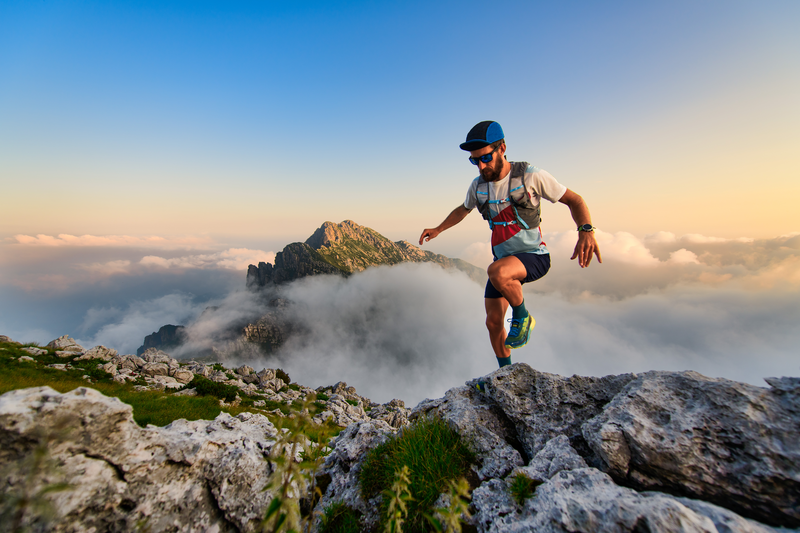
94% of researchers rate our articles as excellent or good
Learn more about the work of our research integrity team to safeguard the quality of each article we publish.
Find out more
MINI REVIEW article
Front. Trop. Dis. , 28 March 2024
Sec. Vector Biology
Volume 5 - 2024 | https://doi.org/10.3389/fitd.2024.1369007
This article is part of the Research Topic Latest Advances in the Biological Control of Vectors of Human Tropical Diseases View all 6 articles
The Phlebotomine sandflies (Diptera, Psychodidae) are hematophagous insects of immense medical and veterinary importance. Since World War II, the intensive use of chemicals to suppress and control sandfly populations resulted in development of insecticide resistance and resurgence among the sandfly populations worldwide. The use of chemicals also negatively impacted diverse non-target organisms, overall agroecosystem, crop productivity, human health etc. Due to the multiple adverse effects of the chemical compounds, more eco-friendly approaches have been evaluated. The application of entomopathogenic organisms such as nematodes, protists or mites as biocontrol agents has been vastly explored and applied in the field of forestry and agriculture. In comparison, only a little attention was given to blood-sucking insects such as sandflies. This review summarizes the findings related to the detection and use of entomopathogenic protists, nematodes, and mites in the field of biological control of sandflies. It highlights the potential of these organisms to be further explored and used for biocontrol of immature and adult stages of sandflies.
The Phlebotomine sandflies (Diptera, Psychodidae) are hematophagous insects of immense medical and veterinary importance. There are approximately 1000 sandfly species, among which 98 are confirmed and/or suspected vectors of pathogens infectious to the humans and animals (1). Sandflies can transmit bacteria from the genus Bartonella (2), various viruses (Rhabdoviridae, Peribunyaviridae, Flaviviridae, Reoviridae, and Phenuiviridae) (3), and most importantly protozoan parasites from genus Leishmania - causative agent of leishmaniasis. Leishmaniasis can manifest with plethora of symptoms, being endemic in more than 100 countries worldwide, with more than 1 million reported cases and 70.000 deaths annually (4).
Due to the complexity of epidemiological and biological conditions associated with leishmaniasis transmission (5), the effective leishmaniasis control requires application of well-planned control strategies addressing multiple aspects such as effective diagnosis and treatment of the disease, study of vectors and reservoirs, together with the development of new approaches for vector management (6).
Combating leishmaniasis proved to be extremely challenging especially in the field of sandfly vector management, which among others requires the continuous vector surveillance and implementation of different control strategies that are mostly based on insecticide use (7). Chemical strategies for the control of sandflies have gained massive popularity due to their rapid and potent effect and have been the main go to approach since the World War II (8). The emergence of sandfly insecticide resistance, as well as the immense accumulating negative effect of these chemicals on the environment and non-targeted organisms, significantly crippled the use of chemicals as the ultimate solution for sandfly and leishmaniasis control (8). These limitations strongly point toward the urgent need for more effective and novel methods that will have minimal environmental effects.
Among the environmentally friendly insect vector control strategies, biocontrol approaches have received increasing attention and popularity over the past 20 years. Biocontrol explores the potential of (micro)organisms to control the insect vector populations, and can be based on the predation, parasitism or pathobiological relationship between the biological control organism and the targeted insect hosts. As a result, numerous organisms were incriminated, and new strategies including the use of entomopathogens have emerged.
Entomopathogens are organisms that cause disease and/or death of insects (9). Several entomopathogens have been described in sandflies, including viruses, bacteria, protozoa, fungi, nematodes, and mites. Some of them are capable of killing the insect host even within a few hours, while others impact their longevity, fecundity and/or reproduction potential. The studies of entomopathogens as biological weapons for the control of sandfly population commenced early in the 19th century. These studies were very attractive, and a large number of publications have been produced targeting the identification of different pathogens. Unfortunately, under the pressure of commercial insecticides development, and their great success in controlling the sandfly populations (8), the exploration and further exploitation of entomopathogens as biological agents in sandfly control was neglected. The newly gained interest in the use of biological agents for the control of sandflies emerged after the encouraging results from the experiments involving Wolbachia in mosquitoes, paratransgenic approach for the control of Trypanosoma cruzi in triatomine bugs and development of sterile tsetse flies (10–12).
Compared to the other insects with medical and veterinary importance (or other insect pests), where investigations of biological control agents/approaches have been tested in practice and showed very promising results (11–13), the field of biological control of sandflies is still well behind. The majority of studies related to the biological control of sandflies and diseases they transmit were focused on the use of bacteria and fungi. The bacteria have been vastly explored in their role as pathogens (14), larvicides (15), for the development of paratransgenic approach (16) etc., while fungi were mainly evaluated as entomopathogens (17). In comparison to the massive amount of data generated in relation to the bacteria and fungi, other organisms such as protists, nematodes and mites have been neglected. This review focuses on summarizing the impact, importance and potential of protists, nematodes, and mites as agents for the biological control of sandflies, and ultimately leishmaniasis.
In total five databases including Science Direct, Scopus, PubMed, Google Scholar and Web of Science were screened for the presence of publications related to the protists, nematodes, and mites in association to different sandfly species. An intensive literature search was carried out using general terms (in English language) such as “mite”, “nematode”, and “protist” in combination with terms “sandfly”, “sand fly”, “Phlebotomine” or general sandfly genus/species names. As the initial search showed predominance of very old publications dating back to 1930-ies, that were written in native language of the corresponding author and available online only in the form of title record; the search was refined to include publications available at least in an abstract form regardless of the written language. After reading all collected publications, final filtering was performed and all studies that showed duplicate, insufficient, or not relevant data were removed.
Nematodes are microscopic, non-segmented, elongated, colorless roundworms without appendages. Entomopathogenic nematode parasitize insects and can cause disease within an insect resulting in its death (18). Entomopathogenic nematodes described so far belong to more than 40 families, among which Steinernematidae and Heterorhabditidae have received the most attention. Members of these two families have many attributes of effective biological control agents, and have been utilized as classical, conservational, and augmentative biological control agents (19).
Entomopathogenic nematodes are highly diverse, complex, and specialized, and they work in tandem with their symbiont bacteria in causing rapid mortality within the insect host population. The life cycle of most nematodes includes an egg, four juvenile stages, and an adult stage. The third juvenile stage is the infective, or dauer stage, and is the only free-living stage. Infective juveniles locate, attack, and infect an insect host. Infective juvenile nematodes penetrate the insect’s body cavity through natural body openings, or by breaking hosts cuticle with the dorsal tooth/hook. Once inside the hosts body, the infective juveniles release bacteria that live symbiotically within their gut. The nematode-bacterium relationship is highly specific, and within Steinernematids only Xenorhabdus sp. bacteria co-exist, while within Heterorhabditids only Photorhabdus bacteria co-exist (20). Once released into the host, the bacteria multiply quickly and cause the host to die generally within 24 to 48 hours. Entomopathogenic nematodes feed on bacteria that they released, as well as on the insect host tissue. After nematodes mature, they produce a high number of new juveniles that may undergo several life cycles within a single insect host. For the representatives of Steinernematids and Heterorhabditids it takes approximately 37 days to complete a life cycle inside an insect from egg to egg. Emergence of dauers from the host requires about 6 to 11 days for Seinernematids and 12 to 14 days for Heterorhabditids (18). When the insect host has been completely consumed, the infective juveniles (containing symbiotic bacteria), emerge from the empty shell of the host and begin the search for a new host.
Entomopathogenic nematodes are effective biocontrol organisms that have potential to infect and kill soil-dwelling larvae as well as above-ground adult forms of sandflies. The detrimental effect of nematodes such as Tricephalobus steineri in combination with Procephalobus sp. has been confirmed under laboratory conditions on Phlebotomus papatasi (21). This nematode declined colony productivity within 6 months from over 10.000 flies per week to less than 100 per week. Nematoda Anandranema phlebotophaga is a single auto generation nematode that is causing infertility in Lutzomyia longipalpis (22). Nematodes belonging to the genus Anandranema were also discovered in Lutzomyia fischeri but their effect on the insect was not evaluated (23). Didilia ooglypta was found to be a parasitic nematode of P. papatasi and P. sergenti (24). Further studies revealed that this nematode prolonged or stopped the development of larvae, reduced the longevity of adult flies, hindered the rotation of male external genitalia preventing them to mate, while adult females refused opportunities to engorge on blood (25). The nematode from the family Steinernematidae negatively affected the survival and blood feeding of Lu. longipalpis females (26). On the other hand, the presence of Apbelenchoides bicaudatus and Coenorhabditis sp. nematodes that were recorded in the colony of Lu. youngi, appeared to maintain the rearing medium in good condition (27). According to the authors these nematodes formed a useful source of protein in the food, particularly for the first instar larvae (27). The effect of Steinernema feltiae, St. abbasi, St. carpocapsae DD136, St. scapterisci, Steinernema sp., Heterorhabditis bacteriophora HP88, Heterorhabditis sp., and H. indica was evaluated under laboratory conditions on two sandfly species – P. papatasi and P. sergenti (28). It is observed that Heterorhabditis species were generally more virulent than Steinernema species, and that P. sergenti was relatively more susceptible than P. papatasi (28). Further on, the effect of Steinernema carpocapsae DD136, Steinernema sp. SII., St. carpocapsae, St. abbasi Ab, and H. bacteriophora HP88 were evaluated on the P. papatasi larvae, and demonstrated 42-94% mortality rate depending on the nematode (29). Field studies in Ethiopia showed that nematode found only in Phlebotomus orientalis females (0.8%) caused insects reproductive organ destruction (30). In addition to above mentioned nematodes, presence of Mastophorus muris was recorded in P. ariasi, P. perniciosus and Sergentomyia minuta larvae (31–33); Rictularia proni was found in P. ariasi larvae (34); Entomopathogenic nematode of the genus Howardula were detected in wild collected Phlebotomus argentipes and Sergentomyia monticola (35); Nematode belonging to Steinernematidae family were observed in the hemocoel of one specimen of P. tobbi (36); Natural population of Pintomyia fischeri was found positive for Tylenchid nematodes (37); Not fully identified nematodes were detected in Lutzomyia cayennensis braci and Lu. cruciata (38, 39). The list of nematodes found in sandflies is summarized in Table 1.
Table 1 Entomopathogenic nematodes, protists and mites that were found in association with sandflies.
Many factors can influence the successful use of nematodes as biological agents. Matching the biology and ecology of the nematode with the sandfly host is a crucial step towards successful application. It is proven that nematodes are most efficient in habitats that provide protection from environmental extremes, especially in soil (72). This characteristic makes them a good candidate for the application in control of immature stages of sandflies that dwell in soil, burrows, and different protected habitats. Nowadays, nematodes are cultured on a large scale in laboratories, and are available from many commercial suppliers, which is an additional incentive for their further exploration.
Species from Microspora (Microsporidians), Sarcomastigophora (Flagellates and Rhizopods) and Apicomplexa (Gregarines and Coccidians), are referred as entomopathogenic protists (73). These protists can cause disease in insects and are of interest as agents of biological control. In the field of sandfly biological control, gregarines are the only explored entomopathogenic protists. Gregarines are monoxenous parasites and can be found in the body cavity or digestive tract of several invertebrate hosts including sandflies. The life cycle of gregarines, their taxonomy and host specificity are complex and still poorly understood.
The infective stages of gregarines are oocysts containing sporozoites. After oocysts dehiscence, sporozoites are released and they attach to the host epithelium or develop intracellularly into trophozoites. Detachment of the trophozoites from the host cell is followed by the sexual phase of the life cycle resulting in the formation of a gametocyst. Gametocyst containing gamonts undergoes multiple nuclear divisions leading to the production of gametes. Further on during sporogony, zygotes differentiate by mitoses into oocysts with sporozoites.
Ascogregarina chagasi later named Psychodiella chagasi is the most studied sandfly gregarine. In sandflies, the first instar larvae are infected by swallowing oocysts. The sporozoites are released from oocyst into the larval midgut where they attach to the epithelial cells and develop into trophozoites (74). In larvae, the gamonts are found mostly in the ectoperitrophic space of the intestine and in the intestinal lumen, where sexual development and oocysts production occurs. The gregarines can complete the life cycle in the larvae, and larval feces containing oocysts serve as a source of horizontal transmission. In adults, the gregarines form syzygies and gametocysts with oocysts in the body cavity. The gametocysts attach to the accessory glands of females, and the oocysts are injected into their lumen (49). This is a unique mechanism of vertical transmission supporting the hypothesis about sandflies and gregarines co-evolution (42). During oviposition, oocysts are attached to the sandfly egg chorion, which serves as a source of infection for newly hatched first instar larvae. The life cycle of most sandfly gregarines is similar to the above-described general life cycle of As. chagasi, with the exception of Ascogregarina mackiei (Psychodiella mackiei). The development of sporozoites and trophozoites of As. mackei in sandfly larvae is intracellular, and this is the only sandfly gregarine with intracellular development (40).
The host specificity studies of gregarines were conducted in detail in mosquitos, showing rather contradictory results (75, 76). Unlike extensive studies of mosquitoes, data about sandfly gregarine specificity is rather limited. Within the study of Wu and Tesh (1989) (43) seven sandfly species including P. papatasi, P. argentipes, P. perniciosus, Lu, serrana, Lu. abonnenci, Lu. columbiana and Lu. longipalpis were infected with oocysts of Ascogregarina chagasi (now Psychodiella chagasi). It is demonstrated that trophozoites gregarine life stages can be found in P. papatasi, Lu. columbiana, Lu. serrana and two strains of Lu. longipalpis (Brazilian and Columbian) (43). It is also shown that As. chagasi were able to complete their life cycle only in the Brazilian strain of Lu. longipalpis, while the infection rate and parasite density were higher in the Colombian strain. Infection with As. chagasi significantly reduced adult longevity of the Brazilian strain of Lu. longipalpis, but it had little effect on fecundity (43). Since As. chagasi demonstrated the variability in susceptibility toward different strains of Lu. longipalpis, it is speculated that these gregarines have strict host specificity (43).
In total, gregarines found in sandflies include 5 species from genus Psychodiella – Ps. chagasi, Ps. mackiei, Ps. saraviae, Ps. sergenti and Ps. tobbi (Table 1). Psychodiella chagasi was firstly described in the hemocoel and accessory glands of the Lu. longipalpis (44), and it was later found in Lutzomyia sallesi, Lu. flaviscutellata, Lu. townsendi, Lu. sordelli, Lu. cruzi and Lu. evandroi (42, 43, 45, 46). Psychodiella saraviae was described from blood-fed females of Lutzomyia lichyi with gametocysts attached to accessory glands and oocysts in the lumen (42) and was further detected in Lutzomyia schreiberi (45). Psychodiella mackiei was described from Phlebotomus argentipes (40) and later was found in P. papatasi (41). Psychodiella sergenti and Ps. tobbi are found in laboratory reared sandflies (Phlebotomus sergenti and P. tobbi, respectively) (47). Psychodiella sergenti gamonts are found in the hemocoel of Phlebotomus sergenti adults, and the sexual development of this gregarine occurs exclusively in blood-fed females (48). Psychodiella sergenti have a negative impact on its host, as gregarine infection significantly decreases the survival of various sandfly stages, but it does not impact the fecundity and mortality of blood-fed females (47, 48). Negative impact on the adult sandfly longevity was also observed in correlation with Ps. chagasi (43), and this species is known as common pathogen in laboratory-reared colonies of Lu. longipalpis (77).
Gregarines have been reported from more than 20 sandfly species but only a few of them were denominated. A number of studies recorded unidentified gregarine species from sandfly species such as Lutzomyia vexatrix occidentis (50), Lutzomyia shanonni (51), Lu. cruciata (38), Lu. flaviscutellata (49). Acephaline gregarines of one or more species were found in the hemocoel of ten species of sandflies: Lutzomyia camposi, Lu. cruciata, Lu. gomezi, Lu. hartmanni, Lu. panamensis, Lu. sanguinaria, Lu. shannoni, Lu. trapidoi, Lu. trinidadensis, Lu. ylephiletor (52). It is speculated that gregarines are not common parasites of these sandflies as only 18 from more than 6.000 females were found infected (52). Neogregarine were found in the fat body of P. ariasi (33), while trophozoite forms are found in the gut and/or sporocysts in the accessory glands of Lu. whitmani, Lu. edwardsi, Lu.firmatoi, Lu. sallesi, Lu. fischeri and Lu. amarali (53).
Other protist found in sandflies include Adelina sp. that was reported in Se. minuta and P. perniciosus (78, 79). The mechanisms of insect host exploitation and disruption by Adelina sp. is not well-characterized. Studies conducted on Tribolium and several other insects indicate that Adelina sp. can impact behavioral changes, have population regulation role and even cause death of the host insect (80). Adelina sp. are cosmopolitan organisms and the main transmission pathway for this coccidian parasite is via ingestion of contaminated food. Some species develop within the gut and disseminate oocysts through the host feces, while others inhabit the fat tissue (78).
The gregarine infection reduces the resistance of insects, and negatively affects their development and reproduction (81). The studies of sandfly gregarines are unfortunately very rudimentary, and the potential of these protists as agents of biological control of sandflies is underestimated.
Mites are ectoparasites, and they can be phoretic, parasitic or both. Mites attach to the insect exoskeleton, which is harmful to the host, and if they are parasitic, they depend on their insect host for sustenance, maturation, and multiplication. Mites can parasitize on different developmental forms of sandflies, and some are known to affect larvae, while others can be found on adults.
Up to the present moment a total of 15 mite families have been associated with sandflies and they include Ascidae, Acaridae, Cheyletidae, Microtrombidiidae, Microdispidae, Trombidiidae, Erythraeidae, Hydrachnidia, Stigmaeidae, Parasitidae, Phytoseiidae, Uropodidae, Tenuipalpidae, Pterygosomatidae, and Oribatulidae (61). Among them, Microtrombidiidae, Trombidiidae, and Erythraeidae, which are terrestrial parasitengone mites, constitute a large group of natural enemies and in their larval, deutonymphal and/or adult stage are ectoparasites on various arthropod groups including sandflies.
The larva of the Biskratrombium coineaui are ectoparasites of adult P. paptasi, while B. persicum larvae are ectoparasites on the adults of P. alexandri, P. papatasi and Sergentomyia mervynae (57, 58). Sandfly hosts of Microtrombidium hindustanicum are P. papatasi, P. argentipes, P. serganti, Sergentomyia babu and Se. indica (54, 55). Phlebotomus martini is a host for Microtrombidium nigeriense and Kenyatrombium macfarlanei (55, 56), Sergentomyia clydei is a host of Microtrombidium lewisi, while Microtrombidium spp. has been associated with Lutzomyia furcata (55, 56).
Numerous species of the family Stigmaeidae including genus Eustigmaeus and Stigmaeus are sandfly parasites. From genus Eustigmaeus species such as Eustigmaeus dyemkoumai, E. gamma, E. gorgasi, E. johnstoni, E. lirella and E. parasiticus are most frequently found in association with sandflies. Eustigmaeus dyemkoumai is ectoparasite of Phlebotomus duboscqi (59); E. gamma and E. gorgasi are parsiting on P. pius (59); E. lirella on Lutzomyia apache (60) and E. parasiticus on Phlebotomus sp. and Lutzomyia gomezi (59). Eustigmaeus johnstoni is by far found in most sandfly species including Phlebotomus longicuspis, P. papatasi, P. alexandri, P. bergeroti, P. sergenti, Sergentomyia africana, Se. dreyfussi, Se. magna, Se. clydei, and Se. sintoni (61–67). Species in the genus Stigmaeus including St. smithi and St. sinai are found on P. papatasi, Stigmaeus furcatus was found on P. alexandri (61), while Stigrnaeus youngi has been associated with P. martini, P. duboscqi, P. papatasi, P. sergenti, P. argentipes, Sergentomyia theodori, Se. adleri, Se. tiberiadis pakistanica, Se. clydei, Se. schwetzi, Se. magna and Se. sintoni (59, 61, 68, 69). Species of the genus Ledermuelleria, also belonging to family Stigmaeidae, have been found on Phlebotomus pius, Lutzomyia gomezi, and Lu. shannoni, while not fully identified members of the Uropodidae were recorded on Phlebotomus aclydiferus (38, 52). Bochartia sp. has been recorded on Sergentomyia falax (56). Unidentified species from Eustigmaeus and Stigmaeus genus were found on P. perniciosus (70). An adult female of a Phytoseiidae, most likely being Typhlodromus evansi or T. primulae, was found on a Lu. shannoni (38). The research of mites in the field of sandflies was mostly focused on purely reporting the presence of mites (Table 1). Although relatively high number of mite species has been detected in association with sandflies, most of them are parasitic with very limited number of predatory species (38, 71, 82). Their relation and potential impact on sandflies were only briefly explored within the study of Dinesh et al., 2014 (82) showing good potential to be used as agents of biological control. This study evaluated the predatory ability of mites and spiders living in the same dwellings as sandflies. The mites and spiders were collected together with sandflies directly from the field and evaluated under laboratory conditions (82). Nymph and adult forms of mites were attacking and penetrating the larval body, damaging, and eating away its content leading to the death of larvae. The mites caused a decline in sandfly population within 15 days, with only 5% of the larvae being able to reach adult stage. On the contrary, spiders were found more efficient in predating adult forms of sandflies (82). The exact species of these predator mites and spider has not been determined, but it is demonstrated that they have potential to be used as biological agents of sandfly control (82). Most recently, Tyrophagus sp. and Stratiolaelaps scimitus, were recorded among the different species of laboratory reared sandflies (71). These two mite species impacted the productivity of various sandfly colonies leading to significant colony declines (overall colony growth fell drastically by 30.9%-31,6% depending on the sandfly species). It has been shown that mites from the Tyrophagus genus can contribute to the sandfly colony decrease by competing for the food source with sandfly larvae, and by secreting metabolites that stop larval development (83). On the contrary, Stratiolaelaps scimitus are predatory mites that feed on fungus gnats, thrips pupae and other small insects in the soil (84), and their impact on sandfly colonies needs to be investigated to a greater extent (71). Mites are generally commonly observed within sandfly colonies, and if they are present in high numbers without any control mechanisms in place, they can lead to sandfly colony decimation (71, 82). The general presence of mites even more discourages scientists from colonizing sandflies under laboratory conditions which hinders the progress of further studies. The use of predatory mites for the control of insect populations has a long history especially in the agricultural settings (85, 86). Several species of predatory mites dwelling in soil showed success in insect control (84), and these species might be considered for the control of immature forms of sandflies.
Since World War II, the intensive use of chemicals for insect control resulted in development of insecticide resistance/resurgence among the insect populations worldwide. The chemical overuse negatively impacted non-target organisms, overall agroecosystem, crop productivity, human health etc. Due to the multiple adverse effects of insecticides, more eco-friendly approaches that are safe and effective have been developed and tested. The application of entomopathogenic organisms such as nematodes, protists or mites as biocontrol agents has been vastly explored and applied in the field of forestry and agriculture. In comparison, only a little attention was given to blood-sucking insects such as sandflies.
Findings herein reported demonstrated that the majority of studies in the field of sandfly and leishmaniasis control are mainly focused on pure detection and identification of entomopathogenic organisms (Table 1), while the deeper understanding of their interaction with sandflies and overall impact on sandfly population is lacking. Considering the complexity of the sandfly life cycle, and the fact that they have below and above the ground developmental stages, the application of different biological agents for their control might be beneficial. The exploration of predatory and pathogenic organisms, along with the development of paratransgenic approach and the application of green chemistry must be prioritized.
SV: Conceptualization, Writing – original draft, Writing – review & editing.
The author(s) declare that no financial support was received for the research, authorship, and/or publication of this article.
This manuscript is prepared during the authors work on the Paratransgenesis as a weapon against leishmaniasis (PartoLeish) project. European Commission Horizon 2020 Marie Skłodowska-Curie Actions and The Scientific and Technological Research Council of Türkiye (TÜBİTAK) Cofund program (Co-Funded Brain Circulation Scheme2 - CoCirculation2; TÜBİTAK 2236; Project No: 121C042).
The author declares that the research was conducted in the absence of any commercial or financial relationships that could be construed as a potential conflict of interest.
All claims expressed in this article are solely those of the authors and do not necessarily represent those of their affiliated organizations, or those of the publisher, the editors and the reviewers. Any product that may be evaluated in this article, or claim that may be made by its manufacturer, is not guaranteed or endorsed by the publisher.
1. Shimabukuro PHF, De Andrade AJ, Galati EAB. Checklist of American sand flies (Diptera, psychodidae, phlebotominae): Genera, species, and their distribution. Zookeys. (2017) 2017:67–106. doi: 10.3897/zookeys.660.10508
2. Diniz PPVP, Chomel BB, Guptill L, Breitschwerdt EB. Bartonellosis. In: Greene’s infectious diseases of the dog and cat, Fifth Edition, vol. p. (2022). p. 853–75. Elsevier.
3. Jancarova M, Polanska N, Volf P, Dvorak V. The role of sand flies as vectors of viruses other than phleboviruses. J Gen Virol. (2023) 104. doi: 10.1099/jgv.0.001837
4. Torres-Guerrero E, Quintanilla-Cedillo MR, Ruiz-Esmenjaud J, Arenas R. Leishmaniasis: a review. F1000Research. (2017) 6:750. doi: 10.12688/f1000research
5. Serafim TD, Coutinho-Abreu IV, Dey R, Kissinger R, Valenzuela JG, Oliveira F, et al. Leishmaniasis: the act of transmission. Vol. 37 Trends Parasitol. (2021) p:976–87. doi: 10.1016/j.pt.2021.07.003
6. Montenegro Quiñonez CA, Runge-Ranzinger S, Rahman KM, Horstick O. Effectiveness of vector control methods for the control of cutaneous and visceral leishmaniasis: A meta-review. PloS Negl Trop Dis. (2021) 15(5):e0009309. doi: 10.1371/journal.pntd.0009309
7. Garlapati R, Iniguez E, Serafim TD, Mishra PK, Rooj B, Sinha B, et al. Towards a sustainable vector-control strategy in the post kala-azar elimination era. Front Cell Infect Microbiol. (2021) 11. doi: 10.3389/fcimb.2021.641632
8. Balaska S, Fotakis EA, Chaskopoulou A, Vontas J. Chemical control and insecticide resistance status of sand fly vectors worldwide. PloS Negl Trop Dis. (2021) 15:e0009586. doi: 10.1371/journal.pntd.0009586
9. Sabbahi R, Hock V, Azzaoui K, Saoiabi S, Hammouti B. A global perspective of entomopathogens as microbial biocontrol agents of insect pests. J Agric Food Res. (2022) 10:100376. doi: 10.1016/j.jafr.2022.100376
10. Taracena ML, Oliveira PL, Almendares O, Umaña C, Lowenberger C, Dotson EM, et al. Genetically modifying the insect gut microbiota to control chagas disease vectors through systemic RNAi. PloS Negl Trop Dis. (2015) 9(2):e0003358. doi: 10.1371/journal.pntd.0003358
11. Utarini A, Indriani C, Ahmad RA, Tantowijoyo W, Arguni E, Ansari MR, et al. Efficacy of wolbachia-infected mosquito deployments for the control of dengue. N Engl J Med. (2021) 384:2177–86. doi: 10.1056/NEJMoa2030243
12. Vreysen MJB, Saleh K, Mramba F, Parker A, Feldmann U, Dyck VA, et al. Sterile insects to enhance agricultural development: the case of sustainable tsetse eradication on unguja island, zanzibar, using an area-wide integrated pest management approach. PloS Negl Trop Dis. (2014) 8(5):e2857. doi: 10.1371/journal.pntd.0002857
13. Dias JCP. Southern Cone Initiative for the elimination of domestic populations of Triatoma infestans and the interruption of transfusional Chagas disease. Historical aspects, present situation, and perspectives. Memorias do Instituto Oswaldo Cruz. (2007) 4:11–8. doi: 10.1590/S0074-02762007005000092
14. Berry C. The bacterium, Lysinibacillus sphaericus, as an insect pathogen. J Invertebrate Pathology. (2012) 109:1–10. doi: 10.1016/j.jip.2011.11.008
15. Robert LL, Perich MJ, Schlein Y, Jacobson RL, Wirtz RA, Lawyer PG, et al. Phlebotomine sand fly control using bait-fed adults to carry the larvicide Bacillus sphaericus to the larval habitat. J Am Mosq Control Assoc. (1997) 13:140–4.
16. Wijerathna T, Gunathunga S, Gunathilaka N. Recent developments and future directions in the paratransgenesis based control of Leishmania transmission. Biol Control. (2020) 145:104260. doi: 10.1016/j.biocontrol.2020.104260
17. Amóra SSA, Bevilaqua CML, Feijó FMC, Silva MA, Pereira RHMA, Silva SC, et al. Evaluation of the fungus Beauveria bassiana (Deuteromycotina: Hyphomycetes), a potential biological control agent of Lutzomyia longipalpis (Diptera, Psychodidae). Biol Control. (2009) 50:329–35. doi: 10.1016/j.biocontrol.2009.05.004
18. Chitra P, Sujatha K, Jeyasankar A. Entomopathogenic nematode as a biocontrol agent – Recent trends – A Review. Int J Adv Res Biol Sci. (2017) 4:9–20. doi: 10.22192/ijarbs
19. Irsad, Shahid M, Haq E, Mohamed A, Rizvi PQ, Kolanthasamy E. Entomopathogen-based biopesticides: insights into unraveling their potential in insect pest management. Front Microbiol. (2023) 14. doi: 10.3389/fmicb.2023.1208237
20. Tarasco E, Fanelli E, Salvemini C, El-Khoury Y, Troccoli A, Vovlas A, et al. Entomopathogenic nematodes and their symbiotic bacteria: from genes to field uses. Front Insect Sci. (2023) 3. doi: 10.3389/finsc.2023.1195254
21. Temeyer KB, Schlechte KG, Dandeneau LB. Sand fly colony crash tentatively attributed to nematode infestation. J Med Entomol. (2020) 57:1301–4. doi: 10.1093/jme/tjz256
22. Poinar GO, Ferro C, Morales A, Tesh RB. Anandranema phlebotophaga n. gen., n. sp. (Allantonematidae : Tylenchida), a new nematode parasite of phlebotomine sand flies (Psychodidae : Diptera) with notes on experimental infections of these insects with parasitic rhabditoids. Fundam Appl Nematol. (1993) 16:11–6.
23. Souza M, Carvalho R, Wermelingerm E, MaChado R, Ponte C, Lima D, et al. Infecção natural de Lutzomyia fischeri (Pinto, 1912) por nematódeos (Nematoda: Tylenchida) na localidade de pau da fome, município do Rio de Janeiro, estado do Rio de Janeiro, Brasil: Nota de Pesquisa. Rev Ciências Tecnol. (2011) 11:53–5.
24. Tang Y, Killick-Kendrick R, Hominick WM. Life cycle of Didilia ooglypta (Nematoda: Tetradonematidae), a parasite of phlebotomine sandflies of Afghanistan. Nematologica. (1997) 43:491–503. doi: 10.1163/005125997X00084
25. Killick-Kendrick R, Killick-Kendrick M, Qala I Nawi NA, Ashford RW, Tang Y. Preliminary observations on a Tetradonematid Nematode of Phlebotomine sandflies of Afghanistan. Ann Parasitol Hum Comparée. (1989) 64:332–9. doi: 10.1051/parasite/1989645332
26. Secundino NFC, Araújo MSS, Oliveira GHB, Massara CL, Carvalho OS, Lanfredi RM, et al. Preliminary description of a new entomoparasitic nematode infecting Lutzomyia longipalpis sand fly, the vector of visceral leishmaniasis in the new world. J Invertebr Pathol. (2002) 80:35–40. doi: 10.1016/S0022-2011(02)00046-0
27. Killick-Kendrick M, Killick-Kendrick R, Añez N, Nieves E, Scorza JV, Tang Y. The colonisation of Lutzomyia youngi and the putative role of free-living nematodes in the biology of phlebotomine sandfly larvae. Parasite. (1997) 4:269–71. doi: 10.1051/parasite/1997043269
28. El Sawaf BM, El-Sadawy HA, Georgy JM, Kassem HA. Evaluation of Entomopathogenic nematodes against the sand fly vectors Phlebotomus papatasi (Scopoli) and Phlebotomus sergenti “Parrot” (Diptera: Psychodidae). J Egypt Ger Soc Zool. (2011) 62E:41–55.
29. El-Sadawy HA, Ramadan MY, Abdel Megeed KN, Ali HH, El Sattar SA, Elakabawy LM. Biological control of phlebotomus papatasi larvae by using entomopathogenic nematodes and its symbiotic bacterial toxins. Trop Biomed. (2020) 37:288–302.
30. Ashford RW. Sandflies (Diptera: Phlebotomidae) from Ethiopia: taxonomic and biological notes. J Med Entomol. (1974) 11:605–16. doi: 10.1093/jmedent/11.5.605
31. Young DG, Lewis DJ. Pathogens of Psychodidae (Phlebotomine sand flies). Bull World Health Organ. (1977) 55:9–24.
32. Quentin JC. Morphogénèse larvaire du Spiruride Mastophorus muris (Gmelin, 1790). Ann Parasitol Hum comparée. (1970) 45:839–55. doi: 10.1051/parasite/1970456839
33. Killick-Kendrick R, Leaney AJ, Molyneux HD, Rioux AJ. Parasites of phlebotomus ariasi. Trans R Soc Trop Med Hyg. (1976) 70.
34. Rioux JA, Chabaud AG, Bain O, Quentin JC, Durette-Desset MC. Infestation spontanée de Phlebotomus ariasi par Rictularia proni, Spiruride parasite du mulot. Les terriers de mulots sont-ils des gîtes larvaires à Phlébotomes. Ann Parasitol Hum comparée. (1969) 44:757–60. doi: 10.1051/parasite/1969446757
35. Saini P PMA, Mathew J TS, Kumar NP, Kumar A. Genetic characterization of a new entomo−pathogenic nematode (Tylenchida: Allantonematidae) parasite in wild−caught sandflies (Diptera: Psychodidae) from Western Ghats, India. Int J Trop Insect Sci. (2023) 43(6):2145–50. doi: 10.1007/s42690-023-01124-1
36. Karakuş M, Arserim SK, Töz SÖ, Özbel Y. [Detection of entomopathogen nematode [EPN - sand flies (Phlebotomus tobbi)] caught in the wild in Aydin, Kuşadasi town and its assessment as a biological control agent]. Turkiye Parazitol Derg. (2013) 37:36–9. doi: 10.5152/tpd.2013.09
37. Fernández MS, Santini MS, Diaz JI, Villarquide L, Lestani E, Salomón OD, et al. Parasitism by tylenchid nematodes in natural populations of pintomyia fischeri (Diptera: psychodidae: phlebotominae) in Argentina. SM Trop Med J. (2016) 1:1–3. doi: 10.36876/smtmj
38. Lewis DJ. Internal structural features of some central american phlebotomine sandflies. Ann Trop Med Parasitol. (1965) 59:375–85. doi: 10.1080/00034983.1965.11686322
39. Lewis DJ. Phlebotomine sand-flies from cayman brac island (Diptera: psychodidae). J Nat Hist. (1967) 2:73–83. doi: 10.1080/00222936800770631
40. Shortt HE, Swaminath CS. Monocystis mackiei n. sp. parasitic in Phlebotomus argentipes. Ann Brun Indian J Med Res. (1927) 15:539–53.
42. Ostrovska K, Warburg A, Montoya-Lerma J. Ascogregarina saraviae N. Sp. (Apicomplexa: Lecudinidae) in Lutzomyia lichyi (Diptera: Psychodidae). J Protozool. (1990) 37:69–70. doi: 10.1111/j.1550-7408.1990.tb05872.x
43. Wu WK, Tesh RB. Experimental infection of Old and New World phlebotomine sand flies (Diptera: Psychodidae) with Ascogregarina chagasi (Eugregarinorida: Lecudinidae). J Med Entomol. (1989) 26:237–42. doi: 10.1093/jmedent/26.4.237
44. Adler S, Mayrink W. A gregarine, Monocystis chagasi n. sp., of Phlebotomus longipalpis. Remarks on the accessory glands of P. longipalpis. Rev Inst Med Trop Sao Paulo. (1961) 3:230–8.
45. Oliveira SMP, Afonso RCH, Dias CMG, Brazil RP. Ocorrencia de Ascogregarina chagasi em Lutzomyia sallesi e L. sordelli e Ascogregarina saraviae em L. schreiberi, capturados no Rio de Janeiro. In: XIII congresso brasileiro de parasitologia. Rio de Janeiro (1991). p. 102.
46. Brazil BG, Teixeira PMF, Minafra CAJ, Temeljkovitch M, Almeida EC, Brazil R. Infeccao natural de Lutzomyia cruzi (Mangabeira, 1938) (Diptera: Psychodidae: Phlebotominae) por Ascogregarina chagasi (Adler and Mayrink, 1964). Entomol Y Vectores. (2002) 9:289–93.
47. Lantová L, Ghosh K, Svobodová M, Braig HR, Rowton E, Weina P, et al. The life cycle and host specificity of Psychodiella sergenti n. sp. and Ps. tobbi n. sp. (Protozoa: Apicomplexa) in sand flies Phlebotomus sergenti and Ph. tobbi (Diptera: Psychodidae). J Invertebr Pathol. (2010) 105:182–9. doi: 10.1016/j.jip.2010.07.001
48. Lantova L, Svobodova M, Volf P. Effects of Psychodiella sergenti (Apicomplexa, Eugregarinorida) on its natural host Phlebotomus sergenti (Diptera, Psychodidae). J Med Entomol. (2011) 48:985–90. doi: 10.1603/ME11018
49. Lewis DJ, Lainson R, Shaw JJ. Determination of parous rates in Phlebotomine sandflies with special reference to Amazonian species. Bull Entomol Res. (1970) 60:209–19. doi: 10.1017/S0007485300040736
50. Ayala SC. Gregarine infections in the California sandfly, Lutzomyia vexatrix occidentis. J Invertebr Pathol. (1971) 17:440–1. doi: 10.1016/0022-2011(71)90020-6
51. Garnham PCC, Lewis DJ. Parasites of British Honduras with special reference to Leishmaniasis. Trans R Soc Trop Med Hyg. (1959) 53:12–20. doi: 10.1016/0035-9203(59)90080-X
52. McConnell E, Correa M. Trypanosomes and other microorganisms from Panamanian phlebotomus. J Parasitol. (1964) 50:523–8. doi: 10.2307/3275613
53. Mayrink W, Paul W, Coelho MV, Dias M, Martins AV, Magalhães PA, et al. Epidemiology of dermal leishmaniasis in the rio doce valley, state of minas gerais, Brazil. Ann Trop Med Parasitol. (1979) 73:123–37. doi: 10.1080/00034983.1979.11687239
54. Rathnaswamy GK, Ramakrishna NR. Report on a sandfly survey of Madras city. Indian J Entomol. (1954) 16:29–36.
55. Felska M, Wohltmann A, Makol J. A synopsis of host-parasite associations between Trombidioidea (Trombidiformes: Prostigmata, Parasitengona) and arthropod hosts. SystemaT Appl Acarol. (2018) 23:1375–479. doi: 10.11158/saa.23.7
56. Fain A, Baker A. On some larval Microtrombidiidae (Acari: Prostigmata) parasitic on Phlebotomine Sandflies (Diptera: Psychodidae). Bull Annis Soc r beige Ent. (1993) 129:325–39.
57. Fain A, Izri M. Un nouveau Trombidiide larvaire (Acari : Trombidiidae) parasite de Phlébotomes (Insecta, Diptera) d ‘Algérie. Bull Inst r Sci Nat Belg Entomol. (1993) 63:113–7.
58. Majidi M, Hajiqanbar H, Saboori A. The second species of Biskratrombium (Trombidiformes: Microtrombidiidae) ectoparasitic on phlebotomine sandflies (Diptera: Psychodidae) from Iran. Parasitol Res. (2020) 119:795–803. doi: 10.1007/s00436-020-06600-y
59. Lewis D, Macfarlane D. The mites of phlebotomine sandflies (Diptera: Psychodidae). Soc Protozool Spec Publ. (1981) 1:177–83.
60. Reeves WK, Kato CY, Gilchriest T. Pathogen screening and bionomics of lutzomyia apache (Diptera: Psychodidae) in wyoming, USA. J Am Mosq Control Assoc. (2008) 24:444–7. doi: 10.2987/5745.1
61. Majidi M, Hajiqanbar H, Saboori A. Parasitic stigmaeid mites (Acari: Stigmaeidae) of phlebotomine sandflies (Diptera: Psychodidae) in Fars Province, southern Iran. Int J Acarol. (2019) 45:41–7. doi: 10.1080/01647954.2018.1549098
62. Zhang Z, Gerson U. Eustigmaeus johnstoni, new species (Acari: Stigmaeidae), parasitic on phlebotomine sandflies (Diptera: Psychodidae). Tijdschr voor Entomol. (1995) 138:297–301.
63. Shehata M, Baker A. Mites infesting phlebotomine sandflies in southern Sinai, Egypt. Med Vet Entomol. (1996) 10:193–6. doi: 10.1111/j.1365-2915.1996.tb00730.x
64. Ozbel Y, Akkafa F, Ozensoy S, Balcioglu I, Ulukanligil M, Alkan MZ. Mites of Phlebotomus sergenti collected in Sanliurfa, Turkey. Acta Parasitol Turc. (1999) 23:153–5.
65. Badakhshan M, Sadraei J, Moin-Vaziri V. The first report of Eustigmaeus johnstoni (Acari: Stigmaeidae)parasitic mite of phlebotominae sand flies from Iran. J Arthropod Borne Dis. (2013) 7:94–8.
66. Pekağırbaş M, Karakuş M, Arserim SK, Doğan S, Eren H, Töz S, et al. Eustigmaeus johnstoni Zhang - Gerson (Acari: Stigmaeidae) a Parasitic Mite Species Detected in Phlebotomus papatasi (Diptera: Psychodidae) Specimens Collected from Aydın, Turkey. Turkiye parazitolojii Derg. (2017) 41:139–42. doi: 10.5152/tpd.2017.5248
67. Pekağırbaş M, Karakuş M, Yilmaz A, Erişöz Kasap Ö, Sevsay S, Özbel Y, et al. Two parasitic mite species on Phlebotominae sand flies (Diptera: Psychodidae) from Türkiye: Biskratrombium persicum (Microtrombidiidae) and Eustigmaeus johnstoni (Stigmaeidae). Acarol Stud. (2023) 5:11–6. doi: 10.47121/acarolstud.1209774
68. Hirst S. Report on the Acari found on or associated with sand flies in India. Indian J Med Res. (1926) 13:1023–6.
69. Wood T. Redescription of stigmaeus youngi (Hirst): acari, stigmaeidae. Acarologia. (1972) 53:383–415.
70. Martinez Ortega E, Conesa Gallego CE, Macfarlane D, Ward RD. Ectoparasitic mites on phlebotomine sandflies (Diptera: Psychodidae) from Spain. Ann Trop Med Parasitol. (1983) 77:545–6.
71. Nzelu CO, Meneses C, Bowhay C, Coutinho-Abreu IV, Bennett E, Bahrami S, et al. Disentangling detrimental sand fly-mite interactions in a closed laboratory sand fly colony: implications for vector-borne disease studies and guidelines for overcoming severe mite infestations. Parasites Vectors. BioMed Central;. (2024) 17(1):11. doi: 10.1186/s13071-023-06074-8
72. Lacey LA, Georgis R. Entomopathogenic nematodes for control of insect pests above and below ground with comments on commercial production. J Nematol. (2012) 44:218–25.
73. Bessette E, Williams B. Protists in the insect rearing industry: benign passengers or potential risk? Insects. (2022) 13(5):482. doi: 10.3390/insects13050482
74. Warburg A, Ostrovska K. Host-parasite relationships of Ascogregarina chagasi (eugregarinorida, aseptatorina, lecudinidae) in Luzomyia longipalpis (Diptera: Psychodidae). Int J Parasitol. (1991) 21:91–8. doi: 10.1016/0020-7519(91)90124-P
75. Munstermann LE, Levine ND. Ascogregarina geniculati sp. n. (Protozoa, Apicomplexa) from the Mosquito Aedes geniculatus. J Parasitol. (1983) 69:769. doi: 10.2307/3281155
76. Munstermann LE, Wesson DM. First record of Ascogregarina Taiwanensis (Apicomplexa: Lecudinidae) in North American Aedes albopictus. J Am Mosq Control Assoc. (1990) 6:235–43.
77. Dougherty MJ, Ward RD. Methods of reducing Ascogregarina chagasi parasitaemia in laboratory colonies of Lutzomyia longipalpis. Parassitologia. (1991) 33 Suppl:185–91.
78. Rioux J-A, Léger N, Manier J-F, Croset H. Adelina sp., parasite de phlebotomes. Ann Parasitol Hum Comparée. (1972) 47:347–50. doi: 10.1051/parasite/1972472347
79. Milutinović M, Petrović. Z, Miščević Z, Biševac L. The finding of Adelina sp. (Coccidia, Adeleidae) and microfilariae (Filariata, Filariidae) in sandflies (Diptera, Phlebotomidae) in the area of Ulcinj-Yugoslavia. Acta Vet Brno. (1995) 45:331–6.
80. Santana-Hernández KM, Priestnall SL, Modrý D, Rodríguez-Ponce E. Dispersion of adeleid oocysts by vertebrates in Gran Canaria, Spain: Report and literature review. Parasitology. (2021) 148:1588–94. doi: 10.1017/S0031182021001244
81. Lantova L. Sand fly gregarines: their molecular characterization and host-parasite interactions. [dissertation/master’s thesis]. Univerzita Karlova, Přírodovědecká fakulta (2011).
82. Dinesh DS, Kumar V, Kesari S, Das P. Mites and spiders act as biological control agent to sand flies. Asian Pacific J Trop Dis. (2014) 4(S1):S463–6. doi: 10.1016/S2222-1808(14)60491-6
83. Volf P, Volfova V. Establishment and maintenance of sand fly colonies. J Vector Ecol. (2011) 36:S1–9. doi: 10.1111/j.1948-7134.2011.00106.x
84. Beretta GM, Deere JA, Messelink GJ, Muñoz-Cárdenas K, Janssen A. Review: predatory soil mites as biocontrol agents of above- and below-ground plant pests. Exp Appl Acarol. (2022) 87:143–62. doi: 10.1007/s10493-022-00723-w
85. Van Driesche RG, Lyon S, Stanek EJ, Xu B, Nunn C. Evaluation of efficacy of Neoseiulus cucumeris for control of western flower thrips in spring bedding crops. Biol Control. (2006) 36:203–15. doi: 10.1016/j.biocontrol.2005.08.011
Keywords: sandfly, nematode, mite, protist, entomopathogens, insect control
Citation: Vaselek S (2024) The role of protists, nematodes and mites as natural control agents of sandfly populations. Front. Trop. Dis 5:1369007. doi: 10.3389/fitd.2024.1369007
Received: 11 January 2024; Accepted: 27 February 2024;
Published: 28 March 2024.
Edited by:
Oscar Daniel Salomón, National Institute of Tropical Medicine (INMeT), ArgentinaReviewed by:
Fernando Ariel Genta, Oswaldo Cruz Foundation (Fiocruz), BrazilCopyright © 2024 Vaselek. This is an open-access article distributed under the terms of the Creative Commons Attribution License (CC BY). The use, distribution or reproduction in other forums is permitted, provided the original author(s) and the copyright owner(s) are credited and that the original publication in this journal is cited, in accordance with accepted academic practice. No use, distribution or reproduction is permitted which does not comply with these terms.
*Correspondence: Slavica Vaselek, c2xhdmljYS52YXNlbGVrQGdtYWlsLmNvbQ==
Disclaimer: All claims expressed in this article are solely those of the authors and do not necessarily represent those of their affiliated organizations, or those of the publisher, the editors and the reviewers. Any product that may be evaluated in this article or claim that may be made by its manufacturer is not guaranteed or endorsed by the publisher.
Research integrity at Frontiers
Learn more about the work of our research integrity team to safeguard the quality of each article we publish.