- 1Departamento de Microbiologia, Imunologia e Parasitologia, Universidade Federal de São Paulo - Escola Paulista de Medicina (UNIFESP/EPM), São Paulo, Brazil
- 2Departamento de Parasitologia, Instituto de Ciências Biomédicas, Universidade de São Paulo, São Paulo, Brazil
- 3Instituto de Investigação em Imunologia (III), Instituto Nacional de Ciência e Tecnologia (INCT), São Paulo, Brazil
- 4Instituto Butantan, São Paulo, Brazil
- 5Laboratório de Imunologia, Instituto do Coração (InCor), Hospital das Clínicas- Faculdade de Medicina- Universidade de São Paulo (HCFMUSP), São Paulo, Brazil
- 6Laboratório de Biologia Molecular, Departamento de Medicina, Hospital Universitário, Universidade Federal de Sergipe, Aracaju, Brazil
- 7Laboratório de Investigação Médica (LIM-19), Hospital das Clínicas- Faculdade de Medicina- Universidade de São Paulo (HC- FMUSP), São Paulo, Brazil
Introduction: Zika virus (ZIKV) infection has been associated to Guillain-Barré syndrome in adults and congenital malformations during pregnancy, leading to the manifestation of congenital Zika syndrome (CZS). The ZIKV envelope protein (EZIKV), prominently displayed on the virus surface, is a primary target for the humoral immune response. However, limited information exists regarding its capacity to induce cellular immunity, particularly in pregnant women with a history of ZIKV infection. The EZIKV protein comprises three domains: the central domain (EDI), a dimerization domain (EDII), and a domain responsible for binding to the cell surface receptor (EDIII). To examine the regions of EZIKV targeted by cellular immunity, we examined cellular immune responses in a cohort of mothers infected with ZIKV, whose infants exhibited microcephaly.
Methods: To assess the ZIKV-specific response, we used inactivated virus and different recombinant viral envelope proteins (EZIKV, EDI/IIZIKV and EDIIIZIKV). All women in the study contracted the infection during pregnancy, with 72% experiencing symptoms such as fever, rash, joint pain, and retro-orbital pain. Peripheral blood mononuclear cells (PMBC) were collected post- ZIKV diagnosis confirmation, with a median time of 18 months (IQR 13.5-19) after parturition. Using the ELISpot assay, we quantified specific interferon-gamma (IFNγ) producing cells by stimulating PBMC with either inactivated ZIKV particles or equimolar amounts of recombinant EZIKV, EDI/IIZIKV and EDIIIZIKV.
Results and discussion: Our findings demonstrate the induction of IFN-γ producing cells in PBMC from ZIKV-convalescent mothers, whose infants manifested microcephaly, upon stimulation with both inactivated ZIKV particles and recombinant proteins. The identification of immunodominant regions within ZIKV can contribute for the development of targeted treatments and vaccine candidates tailored for pregnant women.
Introduction
Zika virus (ZIKV), a mosquito-borne flavivirus closely related to yellow fever, dengue, and West Nile viruses (1) has undergone rapid global dissemination since 2015, with over 80 countries reporting local transmission (2). While primarily transmitted by Aedes mosquitos, non-vector transmission can also occur including sexual contact, transfusion, and vertical transmission from mother to child (3, 4).
Most ZIKV infections are asymptomatic, with a minority causing self-limited acute febrile illnesses characterized by fever, headache, arthralgia, myalgia, fatigue, and rash (5). However, in adults, ZIKV infection has been sporadically associated with Guillain-Barré syndrome (GBS) (6, 7). In pregnant women, the virus can persist for weeks in the reproductive tract (8, 9), and fetal infection has been associated with congenital malformations such as brain calcification, microcephaly, and spontaneous abortion, defining the Congenital Zika Syndrome (CZS) (10–12).
Studies suggest that approximately 20% of infants born to mothers exposed to Zika virus during pregnancy, who initially exhibited no signs of birth defects, later displayed impaired cognitive development and other neurological abnormalities (13, 14).
The rapid global spread of ZIKV and its association with neurological complications highlight the urgent need for an effective vaccine and specific treatment. Despite scientific efforts, no licensed therapeutic or prophylactic vaccines against ZIKV have been developed until now (15).
The ZIKV genome is a single-stranded positive-sense RNA (ssRNA) that encodes a polyprotein, subsequently cleaved into three structural proteins (Capsid (C), Premembrane/Membrane (prM/M) and Envelope (E)) and seven non-structural proteins (NS1, NS2A, NS2B, NS3, NS4A, NS4B, and NS5), vital for virus replication and assembly (16, 17). The Envelope (E) protein orchestrates viral assembly, binds to cell receptors, and is essential for the subsequent fusion of the membrane involved in virus entry into the target cell (18). Similar to other flaviviruses, the ZIKV E protein comprises three distinct domains: the central domain (EDI), the fusion peptide-containing dimerization domain (EDII), and the cell surface receptor-binding (EDIII) (19).
Several studies have demonstrated the highly antigenic structure of the E protein, serving as the primary target for host antibody responses, including several neutralizing antibodies (20, 21). While the humoral response is fundamental in protection against ZIKV infection, the involvement of CD4+ and CD8+ T cell responses is essential for complete virus elimination. Despite various animal studies highlighting the importance of CD4+ and CD8+ T cell responses against ZIKV (22–27), limited and conflicting data exist regarding the preferred target regions for human-specific cellular immune response to ZIKV (28, 29). Furthermore, despite ZIKV’s unique impact on fetal health, few studies have investigated the immune response of pregnant women. A recent longitudinal study with 10 non-pregnant women with acute ZIKV infection revealed that CD8+ T cell responses are directed more towards non-structural antigens, while CD4+ T cell responses are more balanced between structural and non-structural antigens (30). Similarly, ZIKV-specific CD4+ memory T cell responses were observed in mothers infected with ZIKV during pregnancy, with no discernible differences in T cell responses between children affected or unaffected by CZS (31).
In this study, we investigated whether ZIKV inactivated particles and different ZIKV-envelope proteins induce cellular-mediated immunity after ZIKV infection in cells from convalescent mothers of newborns with microcephaly in vitro.
Materials and methods
Participants
A dual-center study was conducted at the Hospital Universitário da Universidade Federal de Sergipe and Universidade Federal de São Paulo. Cryopreserved peripheral blood mononuclear cells (PBMC) from 32 ZIKV-infected women, who delivered babies with microcephaly, were used. Participant characteristics are detailed in Table 1. PBMCs were collected post ZIKV-positive diagnosis via ELISA (IgG Euroimunn) approximately 18 months (IQR 13.5-19) after parturition. Additionally, 10 healthy ZIKV-seronegative participants were recruited at Universidade Federal de São Paulo and utilized as controls. All participants provided written informed consent, and the study received approval from the local ethics committee (CAAE: 54835916.2.0000.5546 and CAAE: 80487717.7.0000.5505).
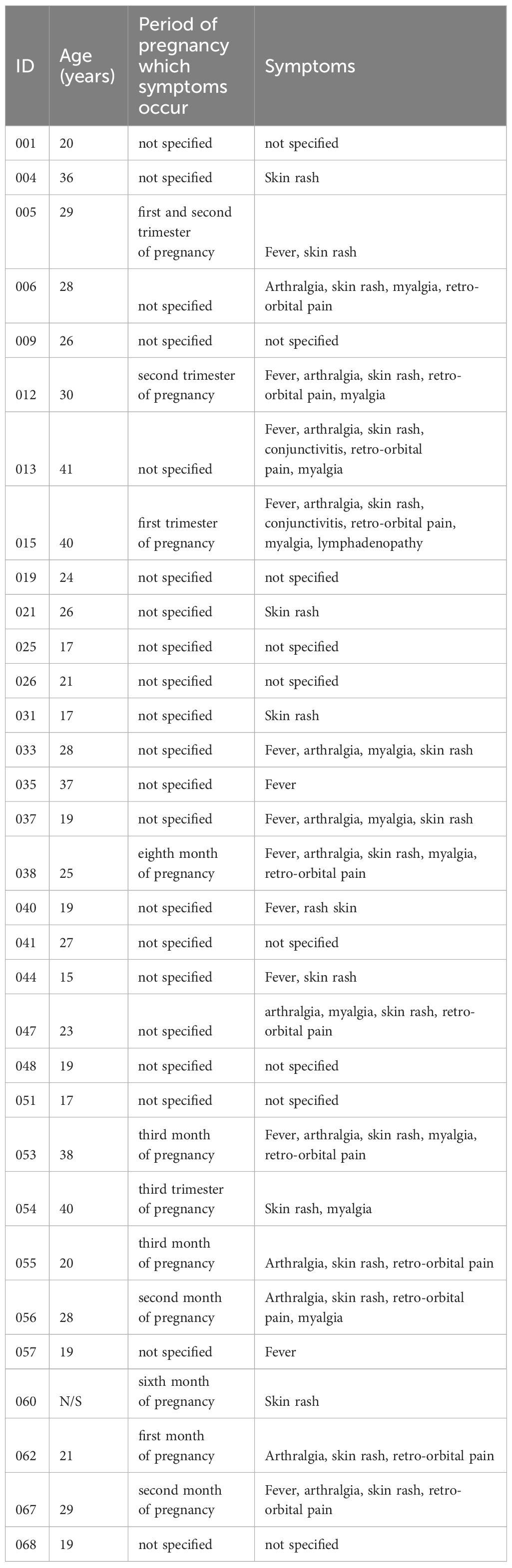
Table 1 Cohort of participants exposed to Zika virus during pregnancy that gave birth to babies affected by microcephaly.
Sample collection
PBMC were isolated from participant blood using Ficoll-Paque (GE Healthcare) density-gradient sedimentation. Subsequently, PBMC were washed twice in Hank’s balanced salt solution (Gibco) and cryopreserved in 90% fetal bovine serum (FBS; Gibco) and 10% dimethyl sulfoxide (DMSO; Sigma). Cryopreserved cells were stored in liquid nitrogen until use.
Inactivated Zika virus particles
ZIKV-Br (GenBank accession number MH882531.1) was provided by Dr. Danielle Bruna Leal de Oliveira (Laboratório de Virologia Clínica e Molecular, University of São Paulo, Brazil). Virus propagation was performed using Aedes albopictus mosquito cells (clone C6/36), as previously described (32). The virus was precipitated with 50% polyethylene glycol (Synth), resuspended in DMEM (Gibco) plus 25 mM HEPES (Gibco), and stored at -80°C until use. For inactivation, we exposed virus preparations to UV light for up to 60 minutes (33).
Expression and purification of recombinant EZIKV protein and its ectodomains EDI/IIZIKV and EDIIIZIKV
The E. coli BL21 (DE3) RIL strain, harboring the plasmids pET21a-E, pET21a-EDI/II and pET21a-EDIII (34, 35), were cultivated in LB medium containing 100 µg/mL ampicillin exactly as described by Lunardelli et al., 2022. After induction with 0.01mM Isopropyl β-D-thiogalactoside (IPTG, Sigma), bacterial pellets were suspended and lysed in the APLAB-10 homogenizer (ARTEPEÇAS, Brazil). The inclusion bodies were solubilized in a urea-containing solution, and the recombinant protein was refolded and purified using nickel affinity chromatography with a HisPur™ Ni-NTA Superflow Agarose column (Thermo Scientific™), as recommended by the manufacturer. Purified EZIKV, EDI/IIZIKV and EDIIIZIKV were assessed by 15% SDS-PAGE gel under reducing conditions.
Dot blot
Approximately 10 μL containing 2x105 PFU of inactivated ZIKV or 10 μL containing 3 μg of BSA (negative control) were added to nitrocellulose membranes (Hybond-C extra nitrocellulose – GE Healthcare). After drying, the membranes were blocked with PBS Tween 20 (PBST) (0.02% v/v), non-fat milk (5% w/v) and BSA (2.5% w/v) or PBS BSA (5% w/v, for human sample), for 2 h at room temperature (rt). Next, the membranes were washed three times with PBST (0,05% v/v) and incubated with monoclonal pan-flavivirus antibody 4G2 (1 μg/mL) or human serum (ZIKV-infected patient or healthy individual, 1:500) for 2 h at rt. After 3 washes with PBST (0.05% v/v) the membranes were incubated with horseradish peroxidase-labeled goat anti-mouse IgG (1:5000; KPL) or alkaline phosphatase AffinePure goat anti-human IgG (1:2000; Jackson ImmunoResearch) for 1 h. After 3 washes with PBST, the reaction was developed with a chemiluminescence detection system ECL (GE Healthcare) or NBT/BCIP (Thermo Fisher Scientific) according to manufacturer’s instructions and analyzed by Alliance 4.7 software (Uvitec; Cambridge).
Western blot
Approximately 1 μg of recombinant EZIKV, EDI/IIZIKV or EDIIIZIKV proteins were subjected to SDS-PAGE gel electrophoresis under reducing conditions and transferred to nitrocellulose membranes (Hybond-C extra nitrocellulose – GE Healthcare). Next, the membrane was blocked with PBS BSA (5% w/v), overnight at 4°C. The membrane was washed three times with PBST (0,05% v/v) and incubated human serum (ZIKV-infected patient, 1:500) for 2 h. After 3 washes with PBST (0.05% v/v) the membrane was incubated with alkaline phosphatase AffinePure goat anti-human IgG (1:2000; Jackson ImmunoResearch) for 1 h. After 3 washes with PBST, the reaction was developed with NBT/BCIP (Thermo Fisher Scientific) according to manufacturer’s instructions and analyzed by Alliance 4.7 software (Uvitec; Cambridge).
Detection of IFN-γ producing cells by ELISPOT
The ELISPOT assay was performed using human IFN-γ ELISPOT Ready-SET-Go! (eBiosciences) according to manufacturer’s instructions. At the time of the assay, PBMC were rapidly thawed in a 37°C water bath, washed and transferred to tubes containing R10 (RPMI supplemented with 10% of fetal bovine serum, 2 mM l-glutamine, 1% v/v vitamin solution, 1 mM sodium pyruvate, 1% v/v non-essential amino acids solution, 40 μg/mL of gentamicin, 2-mercaptoethanol (all from Gibco), 20 μg/mL of Ciprofloxacin (Ciprobacter, Isofarma) and 30 U/mL of recombinant IL-2 (Zodiac) and incubated in a 5% CO2 chamber at 37°C for 20 hours. After this period, cells were counted, checked for viability by Trypan blue dye exclusion (only samples with 80% or more viable cells were used) and resuspended (concentration 2x106 cells/mL; 100 μl/well – 2x105 cells/well) in R10. Then the cells were stimulated with inactivated ZIKV particles (1x105 PFU/well), equimolar amounts of recombinant EZIKV (10 μg/mL) protein and its ectodomains EDI/IIZIKV (7.78 μg/mL) and EDIIIZIKV (2.44 μg/mL), medium alone as negative control or phorbol 12-myristate 13-acetate (PMA) and ionomycin (50 ng/mL and 1 μg/mL, respectively) as positive control. Spots were counted using an AID ELISPOT Reader System (Autoimmun Diagnostika GmbH, Germany). The number of IFN-γ producing cells/106 PBMC was calculated after subtracting the negative control values and the cutoff was 22 spots for inactivated ZIKV and 64; 65; 96 spots per million cells for EZIKV, EDI/IIZIKV and EDIIIZIKV, respectively.
Data analysis
Statistical significance (p-values) was calculated by Kruskal-Wallis followed by Dunn’s post hoc test for multiple comparisons (ZIKV vs EZIKV and ectodomains) or Mann-Whitney test. Statistical analysis and graphical representation were conducted using GraphPad Prism version 9.0 software.
Results
Characteristics of convalescent samples
All 32 mothers delivered babies with microcephaly attributed to ZIKV infection. The majority (21/32 or 65.62%) were unable to specify the exact onset of ZIKV-related symptoms. Seven (21.87%) participants reported symptom initiation during the first trimester of pregnancy, and the remaining (4/32 or 12.5%) during the second or third trimester. Seventy-two percent (72%) of the participants presented one or more symptoms, including fever (18.3%), rash (29.5%), arthralgia (16.9%), conjunctivitis (2.8%), retro-orbital pain (15.4%), myalgia (15.49%) and lymphadenopathy (1.4%) (Figure 1A). Blood samples were collected post ZIKV diagnosis confirmation, with a median time of 18 months (IQR 13.5-19) after delivery. Serum samples were tested for IgG detection for both ZIKV and DENV infection, following the Brazilian Ministry of Health recommendations. All participants tested positive for ZIKV and DENV, with significantly higher reactivity against ZIKV (Figure 1B).
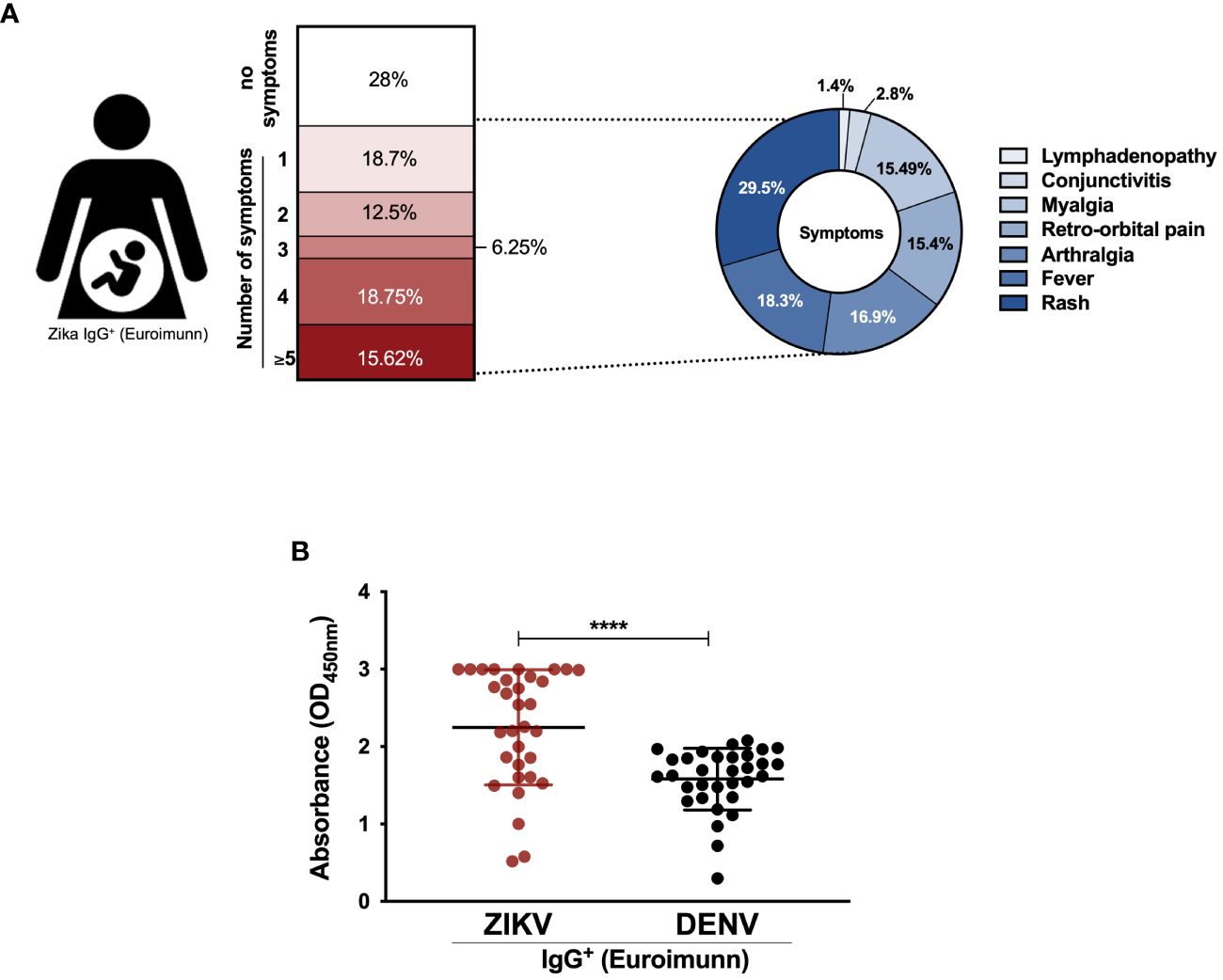
Figure 1 Cohort characteristics. (A) Schematic diagram of symptoms from convalescent mothers of newborns with microcephaly; (B) Antibody response against dengue virus (DENV) and Zika virus (ZIKV) by ELISA (IgG Euroimunn). Statistical significance was tested by Mann-Whitney test. ****p<0.0001.
Antigenicity of the recombinant proteins and inactivated virus
EZIKV (50kDa), EDI/IIZIKV (36kDa) and EDIIIZIKV (11kDa) were purified by affinity chromatography. To assess whether the recombinant proteins retained their antigenicity, a western blot analysis was conducted using serum from a convalescent individual infected with ZIKV. The serum antibodies recognized all EZIKV recombinant proteins indicating that the recombinant proteins retained their antigenic properties (Supplementary Figure 1A). Subsequently, serum from a ZIKV-infected individual and the monoclonal antibody 4G2 (pan flavivirus) recognized the inactivated ZIKV by dot blot analysis (Supplementary Figure 1B), confirming that it retained conformational and antigenic properties post inactivation. In contrast, no recognition of the inactivated ZIKV was observed by the control serum (ZIKV-).
Inactivated ZIKV and different E-proteins induce specific IFNγ-secreting cells from convalescent mothers
To analyze whether PBMC from convalescent mothers could produce specific IFN-γ against inactivated virus or different ZIKV-envelope proteins, an ELISpot assay was performed. Initially, PBMC from all participants produced IFN-γ when stimulated with inactivated ZIKV particles (Figure 2), with most (31/32) displaying a higher number of IFN-γ-producing cells compared to PBMC from healthy controls. Subsequently, the response against the recombinant EZIKV protein and its ectodomains was evaluated. The majority of PBMC from infected participants produced IFN-γ when stimulated with recombinant EZIKV protein (90.6% of positivity, Figure 3A), and its ectodomains EDI/IIZIKV (96.9% of positivity) and EDIIIZIKV (90.6% of positivity) (Figures 3B, C, respectively). Only one participant (#068) presented values below the cutoff (22 spots). In contrast, PBMC from healthy controls exhibited a low number of specific IFN-γ producing cells when stimulated with inactivated ZIKV particles (Figure 2), EZIKV protein, or its ectodomains (Figure 3). Notably, PBMC of participant #068 failed to produce IFN-γ against all stimuli tested, despite the high antibody titers against ZIKV in the serum. A comparison of the number of IFN-γ producing cells among all stimuli (Figure 4A) revealed a difference in the magnitude of the response. In general, when a sample was positive for the EZIKV protein, it also responded to its ectodomains, suggesting that the ectodomains are as antigenic as the entire EZIKV protein. Samples from healthy controls showed numbers of IFN-γ-producing cells below their respective cutoffs (Supplementary Figure 2).
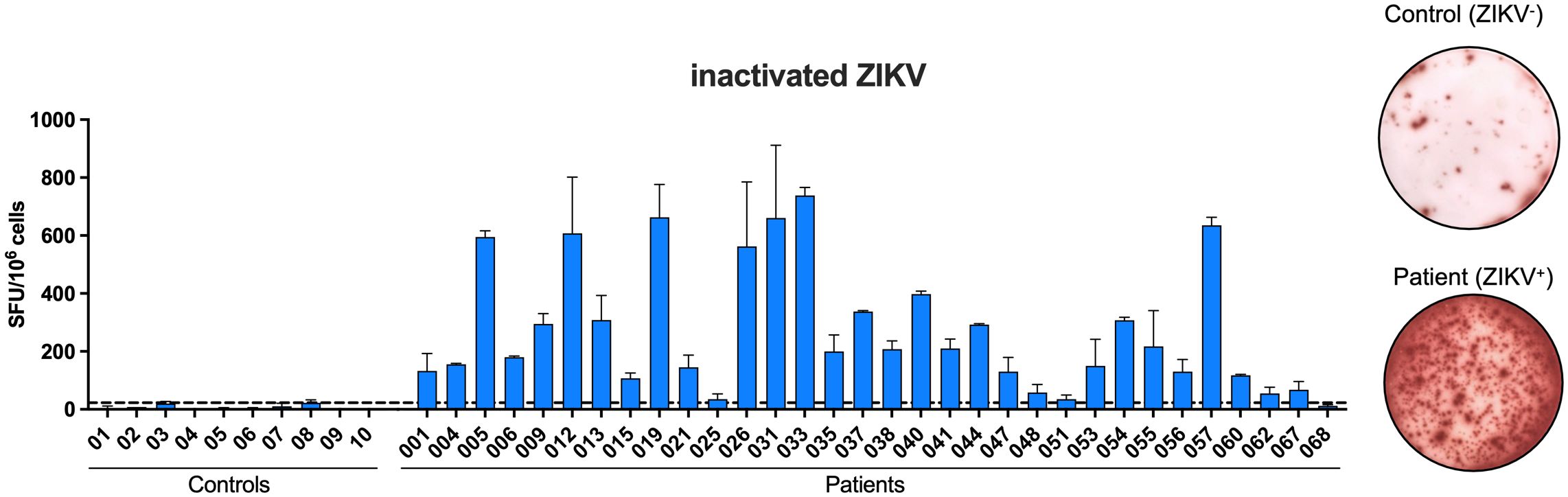
Figure 2 Peripheral blood mononuclear cells (PBMC) of convalescent mothers of newborns with microcephaly produce ZIKV-specific IFN-γ. PBMC were cultured in the presence of inactivated ZIKV (105 PFU) for 24 h to evaluate the number of IFNγ-producing cells by ELISpot assay. SFU, spot-forming units. Cutoff = 22.63 SFU/106 cells.
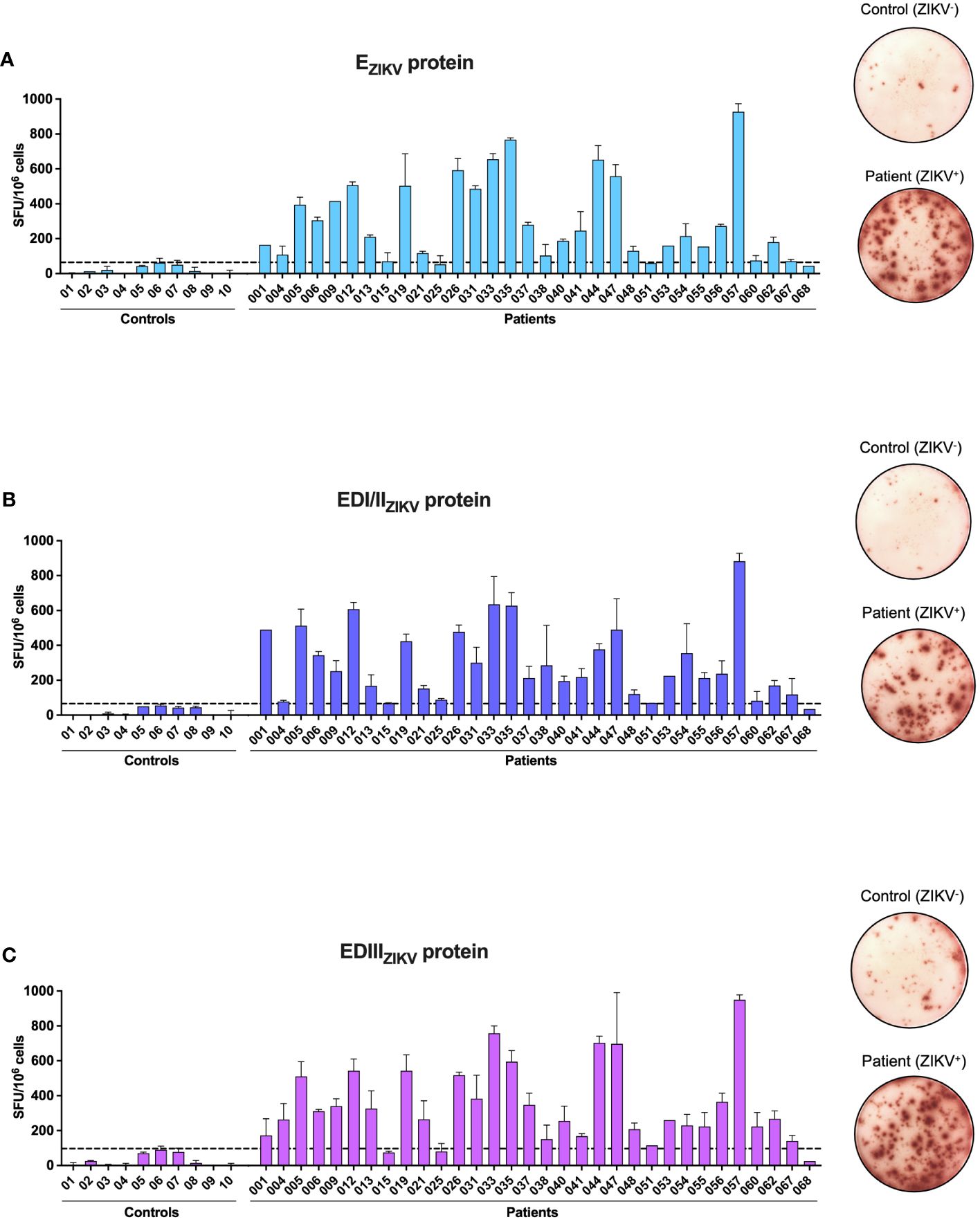
Figure 3 Convalescent PBMC produce IFN-γ against recombinant EZIKV and its ectodomains. PBMC were cultured in the presence of recombinant EZIKV protein (A) or its ectodomains EDI/EDIIZIKV (B) and EDIIIZIKV (C) for 24 h to evaluate the number of IFN-γ-producing cells by ELISpot assay. Cells were cultured with equimolar amounts of recombinant EZIKV protein or its ectodomains. SFU, spot-forming units. Cutoff EZIKV = 64 SFU/106 cells; EDI/EDIIZIKV = 65.86 SFU/106 cells and EDIIIZIKV = 96.51 SFU/106 cells.
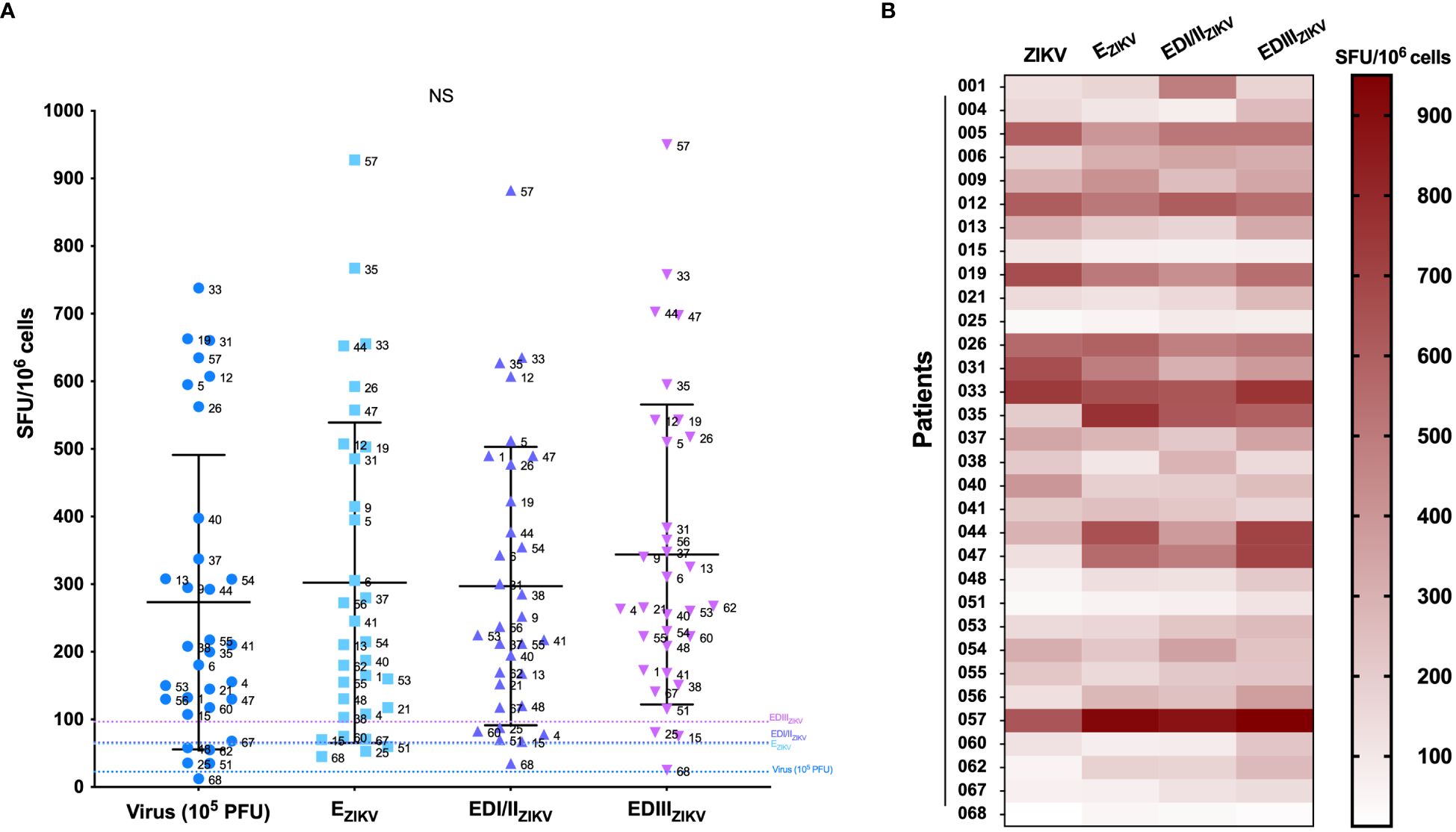
Figure 4 Comparison of the number of IFN-γ producing cells. (A) PBMC were cultured in the presence of inactivated ZIKV (105 PFU), EZIKV protein or its ectodomains EDI/EDIIZIKV and EDIIIZIKV (equimolar amounts) for 24 h to evaluate the number of IFN-γ-producing cells by ELISpot assay. SFU, spot forming units. Cutoff EZIKV = 64 SFU/106 cells; EDI/EDIIZIKV = 65.86 SFU/106 cells and EDIIIZIKV = 96.51 SFU/106 cells. Statistical significance was tested using the Kruskal-Wallis and Dunn’s post hoc tests for multiple comparisons. (B) Heat map showing the number of IFN-γ-producing cells (SFU) for each stimulus. NS, Non-significant.
Figure 4B displays the magnitude of the IFN-γ response for each participant. Overall, a high specific cellular response was observed, with patient #057 presenting the highest magnitude against the three recombinant proteins tested. Additional analysis (Figure 5) revealed a significant correlation of IFN-γ production between ZIKV virus particles and all recombinant proteins, suggesting that the recombinant proteins preserved their structures similarly to the native virus. Furthermore, no significant correlation was observed between the number of symptoms and cellular immunity against the virus nor to recombinant proteins (Supplementary Figure 3).
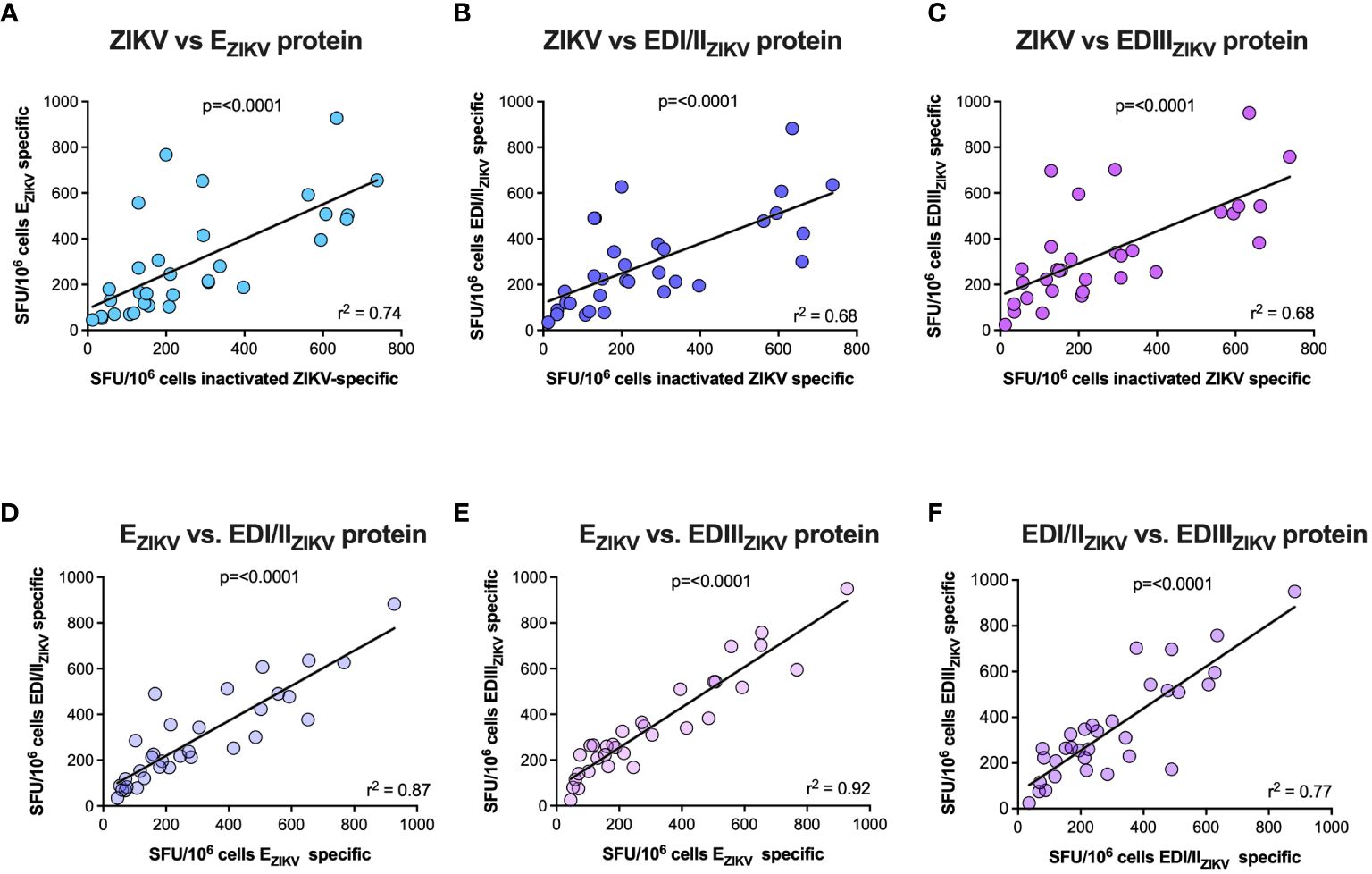
Figure 5 Correlation of IFN-γ response. Spearman correlation was used to evaluate the correlation between the number of IFN-γ-producing cells when stimulated with ZIKV inactivated versus EZIKV (A), ZIKV inactivated versus EDI/IIZIKV (B), ZIKV inactivated versus EDIIIZIKV (C), EZIKV versus EDI/IIZIKV (D), EZIKV versus EDIIIZIKV (E), and EDI/IIZIKV versus EDIIIZIKV (F).
Discussion
The recent global spread of ZIKV infection, together with its association with neurologic morbidity in neonates and adults, underscores the urgent need for a safe and effective vaccine against this virus. Success in developing vaccines for flavivirus, such as the yellow fever and Japanese encephalitis viruses, demonstrated the feasibility of vaccine-induced immunity (36, 37). However, existing vaccines based on live attenuated viruses have limitations, with contraindications for certain populations, such as children (<6months of age), pregnant women, and immunocompromised individuals (38, 39). In this study, we aimed to better understand the specific regions of EZIKV targeted by cellular immunity, with a focus on understanding T cell responses in PBMC from women infected with ZIKV during pregnancy. All mothers in the cohort delivered babies with microcephaly and tested seropositive for both ZIKV and DENV.
The ZIKV envelope protein, essential for virus entry, is the main antigen that triggers the host immune response (18). The EDIIIZIKV domain, in particular, has shown promise as a vaccine candidate, inducing protection against ZIKV challenge in mice (40–42). Furthermore, antibodies generated against EDIII persist for a long period of time (43). Our study revealed that not only the entire envelope protein (EZIKV) but also its structural domains, EDI/IIZIKV and EDIIIZIKV, induced robust adaptive immune responses.
Characterization of the immune response against the ZIKV in animal models has been extensively investigated by various studies (22, 23, 27, 44), including our group, revealing increased recognition of envelope regions by the adaptive immune response, presenting promising implications for the ZIKV diagnosis and vaccine development (35, 45). However, elucidating the immune response against ZIKV in humans poses challenges, primarily attributable to a substantial decline in the reported cases.
A recent study, using PBMC from ZIKV-infected pregnant women, analyzed the immune response 2-3 years post-infection. This study demonstrated sustained CD4+ T cell immunity, but the same was not observed for CD8+ T cells. Intriguingly, the T cell response of the mothers against ZIKV did not exhibit a clear correlation with the clinical outcomes of their children (31). Using PBMC from convalescent ZIKV patients, we detected a specific cellular immune response 1.5 years post-infection, suggesting that natural infection induces the generation of memory T cells capable of producing IFN-γ against the inactivated virus and various EZIKV proteins.
A longitudinal study involving 10 nonpregnant ZIKV-infected women observed the presence of ZIKV antibodies and virus-specific CD4+ and CD8+ T cells (30). Indeed, structural proteins of ZIKV, including E, prM, and C, emerged as major targets for both CD4+ and CD8+ T cell responses (28). Notably, the immune response against ZIKV is detectable during acute infection, with CD4+ T cell responses primarily targeting E, prM, C and NS5 (46). Prior exposure to DENV was suggested to influence the T cell response to subsequent ZIKV infection (28), with cross-reactive T cells potentially expanded via stimulation with ZIKV peptides (47).
Considering the co-circulation of DENV and ZIKV in Brazil (48), it is probable that our cohort was exposed to DENV before ZIKV, supported by the positive serology for DENV. However, the chronological sequence of DENV exposure and ZIKV infection remains uncertain and could potentially influence or alter the observed responses. We cannot exclude the possibility that DENV exposure could have occurred after ZIKV infection and may have skewed or altered the responses in some way. Although several studies evaluated T cell immune responses against ZIKV proteins in DENV immune or non-immune participants (27, 49–51), the precise influence of a previous DENV exposure on ZIKV response modulation remains unclear. A study indicated that acute ZIKV infection proceeded by DENV infection had limited effects on T cells (30, 52). In DENV-naïve/ZIKV-infected patients, CD4+ and CD8+ T cell responses targeted envelope proteins (53). Thus, even without being able to state whether previous exposure to the DENV virus can interfere with the modulation of the response against ZIKV, all mothers presented strong immune response against envelope proteins when compared to control individuals, thus demonstrating that the EZIKV-immune response is long-term and specific.
Finally, interpretation of our findings must consider certain limitations. A longitudinal study, following volunteers during the acute phase rather than just convalescence, would provide a more comprehensive understanding of acquired immunity to ZIKV. Additionally, the sample size was limited, and it would be valuable to compare immune responses from mothers who were infected and did not have babies with microcephaly. Unfortunately, such cases were not available and further studies are required to address these issues.
In summary, our findings highlight the recognition of ZIKV envelope protein regions by memory T cells in mothers 1.5 years post-infection. These results provide important insights into vulnerable regions of viral proteins, contributing to the development of specific treatments (monoclonal antibodies) and an effective and safe vaccine suitable for administration to pregnant women.
Conclusion
Taken together, our data reveal that distinct EZIKV proteins elicited specific IFN-γ production in PBMC derived from women previously infected with ZIKV. This feature holds significant promise for helping the design of safe ZIKV vaccines tailored for pregnant women.
Data availability statement
The raw data supporting the conclusions of this article will be made available by the authors, without undue reservation.
Ethics statement
The studies involving humans were approved by ethics committee from Federal University of Sergipe and Federal University of São Paulo committee (CAAE: 54835916.2.0000.5546 and CAAE: 80487717.7.0000.5505). The studies were conducted in accordance with the local legislation and institutional requirements. The participants provided their written informed consent to participate in this study.
Author contributions
JA: Data curation, Formal analysis, Investigation, Methodology, Validation, Writing – original draft, Writing – review & editing. VL: Data curation, Formal analysis, Investigation, Methodology, Validation, Writing – original draft, Writing – review & editing. SB: Conceptualization, Methodology, Resources, Writing – original draft, Writing – review & editing. VF: Resources, Writing – original draft. RMA: Resources, Writing – original draft. JK: Conceptualization, Funding acquisition, Methodology, Resources, Writing – original draft. RPA: Resources, Writing – original draft. EC-N: Conceptualization, Funding acquisition, Methodology, Resources, Writing – original draft. DR: Conceptualization, Data curation, Formal analysis, Funding acquisition, Investigation, Methodology, Project administration, Resources, Supervision, Validation, Visualization, Writing – original draft, Writing – review & editing.
Funding
The author(s) declare financial support was received for the research, authorship, and/or publication of this article. This research was supported by Fundação de Amparo à Pesquisa do Estado de São Paulo (grant numbers 2017-1741-7; 2021/13004-0), Conselho Nacional de Desenvolvimento Científico e Tecnológico (CNPq/DECIT/CAPES grant number 440400/2016-3; (INCT/CNPq-iii grant 465434/2014). DR, SB, JK and ECN are recipients of CNPq fellowships.
Conflict of interest
The authors declare that the research was conducted in the absence of any commercial or financial relationships that could be construed as a potential conflict of interest.
Publisher’s note
All claims expressed in this article are solely those of the authors and do not necessarily represent those of their affiliated organizations, or those of the publisher, the editors and the reviewers. Any product that may be evaluated in this article, or claim that may be made by its manufacturer, is not guaranteed or endorsed by the publisher.
Supplementary material
The Supplementary Material for this article can be found online at: https://www.frontiersin.org/articles/10.3389/fitd.2024.1369608/full#supplementary-material
References
1. Gatherer D, Kohl A. Zika virus: A previously slow pandemic spreads rapidly through the americas. J Gen Virol. (2016) 97:269–73. doi: 10.1099/jgv.0.000381
2. WHO. Zika Virus, Microcephaly, Guillain-Barré Syndrome. Report S, editor. World Health Organization (2017).
3. Brooks JT, Friedman A, Kachur RE, LaFlam M, Peters PJ, Jamieson DJ. Update: interim guidance for prevention of sexual transmission of Zika virus - United States, july 2016. MMWR Morb Mortal Wkly Rep. (2016) 65:745–7. doi: 10.15585/mmwr.mm6529e2
4. Collins MH, Waggoner JJ. Detecting vertical Zika transmission: emerging diagnostic approaches for an emerged flavivirus. ACS Infect Dis. (2019) 5:1055–69. doi: 10.1021/acsinfecdis.9b00003
5. Haddow AD, Schuh AJ, Yasuda CY, Kasper MR, Heang V, Huy R, et al. Genetic characterization of Zika virus strains: geographic expansion of the asian lineage. PloS Negl Trop Dis. (2012) 6:e1477. doi: 10.1371/journal.pntd.0001477
6. Cao-Lormeau VM, Blake A, Mons S, Lastere S, Roche C, Vanhomwegen J, et al. Guillain-barre syndrome outbreak associated with Zika virus infection in French Polynesia: A case-control study. Lancet. (2016) 387:1531–9. doi: 10.1016/S0140-6736(16)00562-6
7. Styczynski AR, Malta J, Krow-Lucal ER, Percio J, Nobrega ME, Vargas A, et al. Increased rates of guillain-barre syndrome associated with Zika virus outbreak in the salvador metropolitan area, Brazil. PloS Negl Trop Dis. (2017) 11:e0005869. doi: 10.1371/journal.pntd.0005869
8. Govero J, Esakky P, Scheaffer SM, Fernandez E, Drury A, Platt DJ, et al. Zika virus infection damages the testes in mice. Nature. (2016) 540:438–42. doi: 10.1038/nature20556
9. Tang WW, Young MP, Mamidi A, Regla-Nava JA, Kim K, Shresta S. A mouse model of Zika virus sexual transmission and vaginal viral replication. Cell Rep. (2016) 17:3091–8. doi: 10.1016/j.celrep.2016.11.070
10. Brasil P, Pereira JP Jr., Moreira ME, Ribeiro Nogueira RM, Damasceno L, Wakimoto M, et al. Zika virus infection in pregnant women in rio de janeiro. N Engl J Med. (2016) 375:2321–34. doi: 10.1056/NEJMoa1602412
11. Franca GV, Schuler-Faccini L, Oliveira WK, Henriques CM, Carmo EH, Pedi VD, et al. Congenital Zika virus syndrome in Brazil: A case series of the first 1501 livebirths with complete investigation. Lancet. (2016) 388:891–7. doi: 10.1016/S0140-6736(16)30902-3
12. Kleber de Oliveira W, Cortez-Escalante J, De Oliveira WT, do Carmo GM, Henriques CM, Coelho GE, et al. Increase in reported prevalence of microcephaly in infants born to women living in areas with confirmed Zika virus transmission during the first trimester of pregnancy - Brazil, 2015. MMWR Morb Mortal Wkly Rep. (2016) 65:242–7. doi: 10.15585/mmwr.mm6509e2
13. Einspieler C, Utsch F, Brasil P, Panvequio Aizawa CY, Peyton C, Hydee Hasue R, et al. Association of infants exposed to prenatal Zika virus infection with their clinical, neurologic, and developmental status evaluated via the general movement assessment tool. JAMA Netw Open. (2019) 2:e187235. doi: 10.1001/jamanetworkopen.2018.7235
14. Mulkey SB, Arroyave-Wessel M, Peyton C, Bulas DI, Fourzali Y, Jiang J, et al. Neurodevelopmental abnormalities in children with in utero Zika virus exposure without congenital Zika syndrome. JAMA Pediatr. (2020) 174:269–76. doi: 10.1001/jamapediatrics.2019.5204
15. Lunardelli VAS, Apostolico JS, Fernandes ER, Santoro Rosa D. Zika virus-an update on the current efforts for vaccine development. Hum Vaccin Immunother. (2021) 17:904–8. doi: 10.1080/21645515.2020.1796428
16. Sirohi D, Chen Z, Sun L, Klose T, Pierson TC, Rossmann MG, et al. The 3.8 a resolution cryo-em structure of Zika virus. Science. (2016) 352:467–70. doi: 10.1126/science.aaf5316
17. Faye O, Freire CC, Iamarino A, Faye O, de Oliveira JV, Diallo M, et al. Molecular evolution of Zika virus during its emergence in the 20(Th) century. PloS Negl Trop Dis. (2014) 8:e2636. doi: 10.1371/journal.pntd.0002636
18. Dai L, Song J, Lu X, Deng YQ, Musyoki AM, Cheng H, et al. Structures of the Zika virus envelope protein and its complex with a flavivirus broadly protective antibody. Cell Host Microbe. (2016) 19:696–704. doi: 10.1016/j.chom.2016.04.013
19. Whitehead SS, Blaney JE, Durbin AP, Murphy BR. Prospects for a dengue virus vaccine. Nat Rev Microbiol. (2007) 5:518–28. doi: 10.1038/nrmicro1690
20. Stettler K, Beltramello M, Espinosa DA, Graham V, Cassotta A, Bianchi S, et al. Specificity, cross-reactivity, and function of antibodies elicited by Zika virus infection. Science. (2016) 353:823–6. doi: 10.1126/science.aaf8505
21. Sapparapu G, Fernandez E, Kose N, Bin C, Fox JM, Bombardi RG, et al. Neutralizing human antibodies prevent Zika virus replication and fetal disease in mice. Nature. (2016) 540:443–7. doi: 10.1038/nature20564
22. Elong Ngono A, Vizcarra EA, Tang WW, Sheets N, Joo Y, Kim K, et al. Mapping and role of the Cd8+ T cell response during primary Zika virus infection in mice. Cell Host Microbe. (2017) 21:35–46. doi: 10.1016/j.chom.2016.12.010
23. Lucas CGO, Kitoko JZ, Ferreira FM, Suzart VG, Papa MP, Coelho SVA, et al. Critical role of Cd4(+) T cells and ifngamma signaling in antibody-mediated resistance to Zika virus infection. Nat Commun. (2018) 9:3136. doi: 10.1038/s41467-018-05519-4
24. Hassert M, Wolf KJ, Schwetye KE, DiPaolo RJ, Brien JD, Pinto AK. Cd4+T cells mediate protection against Zika associated severe disease in a mouse model of infection. PloS Pathog. (2018) 14:e1007237. doi: 10.1371/journal.ppat.1007237
25. Scott JM, Lebratti TJ, Richner JM, Jiang X, Fernandez E, Zhao H, et al. Cellular and humoral immunity protect against vaginal Zika virus infection in mice. J Virol. (2018) 92(7):e00038-18. doi: 10.1128/JVI.00038-18
26. Elong Ngono A, Young MP, Bunz M, Xu Z, Hattakam S, Vizcarra E, et al. Cd4+ T cells promote humoral immunity and viral control during Zika virus infection. PloS Pathog. (2019) 15:e1007474. doi: 10.1371/journal.ppat.1007474
27. Regla-Nava JA, Elong Ngono A, Viramontes KM, Huynh AT, Wang YT, Nguyen AT, et al. Cross-reactive dengue virus-specific Cd8(+) T cells protect against Zika virus during pregnancy. Nat Commun. (2018) 9:3042. doi: 10.1038/s41467-018-05458-0
28. Grifoni A, Pham J, Sidney J, O’Rourke PH, Paul S, Peters B, et al. Prior dengue virus exposure shapes T cell immunity to Zika virus in humans. J Virol. (2017) 91(24):e01469-17. doi: 10.1128/JVI.01469-17
29. Delgado FG, Torres KI, Castellanos JE, Romero-Sanchez C, Simon-Loriere E, Sakuntabhai A, et al. Improved Immune Responses against Zika Virus after Sequential Dengue and Zika Virus Infection in Humans. Viruses. (2018) 10(9):480. doi: 10.3390/v10090480
30. Tonnerre P, Melgaco JG, Torres-Cornejo A, Pinto MA, Yue C, Blumel J, et al. Evolution of the innate and adaptive immune response in women with acute Zika virus infection. Nat Microbiol. (2020) 5:76–83. doi: 10.1038/s41564-019-0618-z
31. Badolato-Correa J, Carvalho FR, Paiva IA, Familiar-Macedo D, Dias HG, Pauvolid-Correa A, et al. Differential longevity of memory Cd4 and Cd8 T cells in a cohort of the mothers with a history of zikv infection and their children. Front Immunol. (2021) 12:610456. doi: 10.3389/fimmu.2021.610456
32. Medina F, Medina JF, Colon C, Vergne E, Santiago GA, Munoz-Jordan JL. Dengue virus: isolation, propagation, quantification, and storage. Curr Protoc Microbiol. (2012). doi: 10.1002/9780471729259.mc15d02s27
33. Muller JA, Harms M, Schubert A, Jansen S, Michel D, Mertens T, et al. Inactivation and environmental stability of Zika virus. Emerg Infect Dis. (2016) 22:1685–7. doi: 10.3201/eid2209.160664
34. Amaral MP, Apostolico JS, Tomita N, Coirada FC, Lunardelli VAS, Fernandes ER, et al. Homologous prime-boost with Zika virus envelope protein and poly (I:C) induces robust specific humoral and cellular immune responses. Vaccine. (2020) 38:3653–64. doi: 10.1016/j.vaccine.2020.03.037
35. Lunardelli VAS, de Souza Apostolico J, Souza HFS, Coirada FC, Martinho JA, Astray RM, et al. Zikv-envelope proteins induce specific humoral and cellular immunity in distinct mice strains. Sci Rep. (2022) 12:15733. doi: 10.1038/s41598-022-20183-x
36. Garske T, Van Kerkhove MD, Yactayo S, Ronveaux O, Lewis RF, Staples JE, et al. Yellow fever in Africa: estimating the burden of disease and impact of mass vaccination from outbreak and serological data. PloS Med. (2014) 11:e1001638. doi: 10.1371/journal.pmed.1001638
37. Hu XT, Li QF, Ma C, Zhao ZX, He LF, Tang TT, et al. Reduction patterns of Japanese encephalitis incidence following vaccine introduction into long-term expanded program on immunization in yunnan province, China. Infect Dis Poverty. (2019) 8:102. doi: 10.1186/s40249-019-0608-7
38. WHO. Detection and Investigation of Serious Adverse Events Following Yellow Fever Vaccination. Available online at: https://appswhoint/iris/bitstream/handle/10665/70251/WHO_HSE_GAR_ERI_20102_engpdf;jsessionid=00CE1944BF3BB748F23372FB80C8AA48?sequence=1 (Accessed 1 July 2021).
39. Bae HG, Domingo C, Tenorio A, de Ory F, Munoz J, Weber P, et al. Immune response during adverse events after 17d-derived yellow fever vaccination in europe. J Infect Dis. (2008) 197:1577–84. doi: 10.1086/587844
40. Yang M, Dent M, Lai H, Sun H, Chen Q. Immunization of Zika virus envelope protein domain iii induces specific and neutralizing immune responses against Zika virus. Vaccine. (2017) 35:4287–94. doi: 10.1016/j.vaccine.2017.04.052
41. Yang M, Lai H, Sun H, Chen Q. Virus-like particles that display Zika virus envelope protein domain iii induce potent neutralizing immune responses in mice. Sci Rep. (2017) 7:7679. doi: 10.1038/s41598-017-08247-9
42. Tai W, He L, Wang Y, Sun S, Zhao G, Luo C, et al. Critical neutralizing fragment of Zika virus ediii elicits cross-neutralization and protection against divergent Zika viruses. Emerg Microbes Infect. (2018) 7:7. doi: 10.1038/s41426-017-0007-8
43. Yu L, Wang R, Gao F, Li M, Liu J, Wang J, et al. Delineating antibody recognition against Zika virus during natural infection. JCI Insight. (2017) 2(12):e93042. doi: 10.1172/jci.insight.93042
44. Lima NS, Rolland M, Modjarrad K, Trautmann L. T cell immunity and Zika virus vaccine development. Trends Immunol. (2017) 38:594–605. doi: 10.1016/j.it.2017.05.004
45. Lunardelli VAS, Almeida BDS, Apostolico JS, Rezende T, Yamamoto MM, Pereira SS, et al. Diagnostic and vaccine potential of Zika virus envelope protein (E) derivates produced in bacterial and insect cells. Front Immunol. (2023) 14:1071041. doi: 10.3389/fimmu.2023.1071041
46. Lai L, Rouphael N, Xu Y, Natrajan MS, Beck A, Hart M, et al. Innate, T-, and B-cell responses in acute human Zika patients. Clin Infect Dis. (2018) 66:1–10. doi: 10.1093/cid/cix732
47. Schouest B, Grifoni A, Pham J, Mateus J, Sydney J, Brien JD, et al. Pre-existing T cell memory against Zika virus. J Virol. (2021) 95(12):e00132-21. doi: 10.1128/JVI.00132-21
48. Reynolds CJ, Suleyman OM, Ortega-Prieto AM, Skelton JK, Bonnesoeur P, Blohm A, et al. T cell immunity to Zika virus targets immunodominant epitopes that show cross-reactivity with other flaviviruses. Sci Rep. (2018) 8:672. doi: 10.1038/s41598-017-18781-1
49. Reynolds CJ, Watber P, Santos CNO, Ribeiro DR, Alves JC, Fonseca ABL, et al. Strong cd4 T cell responses to Zika virus antigens in a cohort of dengue virus immune mothers of congenital Zika virus syndrome infants. Front Immunol. (2020) 11:185. doi: 10.3389/fimmu.2020.00185
50. Wen J, Elong Ngono A, Regla-Nava JA, Kim K, Gorman MJ, Diamond MS, et al. Dengue virus-reactive Cd8(+) T cells mediate cross-protection against subsequent Zika virus challenge. Nat Commun. (2017) 8:1459. doi: 10.1038/s41467-017-01669-z
51. Wen J, Tang WW, Sheets N, Ellison J, Sette A, Kim K, et al. Identification of Zika virus epitopes reveals immunodominant and protective roles for dengue virus cross-reactive Cd8(+) T cells. Nat Microbiol. (2017) 2:17036. doi: 10.1038/nmicrobiol.2017.36
52. Grifoni A, Costa-Ramos P, Pham J, Tian Y, Rosales SL, Seumois G, et al. Cutting edge: transcriptional profiling reveals multifunctional and cytotoxic antiviral responses of Zika virus-specific Cd8(+) T cells. J Immunol. (2018) 201:3487–91. doi: 10.4049/jimmunol.1801090
Keywords: Zika virus, cellular immune response, T cells, pregnancy, microcephaly
Citation: Apostolico JdS, Lunardelli VAS, Boscardin SB, Botosso VF, Astray RM, Kalil J, de Almeida RP, Cunha-Neto E and Rosa DS (2024) Durable cellular immune response against inactivated ZIKV and envelope proteins in ZIKV-infected women during pregnancy. Front. Trop. Dis 5:1369608. doi: 10.3389/fitd.2024.1369608
Received: 12 January 2024; Accepted: 01 April 2024;
Published: 26 April 2024.
Edited by:
Antonio Carlos De Albuquerque Bandeira, Faculdade de Tecnologia e Ciências, BrazilReviewed by:
Gubio Campos, Federal University of Bahia (UFBA), BrazilBeatriz Arns, Moinhos de Vento Hospital, Brazil
Copyright © 2024 Apostolico, Lunardelli, Boscardin, Botosso, Astray, Kalil, de Almeida, Cunha-Neto and Rosa. This is an open-access article distributed under the terms of the Creative Commons Attribution License (CC BY). The use, distribution or reproduction in other forums is permitted, provided the original author(s) and the copyright owner(s) are credited and that the original publication in this journal is cited, in accordance with accepted academic practice. No use, distribution or reproduction is permitted which does not comply with these terms.
*Correspondence: Daniela Santoro Rosa, dsrosa@unifesp.br
†These authors have contributed equally to this work