- 1International Centre of Insect Physiology and Ecology (icipe), Nairobi, Kenya
- 2Department of Biochemistry and Biotechnology, Technical University of Kenya (TU-K), Nairobi, Kenya
- 3Department of Zoology and Entomology, University of Pretoria, Pretoria, South Africa
Introduction: Previously, we documented that the malaria vector Anopheles gambiae responds to volatile emissions from the root exudate water of the invasive plant, Parthenium hysterophorus. However, the origin of the volatiles remains to be investigated. Here, we isolated bacteria from the root exudate water of the plant, test the influence of their volatiles in gravid An. gambiae oviposition, and examined relationships between volatile profiles and oviposition.
Methods: Bacteria from root exudate water of P. hysterophorus were isolated using culture on Luria Bertani medium and identified by sequencing the 16S rRNA gene. Cultures of individual isolates were evaluated for egg laying response by gravid An. gambiae and number of eggs laid compared using generalized linear models relative to those in crude bacteria-mixture. Headspace volatile emissions of the bacterial isolates were analyzed by gas chromatography coupled to mass spectrometry (GC-MS) and relationships between volatile organic compound (VOC) profiles and gravid mosquito oviposition examined using Random Forest Analysis. Proximate analysis was performed to assess the difference in volatile chemistry among the different isolates.
Results: Three isolates were identified as Gram-negative bacteria belonging to two families: Enterobacteriaceae (Enterobacter sp. and Enterobacter mori) and Alcaligenaceae (Alcaligens aquatilis). An. gambiae laid 3-fold more eggs in cultures of A. aquatilis than in those of Enterobacter sp. In turn, approx. 4-fold more eggs were laid in cultures of E. mori than A. aquatilis. Overall, 16 VOCs were identified in the headspace of the isolates belonging to the chemical classes benzenoids, pyrazines, aldehydes, terpenes, alcohols, alkanes, and indoles. Random Forest Analysis identified 10 compounds contributing the most to the attraction of odors of the bacteria isolates to oviposition. Specifically, dodecane and indole were emitted in higher amounts in odors of Enterobacter sp than the other two species. Proximate analysis revealed differential attraction of the isolates on the gravid mosquito to be associated with their volatile profiles.
Conclusion: Our results provide first report of E. mori or A. aquatilis mediating attractive oviposition responses in An. gambiae in support of the important role microbes play in insect oviposition. The potential use of the microbes and associated volatiles in malaria vector management needs further investigation.
Introduction
Malaria is a major public health threat in sub-Saharan Africa, where the disease kills thousands of people each year (1). The disease is caused by Plasmodium parasites transmitted among people by the bites of infective female Anopheles mosquitoes (1). To break the parasite transmission cycle and reduce the disease burden, control efforts have largely focused on interventions that target Anopheles vectors. Examples include long lasting insecticide nets (LLINs) and indoor residual spraying (IRS), both targeting mosquitoes that feed and rest indoors (2–4). However, impediments to effective control using these insecticide-based tools include the emergence and spread of insecticide resistance among major malaria vectors (5, 6). As such, it is essential to embrace alternative ways to control vector populations. For example, semiochemical-baited traps, especially those that mediate oviposition represents promising complementary tools for mosquito control (7–10).
To ensure reproductive success, gravid mosquitoes are known to identify and select oviposition sites that can support the growth and survival of their offspring (9, 10). Detection of these preferred oviposition environments by gravid mosquitoes is achieved by integrating a range of cues including olfactory, tactile and visual cues (9). These oviposition cues may be exploited in vector control programmes in several ways. Firstly, olfactory cues can be used to deter mosquitoes from egg laying in a particular environment (8). Secondly, in a “lure and kill” strategy, oviposition attractants can be used as baits to attract gravid mosquitoes in ovitraps laced with larvicidal compounds (11–13). Also, oviposition attractants can be applied as baits in traps for surveillance to track spatial and temporal distribution of mosquitoes (7, 12). Successful deployment of oviposition cues in vector surveillance/control requires an understanding of how gravid females interact with the semiochemicals.
Previous studies have shown that mosquitoes largely rely on olfactory cues to select suitable oviposition substrates (12). Currently, the most documented olfactory cues are those of plant origin because plants constitute most of the biomass in the terrestrial ecosystem. Examples of oviposition attractants that are plant-derived include α-pinene, 6-methyl-5-hepten-2-one (sulcatone), 3-carene, ß-caryophyllene, decanal, nonanal, p-cymene, o-xylene, styrene, 1,8-cineole, N-ethylbenzenamine, undecane and eicosane (14, 15). Some of these compounds elicit antennal responses (14, 16) and in blends attract gravid malaria vector Anopheles arabiensis in laboratory behavioral assays (14, 15). Microorganisms including bacteria, fungi and algae have also been linked to emission of volatiles that attract mosquitoes to oviposition sites. For example, the An. arabiensis oviposition attractant cedrol is produced by the fungal species, Fusarium sp. found on grass rhizomes (11, 17). The algal (Spirogyra majuscule) volatiles ethyl acetate, pentacosane, hexacosane, octacosane and docosane have been demonstrated to mediate oviposition behavior of Anopheles pseudopectipennis (18). Bacteria isolated from soil and water of oviposition sites were found to elicit oviposition response in An. gambiae mosquitoes (13, 19). Similarly, volatiles of bacteria origin (e.g. geosmin) and from plant infusions have been implicated in the attraction of other gravid mosquito species (20–23). However, the volatile profile of a given oviposition substrate may depend on the inhabiting microbial species.
To date, few data exist on the bacterial diversity associated with plant root exudates as well as their attractive potential to gravid mosquitoes. Malaria vector Anopheles arabiensis commonly feeds on plants and selectively on the invasive species P. hysterophorus (16). Coincidentally this plant has spread over a large expanse of East Africa, especially western Kenya, which records the highest burden of malaria in Kenya (24). Previously, we documented that the An. gambiae preferred host-plant P. hysterophorus, releases volatiles in its root exudates that attract gravid female mosquitoes (24). In the current study, we posited that microorganisms, specifically bacteria in the root exudate water emit volatiles that influence gravid mosquito attraction. The purpose of the current study was to (a) isolate and identify the bacteria associated with the root exudate water of P. hysterophorus, (b) test the oviposition responses of gravid An. gambiae to volatile emissions of the isolated bacteria, and (c) analyze the bacterial volatile emission profiles.
Materials and methods
Plants
Seeds were collected from wild P. hysterophorus growing at the garden of the International Centre of Insect Physiology and Ecology (icipe), Nairobi, Kenya (1°16′60″S; 36°49′0″E). The seeds were air-dried and planted in plastic pots (12 cm base diameter, 23 cm top diameter and 22 cm depth) with sterilized sand (autoclaved at 121°C for 40 min) and grown using nutrient solution as previously described (25). The plants were watered daily and maintained in a screen house at 22 ± 2 °C and 60–70% relative humidity (RH).
Root exudate water
Flowering P. hysterophorus plants (~6 weeks old), were removed from the plastic pots gently avoiding root damage. Thereafter, the roots were wrapped with aluminum foil to maintain the below ground environment. The plants were transported to the laboratory and roots washed gently with distilled water to remove soil. The plant roots were then sterilized using 0.1% sodium hypochlorite and rinsed with sterilized water. Batches of four plants were soaked in 50 ml of distilled water in a glass beaker wrapped with four layers of aluminum foil held tightly to the flask with three tight-fitting rubber bands. After 24 h, the plants were removed and discarded and the water containing the exudate transferred into sterile 50 mL falcon tubes. This experiment was performed in triplicate to obtain root exudate water for bacteria isolation and chemical analysis.
Bacteria
The bacteria used in headspace solid phase micro-extraction (HS-SPME) volatile collection were grown in Luria bertani (LB) agar and incubated overnight at 37°C. Briefly, 100 µL of the root exudate were spread out on the agar using sterile beads. The mixed cultures were then subjected to subsequent sub-culturing to obtain pure cultures. The pure colonies were classified based on macroscopic observation (shape, size and color). The individual colonies were re-inoculated in LB broth to obtain stock bacterial cultures for use in mosquito oviposition bioassays and molecular analysis to identify the isolates.
DNA extraction and identification of bacterial isolates
To obtain bacteria cells, I mL of each pure isolate was aliquoted into 1.5 m L vial and centrifuged for 5 min at 800xg. Thereafter the supernatant was removed, the pellet re-suspended in lysis buffer and DNA was extracted from the isolated bacteria using the Isolate II Genomic DNA extraction Kit (Bioline, London UK) as per the manufacturer’s protocol. PCR was used to amplify a portion of the 16S rRNA gene using the V1-V3 primers Forward: 5’-GAGTTTGATCNTGGCTCAG-3’ and Reverse: 5’-GTNTTACNGCGGCKGCTG-3’ (26). The PCR reaction (20µL) contained 10 μM of each forward and reverse primer, 4xTaq Rxn buffer (Bioline, London UK) and 0.4 µL of Mytaq DNA polymerase, 11.1 µL of nuclease free water and finally 2µL of DNA sample (~12.5ng). The PCR reaction was carried out in Pro-Flex Thermol Cycler with cycle conditions consisting of initial denaturation at 95°C for 1 min, followed by 35 cycles of 95°C for 15 s, 60°C for 20 s, 72°C for 10 s and a final extension at 72°C for 10 min. Amplicons were resolved in agarose gel (1.5%) electrophoresis after staining with ethidium bromide. The PCR products were purified using QIAquick PCR & Gel cleanup Kit (Qiagen Ltd, North Manchester, UK) following the manufacturer’s protocol, and outsourced for Sanger sequencing at Macrogen Europe BV (Amsterdam, the Netherlands) using both primers. The resulting sequences were cleaned and aligned using MEGA v. 11 (27) and queried against the National Center for Biotechnology and Information (NCBI) database (http://blast.ncbi.nlm.nih.gov/Blast.cgi) with the online BLASTn tool (28). Sequence homology greater than >94% to GenBank database reference sequence were used as the criteria for assigning the identity of each isolate (22, 28).
Mosquitoes
Anopheles gambiae (Mbita strain) used in this study were from a colony established in 2018 and maintained at icipe. The adults were reared at 72% relative humidity (RH) and 28 ± 2°C with a photoperiod of 12 h: 12 h (Light : Dark). For egg development, the mosquitoes were offered human blood through arm feeding, two to three times weekly and had ad libitum access to 6% glucose water solution (wt:vol). Gravid females were selected and used for oviposition experiments. Eggs were laid in oviposition cups (7 cm top diameter, 7 cm depth and 4 cm base diameter) lined with Whatman filter paper (GE Healthcare UK Ltd, Buckinghamshire, London, UK) and transferred to rearing trays (39 × 28 × 4 cm depth). On hatching, larvae were reared at a density of ~500 per tray and the rearing water was changed after every two days. All larval instars were fed daily with Tetramin fish food (Tetra, Germany) until pupation. Pupae were transferred to emergence cups containing 15 ml water and placed in a new cage (15 x 15 x 15 cm). Emerged adults (1 day old) were maintained on 6% glucose water solution as described above.
Oviposition response assays
Three bacterial isolates were assessed for their ability to influence oviposition response of gravid An. gambiae in a dual-choice assay. Twelve gravid mosquitoes were presented a choice between the treatment (bacteria suspended in saline water) and normal saline water (0.9% w/v) contained in similar oviposition cups and the number of eggs laid counted using a microscope (Leica M127, Switzerland) after every 24 h for four consecutive days (24). The oviposition cups were placed diagonally in the experimental cages and their positions interchanged every 24 h to avoid positional bias (22, 24). Each cup was lined with a Whatman filter paper (GE Healthcare UK Ltd, Buckinghamshire, London, UK) and filled with 30 ml of the test solution (saline water with one of the bacterial species) or an equivalent volume of saline water as the control (Saline normally should not contain any bacteria) (13). The bioassays were performed in triplicate and repeated once. The oviposition bioassays were performed with each isolate at three concentrations of different optical densities (OD) i.e. 0.1-0.2 (5.4x106 bacteria cells/ml), 0.5-0.6 (1.1x107 cells/ml) and 0.8-0.9 (2.2x107 cells/ml) (12). Estimate of the concentrations were obtained by counting bacteria cells of known OD (dilution factor of X20) under microscope using a hemecytometer.
Solid phase micro-extraction
Individual bacteria isolates grown in LB agar in a 90 mm Petri dish were placed inside a 250 mL glass beaker and sealed with aluminum foil. The headspace volatiles were collected by solid phase microextraction (SPME) using polydimethylsiloxane/Divinylbenzene (PDMS/DVB) fiber (Supelco, Bellefonte, USA) pre-cleaned via thermal desorption at 250 °C for 30 min. Volatiles were trapped for 24 hr and then analyzed by thermal desorption immediately in a gas chromatography-mass Spectrometry (GC-MS). Flasks with LB agar only served as controls. In preliminary analysis, the GC-MS profile of bacterial isolates in agar and broth were observed to be similar (data not shown), hence the use of agar method was selected because of ease of mobility and low risk of contamination.
Gas chromatography-mass spectrometry analysis
Volatiles were analyzed on a 7890B gas chromatograph (Agilent Technologies, Inc., Santa Clara, CA, USA) coupled to a mass spectrometer (5977A series). The GC was fitted with a HP-5 MS low bleed capillary column (30m x 0.25 mm i.d., 0.25 µm) (J&W, Folsom, CA USA) and helium served as the carrier gas at a flow rate of 1.2 ml/min. The inlet temperature was set at 270 °C whilst the transfer line temperature was 280 °C. The column oven temperature was programmed from 35 to 285 °C, with the initial temperature maintained for 5 min then 10 °C/min to 280 °C for 5.5 min and finally 5 °C/min to 285 °C for 34.9 min. The mass selective detector was maintained at ion source temperature of 230 °C and a quadrupole temperature of 180 °C. Electron impact (EI) mass spectra were obtained at the acceleration energy of 70 eV. SPME-collected volatiles were analyzed by manually inserting the fiber into the GC-MS injector port (250 °C). Fragment ions were analyzed over 38-550 m/z mass range in the full scan mode. The filament delay time was set at 3.0 min. The samples were analyzed in triplicate. The volatile compounds were identified based on the mass spectral library data from Adams and National Institute of Standards and Technology (NIST). Pure standards from sigma analyzed in the same conditions were also used for comparison.
Statistical analysis
The oviposition activity index (OAI) was calculated according to the formula described in (23);
Where Nt refer to the number of eggs laid in the treatment while Nc the number of eggs laid in control (water). OAI ranges from -1 to +1; 0 indicates a neutral response; a positive value indicates an attraction towards the treatment while negative value indicates the converse. The difference in oviposition activity index was compared using one-way ANOVA and Duncan post-hoc test. The number of eggs laid per treatment was analyzed by generalized linear model (GLM) using negative binomial. The model validity was assessed by inspection of residuals (24). The numbers of eggs laid per treatment served as the response variable while the treatments were used as the predictor variable. Mean of peak areas (n=3) of VOCs obtained using GC-MS was also compared using one-way ANOVA and Duncan post-hoc test. Principle component analysis was performed on the VOC profile of the bacteria isolates. All statistical analyses were performed using SPSS 23.0 software (IBM SPSS Statistics) and results considered significant at p ≤ 0.05. Random Forest Analysis (RFA) was performed using the random forest package in R software version 3.4.3 (R core development team). The relative abundance of each identified VOC following GC/MS analysis was used in the RFA analysis executed by running 100,000 iterations (ntree) with 4 volatiles randomly selected at each split (mtry=√q where q is the total number of volatiles) from a total of 16 variables (VOCs). The function ‘importance’ was used to generate the mean decrease in accuracy (MDA) and volatile with the highest value considered the most important (24).
Results
Identification of bacterial cultures
Using Luria bertani (LB media), three bacterial strains were isolated from P. hysterophorus root exudate water. The pure colonies were designated as Isolate A-C based on macroscopic morphological differences (e.g. shape, size, color). For each isolate, a fragment of the 16S rRNA gene was amplified and sequenced followed by query in GenBank database. Sequences (477-485 nt) of two of the isolates were identical to the Gram-negative bacteria species Enterobacter mori (95% identity) and Enterobacter sp. (99% identity) in the GenBank (Table 1) with both belonging to the family Enterobacteriaceae. The other isolate, also a Gram-negative bacterium showed a 94% similarity to Alcaligens aquatilis belonging to the family Alcaligenaceae (Table 1).
Oviposition response of gravid mosquitoes to bacterial isolates
Gravid mosquitoes were presented with a choice between saline water (control, 0.9% w/v) and treatment [either crude bacterial mixture, isolate A (Enterobacter sp.), B (A. aquatilis) or C (E. mori)]. As stated earlier, each treatment was tested at three concentrations of different OD (i.e. 0.1-0.2, 0.5-0.6 and 0.8-0.9). Based on the number of eggs laid in each treatment relative to the control, the oviposition activity index (OAI) was estimated as a measure of attractiveness of each isolate to the gravid mosquito (Figure 1). Overall, the crude bacterial mixture was attractive to the mosquitoes at the lowest optical density of 0.1-0.2 (68.7% of eggs laid, OAI=0.37( ± 0.3), χ22,57=12.2, p<0.001) while the higher bacterial densities were less attractive (4.8%, OAI= -0.9( ± 0.1), χ22,57=9.7, p<0.001). Similarly, Enterobacter sp. and A. aquatilis at the highest bacterial density (OD of 0.8-0.9) were less attractive to the mosquitoes; 36.0%, OAI = -0.26 ( ± 0.3), χ22,142=0.4, p=0.51, and 16.7%, OAI= -0.67 ( ± 0.3), χ2 2,769=30.95, p<0.001 respectively. Likewise, at the lowest bacterial density (OD of 0.1-0.2), gravid mosquitoes avoided odors released from A. aquatilis (39.0%, OAI= -0.22 ( ± 0.4), χ22,148=0.3, p=0.58 and E. mori (27.1%, OAI= -1.00 ( ± 0.4), χ22,130=1.4, p=0.23, respectively. Notably, for each of the bacterial isolate, the highest OAI was recorded at the OD of 0.5-0.6; this optimum density was selected for further evaluation in binary oviposition assays.
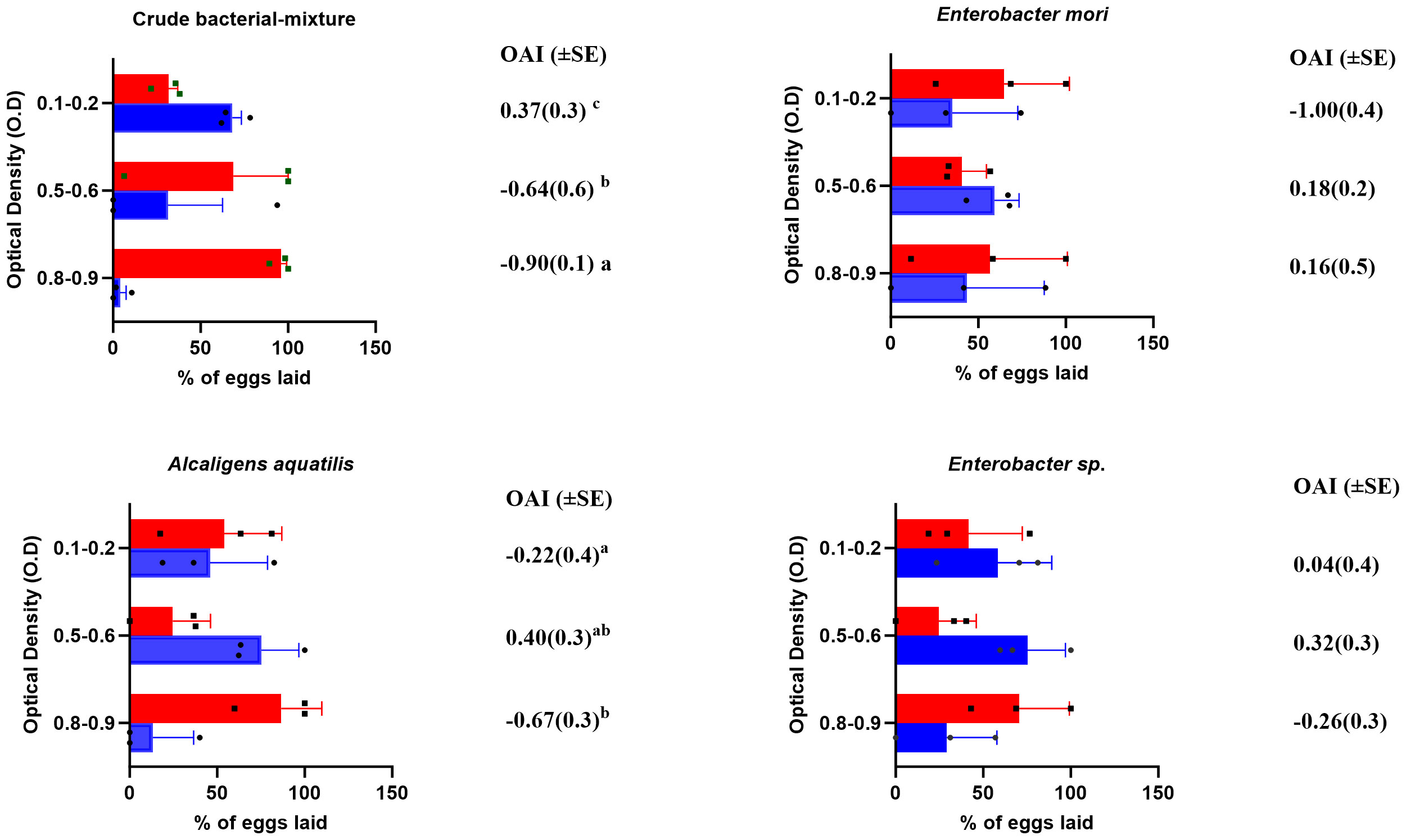
Figure 1 A bar graph showing oviposition responses of gravid mosquitoes at different doses of bacterial isolates i.e. 0.1-0.2 (5.4x106 cells/ml), 0.5-0.6 (1.1x107 cells/ml) and 0.8-0.9 (2.2x107 cells/ml). Crude bacteria mixture: At low (0.1-0.2/5.4x106 cells/ml) and medium doses (0.5-0.6/1.1x107 cells/ml), most eggs were laid in the control and less in the treatment, but at the highest dose (0.8-0.9/2.2x107 cells/ml) most eggs were laid in the treatment arm of the experiment; E. Mori & A. aquatilis: For these treatments, at the medium dose, most eggs were laid in the arm containing the bacterial isolates than in the control. Enterobacter sp.: A higher proportion of eggs were laid in the treatment arm at medium and higher doses. Optical density (OD) is the proxy estimate for bacteria mass per unit volume; SE=standard error; Difference in OAI was detected using one-way ANOVA and Duncan post-hoc test at p ≤ 0.05. Letters a, b and c indicate differences between treatments. The bars in green indicate proportion of eggs laid in the control water whilst the alternative colors indicate the proportion of eggs laid in the treatments.
Females laid significantly more eggs in E. mori odors than to those released by A. aquatilis (χ25,284=5.6, p=0.02, Figure 2). Overall, twice as many more eggs were laid in the treatment containing E. mori than in A. aquatilis (Figure 2). On the other hand, significantly more eggs were laid in the treatment containing A. aquatilis than Enterobacter sp. (χ2 5,187=3.9, p=0.04, Figure 2). Notably, none of the isolates was as attractive as the crude bacterial mixture (Figure 2).
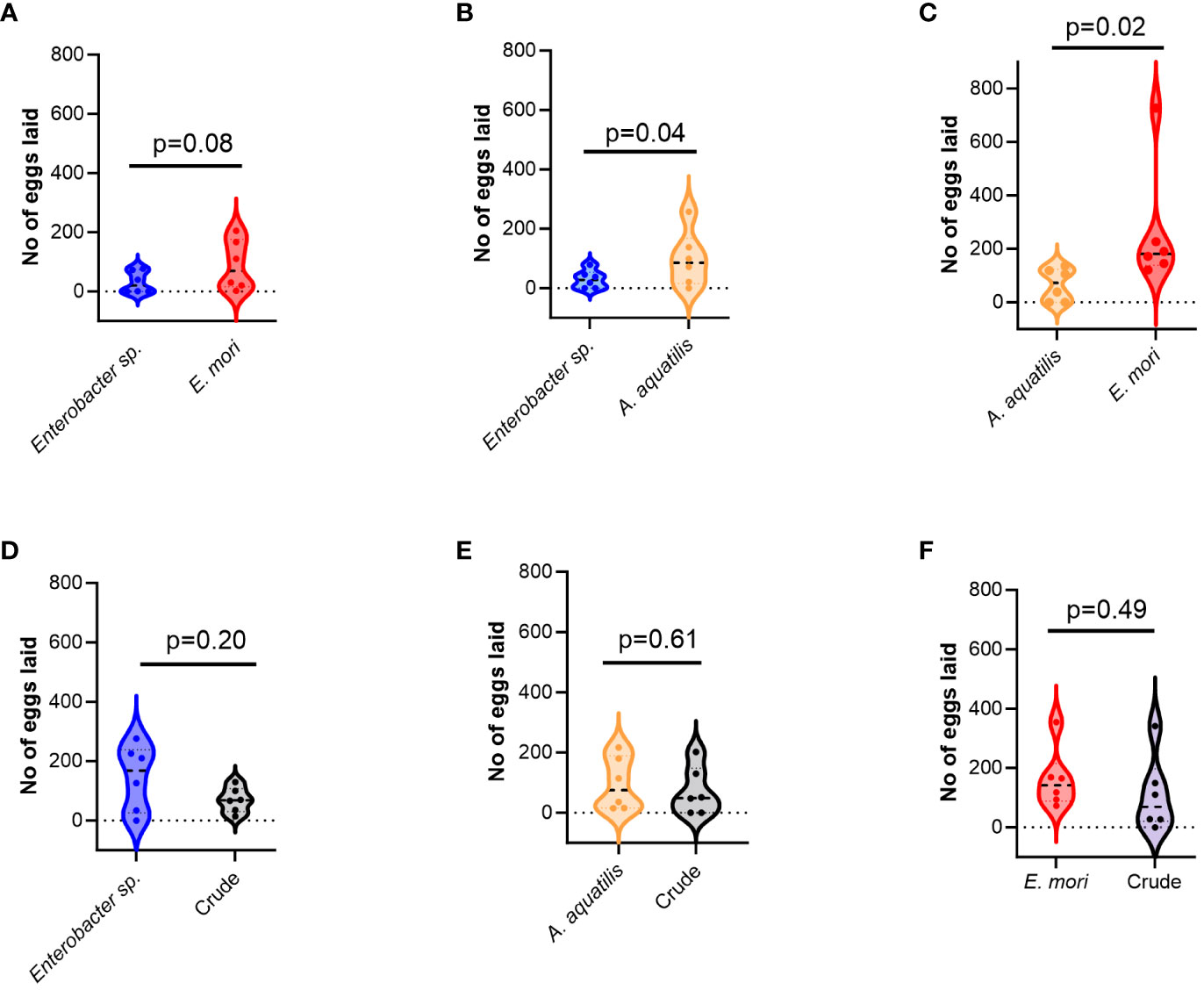
Figure 2 Violin plots depicting number of eggs laid by gravid An. gambiae when given a binary choice between different pairs of treatments. (A) Eggs laid in response to Enterobacter sp and E. mori odors was not significantly different (B) Females laid significantly more eggs in treatment containing A. aquatilis odors than Enterobacter sp. (C) Twice as many more eggs were laid in the treatment containing E. mori than in A. aquatilis (D–F) none of the isolates was as attractive as the crude bacterial mixture
Analysis of bacterial volatiles
In GC-MS analysis, a total of 16 volatile organic compounds (VOCs) were identified in the headspace of the bacterial isolates belonging to different chemical classes namely benzenoids (styrene), pyrazines (2,6, dimethyl-pyrazine), aldehydes (benzaldehyde, nonanal, decanal), terpenes (1,8 cineole, o-cymene, p-cymene, cedrene), alcohols (phenylethyl alcohol), alkanes (dodecane, tetradecane, pentadecane, hexadecane) and indole. Quantitative analysis revealed a heterogeneous distribution of VOCs among the bacteria species (Table 2). For example, whereas benzaldehyde, was 16-fold more abundant in E. mori than in the cultures of other bacterial isolates, cedrene and phenyl ethyl alcohol were 6- and 28- fold respectively, more abundant in A. aquatilis than in E. mori. On the other hand, Enterobacter sp. released higher amounts of dodecane, 2,6-dimethyl-pyrazine and indole (Table 2). RFA identified dodecane and indole as the most important compounds (abundant and permanently present) contributing to oviposition responses (Figure 3A). Principle component analysis on the VOC profiles showed separation of the three isolates (Figure 3B).
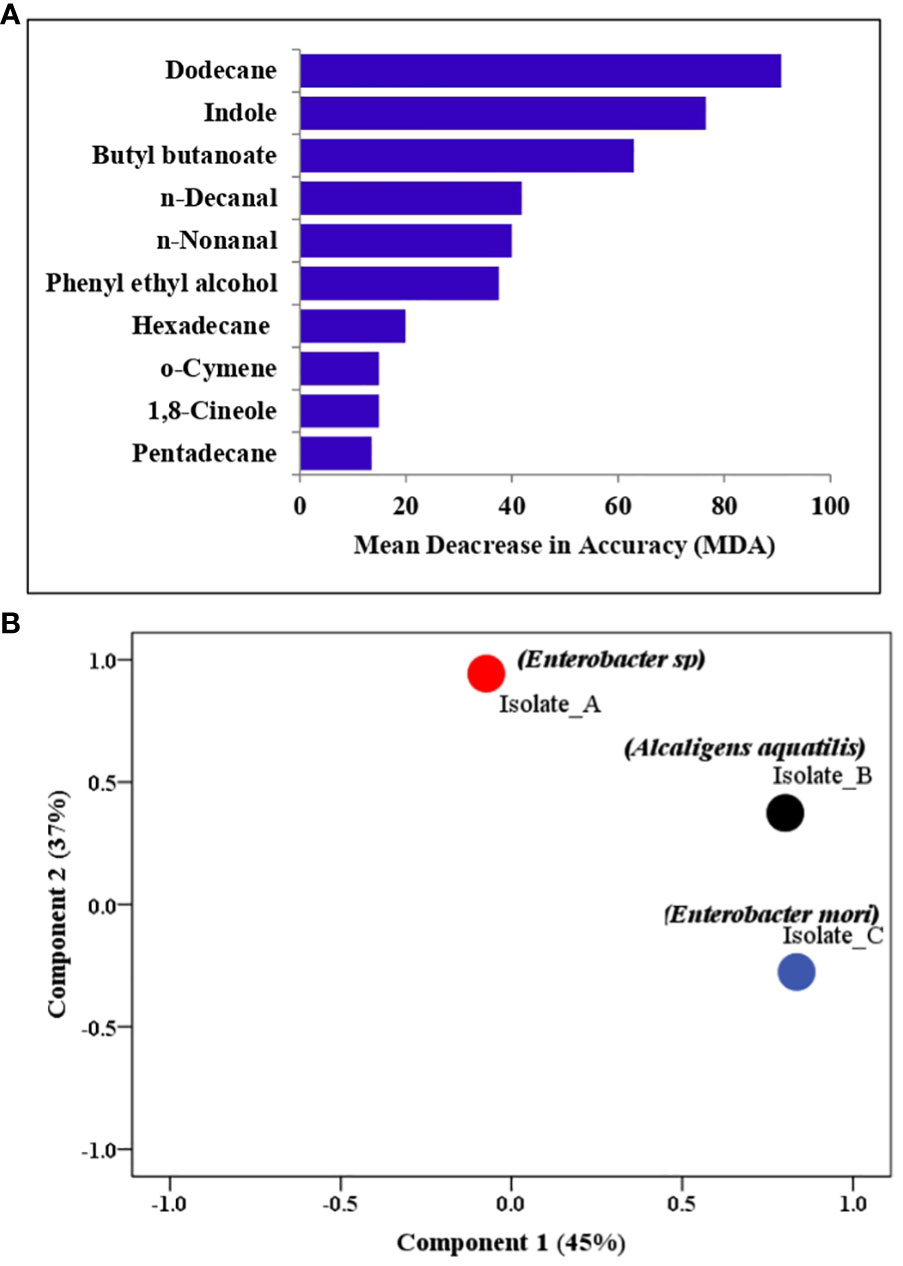
Figure 3 (A) Bar graph depicting the most important compounds (abundant and permanently present) contributing to oviposition responses (B) Principal component analysis (PCA) plots showing the variation of the isolates based on the VOCs profile. Component 1 explained 37% of the total variation while component 2 explained 45% of the total variation.
Discussion
Selection of suitable oviposition sites is a critical determinant of reproductive success in mosquitoes (9). In this study, we evaluated the attractive ability of cultivated bacteria from plant root exudate to the gravid malaria mosquito and analyzed volatiles emitted by these microbes. We show that bacteria associated with P. hysterophorus root exudate alter egg-laying decisions by gravid mosquitoes. Furthermore, our analyses reveal VOCs emitted by the associated bacterial species, and variation in the profiles which could account for differences in responses. The data is consistent with previous studies implicating microbial influence of odor-oriented behaviors in mosquito oviposition (11, 13, 17).
By employing a culture-based approach, only three bacterial isolates were recovered and identified as Enterobacter sp. (Enterobacteriaceae), A. aquatilis (Alcaligenaceae) and E. mori (Enterobacteriaceae). Odors from these isolates elicited differential oviposition responses in gravid An. gambiae. We found that olfactory cues from the bacteria E. mori were more attractive to gravid females than those from A. aquatilis. In turn, the mosquito was more attracted to A. aquatilis than Enterobacter sp. This finding is not uncommon as previous studies have reported variation in attractiveness to mosquitoes by different bacterial species (13). Bacteria belonging to the family Enterobacteriaceae have been associated with changes in oviposition behavior of Anopheles species (13). Other bacterial families that have been implicated in oviposition response but not identified in the present study include Bacillaceae, Nocardiaceae, Micrococcaceae, Paenibacillaceae and Comamonadaceae (13). The difference could relate to the oviposition substrate type (23). For example, in most previous investigations on mosquito oviposition behavior, the isolated bacteria were from substrates such as soil, lake water, plant infusion and human skins (13, 20, 28, 29). However, influence of culture media type on bacterial composition (29) cannot be ruled out, necessitating use of additional media in future studies. To the best of our knowledge, this is the first report showing the potential effect of bacteria associated with host plant root exudate water on oviposition behavior of gravid Anopheles mosquitoes. Moreover, a review of the literature revealed that no previous study had implicated E. mori or A. aquatilis in the oviposition response of any mosquito vector.
The attraction elicited by odors of different bacterial species to ovipositing females could differ as a function of variations in the composition of VOCs they emit (29). In our study, the abundance of VOCs was observed to vary among the three bacterial isolates. For instance, the aldehydes nonanal and benzaldehyde were abundant in the volatile profiles of E. mori while cedrene was abundant in the volatiles of A. aquatilis. These two aldehydes have been shown to elicit antennal responses in gravid Anopheles mosquitoes (12, 14). Also, nonanal and decanal have been implicated in host seeking behavior of An. gambiae s.l (30, 31). and male swarming behavior (32). Additionally, the known Anopheles oviposition attractant dodecane and indole were emitted in higher amounts in Enterobacter sp. than in the volatile emissions of the other two species. Interestingly, these two compounds were identified by RFA as the most important in contributing to the attraction of the odors of bacterial isolates to oviposition. These and the additional compounds identified may require further investigation to ascertain their roles in mosquito oviposition behavior.
The bacteria sp. that influences mosquito oviposition behavior may be exploited for mosquito control. For instance, the attractive bacteria sp. can be utilized in the development of microbial based oviposition traps to target gravid mosquitoes. Alternatively, the less attractive bacteria species can be used to manipulate breeding sites to produce unattractive volatiles and thus prevent colonization of habitats by mosquitoes. Overall, the positive influence of microbes on the oviposition behavior of gravid mosquitoes agrees with previous findings (13, 33), although, our approach is unique and opens up a novel avenue for identifying lures that can compete with natural breeding sites.
As a limitation, we assayed bacteria singly and using concentration ranges that could differ from the natural root exudate. Generally, it is possible for a combination of bacteria to elicit a stronger oviposition response. Bacterial populations in the natural substrate could be more diverse than just a single species (13, 23, 29), necessitating evaluation of bacteria also in blends. In addition, the current study did not investigate the impact of the bacteria on mosquito life history traits but focused only on oviposition behavior. With respect to VOCs detection, some of the detected VOCs (e.g., 2,6, dimethyl-pyrazine, 1,8 cineole, phenylethyl alcohol, dodecane, hexadecane) did not overlap in the headspace of the bacteria and root exudate water. Perhaps in the root exudate water, only trace amounts of these volatiles were present beyond the detection limit of the GC-MS. Also, the difference can be attributed to the adsorbents employed in volatile trapping. Whereas plant root exudate volatiles were trapped using Super Q (24), SPME was utilized for bacteria headspace samples. Nevertheless, the study provides relevant baseline investigating the role of bacteria associated with host plant root exudate water in mediating mosquito oviposition behavior.
Conclusion
Our study provides a first report demonstrating the ability of culturable bacteria associated with P. hysterophorus root exudate to attract gravid An. gambiae mosquitoes. The bacterial isolates elicited differential attraction to gravid Ae. gambiae mosquito and varied in their emitted VOCs. The attractive isolates (E. mori and A. aquatilis) were associated with abundance of compounds known to attract gravid malaria vectors including nonanal, benzaldehyde and decanal. These VOCs can be exploited in semiochemical-based mosquito control strategies. Collectively, the current findings improve our knowledge of P. hysterophorus root exudate-mosquito-microbe interaction.
Data availability statement
The original contributions presented in the study are included in the article/supplementary material, further inquiries can be directed to the corresponding author/s.
Ethics statement
The manuscript presents research on insects that do not require ethical approval for their study.
Author contributions
TM: Conceptualization, Formal Analysis, Investigation, Methodology, Writing – original draft, Writing – review & editing. BT: Conceptualization, Funding acquisition, Project administration, Resources, Supervision, Writing – original draft, Writing – review & editing. DT: Conceptualization, Data curation, Formal Analysis, Software, Supervision, Writing – original draft, Writing – review & editing.
Funding
The author(s) declare financial support was received for the research, authorship, and/or publication of this article. This work was funded through THRiVE-2, DELTAS Africa grant number DEL-15-011 from Wellcome Trust grant # 107742/Z/15/Z and the UK government. Partial funding was also received from International Foundation for Science (IFS), grant number: I-1-F-6127-1. DT is supported by a Wellcome Trust International Intermediate Fellowship (222005/Z/20/Z). We gratefully acknowledge the financial support for this research by the following organizations and agencies: Swedish International Development Cooperation Agency (Sida), Swiss Agency for Development and Cooperation (SDC), Australian Centre for International Agricultural Research (ACIAR), Federal Democratic Republic of Ethiopia and the Government of the Republic of Kenya. The funders had no role in study design, data collection and analysis, decision to publish, or preparation of the manuscript.
Acknowledgments
The authors thank Mary Koki Paul for her assistance with bacteria culture and isolation, Robinson Kisero for assisting with DNA isolation, Onesmus Wanyama, Milcah Gitau and Paul Odondi for their technical assistance.
Conflict of interest
The authors declare that the research was conducted in the absence of any commercial or financial relationships that could be construed as a potential conflict of interest.
The author(s) declared that they were an editorial board member of Frontiers, at the time of submission. This had no impact on the peer review process and the final decision.
Publisher’s note
All claims expressed in this article are solely those of the authors and do not necessarily represent those of their affiliated organizations, or those of the publisher, the editors and the reviewers. Any product that may be evaluated in this article, or claim that may be made by its manufacturer, is not guaranteed or endorsed by the publisher.
Author disclaimer
The views expressed herein do not necessarily reflect the official opinion of the donors.
References
1. WHO. Fact sheet about Malaria (2022). Available online at: https://www.who.int/news-room/fact-sheets/detail/malaria.
2. Bhatt S, Weiss DJ, Cameron E, Bisanzio D, Mappin B, Dalrymple U, et al. The effect of malaria control on Plasmodium falciparum in Africa between 2000 and 2015. Nature. (2015) 526:207–11. doi: 10.1038/nature15535
3. World Malaria Report 2018. Geneva: World Health Organization. (2018). Licence: CC BY-NC-SA 3.0 IGO.
4. World Health Organization. Handbook for integrated vector management. Geneva: World Health Organization (2012).
5. Hemingway J. The role of vector control in stopping the transmission of malaria: threats and opportunities. Philos Trans R Soc Lond B Biol Sci. (2014) 369:20130431. doi: 10.1098/rstb.2013.0431
6. Milugo TK, Tchouassi DP, Kavishe RA, Dinglasan RR, Torto B. Naturally occurring compounds with larvicidal activity against malaria mosquitoes. Front Trop Dis. (2021) 2. doi: 10.3389/fitd.2021.718804
7. Wooding M, Naudé Y, Rohwer E, Bouwer M. Controlling mosquitoes with semiochemicals: a review. Parasit Vectors. (2020) 13:80. doi: 10.1186/s13071-020-3960-3
8. Nyasembe VO, Torto B. Volatile phytochemicals as mosquito semiochemicals. Phytochem Lett. (2014) 8:196–201. doi: 10.1016/j.phytol.2013.10.003
9. Navarro-Silva M, Marques F, Duque J. Review of semiochemicals that mediate the oviposition of mosquitoes: A possible sustainable tool for the control and monitoring of Culicidae. Rev Bras Entomol. (2009) 53:1–6. doi: 10.1590/S0085-56262009000100002
10. Torto B, Tchouassi DP. Chemical ecology and management of dengue vectors. Annu Rev Entomol. (2024) 69:1. doi: 10.1146/annurev-ento-020123-015755
11. Lindh JM, Okal MN, Herrera-Varela M, Borg-Karlson AK, Torto B, Lindsay SW, et al. Discovery of an oviposition attractant for gravid malaria vectors of the Anopheles Gambiae species complex. Malar. J. (2015) 14:119. doi: 10.1186/s12936-015-0636-0
12. Mweresa C, Mukabana R, van Loon J, Dicke M, Takken W. Use of semiochemicals for surveillance and control of hematophagous insects. Chemoecology. (2020) 30:277–86. doi: 10.1007/s00049-020-00317-1
13. Lindh JM, Nnaste AK, Knols BGJ, Faye I. Oviposition Responses of Anopheles Gambiae s.s. (Diptera: Culicidae) and identification of volatiles from bacteria-containing solutions. J Med Entomol. (2008) 45:11. doi: 10.1093/jmedent/45.6.1039
14. Wondwosen B, Birgersson G, Seyoum E, Tekie H, Torto B, Fillinger U, et al. Rice volatiles lure gravid malaria mosquitoes, Anopheles arabiensis. Sci Rep. (2016) 6:1–8. doi: 10.1038/srep37930
15. Wondwosen B, Birgersson G, Tekie H, Torto B, Ignell R, Hill SR. Sweet attraction: sugarcane pollen-associated volatiles attract gravid Anopheles arabiensis. Malar. J. (2018) 17:90. doi: 10.1186/s12936-018-2245-1
16. Nyasembe VO, Teal PE, Mukabana WR, Tumlinson JH, Torto B. Behavioral response of the malaria vector Anopheles Gambiae to host plant volatiles and synthetic blends. Parasit Vectors. (2012) 5:234. doi: 10.1186/1756-3305-5-234
17. Eneh L, Saijo H, Borg-Karlson AK, Lindh J, Rajarao G. Cedrol, a malaria mosquito oviposition attractant is produced by fungi isolated from rhizomes of the grass Cyperus rotundus. Malar. J. (2016) 15:478. doi: 10.1186/s12936-016-1536-7
18. Torres estrada J, Meza-Alvarez R, Cruz L, Rodríguez M, Jiménez Arredondo J. Attraction of gravid Anopheles pseudopunctipennis females to oviposition substrates by Spirogyra majuscula (Zygnematales : Zygnmataceae) algae under laboratory conditions. J Am Mosq Control Assoc. (2007) 23:18–23. doi: 10.2987/8756-971X(2007)23[18:AOGAPF]2.0.CO;2.
19. Sumba LA, Guda TO, Deng AL, Hassanali A, Beier JC, Knols BGJ. Mediation of oviposition site selection in the African malaria mosquito Anopheles Gambiae (Diptera: Culicidae) by semiochemicals of microbial origin. Int J Trop Insect Sci. (2004) 24:260–5. doi: 10.1079/IJT200433
20. Arbaoui A, Chua T. Bacteria as a source of oviposition attractant for Aedes aEgypti mosquitoes. Trop Biomed. (2014) 31:134–42.
21. Melo N, Wolff GH, Costa-da-Silva AL, Arribas R, Triana MF, Gugger M, et al. Geosmin attracts Aedes aEgypti mosquitoes to oviposition sites. Curr Biol. (2020) 30:127–34. doi: 10.1016/j.cub.2019.11.002
22. Ponnusamy L, Schal C, Wesson DM, Arellano C, Apperson CS. Oviposition responses of Aedes mosquitoes to bacterial isolates from attractive bamboo infusions. Parasit Vectors. (2015) 8:486. doi: 10.1186/s13071-015-1068-y
23. Trexler JD, Apperson CS, Zurek L, Gemeno C, Schal C, Kaufman M, et al. Role of bacteria in mediating the oviposition responses of Aedes albopictus (Diptera: Culicidae). J Med Entomol. (2003) 40:841–8. doi: 10.1603/0022-2585-40.6.841
24. Milugo TK, Tchouassi DP, Kavishe RA, Dinglasan RR, Torto B. Root exudate chemical cues of an invasive plant modulate oviposition behavior and survivorship of a malaria mosquito vector. Sci Rep. (2021) 11:14785. doi: 10.1038/s41598-021-94043-5
25. Kihika R, Murungi LK, Coyne D, Ng’ang’a M, Hassanali A, Teal PEA, et al. Parasitic nematode Meloidogyne incognita interactions with different Capsicum annum cultivars reveal the chemical constituents modulating root herbivory. Sci Rep. (2017) 7:2903. doi: 10.1038/s41598-017-02379-8
26. Soriano-Lerma A, Pérez-Carrasco V, Sánchez-Marañón M, Ortiz-González M, Sánchez-Martín V, Gijón J, et al. Influence of 16S rRNA target region on the outcome of microbiome studies in soil and saliva samples. Sci Rep. (2020) 10:13637. doi: 10.1038/s41598-020-70141-8
27. Tamura K, Stecher G, Kumar S. MEGA11: molecular evolutionary genetics analysis version 11. Mol Biol Evol. (2021) 38:3022–7. doi: 10.1093/molbev/msab120
28. Ponnusamy L, Xu N, Nojima S, Wesson DM, Schal C, Apperson CS. Identification of bacteria and bacteria-associated chemical cues that mediate oviposition site preferences by Aedes aEgypti. Proc Natl Acad Sci USA. (2008) 105:9262–7. doi: 10.1073/pnas.0802505105
29. Michalet S, Minard G, Chevalier W, Meiffren G, Saucereau Y, Tran Van A, et al. Identification of human skin bacteria attractive to the Asian Tiger mosquito. Environ Microbiol. (2019) 21:4662–74. doi: 10.1111/1462-2920.14793
30. Nyasembe VO, Tchouassi DP, Kirwa HK, Foster WA, Teal PEA, Borgemeister C, et al. Development and assessment of plant-based synthetic odor baits for surveillance and control of malaria vectors. PloS One. (2014) 9:e89818. doi: 10.1371/journal.pone.0089818
31. Tchouassi DP, Sang R, Sole CL, Bastos ADS, Teal PEA, Borgemeister C, et al. Common host-derived chemicals increase catches of disease-transmitting mosquitoes and can improve early warning systems for Rift Valley fever virus. PloS Negl Trop Dis. (2013) 7:e2007. doi: 10.1371/journal.pntd.0002007
32. Mozūraitis R, Hajkazemian M, Zawada JW, Szymczak J, Pålsson K, Sekar V, et al. Male swarming aggregation pheromones increase female attraction and mating success among multiple African malaria vector mosquito species. Nat Ecol Evol. (2020) 4:1395–401. doi: 10.1038/s41559-020-1264-9
Keywords: bacteria, oviposition, malaria vector, Parthenium hysterophorus, invasive plants, volatile organic compounds
Citation: Milugo TK, Torto B and Tchouassi DP (2024) Bacteria associated with Parthenium hysterophorus root exudate influence olfactory oviposition responses of Anopheles gambiae. Front. Trop. Dis 5:1359774. doi: 10.3389/fitd.2024.1359774
Received: 21 December 2023; Accepted: 29 February 2024;
Published: 18 March 2024.
Edited by:
Paul O. Mireji, Kenya Agricultural and Livestock Research Organization, KenyaReviewed by:
Rajnikant Dixit, National Institute of Malaria Research (ICMR), IndiaWolfgang Richard Mukabana, University of Nairobi, Kenya
Copyright © 2024 Milugo, Torto and Tchouassi. This is an open-access article distributed under the terms of the Creative Commons Attribution License (CC BY). The use, distribution or reproduction in other forums is permitted, provided the original author(s) and the copyright owner(s) are credited and that the original publication in this journal is cited, in accordance with accepted academic practice. No use, distribution or reproduction is permitted which does not comply with these terms.
*Correspondence: David P. Tchouassi, ZHRjaG91YXNzaUBpY2lwZS5vcmc=