- 1Agroecohealth Unit, International Institute of Tropical Agriculture (IITA-Benin), Cotonou, Benin
- 2Faculty of Science and Technologies, University of Abomey-Calavi, Cotonou, Benin
- 3Biorisk Management Facility (BIMAF), International Institute of Tropical Agriculture (IITA-Benin), Cotonou, Benin
- 4Ecole de Gestion et de Production Végétale et Semencière (EGPVS), Université Nationale d’Agriculture (UNA-Bénin), Kétou, Benin
- 5National University of Sciences, Technologies, Engineering and Mathematics, Ecole Normale Supérieure de Natitingou, Natitingou, Benin
- 6Centre for Research in Infectious Diseases (CRID), Yaoundé, Cameroon
- 7Animal Health, International Livestock Research Institute (ILRI), Bamako, Mali
- 8Vector Biology, Liverpool School of Tropical Medicine, Liverpool, United Kingdom
Background: Neonicotinoids are gradually being introduced into malaria vector control due to their mode of action that targets insect nicotinic acetylcholine receptors (nAChRs). However, prior to scaling up novel insecticides, it is important to assess the susceptibility of the mosquito population to this molecule. The present study aimed to determine the susceptibility level of mosquitos collected from agricultural and urban sites against neonicotinoids in Benin.
Material and methods: An. gambiae sl. larvae were collected from three urban sites and two agricultural sites in southern Benin. An. gambiae sl. larvae collected from three urban and two agricultural sites in southern Benin were exposed to neonicotinoids (clothianidin, acetamiprid, and imidacloprid) for 1 hour, and mortality rates were monitored from 24h to day 7 post-exposure. After molecular identification of the samples, Taqman assays were used to genotype key pyrethroid-resistant markers, and qPCRs were performed to establish the level of expression of detoxification enzymes in resistant mosquitoes.
Results: Overall, resistance to acetamiprid and imidacloprid was observed in the five study sites surveyed whereas full susceptibility was recorded to clothianidin. Anopheles coluzzii was the only species found in all sites. TaqMan genotyping showed a very low presence of key pyrethroid-resistant markers suggesting that metabolic resistance is more implicated in neonicotinoid resistance in Anopheles. coluzzii populations from southern Benin. qPCR performed revealed overexpression of P450 genes (CYP6M2, CYP6P4, CYP6Z1, CYP6Z2, and CYP4G16) and sensory proteins (Sap1, Sap2, and Sap3).
Conclusion: This first assessment of neonicotinoid resistance in Benin showed evidence of neonicotinoid resistance in both agricultural and urban areas. However, full susceptibility to clothianidin was observed, showing that this insecticide could be used to enhance the efficacy of control tools. In addition, there is a need of an integrated approach, including coordinated education on pesticide use, to improve the overall management of insecticide resistance in both agricultural and public health sectors.
Background
Despite widespread strategies to reduce the incidence of malaria in Africa, there was an increase in malaria cases from 227 million in 2019 to 247 million in 2022 with most of this increase coming from countries in the World Health Organization (WHO) African Region (1). In Benin, mosquitoes from An. gambiae s.l. and An. funestus group are the main vectors responsible of malaria transmission in several regions of the country (2–5). Control of malaria relies largely on the use of insecticides to prevent mosquitoes from transmitting the malaria parasite to humans (6, 7). Four main classes of insecticides are recommended by the World Health Organization Pesticide Evaluation Scheme (WHOPES) for use in public health programs: organochlorines, organophosphates, pyrethroids, and carbamates (8, 9). These insecticides are used to saturate bednets (LLINs) or for indoor residual spraying (IRS) (8, 9). Pyrethroids are the main insecticides used because of their relative safety for humans, rapid knock-down effects, and cost effectiveness (10). Unfortunately, resistance to pyrethroids is widespread in African malaria vectors and varying levels of resistance to the other insecticides used for vector control (carbamates, organophosphates, and organochlorines) have been recorded. Insecticides to which vectors are resistant include pyrethroids such as permethrin, deltamethrin, and alphacypermethrin which are commonly incorporated into mosquito nets (11–13) (Corbel et al., 2007; Ngufor et al., 2015, Sovi et al., 2020). In southern Benin, many studies have reported high resistance in populations of An. gambiae s.l., causing a threat to malaria control (11–18). Malaria vector control relies on the mass distribution of LLINs and IRS operations. From 2011 to 2016, an IRS program was implemented with funding from the US President’s Malaria Initiative (PMI), in North Benin and targeted all houses in the Atacora region. During the 6 years of the IRS campaign, two insecticides with different modes of action were used in rotation: bendiocarb (a carbamate) and pirimiphos methyl (an organophosphate), due to the emergence and expansion of resistance of Anopheles vectors to insecticides, especially pyrethroids (19–21). Six years post-IRS implementation (2011–2016), a significant reduction in malaria transmission in the Atacora region was noted (2, 22). In 2017, the IRS program was moved to two other regions (Donga and Alibori) in the North, with hopes that the gains would relatively be sustained because of the seasonality of malaria transmission. However, some studies have already reported resistance to carbamate and organophosphates in malaria vectors (23). This stresses the need for a judicious application of non-pyrethroid insecticides to delay the onset of resistance and preserve efficacy of malaria control tools. Therefore, it is important that regular monitoring is carried out to detect the early occurrence of resistance in malaria vectors. This will better guide National Malaria Control Programs (NMCP) in their choice of insecticide-based tools. In regard to this challenge, there are urgent needs for new tools for vector control to improve the management of malaria transmission. Thus, the WHO has recently recommended the introduction of neonicotinoids, a class of neuro-active insecticides chemically similar to nicotine. Neonicotinoid pesticides were developed in 1980s, were first registered for use in agriculture in the 1990s, and are now the most widely used insecticide in the world, replacing organophosphate and carbamate insecticides (24) with large-scale applications ranging from plant protection, veterinary application, and biocides for invertebrate pest control in fish farming (25). Neonicotinoid insecticides are of great interest due to their different mode of action, preventing cross-resistance with pyrethroids. They selectively bind and interact with the insect nicotinic acetylcholine receptor site (nAChRs), affecting the synapses in the central nervous system (26). Neonicotinoids cause nervous stimulation at low concentrations, but receptor blockage, paralysis, and death at higher concentrations. Neonicotinoids have a high target specificity to insects, a relatively low risk for nontarget mammalian species and the environment, and a versatility in application methods (27). At low doses, neonicotinoids cause decreased activity and tremors, mydriasis or miosis, and incoordination; and at higher doses, they cause hypothermia, staggering gait, salivation, trembling, and spasms. At lethal doses, death is observed within 4 h (28). Neonicotinoids bind more strongly to insect nAChRs than to those of vertebrates, so they are selectively more toxic to insects (29, 30). Due to their low mammalian toxicity (including other non-target species), these insecticides are widely used in agricultural pest management (31). The neonicotinoid class of insecticides includes acetamiprid, clothianidin, dinotefuran, imidacloprid, nitenpyram, thiacloprid, and thiamethoxam, among which clothianidin is mainly used for vector control. Clothianidin was developed alone under the name of SumiShield by Sumitomo and in combination with deltamethrin by Bayer under the name of Fludora Fusion for monitoring insecticide resistance in malaria vectors. A study in Benin showed that the mixture of clothianidin and deltamethrin induced high mortality and has demonstrated a residual efficacy of 8 to 9 months on pyrethroid-resistant An. gambiae sl. (32). In view of the performance of this insecticide, clothianidin was recently recommended by the WHO for indoor residual spraying (33). However, with the increase of insecticide resistance due to agricultural practices, it is important to monitor the efficacy of insecticides in order to improve malaria control strategies. Three neonicotinoids were assessed in this study: clothianidin, acetamiprid, and imidacloprid. We evaluated the resistance level of An. gambiae sl. to neonicotinoids in different agriculture and urban areas to generate data that could serve as a basis for discussion of novel neonicotinoid-based vector control strategies.
Materials and methods
Study sites
This study was conducted in three urban sites, namely, Akogbato (N 06°21.694’, E 002°21.552’), Fifadji (N 06°23.691’, E 002°23.992’), and Ladji (N 06°23.357’, E 002°25.791’) and two agricultural sites: Houeyiho (6°22′ 21″N, 2° 23′ 38″E) and Sèdjè-Denou (6°32’ 87″N, 2°13′ 26″E). The three urban sites are located in Cotonou at least 10 kilometers from each other. Houeyiho is a vegetable farm also located in Cotonou while Sedjè-Denou is a rice farm in Abomey-Calavi (situated 15km from Cotonou) as no rice field could be found in Cotonou near to the other selected sites (Figure 1). Previous studies reported the use of insecticides and fertilizers in rice and vegetable farms (34) whereas no agricultural activities were observed in urban sites. Urban and agricultural sites were chosen in this study to compare the resistance status at the two sites in order to establish whether agriculture or urbanization alone or combined can select for resistance in malaria vectors.
Mosquito collection
Immature stage mosquitos (larvae and pupae) were collected at the study sites using dipping methods (35) from July 2021 to May 2022. Larvae sampled from each site were reared separately to the adult stage for molecular identification and insecticide susceptibility testing. Mosquito larvae were reared under standard insectary conditions (25–27°C and 70–75% relative humidity) and fed daily with powdered Tetramin® baby fish food (Charterhouse Aquatics, London, UK) until their progression to the adult mosquito stage (34). Adult mosquitoes were identified morphologically and An. gambiae sl. were sorted using the identification keys of Gillies and De Meillon (36) and Gillies and Coetzee (37).
Molecular analysis
Species identification
The genomic DNA was extracted from a subset of mosquitoes randomly selected from alive and dead mosquitoes in each of the collection sites. In total, 75 mosquitoes each from Houeyiho and Fifadji, 60 from Ladji, and 100 each from Akogbato and Sèdjè were analyzed using the Livak method (38) and the members of the An. gambiae complex were identified by polymerase chain reaction (PCR) (39, 40). Furthermore, DNA extracted from specimens of Anopheles gambiae sensu stricto were subjected to PCR for identification of ‘Anopheles coluzzii’ and ‘Anopheles gambiae’ (26).
CDC bottles bioassays
The bioassays were performed using Center for Disease Control (CDC) bottles with discrimination concentrations of 75ug and 200ug for acetamiprid and imidacloprid respectively as described by Chouaïbou et al. (41). The bottles used for the bioassay need to be coated inside with a diagnostic dose of the insecticide under evaluation. Stock solutions were prepared from technical-grade insecticide. In this study, the technical grade insecticide was solid with a purity between 97–98%. Therefore, calculations were made based on the purity of the insecticide and a stock solution was prepared. One ml of the stock solution contained the desired amount of insecticide to be added to the bottle. The treatment of bottles was conducted following the CDC guidelines (42, 43). Technical grade neonicotinoids were purchased from Sigma with a purity between 97–98%. The solutions (insecticides mixed with solvents) were conserved at 4°C in the dark for 24 h before use to maximize the solubility (44). The bottles were coated with 1ml of each insecticide under evaluation. The bottles were protected from light using aluminum foil and allowed to dry overnight for complete evaporation of the solvent before use. The different concentrations were first tested on the susceptible Anopheles gambiae Kisumu for quality control of the insecticide solution and 100% mortality was obtained. For each bioassay with field mosquitoes, we used four treated bottles coated with the target insecticide and two control bottles (one coated with acetone only and the second without any substance). The susceptibility tests were carried out as follows: 3–4 replicates of 20 to 25 (3 to 5-day-old) females were transferred from mosquito cages and gently released into test bottles where they were exposed to the active ingredient or into control bottles. The knockdown effect was recorded during 1 hour of exposure and the mosquitoes were transferred into a paper cup and provided with a 10% sugar solution. Mortality was monitored every day for 7 consecutive days under standard laboratory conditions.
Clothianidin tends to crystallize when diluted in acetone alone and the uptake of the active ingredient by the mosquitos becomes low. Therefore, Mero carrier was used together with acetone to prevent crystallization and is manufactured by Bayer Crop Science. Technical grade clothianidin dissolved in a mixture of Mero® (81% Rapeseed oil methyl ester) and acetone was prepared. CDC bottles are treated with the diagnostic dose of 4 μg/bottle of clothianidin as recommended by the WHO. Serial dilutions (1/2, 1/4, 1/8) were made from the diagnostic dose (4ug) and were also tested. One ml of the clothianidin solution (4μg/bottle) was transferred using a pipette and coated a 250ml glass bottle. Two control bottles were also used, one treated with a solution of acetone/Mero mixture and the second without no substance. The exposure time is 60 minutes. Knock-down was recorded at 30 minutes and at 60 minutes. After the 1h exposure period, the mosquitoes were transferred into paper cups and provided with lightly moistened cotton wool containing a 10% sugar solution. Mortality was recorded 24h after exposure.
Genotyping assessment of target-site mutations in field An. gambiae sl.
A TaqMan-based approach was conducted for the genotyping of selected known target-site resistance markers in An. gambiae sl. to determine their frequencies in the mosquito populations. For this analysis, dead and alive mosquitoes were randomly selected from mosquitoes exposed to neonicotinoids in general (acetamiprid, imidacloprid, and clothianidin). The kdr mutations L1014F, L1014S and N1575Y (45), as well as the G119S-Ace1 mutation (46), were then screened in the An. gambiae sl. mosquitoes as previously described using the TaqMan PCR assay. Two fluorophore-labeled specific TaqMan probes were used, FAM to detect the homozygous resistant genotype and HEX to detect the homozygous susceptible genotype, while both FAM and HEX were used to detect the heterozygous genotype. A negative control (water) and positive controls (known as FAM, HEX, and both) were also used in a 10 µl volume that also contained SensiMix (Applied Biosystems, California, USA). The endpoint fluorescence was evaluated using Agilent MXPro software and the relationship between the frequency of the resistant alleles and the insecticide-resistant phenotypes was assessed (47). The total volume of PCR mix was 10ul containing 5μl of SensiMix DNA kit (catalog: SM2–717104), 0.125μl of each probe, 3.875 μl of sigma water, and 1 μl of genomic DNA as described by Tchouakui et al. (48).
Transcriptional profile of metabolic resistant genes in clothianidin-resistant mosquitoes
The expression level of 13 resistant candidate genes previously reported to be associated with pyrethroid resistance in An. gambiae sl. (CYP6M2, CYP6K1, CYP6Z1, CYP6Z2, CYP4G16, CYP6P1, CYP6P2, CYP6P3, GSTe2, GSTD3, Sap1, Sap2, Sap3) were assessed by a quantitative reverse transcription PCR (qRT-PCR) for one urban site (Fifadji) and one agricultural site (Sèdjè) where the highest resistance level was recorded. As described by Tepa et al. (49), three biological replicates of surviving mosquitoes after exposure to clothianidin (survivors from diluted diagnostic dose) and those unexposed were compared to the susceptible strain An. gambiae Kisumu with two housekeeping genes: Elongation factor (AGAP000883) and Ribosomal Protein S7 (AGAP010592). Total RNA was extracted from 3 batches of 10 mosquitoes each and similarly from the susceptible laboratory strain Kisumu, using the Arcturus PicoPure RNA isolation kit (Life Technologies, Carlsbad, CA, USA), according to the manufacturer’s instructions. cDNA (complementary Deoxyribonucleic acid) was synthesized from the purified RNA by reverse transcriptase-PCR using the SuperScript III (Invitrogen, Waltham, MA, USA) and the oligo-dT207 of 19 and RNAse H (New England Biolabs, Ipswich, MA, USA) kit in a total reaction volume of 20 μL. Amplification was performed in an Agilent Mx3005 qRT-PCR thermocycler (Santa Clara, CA, USA) with the following conditions: 95°C for 3 min followed by 40 cycles of 10 s at 95°C and 10 s at 60°C; 1 min at 95°C; 30 s at 55°C and 30 s at 95°C. Samples were amplified in three technical replicates, using three biological replicates for gene expression analysis for each population. MxPRO software (Santa Clara, CA, USA) was used for the calculation of the Ct values for each reaction. Standard curves of assessed genes were established. As the efficiency was different from 100%, the Ct value was adjusted according to efficiency as previously done by Riveron et al. (50). The relative expression was calculated individually according to the 2-DDCT method (51) and plotted with R studio software. Genes were considered as relatively overexpressed to An. gambiae Kisumu when their relative fold changes (FCs) were more than a 2 fold-change (49).
Data analysis
Following the WHO guidelines on insecticide susceptibility, mosquito populations were considered susceptible if mortality at day 7 or 24h post-exposure was ≥98% and resistant if mortality was less than 90%. Mortality rates between 90% and 97% indicate the presence of resistant genes. Fisher’s exact test was used to establish statistical significance between mortality rates. Student’s t test was performed to determine whether the difference observed in the expression level after RT-qPCR was significant. The mean of the three replicates of each sample after normalization with housekeeping genes was used for this analysis.
Results
Molecular identification of mosquitoes tested
PCR assays revealed that all the mosquitoes tested from Houeyiho (n=75), Fifadji (n=75), Ladji (n=60), Akogbato (n=100), and Sèdjè (n=100) were all An. coluzzii (Figure 2).
Susceptibility profile to acetamiprid
Bioassays using acetamiprid revealed resistance to this insecticide in the five study sites surveyed. The highest mortality rate (49%) was observed in Ladji, (urban site) (P<0.0001). There was no statistically significant difference in mortality among sites (P=0.6914) except between Ladji (urban site) and the other study sites. The results showed that mosquitoes from both urban and agricultural sites were resistant to acetamiprid (Figure 3).
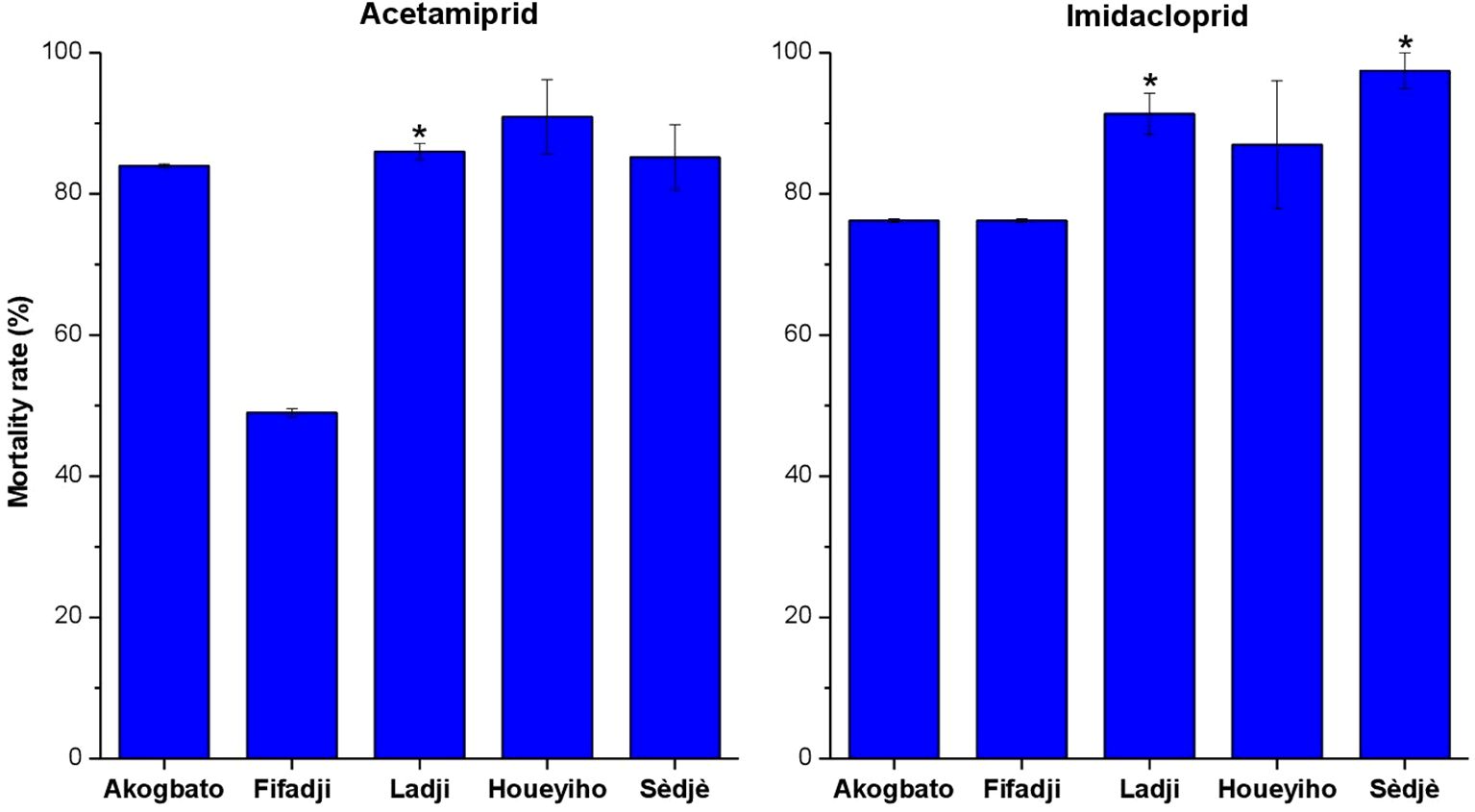
Figure 3 Susceptibility profile of An. Coluzzii to acetamiprid and imidacloprid in urban and agrilcultural sites in Benin. (*) indicates significance difference between treatments.
Susceptibility profile to imidacloprid
Resistance was observed in An. coluzzii population from Ladji (urban site) and suspected in Sèdjè (rice field) with mortality rates of 76.23% and 97.44% respectively. Mortality rates ranging from 76.23% to 86.96% were recorded in Fifadji, Akogbato (urban site), and Houeyiho (agricultural site). A significant difference was observed between mortality rates observed in Ladji (P=0.0035) and Sèdjè (P<0.0001) and the other study sites. Overall, resistance to imidacloprid was low in all surveyed sites compared to acetamiprid (Figure 3).
Susceptibility profile to clothianidin
Full susceptibility was observed for all An. coluzzii populations with a mortality rate of 100% when exposed to clothianidin dissolved in acetone + Mero at a concentration of 4 µg/ml as recommended by the WHO with a gradual decrease in mortality proportionally to the different dilutions made from the diagnostic dose (Figure 4). No mortality was observed in the control bottles. Results of bioassays are summarized in Tables 1A, B.
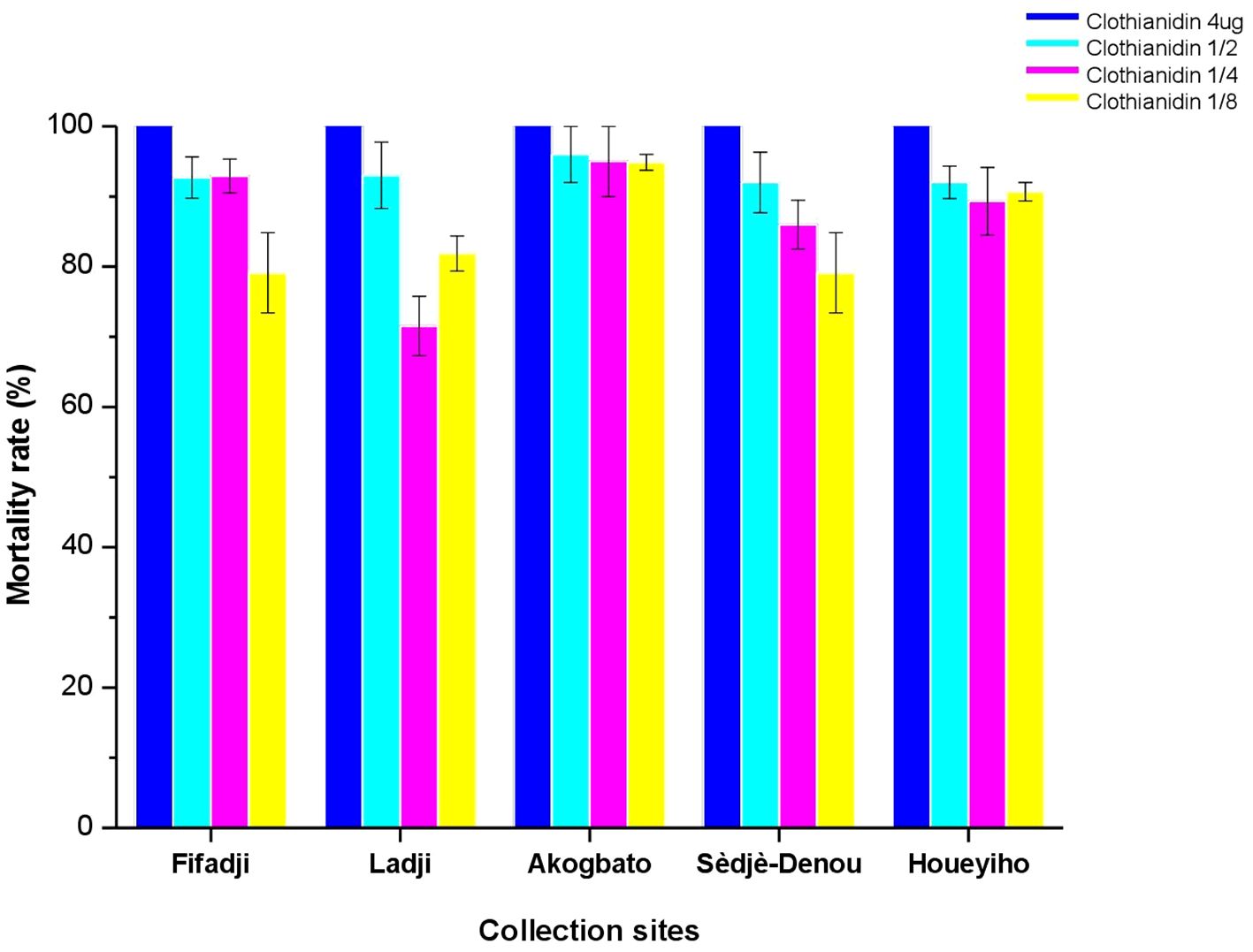
Figure 4 Assessment of clothianidin resistance using acetone and MERO as solvent. Percentage mortality (24 h) of field collected mosquitoes after exposure to each of the four concentrations of clothianidin (with acetone + MERO assolvent).
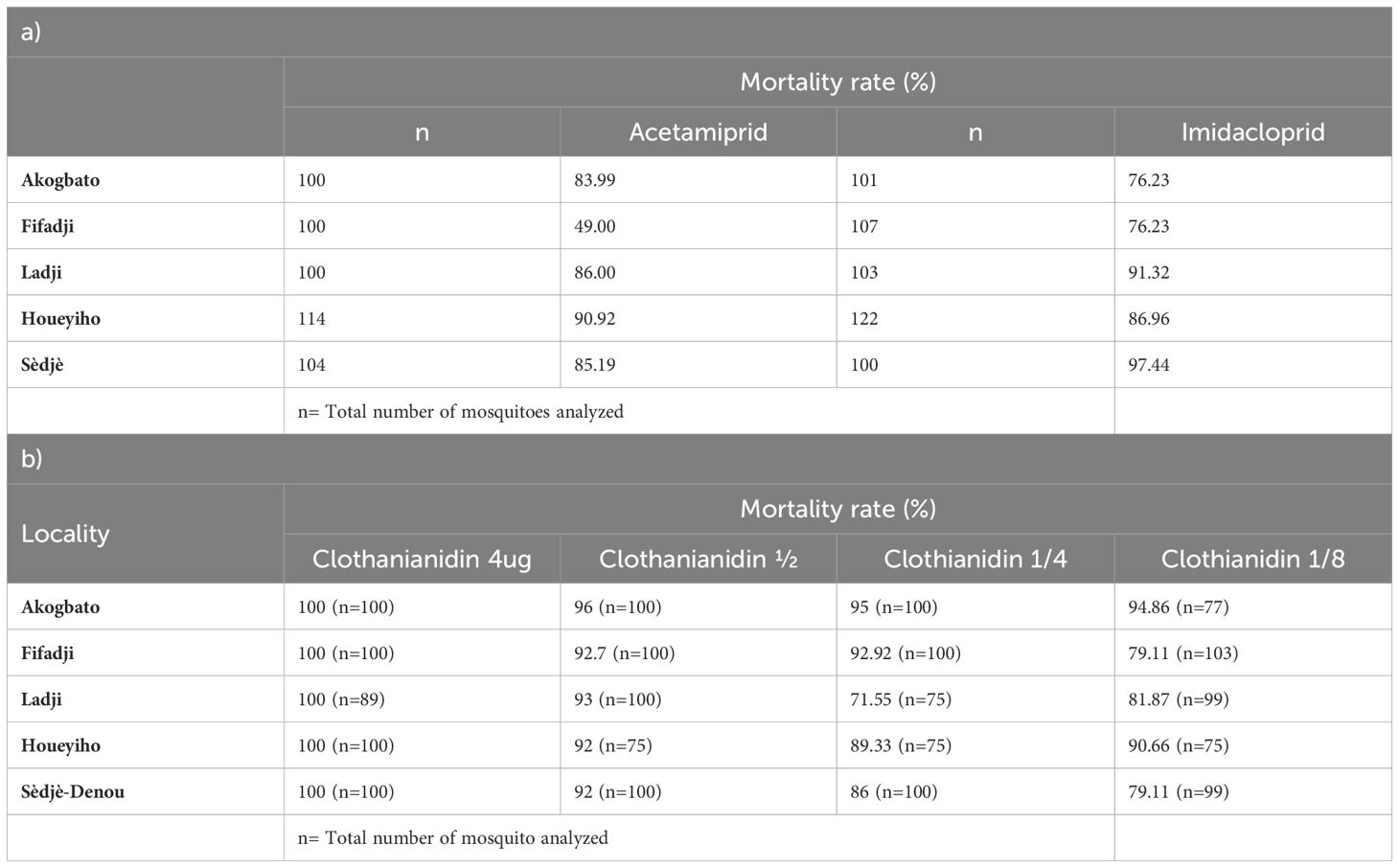
Table 1 A and B: Susceptibility to neonicotinoids profile of An. gambiae sl. collected at surveyed sites.
Genotyping of target site mutations
The frequencies of target-site mutations (Kdr west L1014F, Kdr East L1014S, N1575Y, and Ace1 G119S) were monitored in An. coluzzii populations exposed to neonicotinoids. The allelic frequency of the Kdr west L1014F mutation remain was almost the same in dead and alive mosquitoes. The other mutations (Kdr East L1014S, N1575Y, and Ace1 G119S) were very low or absent. These findings suggest that metabolic mechanisms may be more involved in the observed resistance to neonicotinoids (Table 2).
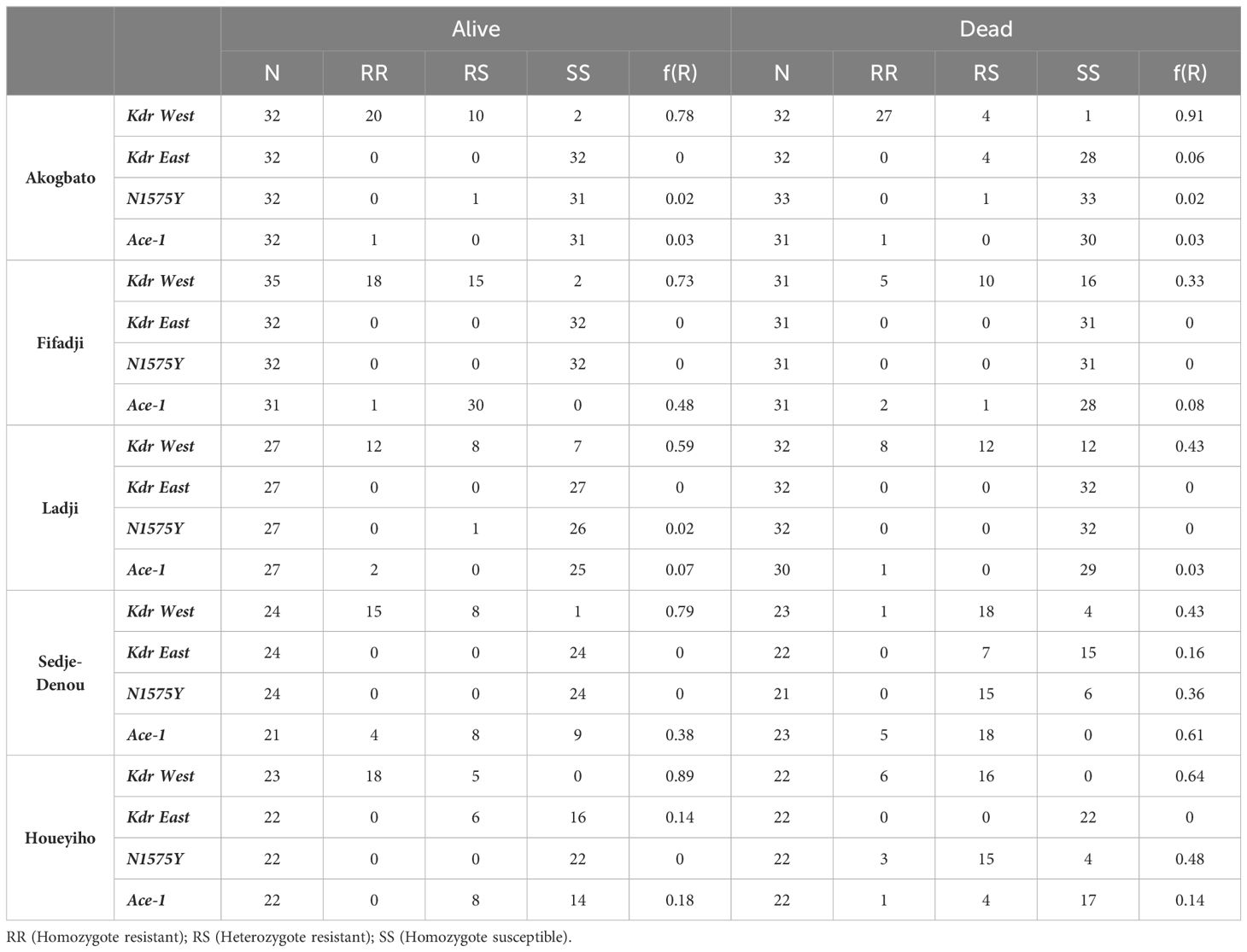
Table 2 Allelic frequencies of Kdr west L1014F, Kdr East L1014S, N1575Y, and Ace1 G119S in dead and alive mosquitoes exposed to neonicotinoids.
Metabolic gene expression
The expression pattern of known resistance-associated genes was evaluated in resistant samples compared to the unexposed control and susceptible An. gambiae kisumu using the RT-qPCR. This analysis was performed for some selected metabolic genes that were previously associated with pyrethroid resistance in An. gambiae sl. mosquitoes. The results from this experiment showed significant overexpression of three sensory appendage protein encoding genes with the following FC, sap1 (FC=9.45, P<0.0001); sap2 (5.61, P=0.025), and sap3 (4.58, P=0.0001), as well as two cytochromes P450, CYP6M2 (6.89, P<0.0001) and CYP4G16 (2.59 P<0,0001), in resistant mosquitoes compared to unexposed mosquitoes at the Sèdjè rice field. At the urban site Fifadji we also observed significant overexpression of sensory protein sap2 (14.60, P=0.006), and two cytochromes P450, CYP6Z1 (19.51, P=0,01) and CYP6Z2 (10.53, P=0,001) in resistant mosquitoes compared to the unexposed (Figures 5, 6). At Fifadji, there was overexpression of Sap3, Sap1 and Cyp6M2 with no significant difference between unexposed and resistant mosquitoes. A similar observation was recorded at Sèdjè with GSTe2, GSTD3, CYP6P1, CYP6Z1, and CYP6Z2. Overall, P450 genes are more overexpressed at both agricultural and urban sites suggesting that the mechanisms underlying neonicotinoid resistance remain the same everywhere. However, more experiments should be carried out through analyses tof more detoxification genes at many sites to confirm this observation.
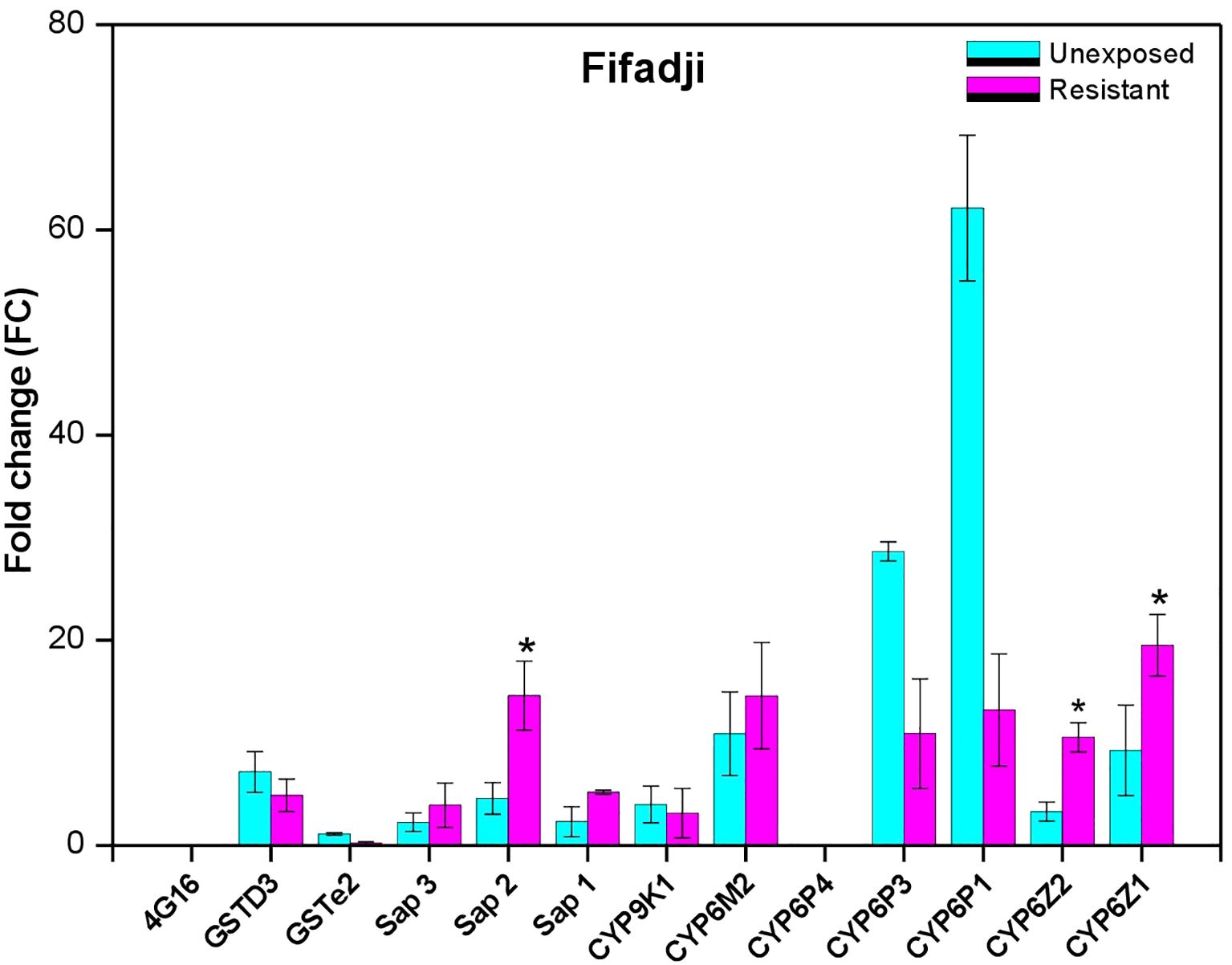
Figure 5 Differential expression by quantitative reverse-transcription polymerase chain reaction of the major insecticide resistance genes in An. gambiae sl. From Fifadji compared to susceptible An. gambiae Kisumu. (*) indicates significant overexpression of the enzyme.
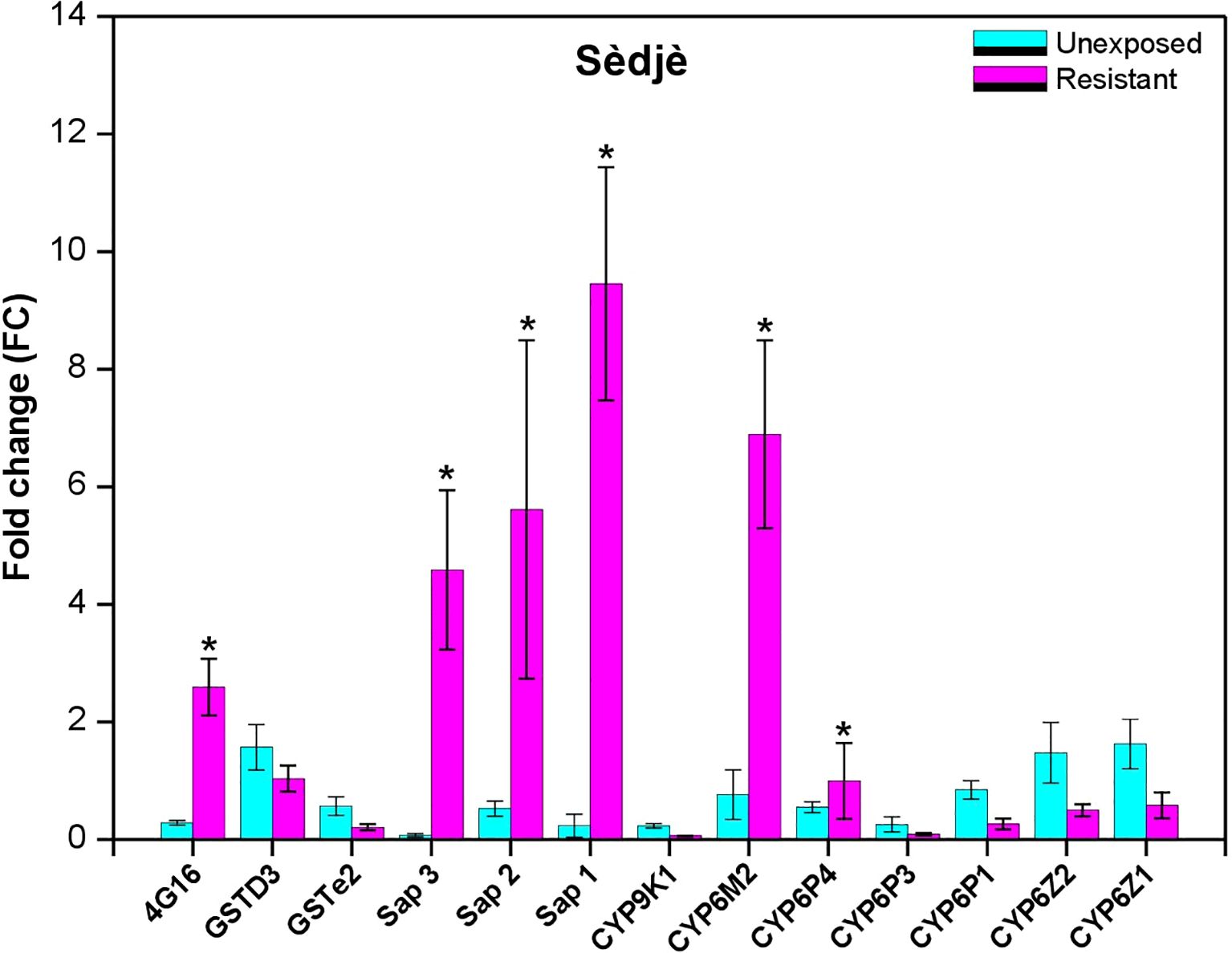
Figure 6 Differential expression by quantitative reverse-transcription polymerase chain reaction of the major insecticide resistance genes in An. gambiae sl. From Sèdjè comapared to susceptible An. gambiae Kisumu. (*) indicates significant overexpression of the enzyme.
Discussion
Prior to scaling up novel insecticides, it is vital to assess if their use in agriculture has already selected mosquito populations with reduced susceptibility in some areas. The present study is the first that explores the resistance to neonicotinoids in the main malaria vector An. coluzzii in Benin and raised the importance of the impact of insecticides used in agriculture on the development of resistance in insects of public health interest.
The susceptibility tests performed in this study confirmed the slower-acting effect of neonicotinoids compared to neurotoxic insecticides such as pyrethroids (47, 52). Indeed, pyrethroids are known to induce rapid mortality within 24 hours whereas acetamiprid/imidacloprid gradually induced mortality which increases over days and final mortality was recorded 7 days post-exposure (53). However, specifically, a new protocol was set-up by the WHO for clothianidin and mortality can be recorded 24h post-exposure. Clothianidin tends to crystallize if used with acetone alone and the uptake of the active ingredient by the mosquito becomes low, causing biases in the mortality rate. Therefore, a Mero carrier was used together with acetone to prevent crystallization. Ongoing activities are being performed to optimize the protocol of the other neonicotinoids (acetamiprid and imidacloprid). Hence, the difference observed in the susceptibility pattern to the three neonicotinoids tested was mainly due to the solvents used, leading to a possibly overestimated resistance (48). This situation makes monitoring resistance to this insecticide class very challenging. Full susceptibility was recorded with clothianidin at all surveyed sites. This observation is in line with results obtained by Chouaibou et al. (41) and Tchouakui et al. (48) confirming that Mero displays strong efficacy with clothianidin whereas ethanol or acetone as a solvent over-estimate the level of resistance in mosquitos due to crystallization. A recent study conducted by Ekra et al. (2024) (54) in three localities in Côte d’Ivoire showed a higher lethal effect of clothianidin on An. gambiae populations. This molecule could support a resistance management plan by being used in an insecticide rotation strategy for vector control interventions and in new types of net incorporating clothianidin. Clothianidin 50 WG was introduced for public health use in Benin in 2021 as part of large-scale community-based IRS campaigns. However, an integrated vector control framework and careful monitoring are important for managing insecticide resistance in malaria vectors. The results revealed resistance to neonicotinoids (acetamiprid and imidacloprid) at all the surveyed sites (urban and agricultural). This observation showed widespread neonicotinoid resistance in both urban and agricultural sites. Resistance against acetamiprid and imidacloprid was also recorded in An. gambiae sl. from Cameroon and Côte d’Ivoire (41, 48) raising the importance of an integrated approach in the control of malaria vectors. The spread of resistance could be due to rapid urbanization and extensive agriculture and results from this study showed the impact of agriculture on public health. Neonicotinoids especially acetamiprid and imidacloprid have never been involved in any vector control strategy in Benin so the resistance observed in Anopheles gambiae sl. vectors to these products could only be attributed to their use in agriculture. The association between the agricultural usage of pesticides and the development of insecticide resistance in Anopheles mosquitoes is widely documented (55–57). The overuse of pesticides in agricultural areas has led to Anopheles mosquito exposure to a diversity of xenobiotic compounds over the last few decades (58). Several studies have hypothesized that pesticide residues contaminate mosquito breeding sites and contribute to resistance selection to insecticides in mosquito populations (34, 58). In addition, neonicotinoids are highly soluble in water and can persist for months in aerobic soils, therefore contaminating mosquito breeding sites following agricultural use (59). An. coluzzii collected from urbanized areas were also resistant to neonicotinoids. These results suggest that others factors such as pollution and xenobiotics present in breeding sites may contribute to the selection of neonicotinoid-resistant vector populations. Indeed, many authors have reported high resistance of An. gambiae sl collected in polluted environments to several classes of insecticides, suggesting that other xenobiotics that are not insecticides, such as petroleum products, could select for insecticide resistance (60–63). In addition, urban agriculture has intensified the use of pesticides in urban areas which can lead to mosquito breeding sites and enhance their tolerance to new insecticides (64, 65). It is important to extend the study to many sites (urban and agricultural) to better appreciate the spread of this resistance in order to design the best strategies.
TaqMan genotyping was used to detect the underlying mechanisms of the observed resistance to neonicotinoids. Kdr East, N1575Y, and Ace-1 allelic frequencies were low or absent, and only kdr West was present with almost equal allelic frequency in alive and dead mosquitoes, suggesting that this mutation is playing little or no role in resistance to neonicotinoids in An. coluzzii populations.
The expression of the metabolic enzymes examined in this study showed the upregulation of almost the same genes in urban and agricultural sites, showing that An. coluzzii uses the same mechanisms against neonicotinoid insecticides everywhere. This information is crucial for designing suitable strategies for malaria vector control. The results from this study showed that resistance is widespread in both agricultural and urban sites. Therefore, it is important to implement an intersectoral collaboration between the Ministry of Health, Ministry of Agriculture, Ministry of Education, Ministry of Urban Planning, municipalities, and farmers organizations through educational programs, community engagement sessions, and field-based farmer field school learning programs. These different sectors should discuss, interact, actively engage, and empower farming communities with basic knowledge and skills regarding malaria issues, crop pests, pesticide management, and general good agricultural practices. These actions could contribute to preventing insecticide resistance in malaria vectors.
Conclusion
These findings showed that agricultural and environmental factors coupled with the misuse and overuse of insecticides by farmers might contribute to the spread of insecticide resistance in malaria vectors. However, full susceptibility was obtained with clothianidin at 4ug/L. This useful information could help policymakers to better plan insecticide resistance management using clothianidin in combination with pyrethroids or in rotation with the current insecticides used to enhance the efficacy of vector control tools and delay the spread of vector resistance. Insecticide resistance is a real threat to malaria control interventions and new tools and approaches are needed to address this issue. The data generated from this study could serve as a baseline in the development of vector control strategies based on the use of neonicotinoids.
Data availability statement
All data generated or analysed during this study are included in the manuscript and its additional file. The datasets supporting the findings herein are included in the article. The genotyping and gene expression profile data have been deposited in Dryad datasets. Data are accessible through these links https://datadryad.org/stash/share/140Jmsx2M9I7PiXKQ8kSe5lpcE8H3KahCkFXFb5N1SI, https://datadryad.org/stash/share/2dEq9dSIUoWA41wO3dC8BYeWXFaeTlzI2-qnM-tx5Pk.
Ethics statement
Ethical approval was not required for the study involving animals in accordance with the local legislation and institutional requirements.
Author contributions
GT: Conceptualization, Investigation, Methodology, Writing – original draft, Writing – review & editing. CD: Investigation, Methodology, Writing – review & editing. GT-Y: Supervision, Writing – review & editing. MK: Investigation, Methodology, Writing – review & editing. SA: Investigation, Methodology, Writing – review & editing. ET: Methodology, Writing – review & editing. DA: Investigation, Methodology, Writing – review & editing. MG: Investigation, Methodology, Writing – review & editing. HS: Supervision, Writing – review & editing. MT: Supervision, Writing – review & editing. MD: Supervision, Writing – review & editing. CW: Conceptualization, Supervision, Writing – review & editing. RD: Conceptualization, Funding acquisition, Supervision, Writing – review & editing.
Funding
The author(s) declare financial support was received for the research, authorship, and/or publication of this article. This research was funded with the Wellcome Trust International Intermediate Fellowship 214201/Z/18/Z awarded to RD.
Acknowledgments
The authors acknowledged Claude Gande for his technical assistance and collaboration during sample collections and laboratory activities in the course of the study. The authors also thank the farmers and populations for their cooperation and assistance during the fieldwork.
Conflict of interest
The authors declare that the research was conducted in the absence of any commercial or financial relationships that could be construed as a potential conflict of interest.
The author(s) declared that they were an editorial board member of Frontiers, at the time of submission. This had no impact on the peer review process and the final decision.
Publisher’s note
All claims expressed in this article are solely those of the authors and do not necessarily represent those of their affiliated organizations, or those of the publisher, the editors and the reviewers. Any product that may be evaluated in this article, or claim that may be made by its manufacturer, is not guaranteed or endorsed by the publisher.
Supplementary material
The Supplementary Material for this article can be found online at: https://www.frontiersin.org/articles/10.3389/fitd.2024.1339811/full#supplementary-material
Supplementary Table S1 | qRT-PCR primer details for gene expression analysis.
References
1. WHO. World malaria report (2022). Available online at: https://www.who.int/publications-detailredirect/9789240040496.
2. Aïkpon R, Sèzonlin M, Tokponon F, Okè M, Oussou O, Oké-Agbo F, et al. Good performances but short lasting efficacy of Actellic 50 EC Indoor Residual Spraying (IRS) on malaria transmission in Benin, West Africa. Parasit Vectors. (2014) 7:256. doi: 10.1186/1756-3305-7-256
3. Djènontin A, Bio-Bangana S, Moiroux N, Henry MC, Bousari O, Chabi J, et al. Culicidae diversity, malaria transmission and insecticide resistance alleles in malaria vectors in Ouidah-Kpomasse-Tori district from Benin (West Africa): A pre-intervention study. Parasit Vectors. (2010) 3:83. doi: 10.1186/1756-3305-3-83
4. Djouaka R, Riveron JM, Yessoufou A, Tchigossou G, Akoton R, Irving H, et al. Multiple insecticide resistance in an infected population of the malaria vector Anopheles funestus in Benin. Parasit Vectors. (2016) 9:453. doi: 10.1186/s13071-016-1723-y
5. Ossè RA, Aïkpon R, Gbédjissi GL, Gnanguenon V, Sèzonlin M, Govoétchan R, et al. A shift from Indoor Residual Spraying (IRS) with bendiocarb to Long-Lasting Insecticidal (mosquito) Nets (LLINs) associated with changes in malaria transmission indicators in pyrethroid resistance areas in Benin. Parasit Vectors. (2013) 6:73. doi: 10.1186/1756-3305-6-73
6. Bhatt S, Weiss DJ, Cameron E, Bisanzio D, Mappin B, Dalrymple U, et al. The effect of malaria control on Plasmodium falciparum in Africa between 2000 and 2015. Nature. (2015) 526:207–11. doi: 10.1038/nature15535
7. Ranson H, Lissenden N. Insecticide resistance in african anopheles mosquitoes: A worsening situation that needs urgent action to maintain malaria control. Trends Parasitol. (2016) 32:187–96. doi: 10.1016/j.pt.2015.11.010
8. WHO. Medecines for malaria Venture. (2018). Available online at: https://www.mmv.org/newsroom/publications/world-malaria-report-2017.
9. WHO. WHO recommended insecticides for indoor residual spraying against malaria vectors. PAHO/WHO | Pan American Health Organization 2018 (2018).
10. Ngufor C, Tungu P, Malima R, Kirby M, Kisinza W, Rowland M. Insecticide-treated net wall hangings for malaria vector control: an experimental hut study in north-eastern Tanzania. Malar J. (2014) 13:366. doi: 10.1186/1475-2875-13-366
11. Corbel V, N’guessan R, Brengues C, Chandre F, Djogbenou L, Martin T, et al. Multiple insecticide resistance mechanisms in Anopheles Gambiae and Culex quinquefasciatus from Benin, West Africa. Acta Trop. (2007) 101:207–16. doi: 10.1016/j.actatropica.2007.01.005
12. Ngufor C, N’Guessan R, Fagbohoun J, Subramaniam K, Odjo A, Fongnikin A, et al. Insecticide resistance profile of Anopheles Gambiae from a phase II field station in Cové, southern Benin: implications for the evaluation of novel vector control products. Malar J. (2015) 14:464. doi: 10.1186/s12936-015-0981-z
13. Sovi A, Keita C, Sinaba Y, Dicko A, Traore I, Cisse MBM, et al. Anopheles Gambiae (s.l.) exhibit high intensity pyrethroid resistance throughout Southern and Central Mali (2016–2018): PBO or next generation LLINs may provide greater control. Parasit Vectors. (2020) 13:239. doi: 10.1186/s13071-020-04100-7
14. Damien GB, Djènontin A, Chaffa E, Yamadjako S, Drame PM, Ndille EE, et al. Effectiveness of insecticidal nets on uncomplicated clinical malaria: a case–control study for operational evaluation. Malar J. (2016) 15:102. doi: 10.1186/s12936-016-1156-2
15. Tokponnon FT, Aholoukpe B, Denon EY, Gnanguenon V, Bokossa A, N’guessan R, et al. Evaluation of the coverage and effective use rate of long-lasting insecticidal nets after nation-wide scale up of their distribution in Benin. Parasit Vectors. (2013) 6:265. doi: 10.1186/1756-3305-6-265
16. Kpanou CD, Sagbohan HW, Dagnon F, Padonou GG, Ossè R, Salako AS, et al. Characterization of resistance profile (intensity and mechanisms) of Anopheles Gambiae in three communes of northern Benin, West Africa. Malar J. (2021) 20:328. doi: 10.1186/s12936-021-03856-2
17. Kpanou CD, Sagbohan HW, Sovi A, Osse R, Padonou GG, Salako A, et al. Assessing insecticide susceptibility and resistance intensity of Anopheles Gambiae sl populations from some districts of Benin Republic, West Africa. J Med Entomol. (2022) 59:949–56. doi: 10.1093/jme/tjac037
18. Aïzoun N, Aïkpon R, Gnanguenon V, Oussou O, Agossa F, Padonou GG, et al. Status of organophosphate and carbamate resistance in Anopheles Gambiae sensu lato from the south and north Benin, West Africa. Parasit Vectors. (2013) 6:274. doi: 10.1186/1756-3305-6-274
19. Djègbè I, Boussari O, Sidick A, Martin T, Ranson H, Chandre F, et al. Dynamics of insecticide resistance in malaria vectors in Benin: first evidence of the presence of L1014S kdr mutation in Anopheles Gambiae from West Africa. Malar J. (2011) 10:261. doi: 10.1186/1475-2875-10-261
20. Djogbénou L, Pasteur N, Bio-Bangana S, Baldet T, Irish SR, Akogbeto M, et al. Malaria vectors in the Republic of Benin: distribution of species and molecular forms of the Anopheles Gambiae complex. Acta Trop. (2010) 114:116–22. doi: 10.1016/j.actatropica.2010.02.001
21. Sovi A, Djègbè I, Soumanou L, Tokponnon F, Gnanguenon V, Azondékon R, et al. Microdistribution of the resistance of malaria vectors to deltamethrin in the region of Plateau (southeastern Benin) in preparation for an assessment of the impact of resistance on the effectiveness of Long Lasting Insecticidal Nets (LLINs). BMC Infect Dis. (2014) 14:103. doi: 10.1186/1471-2334-14-103
22. Akogbéto MC, Aïkpon RY, Azondékon R, Padonou GG, Ossè RA, Agossa FR, et al. Six years of experience in entomological surveillance of indoor residual spraying against malaria transmission in Benin: lessons learned, challenges and outlooks. Malar J. (2015) 14:242. doi: 10.1186/s12936-015-0757-5
23. Salako AS, Ahogni I, Kpanou C, Sovi A, Azondekon R, Sominahouin AA, et al. Baseline entomologic data on malaria transmission in prelude to an indoor residual spraying intervention in the regions of Alibori and Donga, Northern Benin, West Africa. Malar J. (2018) 17:392. doi: 10.1186/s12936-018-2507-y
24. Jeschke P, Nauen R, Schindler M, Elbert A. Overview of the status and global strategy for neonicotinoids. J Agric Food Chem. (2011) 59:2897–908. doi: 10.1021/jf101303g
25. Simon-Delso N, Amaral-Rogers V, Belzunces LP, Bonmatin JM, Chagnon M, Downs C, et al. Systemic insecticides (neonicotinoids and fipronil): trends, uses, mode of action and metabolites. Environ Sci pollut Res. (2015) 22:5–34. doi: 10.1007/s11356-014-3470-y
26. Tomizawa M, Yamamoto I. Structure-activity relationships of nicotinoids and imidacloprid analogs. J Pestic Sci. (1993) 18:91–8. doi: 10.1584/jpestics.18.91
27. Ensley J. Application of highway capacity manual 2010 level-of-service methodologies for planning deficiency analysis. Masters Theses. (2012) 147:1–175.
28. Rose PH. Nicotine and the neonicotinoids. In: Marrs T, editor. Mammalian toxicology of insecticides, vol. 37 . The Royal Society of Chemistry (2012). p. 184–220. doi: 10.1039/9781849733007
29. Tomizawa M, Casida JE. Neonicotinoid insecticide toxicology: Mechanisms of Selective Action. Annu Rev Pharmacol Toxicol. (2005) 45:247–68. doi: 10.1146/annurev.pharmtox.45.120403.095930
30. Anadón A, Ares I, Martínez M, Martínez-Larrañaga MR, Martínez MA. Neurotoxicity of neonicotinoids. In: Aschner M, Costa LG, editors. Advances in neurotoxicology. Elsevier Inc.; Academic Press, Spain, Madrid (2020). p. 167–207.
31. Elbert A, Nauen R, Leicht W. Imidacloprid, a novel chloronicotinyl insecticide: biological activity and agricultural importance. In: Ishaaya I, Degheele D, editors. . Insecticides with novel modes of action: mechanisms and application. Springer, Berlin, Heidelberg (1998).
32. Ngufor C, Fongnikin A, Rowland M, N’Guessan R. Indoor residual spraying with a mixture of clothianidin (a neonicotinoid insecticide) and deltamethrin provides improved control and long residual activity against pyrethroid resistant Anopheles Gambiae sl in Southern Benin. PloS One. (2017) 12:e0189575. doi: 10.1371/journal.pone.0189575
33. WHO. List of WHO prequalified vector control products. Geneva, Switzerland: World Health Organization (2020).
34. Talom AD, Essoung MA, Gbankoto A, Tchigossou G, Akoton R, Sahabi BBA, et al. A preliminary analysis on the effect of copper on Anopheles coluzzii insecticide resistance in vegetable farms in Benin. Sci Rep. (2020) 10:6392. doi: 10.1038/s41598-020-63086-5
35. Service MW. Vector-borne disease control in humans through rice agroecosystem management. Parasitol Today. (1989) 5:228–9. doi: 10.1016/0169-4758(89)90277-9
36. Gillies MT, de Meillon B. The anophelinae of africa south of the sahara (Ethiopian zoogeographical region). Afr Inst Med Res. (1987) 55:1–143.
37. Gillies MT, Coetzee MA. Supplement to the anophelinae of africa south of the sahara (Afrotropical region). Afr Inst Med Res. (1987) 55:1–143.
38. Livak KJ. Organization and Mapping of a Sequence on the Drosophila melanogaster X and Y Chromosomes That Is Transcribed during Spermatogenesis. Genetics. (1984) 107:611–34. doi: 10.1093/genetics/107.4.611
39. Santolamazza F, Mancini E, Simard F, Qi Y, Tu Z, della Torre A. Insertion polymorphisms of SINE200 retrotransposons within speciation islands of Anopheles Gambiae molecular forms. Malar J. (2008) 7:163. doi: 10.1186/1475-2875-7-163
40. Scott JA, Brogdon WG, Collins FH. Identification of single specimens of the Anopheles Gambiae complex by the polymerase chain reaction. Am J Trop Med Hyg. (1993) 49:520–9. doi: 10.4269/ajtmh.1993.49.520
41. Mouhamadou CS, de Souza SS, Fodjo BK, Zoh MG, Bli NK, Koudou BG. Evidence of insecticide resistance selection in wild Anopheles coluzzii mosquitoes due to agricultural pesticide use. Infect Dis Poverty. (2019) 8:64. doi: 10.1186/s40249-019-0572-2
42. Brogdon WG, McAllister JC. Simplification of adult mosquito bioassays through use of time-mortality determinations in glass bottles. J Am Mosq Control Assoc. (1998) 14:159–64.
43. Horstmann CS. Modified CDC bottle assay with single active ingredients of Fludora® fusion. (2016).
44. Fouet C, Ashu AF, Ambadiang MM, Tchapga W, Wondji CS, Kamdem C. Resistance of Anopheles Gambiae to the new insecticide clothianidin associated with unrestricted use of agricultural neonicotinoids in Yaoundé, Cameroon. bioRxiv. (2020), 1–27. doi: 10.1101/2020.08.06.239509
45. Jones CM, Liyanapathirana M, Agossa FR, Weetman D, Ranson H, Donnelly MJ, et al. Footprints of positive selection associated with a mutation (N1575Y) in the voltage-gated sodium channel of. Anopheles Gambiae. Proc Natl Acad Sci U.S.A. (2012) 109:6614–9. doi: 10.1073/pnas.1201475109
46. Bass C, Nikou D, Blagborough AM, Vontas J, Sinden RE, Williamson MS, et al. PCR-based detection of Plasmodium in Anopheles mosquitoes: a comparison of a new high-throughput assay with existing methods. Malar J. (2008) 7:177. doi: 10.1186/1475-2875-7-177
47. Djouaka RJ, Atoyebi SM, Tchigossou GM, Riveron JM, Irving H, Akoton R, et al. Evidence of a multiple insecticide resistance in the malaria vector Anopheles funestus in South West Nigeria. Malar J. (2016) 15:565. doi: 10.1186/s12936-016-1615-9
48. Tchouakui M, Assatse T, Mugenzi LMJ, Menze BD, Nguiffo-Nguete D, Tchapga W, et al. Comparative study of the effect of solvents on the efficacy of neonicotinoid insecticides against malaria vector populations across Africa. Infect Dis Poverty. (2022) 11:35. doi: 10.1186/s40249-022-00962-4
49. Tepa A, Kengne-Ouafo JA, Djova VS, Tchouakui M, Mugenzi LMJ, Djouaka R, et al. Molecular drivers of multiple and elevated resistance to insecticides in a population of the malaria vector anopheles Gambiae in agriculture hotspot of west Cameroon. Genes. (2022) 13:1206. doi: 10.3390/genes13071206
50. Riveron JM, Ibrahim SS, Chanda E, Mzilahowa T, Cuamba N, Irving H, et al. The highly polymorphic CYP6M7 cytochrome P450 gene partners with the directionally selected CYP6P9a and CYP6P9b genes to expand the pyrethroid resistance front in the malaria vector Anopheles funestus in Africa. BMC Genomics. (2014) 15:817. doi: 10.1186/1471-2164-15-817
51. Schmittgen TD, Livak KJ. Analyzing real-time PCR data by the comparative C(T) method. Nat Protoc. (2008) 3:1101–8. doi: 10.1038/nprot.2008.73
52. Anto F, Asoala V, Anyorigiya T, Oduro A, Adjuik M, Owusu-Agyei S, et al. Insecticide resistance profiles for malaria vectors in the Kassena-Nankana district of Ghana. Malar J. (2009) 8:81. doi: 10.1186/1475-2875-8-81
53. Oxborough R, Seyoum A, Yihdego Y, Dabiré R, Gnanguenon V, Wat’senga F, et al. Susceptibility testing of Anopheles malaria vectors with the neonicotinoid insecticide clothianidin; results from 16 African countries, in preparation for indoor residual spraying with new insecticide formulations. Malar J. (2019) 18:1–11. doi: 10.1186/s12936-019-2888-6
54. Ekra AK, Edi CAV, Gbalegba GCN, Zahouli JZB, Danho M, Koudou BG. Can neonicotinoid and pyrrole insecticides manage malaria vector resistance in high pyrethroid resistance areas in Côte d’Ivoire? Malar J. (2024) 23:160. doi: 10.1186/s12936-024-04917-y
55. Guillet P, Hemingway J, Baldet T, Brengues C, Akoobeto M, Chandre F, et al. The role of agricultural use of insecticides in resistance to pyrethroids in Anopheles Gambiae s.l. in Burkina Faso. Am J Trop Med Hyg. (2002) 67:617–22. doi: 10.4269/ajtmh.2002.67.617
56. Hien AS, Soma DD, Hema O, Bayili B, Namountougou M, Gnankiné O, et al. Evidence that agricultural use of pesticides selects pyrethroid resistance within Anopheles Gambiae s.l. populations from cotton growing areas in Burkina Faso, West Africa. PloS One. (2017) 12:e0173098. doi: 10.1371/journal.pone.0173098
57. Nkya TE, Akhouayri I, Poupardin R, Batengana B, Mosha F, Magesa S, et al. Insecticide resistance mechanisms associated with different environments in the malaria vector Anopheles Gambiae: a case study in Tanzania. Malar J. (2014) 13:28. doi: 10.1186/1475-2875-13-28
58. Fouet C, Atkinson P, Kamdem C. Human interventions: driving forces of mosquito evolution. Trends Parasitol. (2018) 34:127–39. doi: 10.1016/j.pt.2017.10.012
59. Bradford BZ, Huseth AS, Groves RL. Widespread detections of neonicotinoid contaminants in central Wisconsin groundwater. PloS One. (2018) 13:e0201753. doi: 10.1371/journal.pone.0201753
60. Antonio-Nkondjio C, Fossog BT, Ndo C, Djantio BM, Togouet SZ, Awono-Ambene P, et al. Anopheles Gambiae distribution and insecticide resistance in the cities of Douala and Yaoundé (Cameroon): influence of urban agriculture and pollution. Malar J. (2011) 10:154. doi: 10.1186/1475-2875-10-154
61. Djouaka RF, Bakare AA, Bankole HS, Doannio JM, Coulibaly ON, Kossou H, et al. Does the spillage of petroleum products in Anopheles breeding sites have an impact on the pyrethroid resistance? Malar J. (2007) 6:159.
62. Fossog Tene B, Poupardin R, Costantini C, Awono-Ambene P, Wondji CS, Ranson H, et al. Resistance to DDT in an urban setting: common mechanisms implicated in both M and S forms of anopheles Gambiae in the city of yaoundé Cameroon. PloS One. (2013) 8:1–9. doi: 10.1371/journal.pone.0061408
63. Kamdem C, Fouet C, Gamez S, White BJ. Pollutants and insecticides drive local adaptation in african malaria mosquitoes. Mol Biol Evol. (2017) 34:1261–75. doi: 10.1093/molbev/msx087
64. Gatton ML, Chitnis N, Churcher T, Donnelly MJ, Ghani AC, Godfray HCJ, et al. The importance of mosquito behavioural adaptations to malaria control in Africa. Evolution. (2013) 67:1218–30. doi: 10.1111/evo.12063
Keywords: Anopheles coluzzii, neonicotinoids, resistance, metabolic mechanism, agriculture, urban
Citation: Tchigossou G, Dossou C, Tepa-Yotto G, Koto M, Atoyebi SM, Tossou E, Adanzounon D, Gouété M, Sina H, Tchouakui M, Dione M, Wondji C and Djouaka R (2024) Resistance to neonicotinoids is associated with metabolic detoxification mechanisms in Anopheles coluzzii from agricultural and urban sites in southern Benin. Front. Trop. Dis 5:1339811. doi: 10.3389/fitd.2024.1339811
Received: 16 November 2023; Accepted: 08 July 2024;
Published: 02 August 2024.
Edited by:
Jacy Gameiro, Juiz de Fora Federal University, BrazilReviewed by:
Muhammad Musa Khan, Zhejiang University, ChinaDelenasaw Yewhalaw, Jimma University, Ethiopia
Copyright © 2024 Tchigossou, Dossou, Tepa-Yotto, Koto, Atoyebi, Tossou, Adanzounon, Gouété, Sina, Tchouakui, Dione, Wondji and Djouaka. This is an open-access article distributed under the terms of the Creative Commons Attribution License (CC BY). The use, distribution or reproduction in other forums is permitted, provided the original author(s) and the copyright owner(s) are credited and that the original publication in this journal is cited, in accordance with accepted academic practice. No use, distribution or reproduction is permitted which does not comply with these terms.
*Correspondence: Rousseau Djouaka, ci5kam91YWthQGNnaWFyLm9yZw==; Genevieve Tchigossou, Zy50Y2hpZ29zc291QGNnaWFyLm9yZw==; Charles Wondji, Y2hhcmxlcy53b25kamlAbHN0bWVkLmFjLnVr