, a nocturnal species, is the major vector of M. perstans in the South-West and Littoral regions of Cameroon (18, 19), which transmits infective larvae (L3) that develop into adult worms which reside in body cavities like peritoneum, pleura and pericardium. Thus, adult worms have only been recovered on rare occasions and consequently research about the development of the different life stages remains limited. Therefore, in vitro cultures provide a crucial tool to unravel many open questions about this filarial nematode. Indeed, we established in vitro cultures of M. perstans microfilariae and adult worms to study the biology of the filarial nematode and test anti-filarial drugs (20–22). However, the implementation of in vitro culture depends on the collection of engorged Culicoides and the isolation of infective larvae, which is a sophisticated and difficult process, since it requires knowledge about the vector and its biting behavior, techniques to collect and maintain engorged midges and isolate L3 from the different body parts. To overcome this issue, we established and optimized the procedure implemented from our previous publications (20, 22) to scale up the production of M. perstans infective larvae, which will facilitate in vitro cultures and large-scale drug testing approaches as well as provide a platform for the implementation of murine M. perstans infection models.
Ethical clearance was obtained from the Cameroon National Ethics Committee, Yaoundé (REF: N° 2022/12/1506/CE/CNERSH/SP) and administrative clearance from the Delegation of Public Health, Littoral Region (Re: DD20/L/MSP/DRSPL/CDS) after approval of the protocol. Special consideration was taken to minimize any health risks to the participants and involvement was strictly voluntary. The objectives of the study were explained in detail to each participant who signed a consent form. The participant’s documents were given a code to protect the integrity of the study subjects. At the end of the study, the participants were treated with doxycycline (200mg daily for 28 days).
The study was carried out in the Yabassi health district (Yabassi Sub-Division), situated in the Nkam Division in the Littoral Region of Cameroon. The study site in the equatorial rain forest lies between latitudes 4°27’16” N and longitudes 9°58’56” E (Figure 1). It experiences an equatorial climate with a long rainy season (April to November) and a short dry season (December to March) with an average annual temperature of 26.8°C. Moderate temperatures range from 23-25°C and mean annual rainfall of 2-4mm. The average annual relative humidity is 80.8%. The main geographical feature is the Nkam River valley, flanked on both sides by a gently undulating plain with an average altitude of 1000m above sea level. The total population was estimated at 12,000 inhabitants, who are mainly farmers (small cash crop plantations of cocoa, palm oil plantains, bananas) and traders, while some are involved in hunting and fishing activities.
Culicoides species were collected using the drop trap technique (18, 19). Microfilarial (mf)-positive volunteers (≥ 21-year-old males) were assessed by thick blood smear technique. The volunteers (two per session, having ≥520mf/ml of blood) were placed under a rectangular netting cage trap (3x2x2x2m), which was raised for 15- 20 min to allow contact between the host and midges and then lowered to trap the attracted midges. After about 15-20 mins (the expected time for most of the trapped night-biting midges to be fully blood-fed), the engorged Culicoides species trapped on the netting materials were gently aspirated by a well-trained fly collector technician with a locally made mechanical aspirator and blown into 50 ml Falcon tubes (Merck KGaA, Darmstadt, Germany), 3/4 filled with plaster of Paris (POP). Using a bright torch light, fully fed midges were separated from (aspirated) non-engorged or partially engorged midges into a different tube and labeled. Fully engorged midges could be seen from the blood-filled reddish abdomen. The POP formed an absorbent layer at the bottom of the tubes to retain moisture. Biting midge collections were performed between 6 p.m. and 6 a.m. and caught midges were maintained in the tubes for 3-5 days in the field as previously described (23). The collected biting midges were fed with 15% sugar solution and then transported to the main research laboratory in Manjo in a cool box for maintenance.
Culicoides species were maintained at 24°C and relative humidity (RH) of 75 ± 5% in the insectarium at the Manjo laboratory (Figure 2). In short, a hole (0.5 cm in diameter) was made in the lid of each rearing tube to permit ventilation and feeding of the midges in captivity. This hole was covered with fine netting (pore size 0.5 mm) on which the sugar solution-moistened cotton was placed for midge-feeding. Engorged Culicoides were fed daily with 15% sugar solution soaked in cotton gauze. Additionally, 3–4 drops of distilled water were added daily using a 10 ml syringe to keep the bottom of the tubes moist. To record midge survival, the rearing tubes were inclined and slightly agitated. Generally, insects have the tendency of moving upward, especially in a closed system. With this in mind, we could easily determine motile and immotile midges. The immotile midges were considered dead after about 10-20 seconds of observation. Dead midges were removed from the tubes daily and stored in 80% alcohol. Mortality of the midges was recorded daily and immobile midges were declared dead after observing them for one minute.
Morphological identification was done on euthanized engorged Culicoides species following the examination of the wing pigmentation pattern and male genitalia under a dissecting microscope using a combination of identification keys (24, 25).
After a total of 12 and 14 days of maintenance in the insectarium, the live Culicoides species were knocked down in tween 20-killing solution for 1-2 minutes and rinsed in distilled water for approximately 15-30 seconds. The dead midges were placed on sterile microscope slides containing a drop of the dissecting medium, RPMI-1640 or DMEM (Sigma-Aldrich, Munich, Germany) supplemented with a 2% antibiotic cocktail (penicillin-streptomycin-neomycin; Thermo Fisher Scientific, Schwerte, Germany).
Under the dissecting microscope (Motic, Wetzlar, Germany), the head, thorax and abdomen of the knocked down midges were separated and teased just after morphological identification. This set-up allowed for 1-2 minutes for the larvae to migrate out of the various anatomical regions. All infective larvae (L3) were isolated into sterile dissecting wells labeled head (H), thorax (T) and abdomen (A) containing the same medium.
Data were collected daily on record sheets and entered into a template designed on Microsoft Office Excel 2007 (Microsoft, Redmond, USA). Analyses were performed using R Statistical Language version 4.4.0 (26). Descriptive analysis was conducted to illustrate the number of collected midges per collection season, and per month, as well as the number of live and dead midges dissected, the numbers of recovered L3 from dissected midges per donor and sampling date and Culicoides body part (head, thorax, and abdomen). The ggplot2 (27) package was used for data visualization. A Mann-Kendall Trend test was used to determine whether the trend was monotonic in time series data (mean daily collection of midges at different months, monthly dissection of C. milnei, C. grahamii and others). The association between the donor’s load and midge survival was investigated by fitting a Cox proportional hazard regression model (also referred to as Cox regression in this document) with “time to death” as the outcome variable and the donor’s microfilaria load as a predictor variable using the function coxph from the package survival 3.7-0 (28, 29). The model was then used to determine the predicted survival probability, and visualized with Kaplan Meier curves using the package survminer 0.4.9 (30). The number of recovered L3 produced by infected live midges was compared among donors using the Kruskal-Wallis rank sum test, followed by Dunn’s multiple comparison test using rank sums with Bonferroni adjustment when the former test revealed a significant difference (p-value<0.05).
A total of 13,658 engorged Culicoides (midges) were collected from February 2022 to January 2023. Five Culicoides species were identified namely; C. milnei, C. grahami, C. inornatipennis, C. fulvithorax and C. neavei as anthropophilic night biters. The mean parasite load of the six different donors, their respective ages and the period of midge collection are shown in Table 1. Two donors were used per session and the mean parasite load of the donors were quantified every 3 months throughout the study period.
The months with the highest number of days of midge collection were March 2022 and October 2022 with 14 days of collection each, while during the months of December 2022 and January 2023 only 3 days of collection each was performed. In total, 13,658 midges were collected within 112 days between February 2022 and January 2023 (Table 2). In general, two donors per week always participated in the midge collection
The average daily collection of engorged Culicoides was analyzed by calculating the number of engorged midges collected during a month divided by the number of days of collection of the respective month. In general, we grouped the months into seasons (Figure 3) and differentiated them into the early dry season (mid-October, November, early December), peak dry season (mid-December, January, February, early March), early rainy season (mid-March, April, early May) and peak rainy season (mid-May, June, July, August, September, early October). February to May (early and peak dry season) showed the lowest values of average daily collection of midges with a weak and gradual increase (33.45 in February, 35.57 in March, 45.44 in April, and 51.50 in May). The increase became more evident from June to October (152.17 in June and 179.21 in October) with slight fluctuations (peak rainy season). The peak average daily midge collection was observed in December (457 midges per day), an early dry season month with low or no rainfall and moderate temperatures (Table 2; Figure 3). After the early dry season, we noticed a drastic drop in collection in January which is the peak dry season. A Mann-Kendall trend test revealed the presence of a monotonic trend (p-value = 0.002), that is the direction of variation (increase here) in the daily average collection remained consistent throughout months of collection.
Figure 3. Variation of average daily collection of midges at different months. Midge collection took place from February 2022 to January 2023 and the year is divided into four seasons: the early dry (mid-October to mid-December, ~2 months), the peak dry (mid-December to mid-March, ~3 months), the early rainy (mid-March to mid-May, ~2 months), and the peak rainy (mid-May to mid-October, ~5 months) seasons. Points represent the average and error bars (in red) represent the standard error of the mean.
The average daily dissection of midges was higher throughout the year in C. milnei as compared to all other species combined (Figure 4). C. milnei displayed a gradual increase in average daily dissection from the peak dry season to the rainy seasons (early and peak) with a drop in September. From the peak rainy season to the early dry season, there is a sharp rise in December (400 midges/day) followed by a drastic drop in January (150 midges per day, the peak dry season. Mann-Kendall trend test revealed a consistent increase in the daily average dissection of midges through months for C. milnei (p-value = 0.003), while other species did not demonstrate a monotonic variation (p-value = 0.45). The trend in other species combined is similar, but we noticed a drop in the number of midges dissected per day during the peak rainy season. There was no monotonic evolution (Mann-Kendall trend test, tau = 0.18, p=0.45) of the average daily dissection of C. grahamii and other midges combined (Figure 4).
Figure 4. Variation of average daily dissection of midges at different months for C. milnei (in red) and other species (in blue). Midge collection took place from February 2022 to January 2023. The year is divided into four seasons: the early dry (mid-October to mid-December, ~2 months), the peak dry (mid-December to mid-March, ~3 months), the early rainy (mid-March to mid-May, ~2 months), and the peak rainy (mid-May to mid-October, ~5 months) seasons. Points represent the average and error bars (in red) represent the standard error of the mean. Points represent the average for the respective species and error bars (in black) represent the standard error of the mean for the associated point.
We investigated the survival over the rearing duration (12 and 14 days) of engorged Culicoides from the different donors using a Cox proportional hazard regression model. In total, six different donors coded AA-FF participated in the study. We assessed the survival probability of engorged Culicoides species 12 days post-infection. At the end of rearing for 12 days post-infection, we obtained some stage two larvae (L2) (Supplementary Table 1). We extended the rearing period to 14 days to permit the development of L3 larvae. Overall, we recorded a gradual decrease in the predicted survival probability until 78.5% at the end of 14 days of laboratory maintenance (Figure 5).
Figure 5. Overall predicted survival probability of engorged Culicoides midges during the laboratory rearing. The curve is derived from a Cox Hazard proportional regression model with “time (in days) to death” as the outcome variable and the donor microfilaria load as the predictor variable.
In general, five out of six donors had predicted survival probability of 95% (Figure 6) and above for the first six days of the rearing process. At the end of the study, we observed that midges that fed on donor FF (16,540mf/ml) with the highest microfilaria load had the lowest predicted survival probability when compared with midges that fed on donor AA (520mf/ml) with the lowest microfilaria load. In contrast, midges that fed on donors with intermediate microfilaria loads (between the two extremes) had clustered values and progression of predicted survival. Of note, the predicted survival probability of the Culidoides species that fed on the donors EE (8,000 mf/ml) and BB (6,000 mf/ml), were assessed for 12 days post-infection, while the survival probability of biting midges engorged from donors AA, CC, DD and FF was assessed for 14 days post-infection. During the 14-day rearing period, Culicoides species which fed on donor AA (520mf/ml) showed the highest (85.7%) predicted survival probability while engorged midges from donor FF (16,540mf/ml) presented the lowest (75.1%) predicted survival probability amongst the four donors (AA, CC, DD, FF) that had the same rearing duration. The biting midges engorged from donor BB (6,000mf/ml) showed a slightly higher (85.2%) survival probability when compared to donor EE (8,000mf/ml; 84.1%) which were both reared for 12 days. There was a statistically significant (log-rank test, p-value<.0.0001) variation in the midges predicted survival probability to donor microfilaria load. Indeed, midges that fed on donors with low microfilaria load survived more under laboratory maintenance (85.7%) than those fed on highly infected donors (75.1%). In other words, the donor microfilaria load was negatively associated with midge survival (Figure 6).
Figure 6. Predicted survival probability of engorged Culicoides midges from the different donors during the laboratory rearing. The log-rank test revealed a significant variation (p<2e-16) in the predicted survival probability of the donor microfilaria load. The curve is derived from a Cox Hazard proportional regression model with “time (in days) to death” as the outcome variable and the donor microfilaria load as the predictor variable.
Culicoides collection and isolation of infective larvae from dissected live and dead midges differs between the six donors. To decipher whether a high microfilaria load is associated with dead midges, we dissected dead midges mainly from late July 2022 to January 2023 (dead midges from donor AA were not analyzed). Donor FF (who participated in the study from May 2022 to January 2023) recorded the highest number of engorged midges (n=7,294) caught and dissected both alive (n=3,931) and dead (n=619), followed by donor DD with 4,021 engorged Culicoides collected from which 2,432 alive and 144 dead midges were obtained (Figure 7). We collected (136) and dissected (101 live midges and 4 dead midges) the least from donor BB (March to May 2022).
Figure 7. Distribution of collected and dissected (alive and dead) Culicoides midges among the different donors.
Out of the five engorged live Culicoides species (C. milnei, C. grahamii, C. inornatipennis, C. fulvithorax and C. neavei) identified from the donors after 12 and 14 days of laboratory maintenance, three were common in all six donors but C. neavei was only obtained from donor DD and EE (Figure 8A). C. milnei was the most abundant species across all the donors accounting for more than 50% of midges identified and dissected for every donor. C. milnei accounted the highest for donor DD (94.74%) followed by 93.16% for donor FF for all midges dissected. Overall, C. milnei contributed 87.23% of all live midges dissected in this study (Figure 8A). In contrast, only four Culicoides species (Figure 8B) were dissected dead from the donors (C. neavei absent). C. milnei accounted for more than 97.13% of all dead midges dissected of four out of five donors (donor BB, 25%) with the highest from donor FF (98.87%) followed by 97.22% from donor DD.
C. milnei accounted for 98.7% (n=6,310 L3) of total L3 recovered, while for dead dissected midges, 99.92% L3 recovery was obtained from the same species (Figure 9A). Throughout the dissection of live and dead midges (Figure 9B), L3 was recovered more from the head (62.54% and 66.11% in live and dead midges, respectively), followed by thorax (28.29% and 28.9% in live and dead midges, respectively) and lastly by abdomen (9.18% and 4.99% in live and dead midges, respectively). The donor FF (16,540mf/ml) with the highest microfilaria load contributed the highest to L3 production (83.65%) followed by donor DD (7,640mf/ml) with 9.05% during dissection of live midges. This was similar to results from the dissection of dead midges in which donor FF still contributed the highest to L3 recovery of 94.48% (Figure 9C).
Figure 9. Contribution of dissected Culicoides species to L3 production. (A) Percentage of L3 found per live and dead Culicoides species dissected. (B) Percentage of L3 found per dissected midge body part in live and dead midges. (C) Percentage of L3 found per donor in live and dead midges.
The overall L3/infected midges from the head, thorax and abdomen (combined) for donors AA, BB, CC, DD and EE was significantly different for midges that fed on donor FF as compared to all other donors (Kruskal-Wallis, P<2.2e-16, Figure 10A). In the head, a significant difference of recovered L3 from infected midges was found between donor FF and all other donors, except donor EE (Kruskal-Wallis, P<2.2e-16, Figure 10B). The L3 from the thorax of infected midges from various donors varied significantly (Kruskal-Wallis, p=1.3e-07, Figure 10C) and the source of this variation was identified between the pairs BB-FF and DD-FF. The L3/infected midge from the abdomen showed no significant difference (Kruskal-Wallis, p=0.53) amongst the donors. No L3 was recovered from the abdomen of live midges that were fed on donor AA and BB. The highest L3 recovered from infected midges was on donor FF for all anatomical regions (head, thorax and abdomen). An overview of live and dead midges and collected L3 from the different donors and body parts of the midges are shown in Tables 3 and 4, respectively. Moreover, a spreadsheet about individual results from the midges is shown in the Supplementary Material.
Figure 10. Distribution of L3 per infected live midge. (A) The overall distribution of recovered L3 from live midges. (B) Distribution of recovered L3 from the head of live midges. (C) Distribution of recovered L3 from the thorax of live midges. (D) Distribution of recovered L3 from the abdomen of live midges. The number of recovered L3 produced by infected live midges was compared among donors using the Kruskal-Wallis rank sum test, followed by Dunn’s multiple comparisons test using rank sums with Bonferroni adjustment when the former test was significant (p-value<0.05).
Table 3. Overview of live Culicoides spp and isolated M. perstans infective larvae from the various parts of the midges.
Table 4. Overview of dead Culicoides spp. and isolated M. perstans infective larvae from the donors BB, CC, DD, EE and FF during collection months February to April 2022 and June to November 2022 (No., number; H, Head; T, Thorax; A, Abdomen).
As depicted in Table 3, out of the 13,658 midges that were collected, a total of 8,123 Culicoides survived the rearing and were dissected over a period of 12 months (February 2022-January 2023). In total, we recovered 6,310 L3 from 2,335 M. perstans-infected midges, resulting in 2.70 L3 per infected midge. A total of 7,086 dissected C. milnei were responsible for 98.7% (6,228 L3) of total L3 recovered. Only 58 L3 were obtained from 690 C. grahamii dissections. In addition, 12 L3 each were obtained from 199 C. inornatipennis and 145 C. fulvithorax. C. neavei (3 collected) were all negative for M. perstans L3 (Table 3). In total, 459 L2 and no L1 were obtained during the dissection process (Supplementary Table 1). The majority of infective larvae were obtained from the head region (n=3,946), followed by thorax (n=1,785) and the abdomen (n=579; Table 3). Moreover, we analyzed dead midges from the donors’ BB, CC, DD, EE, FF (Table 4). In total, 800 dead midges have been analyzed during the collection months of February to April 2022 and June to November 2022. In total 1,322 L3 was recovered from all dead midges but 1,321 have been obtained solely from dead C. milnei midges and 1 L3 from C. grahamii (Table 4). The graphical representation of various replicates and, detailed numbers about infected dead midges and L3s, dissected dead midges and recovered dead L3 from the anatomical regions are shown in Supplementary Figure 1 and Supplementary Tables 2–4 respectively. From dead midges dissected, we recorded 377 infected dead midges. A total of 1,249 dead L3 were obtained from donor FF, who had a high mircofilaria load. In contrast, only 32 dead L3 was obtained from 73 dead infected midges out of 181 dead midges dissected from donor BB, CC, DD and EE combined, who had lower microfilaria numbers in the periphery.
In this study, we established that M. perstans infective larvae can be produced in a large scale from engorged Culicoides milnei using a drop trap technique. Important factors for the efficacy of L3 collection were the session of collection, the microfilaria load of the donor, and the duration of laboratory rearing. Indeed, environmental factors play a crucial role in the transmission of filariae species influencing vector transmission dynamic and fitness and consequently, the efficacy of infective larvae transmission (31, 32), but these factors remain uncertain for M. perstans transmission. Previously, 13 Culicoides spp were identified in Cameroon (24, 25). Here we could show that 5 species (C. milnei, C. grahamii, C. inornatipennis, C. fulvithorax and C. neavei) fed on the donors. C. milnei was the most abundant species collected from which 98.7% (n=6,228) M. perstans L3 were obtained, confirming that this midge species is the major vector for M. perstans transmission in the South-West and Littoral region of Cameroon (18, 19).
Six microfilaria-positive individuals participated in this study and Culicoides midges were collected using the drop trap technique (18, 19). The midges were reared in the insectarium to recover M. perstans L3 from the various parts of the midges. We obtained that extension of the laboratory rearing from 12 to 14 days increases the rates of L3 collection. Within the 12 months of the study, we collected 13,658 midges from which 2,328 (17.04%) harbored 6,294 L3, giving a total of 3 L3 per infected midge. A study with wild-caught Chrysop flies within a 12 months study period in Gabon obtained 50 L3 per infected fly (33). The differences in the L3 rate per vector can be explained by the large size of the Chrysop fly compared to the tiny Culicoides midge. Thus, Chrysop fly can take up more blood and larvae. In contrast to the lower L3 infection rate per midge, we observed a higher midge survival probability of 78.5% compared to the Chrysops survival rate of 43% (33). However, the donor with a high microfilaria load (16,540 mf/ml) had a significantly lower survival probability of engorged midges compared to donors with lower microfilaria loads (520-7,360 mf/ml). These findings confirm our results of higher mortality rates of L3 in Chrysops flies that were infected with 100 Loa loa mf compared to inoculation of 50 Loa loa microfilariae (34). Another study showed that increasing the dose of Dirofilaria immitis microfilariae increases the mortality of Aedes aegypti (35). These findings highlight that infectious dose could be a major factor affecting the survival of the vectors during laboratory rearing.
Vector mortality is a limiting factor of nature in filarial transmission. It has been observed that all the microfilariae-infected midges cannot survive the period which is required for the development of L3 (36). Indeed, further analysis revealed that the majority of dead infected midges have been obtained from the donor with high microfilaria loads in the peripheral blood, suggesting that increased infection dose led to increased mortality of the midges. Production of L3 requires optimal laboratory rearing conditions but also presupposes a balanced selection of volunteers with moderate to high microfilaria numbers (7,000 – 16,000 mf/ml). These findings have been confirmed by other studies about the Loa loa vector mortality since it has been suggested that the emergence of Loa loa L3 from the Chrysops head incurs a significant amount of excess mortality (37) and that Loa loa infection per se but also intensity of infection influence vector mortality (38). Similarly, another study showed that the emergence of Onchocerca volvulus larvae from the head causes great irritation to the fly, eliciting proboscis ‘milking’ actions associated with stress, and damage to the labio-hypopharyngeal membrane and musculature thereby causing its death and overall high mortality rates of the vector (39). Despite the higher mortality rate of midges from donors with high microfilaria load, we determined that midges surviving to day 14 yielded significantly higher numbers of L3 as compared to midges from donors with low to medium parasite densities, confirming results from Wuchereria bancrofti infections (40). In addition, a study using the rodent Bdellonyssus bacoti mites showed that an increase of microfilariae did not result in a proportional increase in the number of infective larvae of Litomosoides galiza (41).
In regards to the collection and survival of the midges, we observed the lowest collection rate (33 collected midges/day) in February, which is the peak dry season characterized by no rainfall. However, the weather is dry thereby creating a warmer environment for the midges which might reduce their abundance and may have affected their survival before being transported to the laboratory for rearing. The month of December (early dry season) recorded the highest collection of midges (457 collected midges per day), which is characterized by little or no rainfall and moderate temperatures. Moderate temperatures and low rainfall might support vector survival and abundance have been confirmed with the filarial vector Culex quinquefasciatus, showing lower vector density in the rainy season in comparison to dry seasons in different endemic areas of the tropics (42). Indeed, environmental factors like temperature, humidity, wind and rainfall have been shown to affect the survival and activity of Culicoides midges (43) and it is suggested that midges can better survive in moderate temperatures (44, 45).
Owing to the significance of blood-feeding in the life cycle of the midge, it is an important component for mansonellosis transmission between human and vector hosts. The efficiency of the passage can be influenced by many factors such as the distribution and periodicity of microfilariae in capillaries of hosts for vector infection, blood-feeding duration, and frequency of transmission from vector to vertebrate. Obtaining human blood for insectary colony maintenance and larvae production can be problematic due to several ethical and safety issues. To avoid using humans as donors for engorgement of midges, artificial membrane systems can be used for this purpose. Membrane-feeding assays have been widely used in transmission research and insectary colony maintenance, but human blood as bait is still a limited source since blood banks mainly provide blood for medical emergencies rather than experimental work at insectaries. Thus, different baits could be used to minimize the limitations of using human hosts or human blood. Artificial membrane feeder systems have been established to evaluate topical mosquito repellents (46), transmission-blocking drugs and vaccines (47), and endectocides (48). Using artificial membrane feeding systems instead of human donors resolves the ethical issues concerning human welfare and avoids sophisticated field work required for the drop trap technique. Indeed, artificial membrane feeding systems (49, 50) and murine models for blood feeding (51) have been established for Culicoides midges. Therefore, further work should focus on the establishment of an artificial feeding system for Culicoides that can be combined with the proposed conditions for large-scale production of L3. We hope that the shown findings encourage laboratories to conduct colony maintenance, vector-parasite interactions, and entomology-related research by seeking inexpensive and effective artificial membrane feeding methods, along with available blood sources that yield the optimum growth and reproductive rates of the vectors of interest.
Finally, we acknowledge two main limitations of the study. Firstly, the collection of Culicoides midges was performed for only one year and thus sessional fluctuations over several years can influence vector abundance and biting behavior. Long-term studies need to be performed to confirm the findings. Secondly, the majority of Culicoides midges was engorged on the high-load microfilaria donor FF. This donor was readily available and had the longest collection period of engorged midges unlike the other donors leading to a sample bias. Further studies should include donors with different microfilariae loads and collect engorged midges in the early dry session over the same collection time to quantitatively analyze the association of microfilaria load with vector survival and L3 collection.
In this study, we implemented a procedure to collect and maintain engorged midges to scale up the production of M. perstans infective larvae. Furthermore, we showed that the early dry season with little or no rainfall and moderate temperatures are optimal for the collection and laboratory maintenance of the midges. We suggest that production of high yield M. perstans L3 requires a mixed set of moderate and high microfilaria donors. Compliance to these multifactorial conditions allows large-scale production of M. perstans L3, which are urgently needed to implement in vitro culture systems for drug testing approaches and the establishment of M. perstans animal infection models.
The original contributions presented in the study are included in the article/Supplementary Material, further inquiries can be directed to the corresponding author/s.
The manuscript presents research on animals that do not require ethical approval for their study. The studies involving humans were approved by the National Institutional Review board, Yaoundé (REF: N° 2022/12/1506/CE/CNERSH/SP) and the Delegation of Public Health, Littoral Region (Re: DD20/L/MSP/DRSPL/CDS). The studies were conducted in accordance with the local legislation and institutional requirements. Written informed consent for participation in this study was provided by the participants.
CK: Formal analysis, Investigation, Methodology, Writing – original draft, Writing – review & editing. REh: Investigation, Methodology, Writing – original draft, Writing – review & editing. FF: Investigation, Methodology, Writing – original draft, Writing – review & editing. FE: Investigation, Methodology, Writing – original draft, Writing – review & editing. AnN: Investigation, Methodology, Writing – original draft, Writing – review & editing. EO: Investigation, Methodology, Writing – original draft, Writing – review & editing. NG: Conceptualization, Formal analysis, Funding acquisition, Investigation, Methodology, Project administration, Resources, Supervision, Validation, Writing – original draft, Writing – review & editing. VC: Investigation, Methodology, Validation, Writing – original draft, Writing – review & editing. REk: Investigation, Methodology, Writing – original draft, Writing – review & editing. FNN: Investigation, Methodology, Writing – original draft, Writing – review & editing. JF: Investigation, Methodology, Writing – original draft, Writing – review & editing. LN: Investigation, Methodology, Writing – original draft, Writing – review & editing. CM: Investigation, Methodology, Writing – original draft, Writing – review & editing. AbN: Investigation, Methodology, Writing – original draft, Writing – review & editing. PE: Investigation, Methodology, Writing – original draft, Writing – review & editing. AH: Supervision, Validation, Writing – original draft, Writing – review & editing. MR: Conceptualization, Formal analysis, Funding acquisition, Investigation, Project administration, Supervision, Writing – original draft, Writing – review & editing. SW: Conceptualization, Formal analysis, Funding acquisition, Investigation, Methodology, Project administration, Resources, Supervision, Validation, Writing – original draft, Writing – review & editing.
The author(s) declare financial support was received for the research, authorship, and/or publication of this article. This work is funded through a grant awarded to SW and MR from the Deutsche Forschungsgemeinschaft (DFG) within the “African-German Cooperation Projects in Infectiology” (RI 3036/1-1). AH is additionally supported by the DFG under Germany’s Excellence Strategy -EXC2151 -390873048 and SW is a Senior Fellow Plus of European and Developing Countries Clinical Trial Partnership (EDCTP2).
We sincerely thank the chiefs and entire populations of all health districts surveyed for accepting and participating in this study. The authors are grateful to the participants for their voluntary participation. We also thank the Cameroon health system for all the facilities put in place during the surveys.
The authors declare that the research was conducted in the absence of any commercial or financial relationships that could be construed as a potential conflict of interest.
All claims expressed in this article are solely those of the authors and do not necessarily represent those of their affiliated organizations, or those of the publisher, the editors and the reviewers. Any product that may be evaluated in this article, or claim that may be made by its manufacturer, is not guaranteed or endorsed by the publisher.
The Supplementary Material for this article can be found online at: https://www.frontiersin.org/articles/10.3389/fitd.2024.1391823/full#supplementary-material
1. Mourembou G, Fenollar F, Lekana-Douki JB, Ndjoyi Mbiguino A, Maghendji Nzondo S, Matsiegui PB, et al. Mansonella, including a potential new species, as common parasites in children in Gabon. PloS Negl Trop Dis. (2015) 9:e0004155. doi: 10.1371/journal.pntd.0004155
2. Simonsen PE, Onapa AW, Asio SM. Mansonella perstans filariasis in Africa. Acta Trop. (2011) 120 Suppl 1:S109–20. doi: 10.1016/j.actatropica.2010.01.014
3. Orihel TC, Eberhard ML. Zoonotic filariasis. Clin Microbiol Rev. (1998) 11:366–81. doi: 10.1128/CMR.11.2.366
4. Ritter M, Hoerauf A, Hubner MP. Human filariasis. In: Encyclopedia of infection and immunity elsevier, vol. 2. Amsterdam, Netherlands. (2021).
5. Ritter M, Ndongmo WPC, Njouendou AJ, Nghochuzie NN, Nchang LC, Tayong DB, et al. Mansonella perstans microfilaremic individuals are characterized by enhanced type 2 helper T and regulatory T and B cell subsets and dampened systemic innate and adaptive immune responses. PloS Negl Trop Dis. (2018) 12:e0006184. doi: 10.1371/journal.pntd.0006184
6. Stensgaard AS, Vounatsou P, Onapa AW, Utzinger J, Pedersen EM, Kristensen TK, et al. Ecological drivers of Mansonella perstans infection in Uganda and patterns of co-endemicity with lymphatic filaria-sis and malaria. PLoSNegl Trop Dis. (2016) 10:e0004319. doi: 10.1371/journal.pntd
7. Muhangi L, Woodburn P, Omara M, Omoding N, Kizito D, Mpairwe H, et al. Associations between mild-to-moderate anaemia in pregnancy and helminth, malaria and HIV infection in Entebbe, Uganda. Trans R Soc Trop Med Hyg. (2007) 101:899–907. doi: 10.1016/j.trstmh.2007.03.017
8. Hillier SD, Booth M, Muhangi L, Nkurunziza P, Khihembo M, Kakande M, et al. Plasmodium falciparum and helminth coinfection in a semi urban population of pregnant women in Uganda. J Infect Dis. (2008) 198:920–7. doi: 10.1086/591183
9. Elliott AM, Mawa PA, Webb EL, Nampijja M, Lyadda N, Bukusuba J, et al. Effects of maternal and infant co-infections, and of maternal immunisation, on the infant response to BCG and tetanus immunisation. Vaccine. (2010) 29:247–55. doi: 10.1016/j.vaccine.2010.10.047
10. Nelson GS. Filarial infections as zoonoses. J Helminthol. (1965) 39:229–50. doi: 10.1017/s0022149x00020630
11. Wanji S, Tayong DB, Layland LE, Datchoua Poutcheu FR, Ndongmo WP, Kengne-Ouafo JA, et al. Update on the distribution of Mansonella perstans in the southern part of Cameroon: influence of ecological factors and mass drug administration with ivermectin. Parasit Vectors. (2016) 9:311. doi: 10.1186/s13071-016-1595-1
12. Coulibaly YI, Dembele B, Diallo AA, Lipner EM, Doumbia SS, Coulibaly SY, et al. A randomized trial of doxycycline for Mansonella perstans infection. N Engl J Med. (2009) 361:1448–58. doi: 10.1056/NEJMoa0900863
13. Batsa Debrah L, Phillips RO, Pfarr K, Klarmann-Schulz U, Opoku VS, Nausch N, et al. The efficacy of doxycycline treatment on mansonella perstans infection: an open-label, randomized trial in Ghana. Am J Trop Med Hyg. (2019) 101:84–92. doi: 10.4269/ajtmh.18-0491
14. Keiser PB, Coulibaly YI, Kubofcik J, Diallo AA, Klion AD, Traoré SF, et al. Molecular identification of Wolbachia from the filarial nematode Mansonella perstans. Mol Biochem Parasitol. (2008) 160:123–8. doi: 10.1016/j.molbiopara.2008.04.012
15. Hoerauf A. Mansonella perstans–the importance of an endosymbiont. N Engl J Med. (2009) 361:1502–4. doi: 10.1056/NEJMe0905193
16. Mellor PS, Boorman J, Baylis M. Culicoides biting midges: Their role as arbovirus vectors. Annu Rev Entomol. (2000) 45:307–40. doi: 10.1146/annurev.ento.45.1.307
17. Meiswinkel R, Venter GJ, Nevill EM. Vectors: culicoides spp. In: Croetzer JAW, Tustin RC, editors. Infectious diseases of livestock, 2nd. Oxford University Press, Cape Town (2004).
18. Wanji S, Tayong DB, Ebai R, Opoku V, Kien CA, Ndongmo WPC, et al. Update on the biology and ecology of Culicoides species in the South-West region of Cameroon with implications on the transmission of Mansonella perstans. Parasit Vectors. (2019) 12:166. doi: 10.1186/s13071-019-3432-9
19. Ebai R, Kien CA, Fombad FF, Esofi F, Ouam E, Ntuh AN, et al. Culicoides species of the Rain Forest Belt of the Littoral Region of Cameroon: Their Incrimination in the Transmission of Mansonella perstans. Pathogens. (2024) 13:146. doi: 10.3390/pathogens13020146
20. Njouendou AJ, Ritter M, Ndongmo WPC, Kien CA, Narcisse GTV, Fombad FF, et al. Successful long-term maintenance of Mansonella perstans in an in vitro culture system. Parasit Vectors. (2017) 10:563. doi: 10.1186/s13071-017-2515-8
21. Njouendou AJ, Kien CA, Esum ME, Ritter M, Chounna Ndongmo WP, Fombad FF, et al. In vitro maintenance of Mansonella perstans microfilariae and its relevance for drug screening. Exp Parasitol. (2019) 206:107769. doi: 10.1016/j.exppara.2019.107769
22. Njouendou AJ, Ritter M, Kien CA, Esum ME, Ndongmo WPC, Fombad FF, et al. Dataset on in vitro maintenance of Mansonella perstans microfilariae and drug testing. Data Brief. (2019) 28:104930. doi: 10.1016/j.dib.2019.104930
23. Hopkins C. Notes on the biology of certain Culicoides studied in the British Cameroons, West Africa, together with observations on their possible role as vectors of Acanthocheilonema perstans. Ann Trop Med Parasitol. (1952) 46:165–72. doi: 10.1080/00034983.1952.11685521
24. Glick JI. Culicoides biting midges (Diptera: Ceratopogonidae) of Kenya. J Med Entomol. (1990) 27:85–195. doi: 10.1093/jmedent/27.2.85
25. Boorman J, Dipeolu OO. A taxonomic study of adult Nigerian Culicoides latreille (Diptera: Ceratopogonidae) species. 22. Makurdi: Entomological Society of Nigeria (1979).
26. R Core Team. R: A language and environment for statistical computing (2024). Available online at: https://www.r-project.org/ (accessed April 24, 2024).
27. Wickham H. ggplot2: elegant graphics for data analysis. Cham, Switzerland: Springer-Verlag New York (2016). Available at: https://ggplot2.tidyverse.org.
28. Therneau T. A Package for Survival Analysis in R. R package version 3.7-0 (2024). Available online at: https://CRAN.R-project.org/package=survival (accessed June 1, 2024).
29. Therneau TM, Grambsch PM. Modeling survival data: extending the cox model. New York: Springer (2000), ISBN: 0-387-98784-3.
30. Kassambara A, Kosinski M, Biecek P. survminer: Drawing Survival Curves using ‘ggplot2’. R package version 0.4.9 (2021). Available online at: https://CRAN.R-project.org/package=survminer (accessed June 1, 2014).
31. Bockarie MJ, Pedersen EM, White GB, Michael E. Role of vector control in the global program to eliminate lymphatic filariasis. Annu Rev Entomol. (2009) 54:469–87. doi: 10.1146/annurev.ento.54.110807.090626
32. Snow LC, Bockarie MJ, Michael E. Transmission dynamics of lymphatic filariasis: vector-specific density dependence in the development of Wuchereria bancrofti infective larvae in mosquitoes. Med Vet Entomol. (2006) 20:261–72. doi: 10.1111/j.1365-2915.2006.00629.x
33. Wahl G, Moukagni R, Toure F, Georges AJ. Large scale collection of viable infective larvae of Loa loa. Trop Med Parasitol. (1995) 46:203–4.
34. Ndzeshang LB, Fombad FF, Njouendou AJ, Chunda VC, Gandjui NVT, Akumtoh DN, et al. Generation of Loa loa infective larvae by experimental infection of the vector, Chrysops silacea. PloS Negl Trop Dis. (2020) 14:e0008415. doi: 10.1371/journal.pntd.0008415
35. McCrea AR, Edgerton EB, Oliver GT, O'Neill FM, Nolan TJ, Lok JB, et al. A novel assay to isolate and quantify third-stage Dirofilaria immitis and Brugia malayi larvae emerging from individual Aedes aEgypti. Parasit Vectors. (2021) 14:30. doi: 10.1186/s13071-020-04529-w
36. Yamamoto H, Kobayashi M, Ogura N, Tsuruoka H, Chigusa Y. Studies on filariasis. VI. The encapsulation of Brugia malayi and B. pahangi larvae in the mosquito, Armigeres subalbatus. Jap J Sanit Zool. (1985) 36:1–6. doi: 10.7601/mez.36.1_1
37. Duke BOL. The transmission of loiasis in the forest fringe area of the British Cameroons. Ann Trop Med Parasitol. (1954) 48:349–55. doi: 10.1080/00034983.1954.11685634
38. Pinder M. The improvement of maintenance conditions for wild-caught Chrysops silacea and the production of infective larvae of Loa loa. Acta Trop. (1991) 49:305–11. doi: 10.1016/0001-706x(91)90081-t
39. Basáñez MG, Townson H, Williams JR, Frontado H, Villamizar NJ, Anderson RM. Density-dependent processes in the transmission of human onchocerciasis: relationship between microfilarial intake and mortality of the simuliid vector. Parasitology. (1996) 113:331–55. doi: 10.1017/s003118200006649x
40. Dickerson JW, Eberhard ML, Lammie PJ, Roberts JM. Further evidence of a skewed distribution of microfilariae in capillary blood. Trop Med Parasitol. (1989) 40:472–3.
41. Diagne M, Petit G, Liot P, Cabaret J, Bain O. The filaria Litomosoides galizai in mites; microfilarial distribution in the host and regulation of the transmission. Ann Parasitol Hum Comp. (1990) 65:193–9. doi: 10.1051/parasite/1990654193
42. Chandra G. Nature limits filarial transmission. Parasit Vectors. (2008) 1:13. doi: 10.1186/1756-3305-1-13
43. Purse BV, Carpenter S, Venter GJ, Bellis G, Mullens BA. Bionomics of temperate and tropical. Culicoides midges: knowledge gaps and consequences for transmission of Culicoides-borne viruses. Annu Rev Entomol. (2015) 60:373–92. doi: 10.1146/annurev-ento-010814-020614
44. Wittmann EJ, Mellor PS, Baylis M. Effect of temperature on the transmission of orbiviruses by the biting midge, Culicoides sonorensis. Med Vet Entomol. (2002) 16:147–56. doi: 10.1046/j.1365-2915.2002.00357.x
45. Lysyk TJ, Danyk T. Effect of temperature on life history parameters of adult Culicoides sonorensis (Diptera: Ceratopogonidae) in relation to geographic origin and vectorial capacity for bluetongue virus. J Med Entomol. (2007) 44:741–51. doi: 10.1603/0022-2585(2007)44[741:eotolh]2.0.co;2
46. Debboun M, Wagman J. In vitro repellency of N,N-diethyl-3-methylbenzamide and N,N-diethylphenylacetamide analogs against Aedes aEgypti and Anopheles stephensi (Diptera: Culicidae). J Med Entomol. (2004) 41:430–4. doi: 10.1603/0022-2585-41.3.430
47. Vallejo AF, Rubiano K, Amado A, Krystosik AR, Herrera S, Arévalo-Herrera M. Optimization of a Membrane Feeding Assay for Plasmodium vivax Infection in Anopheles albimanus. PloS Negl Trop Dis. (2016) 10:e0004807. doi: 10.1371/journal.pntd.0004807
48. Smit MR, Ochomo EO, Aljayyoussi G, Kwambai TK, Abong’o BO, Bousema T, et al. Human direct skin feeding versus membrane feeding to assess the mosquitocidal efficacy of high-dose ivermectin (IVERMAL trial). Clin Infect Dis. (2019) 69:1112–9. doi: 10.1093/cid/ciy1063
49. Langner KF, Darpel KE, Drolet BS, Fischer A, Hampel S, Heselhaus JE, et al. Comparison of cellular and humoral immunoassays for the assessment of summer eczema in horses. Vet Immunol Immunopathol. (2008) 122:126–37. doi: 10.1016/j.vetimm.2007.11.001
50. Rozo-Lopez P, Londono-Renteria B, Drolet BS. Venereal transmission of vesicular stomatitis virus by culicoides sonorensis midges. Pathogens. (2020) 9:316. doi: 10.3390/pathogens9040316
Keywords: Mansonella perstans, infective stage 3 larvae (L3), Culicoides milnei, midges, vector abundance, survival and mortality of Culicoides species
Citation: Kien CA, Ebai R, Fombad FF, Esofi F, Ntuh AN, Ouam E, Gandjui NVT, Chunda VC, Ekanya R, Nietcho FN, Foyet JV, Nchang LC, Magha C, Njouendou AJ, Enyong P, Hoerauf A, Ritter M and Wanji S (2024) Large-scale production of Mansonella perstans infective larvae from engorged Culicoides milnei. Front. Trop. Dis 5:1391823. doi: 10.3389/fitd.2024.1391823
Received: 26 February 2024; Accepted: 19 November 2024;
Published: 10 December 2024.
Edited by:
Jana Held, University of Tübingen, GermanyReviewed by:
David P. Tchouassi, International Centre of Insect Physiology and Ecology (ICIPE), KenyaCopyright © 2024 Kien, Ebai, Fombad, Esofi, Ntuh, Ouam, Gandjui, Chunda, Ekanya, Nietcho, Foyet, Nchang, Magha, Njouendou, Enyong, Hoerauf, Ritter and Wanji. This is an open-access article distributed under the terms of the Creative Commons Attribution License (CC BY). The use, distribution or reproduction in other forums is permitted, provided the original author(s) and the copyright owner(s) are credited and that the original publication in this journal is cited, in accordance with accepted academic practice. No use, distribution or reproduction is permitted which does not comply with these terms.
*Correspondence: Manuel Ritter, bWFudWVsLnJpdHRlckB1a2Jvbm4uZGU=; Samuel Wanji, c3dhbmppQHlhaG9vLmZy
†These authors have contributed equally to this work
Disclaimer: All claims expressed in this article are solely those of the authors and do not necessarily represent those of their affiliated organizations, or those of the publisher, the editors and the reviewers. Any product that may be evaluated in this article or claim that may be made by its manufacturer is not guaranteed or endorsed by the publisher.
Research integrity at Frontiers
Learn more about the work of our research integrity team to safeguard the quality of each article we publish.