- 1Laboratoire d’Entomologie Fondamentale et Appliquée, Unité de Formation et de Recherche en Sciences de la Vie et de la Terre, Université Joseph KI-ZERBO, Ouagadougou, Burkina Faso
- 2Institut de Recherche en Sciences de la Santé/Centre Muraz, Bobo-Dioulasso, Burkina Faso
Wolbachia is a maternally inherited bacterium commonly detected in approximately 50% of arthropod species, including mosquito vector species. Wolbachia species have been detected in different mosquito vectors, but in most malaria vectors, their occurrence in natural populations were reported 10 years ago. Aedes aegypti, the main vector of dengue virus, is generally uninfected by Wolbachia, and records of infection are rare and only include a few populations. This bacterium impacts the biology, ecology, and evolution of vector populations. Wolbachia has attracted considerable interest because of its role in reducing disease transmission. Moreover, this bacterium is known to manipulate insect reproduction by inducing cytoplasmic incompatibility (CI), thus providing new avenues for vector control strategies. Interestingly, wMel or wAlbB Wolbachia infections in Aedes populations exhibit a stable high frequency in most areas and contribute to the reduction of local dengue transmission. In natural populations of Anopheles, although Wolbachia was found, little is known about its role and effect on Plasmodium. If the incompatible insect technique (IIT) and population replacement strategy resulted in significant decreases in the dengue transmission in endemic countries such as the USA, Taiwan, Australia, and Brazil, natural Wolbachia detection in mosquitoes may pose a threat to these vector control strategies, raising the following question: “Does the natural occurrence of Wolbachia in Anopheles sp. and Ae. aegypti populations compromise the success of vector control strategies? This review presents recent achievements of Wolbachia in natural Anopheles and Ae. aegypti populations in terms of prevalence and provides guidelines for the development of Wolbachia-based vector control.
1 Introduction
In tropical and subtropical regions, dengue and malaria remain the two main vector-borne infectious diseases transmitted by Aedes aegypti and Anopheles gambiae s.l., respectively.
Malaria is a life-threatening disease caused by parasites transmitted to people through the bites of infected female An. gambiae s.l mosquitoes. Overall, each year, the number of infected people varies from 154 to 289 million, with approximately 80% of all malaria deaths recorded mostly in children under 5 years of age in endemic regions of Africa (1). Among the arboviroses, i.e., yellow fever virus (YFV), Zika virus, Chikungunya virus (CHYKV), and dengue virus, transmitted generally by Ae. aegypti, dengue virus is the most prevalent in subtropical and tropical areas and remains a major public health concern (2).
Vector control based on chemicals remains the most effective strategy for controlling the transmission of dengue and malaria diseases. Long-lasting insecticide-treated nets (LLINs) and indoor residual spraying (IRS) are the main vector control strategies (3). These methods have significantly contributed to a decrease in malaria incidence. However, the effectiveness of vector control may be constrained by the increasing insecticide resistance in Anopheles vectors in many countries, which has now been observed in almost all African countries (4). Cases of insecticide resistance in Aedes populations have also been reported in many areas (5).
With regard to insecticide resistance occurring in several areas of endemic countries, particularly for both diseases, there is a need for new vector control technologies. Existing control methods, including environmental/mechanical (e.g., reduction source or destruction of breeding sites), biological (e.g., Bacillus thuringiensis var. israelensis, entomopathogenic fungi, larvivorous fish, and copepods), chemical (e.g., insect growth regulators, pyrethroids, and DDT), and endosymbiont Wolbachia and genetic methods (e.g., sterile insect technique and genetically modified mosquitoes), can contribute to a decrease in dengue vector populations (5, 6) and in malaria vectors.
Innovative eco-friendly approaches for the control of vector diseases are under active development and could complement the current mosquito control strategies (5). Among the most promising techniques, the use of essential oils (7–10) has provided valuable data in terms of alternative vector control. The sterile insect technique (SIT; i.e., the use of males sterilized by irradiation) and the incompatible insect technique (IIT; which uses Wolbachia endosymbionts to induce cytoplasmic incompatibility) could lead to population suppression, and the release of males reduces the fertility of wild females (11).
Wolbachia is an endosymbiotic, Gram-negative intracellular bacterium described for the first time within the reproductive tissues of Culex pipiens mosquitoes in 1924 (12). Most of the arthropod Wolbachia strains belong to clades A and B, whereas clades C and D are observed in filarial nematodes (13). These bacteria, which are members of the order Rickettsiales within the class α-Proteobacteria, cannot be cultured outside the host cells. According to Weinert et al. (14) and Bailly-Bechet et al. (15), Wolbachia is considered to be the most abundant symbiont and has been found to infect approximately 50% of all arthropod species.
In previous decades, Wolbachia has received special attention due to the diversity of its phenotypes, including reproductive manipulations (16–19), nutrient synthesis (20), physiological and behavioral modifications, and its impacts on susceptibility to pathogens (21–25).
To the best of our knowledge, studies have reported the occurrence of Wolbachia in around 31 species of Anopheles, but a few of these studies did not investigate its ability to inhibit Plasmodium in host populations. Moreover, a lower prevalence (from 0.2% to 13.24%) of Wolbachia was found in Ae. aegypti populations from Manila (Philippines), Florida, and Panama, whereas those found in New Mexico reached 57% (26). Thereafter, a number of Ae. aegypti samples from New Mexico were screened for confirmation (27). Thus, both real-time PCR and loop-mediated isothermal amplification (LAMP) assays were performed, but no Wolbachia wAlbB strain infection was detected among 120 individual mosquitoes that previously tested positive for Wolbachia (27). According to these studies, molecular detection methods (e.g., LAMP, PCR, antibiotic treatment, intracellular localization by STEM, and FISH) are useful for confirming the presence of Wolbachia.
The two main Wolbachia-based strategies for the reduction of disease transmission are IIT or population suppression and population replacement (see Figure 1). Their implementation through the consecutive releases of males artificially infected with Wolbachia-inducing cytoplasmic incompatibility (CI) and female populations involved in Wolbachia infection transmission have at times been proven to reduce vector competence (28).
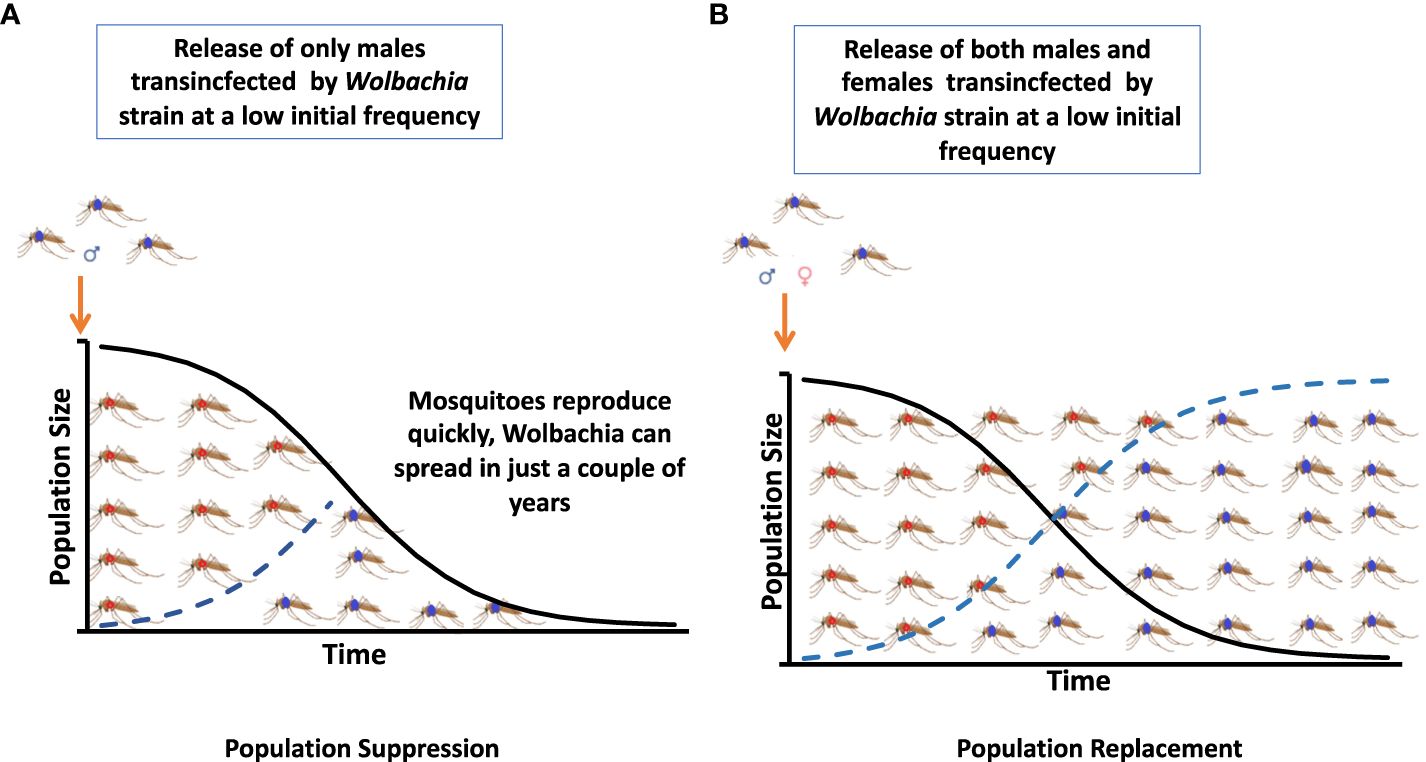
Figure 1 Two strategies based on Wolbachia for the reduction of disease transmission are an incompatible insect technique (IIT) or population suppression and a population replacement strategy. (A) Population suppression. (B) Population replacement.
This review presents recent insights into Wolbachia in natural Anopheles sp. and Ae. aegypti populations in terms of prevalence and outlines its major role in pathogen transmission. It also focuses on the implications of natural infections in Anopheles and Aedes populations for Wolbachia-based disease control strategies.
2 What are endosymbiotic Wolbachia?
Wolbachia is naturally found in many species of arthropods, but can also be transfected to prevent the transmission of diseases (12). This bacterium belongs to the α-Proteobacteria within the order Rickettsiales. It cannot be cultivated outside the host cells. Based on genetic similarity, Wolbachia species are divided into supergroups A, B, C, D, E, F, and H, which appear to be linked to particular host classes (29). Recently, a novel supergroup named S has been identified in the pseudoscorpion Cordylochernes scorpioides, which is most closely related to Wolbachia supergroups C and F (30).
Wolbachia species are vertically and maternally transmitted through the egg cytoplasm and manipulate host reproduction by inducing CI (Figure 2), feminization, killing of male embryos, and parthenogenesis to enhance their spread (12, 29). Maternal transmission and the induction of various phenotypes in the hosts remain the two key features induced by this bacterium (31).
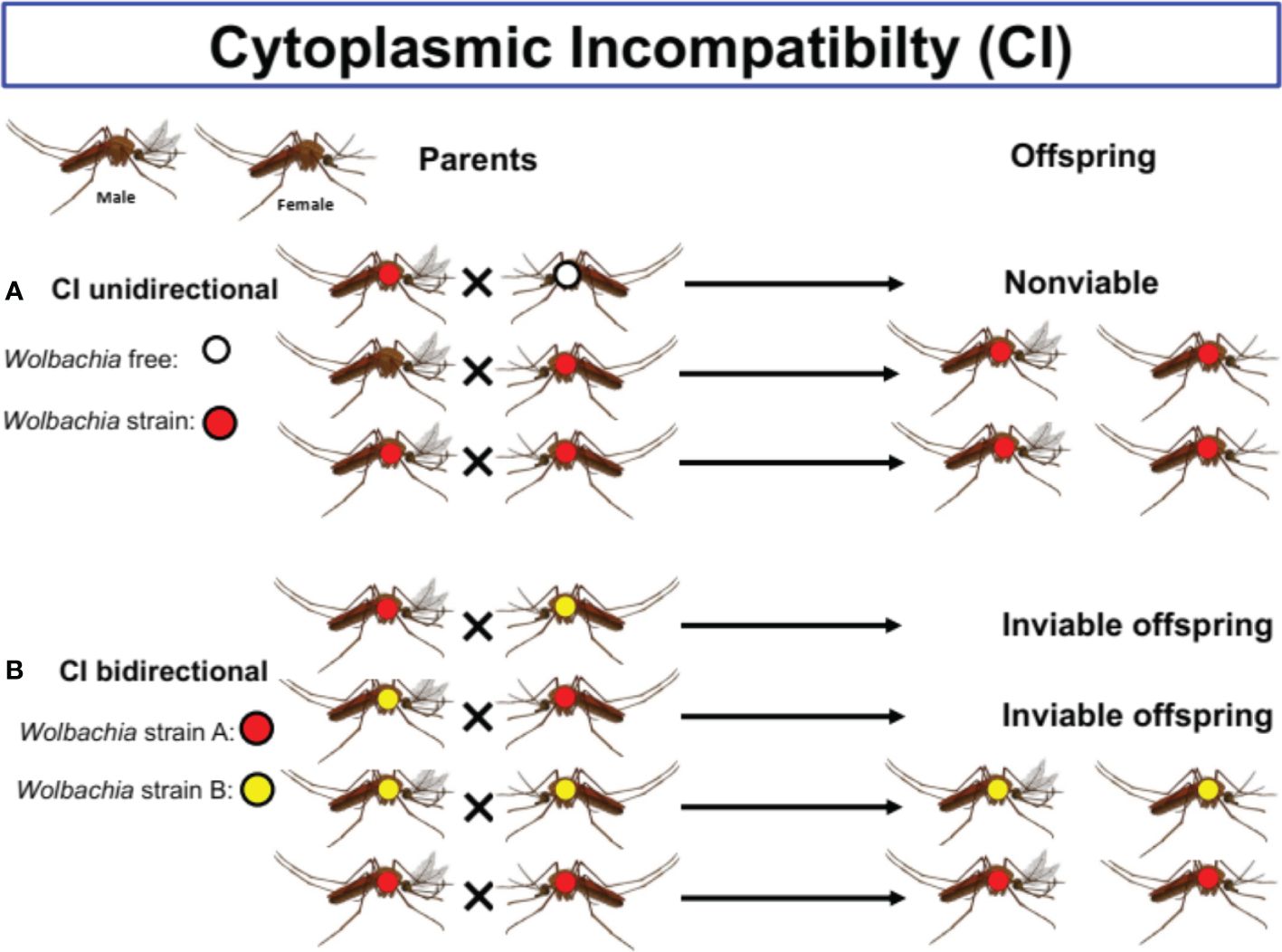
Figure 2 The different types Cytoplasmic Incompatibility (CI) in mosquitoes species. Cytoplasmic incompatibility (CI) prevents infected males from successfully mating with females that lack the same Wolbachia types. (A) Unidirectional CI occurring between Wolbachia infected males and natural uninfected females allows Wolbachia to invade uninfected populations. (B) Bidirectional CI occurring when both reciprocal crosses between males and females infected with different strains of Wolbachia are incompable.
In CI (see Figure 2), Wolbachia induces the death of embryonic offspring from a cross between uninfected females and infected males (18). As far as male killing is concerned, this bacterium causes the death of male offspring (18, 31). Finally, parthenogenesis and feminization induction result from the transformation of potential males into females. In parthenogenesis, zygotes can develop without mating (18, 31).
In general, all phenotypes increase the number of infected females in the host population, thereby increasing the transmission of endosymbionts to the next generation. According to LePage and Bordenstein (29), when a Wolbachia strain (e.g., that induced CI) infection is viable, this leads to a fitness advantage of the infected females compared with uninfected females. The discovery of the Wolbachia strains wMel, showing the capacity to reduce vector competence (32); wMelpop, favoring life-shortening of infected individual populations; and wAlB, highlighting increased resistance against infectious agents causing diseases, provides promise in terms of vector control strategies (12, 29). With these different phenotypes, Wolbachia can be used as a control agent against vector-borne diseases (22, 27, 33).
3 Wolbachia and Anopheles vector populations: prevalence, co-occurrence of Wolbachia and Plasmodium, and effect on Plasmodium sp.
3.1 Prevalence of Wolbachia in natural Anopheles vector populations
Although Wolbachia has been found in approximately 40% of 147 culicine species such as Culex sp. and Aedes albopictus, it was not detected in Anopheles mosquitoes until its first occurrence in natural populations in Africa approximately 10 years ago (34).
According to Bourtzis et al. (35), the absence of Wolbachia could be the outcome of incompatible physiological environments in Anopheles mosquitoes, an inability to obtain Wolbachia by horizontal transmission from other species, or a putative competitive exclusion by native bacteria in Anopheles spp.
In general, nested PCR-targeted 16S rRNA sequencing, quantitative PCR (qPCR), and electron microscopy are used for the detection of Wolbachia in Anopheles populations in most studies. Table 1 displays the techniques used by Walker et al. (36) combining molecular detection and electronic microscopy for the detection of Wolbachia.
Therefore, to show evidence of a stable, maternally transmitted Wolbachia in a host (45), a number of steps must be highlighted, as follows: i) examining Wolbachia in different host tissues using fluorescence in situ hybridization (FISH) or electron microscopy; ii) exhibiting that Wolbachia is and can be maternally transmitted following reciprocal crosses; and iii) showing that this bacterium can be blocked in mosquitoes with antibiotic treatment (27).
The prevalence of Wolbachia in Anopheles mosquitoes could be influenced by either i) native microbiota interference or ii) Wolbachia–host interaction. Wolbachia coexists with native microbiota that could interfere with other bacteria, leading to a lower prevalence rate. Evidence suggests that Asaia, a native microbiome in Anopheles mosquitoes, impedes the vertical transmission of Wolbachia (46, 47) and represents an eventual competitor to Wolbachia. Asaia inhibits the maternal transmission of Wolbachia (48) and could induce the mutual exclusion of Wolbachia in the gonads (49).
Globally, the mechanisms that limit the levels of Wolbachia are not well elucidated. One hypothesis is that Wolbachia can adapt to replication control as a strategy to evade host immunity (50). It is also possible that Anopheles does not represent a suitable host for Wolbachia. Moreover, the immunity or metabolism of Anopheles might limit the presence of Wolbachia.
In Aedes, Wolbachia manipulates the metabolism of host lipids, and cholesterol sequestration might favor protection against viruses (51). The lipid metabolism in Anopheles is not well known; however, poor nutritional stores could explain the inability of Anopheles mosquitoes to support the high densities of Wolbachia (50).
For a long time, it was believed that Wolbachia is absent in wild Anopheles mosquitoes, until 2014, when the WAnga-Burkina Faso (wAnga-BF) strain was detected in An. gambiae collected from Burkina Faso, West Africa (34). This strain was different from those infecting other arthropods, including mosquitoes and other insects (52, 53).
A few years later, other findings showed the occurrence of Wolbachia in other Anopheles vectors in Africa (36, 37, 39–41, 43) and Southeast Asia (Myanmar and India) (54) (Table 1). The bacterium was found not only in An. gambiae and Anopheles coluzzii but also in other Anopheles species such as Anopheles arabiensis (37; 42); Anopheles demeilloni (36); Anopheles moucheti (36, 43); Anopheles funestus (40); Anopheles melas (55); Anopheles nili and Anopheles coustani (43); Anopheles maculatus (s.s.), Anopheles sawadwongporni, Anopheles pseudowillmori, Anopheles dirus (s.s.), and Anopheles baimaii (38); Anopheles carnevalei, Anopheles hancocki, Anopheles implexus, Anopheles jebudensis, Anopheles marshallii, Anopheles nigeriensis, Anopheles paludis, and Anopheles vinckei (43); Anopheles balabacensis, Anopheles latens, Anopheles introlatus, Anopheles macarthuri, Anopheles barbirostris, Anopheles hyrcanus, and Anopheles sinensis (44); and Anopheles culicifacies and Anopheles stephensi (54), totaling around 31 species of Anopheles harboring Wolbachia (Table 1).
To date, the prevalence of Wolbachia has been documented in around 20 wild Anopheles mosquito species. This prevalence varied according to both the location and the Anopheles species. A prevalence of 1% was found in An. funestus in Senegal, West Africa (40), and in Anopheles minimus, An. dirus, An. maculatus, An. pseudowillmori, Anopheles baimii, and An. sawadwongporni in Kayin state (38). Prevalence rates ranging from 2% to 15% were recorded in An. culicifacies and An. stephensi from India (54); in An. carnevalei, An. hancocki, An. implexus, An. marshallii, An. nigeriensis, An. paludis, and An. vinckei populations from Gabon, Central Africa (43); in An. arabiensis from Tanzania (37); and in An. melas from Guinea (55).
The lower prevalence rate of Wolbachia in Anopheles mosquitoes raises many questions, which could be due to the absence of a stable relationship between Wolbachia and its hosts. According to Chrostek and Gerth (45), the detection of Wolbachia in the An. gambiae population was surprising, even though its maternal transmission has been proven in the natural population of An. gambiae s.l. (34, 41, 56). The authors believed that the occurrence of Wolbachia is the result of contamination through several sources and could have been transferred firstly via endoparasitic nematodes or ectoparasitic mites, secondly via plants when Wolbachia might have been transferred from infected to uninfected insects found on the same plants, and thirdly via the water bodies of cohabitating insects infected by Wolbachia. According to Chrostek and Gerth (45), the high diversity of Wolbachia sequences associated with very low titers was incompatible with the notion of a stable, intraovarially transmitted Wolbachia symbiont in An. gambiae.
A proportion of the population of Anopheles displayed a prevalence rate ranging from 20% to 60%. This was the case for An. gambiae collected indoors in Mali (41); An. gambiae s.s. from Kalemie in the DRC (57); An. gambiae s.s.–melas hybrids from Guinea (55); An. nili collected in Gabon (43); and An. sinensis, An. introlatus, and An. latens collected in forest areas (44) (Table 1).
Interestingly, higher prevalence rates were found in An. demeilloni collected in the DRC, Central Africa (89.3% and 100% in 2015 and 2019, respectively) (36); in An. moucheti (71%) (43) and An. gambiae (78%) in Mali (57) (41); and in An. maculatus, An. barbirostris, and An. latens, with prevalence reaching 100% (44), suggesting that natural Wolbachia infections are widespread in these species of Anopheles. Walker et al. (36) provided evidence that An. demeilloni and An. moucheti harbor a high density of Wolbachia strains acquired vertically. Using phylogeographic sequencing data (wsp gene and MLST sequences), the authors showed that the wAnM strain from An. moucheti and the wAnD strain from An. demeilloni span wide geographical locations, which is consistent with the notion of a stably inherited CI induced by Wolbachia strains (43). Thus, the prevalence rates in wild mosquito populations are also consistent with CI-inducing strains, in contrast to most studies exhibiting a low prevalence of Wolbachia in the An. gambiae complex. Interestingly, sequencing of the wAnM genome revealed an interrupted cifB gene that could also be indicative of a variation in the levels of CI induced by this strain (43). Through experiments conducted by Adams et al. (58), it was shown that Wolbachia cifB induced CI in An. gambiae individuals and that the cifB-induced sterility was rescued by the expression of cifA in females. According to Ayala et al. (43), analysis of An. moucheti from an F1 progeny confirmed the absence of biological Wolbachia contamination in their studies. They also suggested that Wolbachia is maternally inherited in wild populations of An. moucheti. Therefore, it should be considered as a potential model species for further investigations of its interactions with Plasmodium infections.
According to Wong et al. (44), vegetation influences the prevalence of Wolbachia, which can be higher in forested areas than in wetlands or islands. The authors believed that the diversity and abundance of the flora and fauna in forested areas harboring more hosts with stable Wolbachia might favor horizontal transfers to other species.
For Hemingway et al. (59), the widespread insecticide resistance observed in malaria vectors in Africa could also explain the spread of Wolbachia into Anopheles populations, resulting in a reduction of malaria transmission, which is in contrast with that observed in Burkina Faso. Wolbachia was found in An. gambiae s.s. in 2006 in Soumousso and VK7 (60), during which the frequency of resistance in these populations was low. After 12 years (2018), coinciding with the high levels of insecticide resistance, Wolbachia was not detected in mosquito populations, raising doubts about the persistence of this bacterium under insecticide pressure. In the mosquito C. pipiens, the physiological costs associated with insecticide resistance limit the ability to control Wolbachia infection (61, 62). Additional investigations under different ecological settings and mosquito host genetic backgrounds are therefore needed to understand the factors affecting the dynamics of wAnga infection and the role of wAnga in vectorial capacity.
3.2 Co-occurrence of Wolbachia and Plasmodium in natural Anopheles and their interactions
In mosquitoes, oocyst development can be perturbed when Wolbachia is naturally found in the mosquitoes (63). Conversely, it can favor sporozoite production (64). More sporozoites were detected in C. pipiens, a vector of Plasmodium relictum naturally infected by Wolbachia, compared with those in C. pipiens without Wolbachia (65).
Interestingly, quantitative analysis of Wolbachia in wAnga-Mali and Plasmodium sporozoite infections in natural An. gambiae s.s. populations from Mali, West Africa, indicated a lower prevalence and intensity of Plasmodium falciparum sporozoite infection in Wolbachia-infected females (41). The presence of wAnga-BF was negatively correlated with the prevalence of P. falciparum sporozoites (56). According to Wong et al. (44), Anopheles mosquitoes with sporozoites (50%) exhibited a higher prevalence of Wolbachia than mosquitoes with oocysts (11.11%).
The wAnM and wAnD strains found naturally in An. moucheti and An. demeilloni, respectively, should have a lot of potential as candidates for use in Wolbachia biocontrol strategies in Anopheles to reduce the transmission of malaria (Figure 3). However, further studies are needed to investigate the ability to inhibit Plasmodium (36). Subsequently, the release of Wolbachia-infected males for population suppression should be performed if these strains are not ubiquitous over their native host populations (36). Conversely, if these strains are shown to inhibit Plasmodium transmission in their native hosts, as exhibited by Shaw et al. (56), selective release in areas showing lower prevalence in natural populations could lead to population replacement (36).
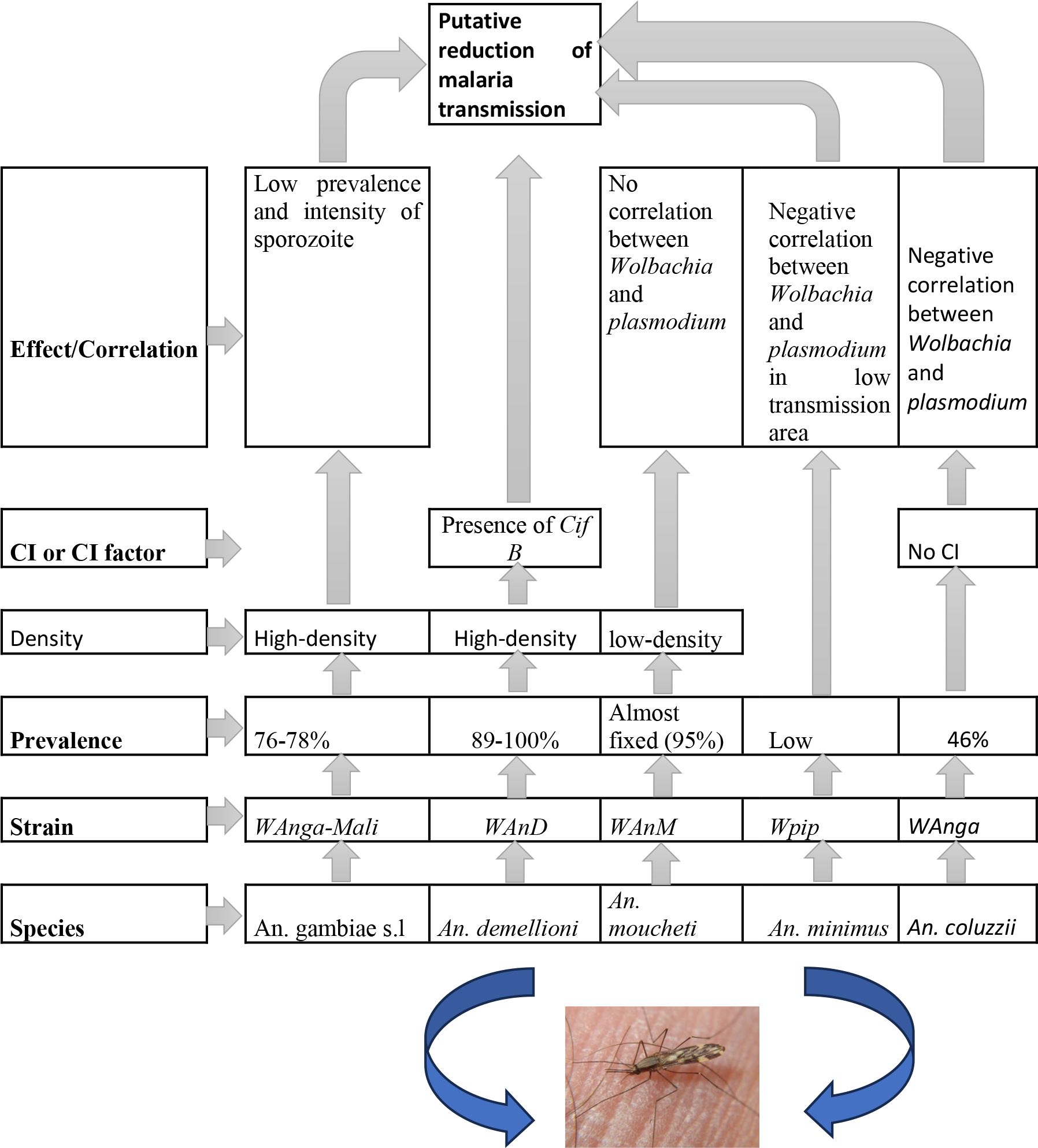
Figure 3 A synthetic view of role of Wolbachia on Plasmodium in natural Anopheles populations and guidelines for development of Wolbachia-based vector control.
3.3 Effect of Wolbachia on Plasmodium in transfected Anopheles sp.
The possible transfection of Wolbachia for malaria control may be exploited when Anopheles species do not harbor natural Wolbachia infections. However, recent studies have reported the occurrence of Wolbachia in many species of Anopheles, which could compromise the current strategies.
Transinfection by embryonic microinjection in Anopheles populations is possible, even though An. stephensi, An. arabiensis, An. gambiae, and An. funestus harbor Wolbachia strains.
Both the wMelPop and wAlbB strains that transiently and somatically infected An. gambiae have been shown to significantly reduce the levels of Plasmodium berghei or P. falciparum oocyst infection in the mosquito midgut (64, 66). For instance, these strains could prevent the development of both P. falciparum oocysts and sporozoites. Bian and Joshi (63) showed that An. stephensi mosquitoes could be stably infected with the Wolbachia wAlbB strain microinjected through eggs (63) and could exhibit both the capability to induce high levels of CI and an impeccable maternal transmission. In addition, Gomes et al. (41), through infection design, found that wAnga-Mali infection impedes the maturation of sporozoites, thus reducing malaria transmission and opening new avenues for strategies to reduce disease transmission. The authors observed Wolbachia invasion in laboratory mosquito populations following several interbreedings of naturally uninfected males with infected females of An. stephensi populations. Moreover, wAlbB conferred resistance to P. falciparum in the mosquito.
However, Wolbachia transfection has not been implemented in field trials as wAlbB provides only partial blockage of parasite transmission (67). Other Wolbachia strains (e.g., wMelPop or wAlbB) that have shown significant results in Ae. aegypti infections and transinfections in An. gambiae could also impede Plasmodium transmission. Before their implementation, Wolbachia strains that could provide better blockage of malaria through Anopheles species need to be identified (67). According to Nazni et al. (68), if Wolbachia shows the ability to block malaria following transfers in Anopheles hosts, IC will also allow Wolbachia to spread into populations after releasing both males and females. Sustainable malaria control using Wolbachia strains such as wAnD and wAnM will finally require the transfection of strains capable not only of inhibiting Plasmodium parasites but also inducing CI without significant fitness costs (Figure 3). Experimental evidence has also shown the possible horizontal transfer of Wolbachia in An. gambiae (48) and An. stephensi (63, 69, 70).
4 Wolbachia and Ae. aegypti vector populations: prevalence and effect on pathogens
4.1 Prevalence of Wolbachia in Ae. aegypti populations
Although Ae. albopictus is known to be infected by Wolbachia at high frequencies, most findings have not mentioned their presence in Ae. aegypti populations for a long time.
The absence of natural infection is beneficial for both population suppression and replacement programs because any CI induced by Wolbachia infection should be unidirectionally incompatible with natural populations (27).
Most recently, the occurrence of Wolbachia in wild populations of Ae. aegypti has been reported in several locations including Florida (71), Malaysia (72), Thailand (73), Texas and the Philippines (74), India (75), New Mexico (26), and Panama (76) (Table 2).
To the best of our knowledge, low prevalence rates of Wolbachia have been reported in Ae. aegypti populations from Panama (0.2%) (76), Florida (4.35%) (71), and the Philippines (13.24%) (74), but reached 25% in Kuala Lumpur, Malaysia (72) (Table 2). Interestingly, the highest prevalence (57.43%) was reported in Ae. aegypti populations from New Mexico (26) (Table 2). Recently, in Meghalaya (Tura, India), this prevalence has reached 73.33% (78).
All of the studies reported variable levels of infection in populations, with a relationship between infections and several Wolbachia supergroups sometimes identified. Overall, all of the sequences found in these studies were closely related to those of wAlbB infection occurring naturally in Ae. albopictus (26, 71, 74, 75). It is possible that Ae. aegypti acquire wAlbB through environmental contamination as these species coexist with Ae. albopictus and share the same ecological niche. These species share several characteristics that provide them with adaptive advantages over others, making them successful invaders (79, 80). Both vectorial species exhibit high ecological plasticity under heterogeneous anthropic, climatic, and environmental conditions.
Carvajal et al. (74) detected a strain of Wolbachia (AAML: Ae. aegypti metropolitan Manila) from supergroups that were not ever found in Diptera species. Four samples belong to supergroups C and D and 85 samples are close to supergroup B. In addition to the detection of the presence of Wolbachia using PCR, other data are needed to confirm the presence of this bacterium in laboratory colonies, such as the loss of infection through antibiotic and assay on maternal transmission of Wolbachia (26, 75). The density of Wolbachia was quite low in the Ae. aegypti population included in the study by Kulkarni et al. (26), although a high frequency was estimated.
4.2 Transinfection of Wolbachia strains into Aedes populations for vector control
The wAlbB strain found in natural populations of Ae. albopictus has been successfully introduced into Ae. aegypti to provide an inherited infection line (81). Interestingly, the Toll and IMD pathways favor the establishment and maintenance of the wAlbB infection in this cell line. The Wolbachia wMelPop (32) and wMel (12) strains from Drosophila are suitable for infecting Ae. aegypti mosquito cell lines and have been extensively used in the transinfection of Ae. aegypti mosquitoes through embryonic microinjection.
Thus, eight Wolbachia strains (wMel, wMelPopCLA, wMelCS, wRi, wAu, wAlbA, wAlbB, and wPip) were successfully transfected with Ae. aegypti (81–84). All of them induced unidirectional CI when introduced into natural uninfected Ae. aegypti, except for the wAu strain (85). According to Ant et al. (84), the Wolbachia strain wAu provided a highly efficient virus transmission blockage in Ae. aegypti. wMel, wMelPopCLA, wMelCS, wRi, and wAu occur naturally in Drosophila species, whereas wAlbA and wAlbB are found in Ae. albopictus and wPip in Culex sp. is used to infect Ae. aegypti.
wAlbB and wMelPopCLA are often transinfected in Anopheles populations. In addition, successful transfers of wAlbB (inducing CI) from Ae. albopictus into An. stephensi have shown that Anopheles spp. can sustain Wolbachia infection (63).
Transinfections were also performed in Ae. albopictus (naturally infected with both wAlbA and wAlbB), in view of creating new crossing types. Bidirectional incompatibility was observed between the transfected and the naturally infected lines when both the wPip and wMel strains were introduced into Wolbachia-cured lines (86, 87). Moreover, a triple-infected (wAlbA, wAlbB, and wPip) Ae. albopictus line was created to express unidirectional CI when crossed with double-infected natural mosquitoes.
4.3 Wolbachia-mediated pathogen interference in Aedes mosquito populations
Most studies have shown that Wolbachia can inhibit pathogens caused by dengue, chikungunya, yellow fever, Zika virus, Plasmodium parasites, and filarial nematodes in infected Ae. aegypti or Anopheles sp. (22, 32, 63, 88) (Table 3). Wolbachia can reduce i) the virus transmission rate by decreasing the number of individuals with infection in the saliva; ii) the virus dissemination rate by decreasing the number of individuals with infection in the head or leg; and iii) the viral load by reducing viral gene copies (28, 63, 87, 89, 90). Moreover, it can reduce the parasite infection rate or parasite loads by i) decreasing the number of individuals infected with the Plasmodium parasite or ii) reducing the number of oocysts in the midgut from infected mosquitoes. In addition, Wolbachia can reduce parasite transmission by reducing the sporozoite load in the mosquito salivary gland (63, 64).
Ae. aegypti infected with wMel or wAlbB is less susceptible to disseminate infection of four serotypes of DENV via the salivary glands (93, 94).
Edenborough et al. (95) recently performed a comprehensive review focusing on three subcellular modifications: i) altered lipid homeostasis; ii) disruption of the intracellular membranes; and iii) changes to the host cell cytoskeleton that can boost Wolbachia to induce its antiviral effect.
Regarding these data, the wAlB, wMel, and wmelPop strains of Wolbachia have been selected for vector control strategies.
5 Wolbachia-based control strategies to reduce vector-borne disease transmission
There are two main Wolbachia-based strategies that result in the reduction of disease transmission: IIT or population suppression and population replacement.
The release of males artificially infected with the endosymbiont Wolbachia strains inducing CI and of females capable of transmitting infection led to population replacement and population suppression (Figure 1). Successful population replacement of Ae. aegypti transinfected by Wolbachia has been achieved in several countries (85).
After the release of infected mosquitoes in Australia, Malaysia, and the USA, Wolbachia infections have maintained a stable high frequency coinciding with a decrease in local dengue transmission (85, 96, 97). The success of both suppression and replacement programs is achieved by establishing community outreach programs with communications experts and educators (98).
5.1 Incompatible insect technique or population suppression
The IIT, which is based on bidirectional/unidirectional CI if a population is uninfected (35), consists in releasing Wolbachia-infected males, inducing CI and preventing the formation of viable offspring (i.e., in CI, Wolbachia induces the death of embryonic offspring from a cross between uninfected females and infected males) (18). This approach allows the sterilization of a large number of females able to transmit pathogens and reduces the total number of insect vectors (29). According to Zheng et al. (99) and Crawford et al. (100), the goal of IIT is to suppress target mosquito populations following mass releases of Wolbachia-infected male mosquitoes able to mate with wild-type females. This technique is analogous to the SIT, which is known to be efficient in controlling vector-borne diseases. Globally, CI then leads to a decline in population size targeted by fewer mosquitoes able to spread arboviruses.
Suppression interventions require the large-scale deployment of millions of adult male mosquitoes across the country. In this technique, infected female mosquitoes are not released as they could accidentally spread Wolbachia into the targeted population. This also leads to less effective suppression as CI would not occur between the released males and females (101). Using populations infected by Wolbachia through introgression (102) and novel Wolbachia transinfections generated via microinjection (99, 103), population suppression can be achieved only through the release of Wolbachia-infected males, resulting in CI with wild females.
For population suppression interventions, the two major dengue vectors generally selected are Ae. aegypti (transfected with the wAlbB strain) and Ae. albopictus (naturally bi-infected with the native wAlbA and wAlbB strains and transfected with the wPip strain). In fact, large-scale deployment was performed in Fresno, CA, using Ae. aegypti (wAlbB), which led to a frequency above 95% of suppression of the target population, as well as a 78% decrease in the female numbers in Miami (100, 104). However, the authors did not investigate the incidence of dengue diseases, which would have allowed appreciating the success of these techniques. The same trend was observed in Guangzhou with triple-infected Ae. albopictus (wAlbAwAlbBwMel with both native wAlbA and wAlbB strains and novel wMel) (99). Recently, a transinfected Ae. aegypti population (wAlbB-Tw) in Taiwan has been shown to lead to population suppression rates reaching up to 100% in laboratory experiments and 70% in semi-field experiments (105).
Interestingly, in Singapore, large-scale deployments combining IIT/SIT with Ae. aegypti (wAlbB) have allowed reaching above 93% of suppression of the target population, with a clear impact providing a proportion varying from 71% to 88% reduction in dengue cases (106). SIT combined with IIT could certainly improve the vector control results in reducing dengue cases.
Compared with other methods that use chemicals, which also reduce insect populations, the approach known as “self-delivering” has the potential to affect certain proportions of the vector population. In fact, releasing must be continued as it is possible that a low proportion of individuals not reached by chemicals can cause a speedy population recovery after termination of insecticide applications (107). However, repeated introductions of infected males are needed to prevent the recovery of mosquito populations.
Stakeholders appreciate the fact that biting females are not released and the self-limiting nature of suppression releases (108). This strategy has a limited effect on the release area beyond crashing the target population because male mosquitoes exhibit a short longevity and cannot spread Wolbachia (108). For these authors, the impact of this approach may be temporary if the target population promptly rebounds after release, requiring repeated interventions (108). In addition, due to inaccurate sex sorting, infected fertile females could be accidentally released into the targeted areas, which could result in replacement and the failure to suppress mosquito populations (101).
Suppression interventions need the development of space-optimized rearing facilities that can produce millions of adult mosquitoes each week (109). Mitigating the establishment of Wolbachia requires the combination of IIT with other strategies such as SIT.
5.2 Replacement
The population replacement strategy involves the release of both Wolbachia-infected male and female mosquitoes that may exhibit increased resistance to the pathogen and suppress the local uninfected population through CI (29). This strategy works as a rapid self-spreading method of Wolbachia into natural populations through the release of a small number of infected mosquitoes (12). This approach was implemented for dengue prevention in two locations in Australia. The release of between 10,000 and 22,000 individuals of Wolbachia-infected Aedes mosquitoes per week for 10 weeks in 2011 has shown interesting results in terms of the dengue elimination program.
In this strategy, individual males suppress the target population through CI and females spread Wolbachia. Population replacement interventions have a proven efficacy, with the rapid spread and long-term stability of Wolbachia infection in target populations at intermediate high frequencies (27). The successful establishment of Wolbachia has generally corresponded to a significant decline in dengue transmission in endemic areas (68, 110). Once Wolbachia has reached fixation in a population, replacement interventions could provide self-sustaining protection after a single deployment period.
Interventions were conducted in Latin America, Asia, and the Pacific through the World Mosquito Program or the Wolbachia Malaysia program. These programs provided mitigation results in terms of reductions in dengue incidence. Thereby, the release of Aedes species infected with wMel led to a post-release frequency of 73% in Yogyakarta (Indonesia) (111), of 100% over 2 years in Cairns, Queensland, Australia (97), a frequency above 80% in Townsville (96), and a frequency above 60% in Rio de Janeiro (110, 112). Consequently, these factors have reduced the incidence of dengue at certain proportions. In Kuala Lumpur, Malaysia, releases of the wAlbB strain of Wolbachia reached a post-release frequency of 98% during the 12 months of the survey, leading to a 40.3% reduction in dengue incidence (68). The introgression of wMel into Ae. aegypti populations reduced the incidence of symptomatic dengue and resulted in fewer hospitalizations due to dengue among participants from Yogyakarta, Indonesia (113). In the same area, following extensive community engagement and releases of wMel-carrying mosquitoes every 2 weeks for 13–15 rounds for 7 months in 2016–2017, 34 dengue cases from the release area and 53 from the control area (incidence of 26 vs. 79 per 100,000 person-years) were estimated (111). This corresponded in the regression model to a 73% reduction in dengue incidence coupled with the Wolbachia intervention (111).
One concern is that technology requires the release of biting females to ensure spread, which could be viewed negatively by stakeholders, even if these mosquitoes are not capable of transmitting arboviruses. The need for Wolbachia-infected mosquitoes to spread and persist in the environment remains the main challenge for replacement interventions. However, high fitness costs were often observed with the loss of wMelPop infection from Ae. aegypti populations after population replacement releases in Vietnam (114).
6 Implications of natural infections on Wolbachia-based disease control strategies
Both the population replacement and suppression techniques rely on novel Wolbachia infection types that induce CI in wild-type mosquito populations.
For a successful spread, the frequency of Wolbachia infection in the population must be above a threshold level, and CI can spread Wolbachia across the population, even though infection causes non-significant costs. If the initial prevalence of adult populations (0.43) is higher than the threshold infection rate (0.4), Wolbachia infection is expected to reach fixation over the next generations (115). The frequency of infection will likely decline until Wolbachia is suppressed from the population when the threshold is not reached (116).
The success of the population replacement or the suppression strategy may be constrained by the presence of natural Wolbachia infections; therefore, potential crossing patterns between mosquitoes with novel Wolbachia infections and wild-type mosquitoes must be considered.
The high prevalence of wAlbB in natural Ae. aegypti populations in New Mexico and in infected colony obtained from wild-collected mosquitoes provided an opportunity to examine the role of Wolbachia in natural Ae. aegypti populations and to assess their interference with virus transmission (26).
With most natural infections found in wild-type populations of Ae. aegypti, the release of Wolbachia-infected male mosquitoes into an uninfected population will lead to CI. Reduced egg hatching from crosses between infected males and uninfected females favors infected females (27). The same authors also showed that putative crossing patterns between mosquitoes with novel Wolbachia infections inducing CI and mosquito populations with or without natural Wolbachia infections can lead to the possibility of four main outcomes. Subsequently, after the release of transinfected individuals, the following can occur: i) unidirectional CI via crosses between male mosquitoes with a novel Wolbachia infection and uninfected female mosquitoes; ii) no CI between novel and natural Wolbachia infections (compatible)—in this situation, population suppression is not possible; iii) bidirectional incompatibility that occurs between either males with a novel Wolbachia infection and females with natural Wolbachia infections or males with natural Wolbachia infections and females with a novel Wolbachia infection, which favor population suppression, as observed in Ae. albopictus (86); and iv) unidirectional CI may happen in favor of natural Wolbachia infection (when males from naturally infected mosquitoes mate with females with a novel Wolbachia infection) or in favor of novel infection (when males with a novel Wolbachia infection mate with females from naturally infected mosquitoes).
7 Concluding remarks
The occurrence of Wolbachia in natural populations at low or high frequencies raises the question about rethinking the Wolbachia-based control strategies. This led us to ask the following question: Does the natural occurrence of Wolbachia in Anopheles sp. and Ae. aegypti populations compromise the success of vector control strategies? In this paper, we aimed to answer this question and to propose guidelines for the development of Wolbachia-based vector control (Figure 3).
Several strains (wAnga-BF, wAnga-Mali, wPip, wAnM, and wAnD) of this endosymbiont in Anopheles populations have been reported. Among them, the wAnM and wAnD strains found in wild An. moucheti and An. demeilloni exhibit a lot of potential, suggesting their use in Wolbachia biocontrol strategies. In particular, the presence of Wolbachia cifB inducing CI provides promise in terms of vector control strategies and could contribute to reducing malaria transmission.
The occurrence of Wolbachia in Anopheles vectors does not exclude their infection by other strains known to produce CI. The transinfections of Wolbachia strains (wMelPop or wAlbB) in An. gambiae could provide significant outcomes in terms of reducing Plasmodium transmission. Figure 4 shows a synthetic view of the strains of Wolbachia transfected in mosquito populations and their impact on disease control. Laboratory transinfection of Wolbachia to Anopheles vectors of malaria was restricted to somatic tissues, and transinfection failures have generally been observed. However, previous research suggests that Anopheles disease vectors can support Wolbachia infections, which opens new opportunities for their use in disease suppression (67). Interestingly, An. stephensi mosquitoes stably infected with the wAlbB strain exhibited perfect vertical transmission, complete CI expression, strong pathogen blocking, and low fitness cost (63).
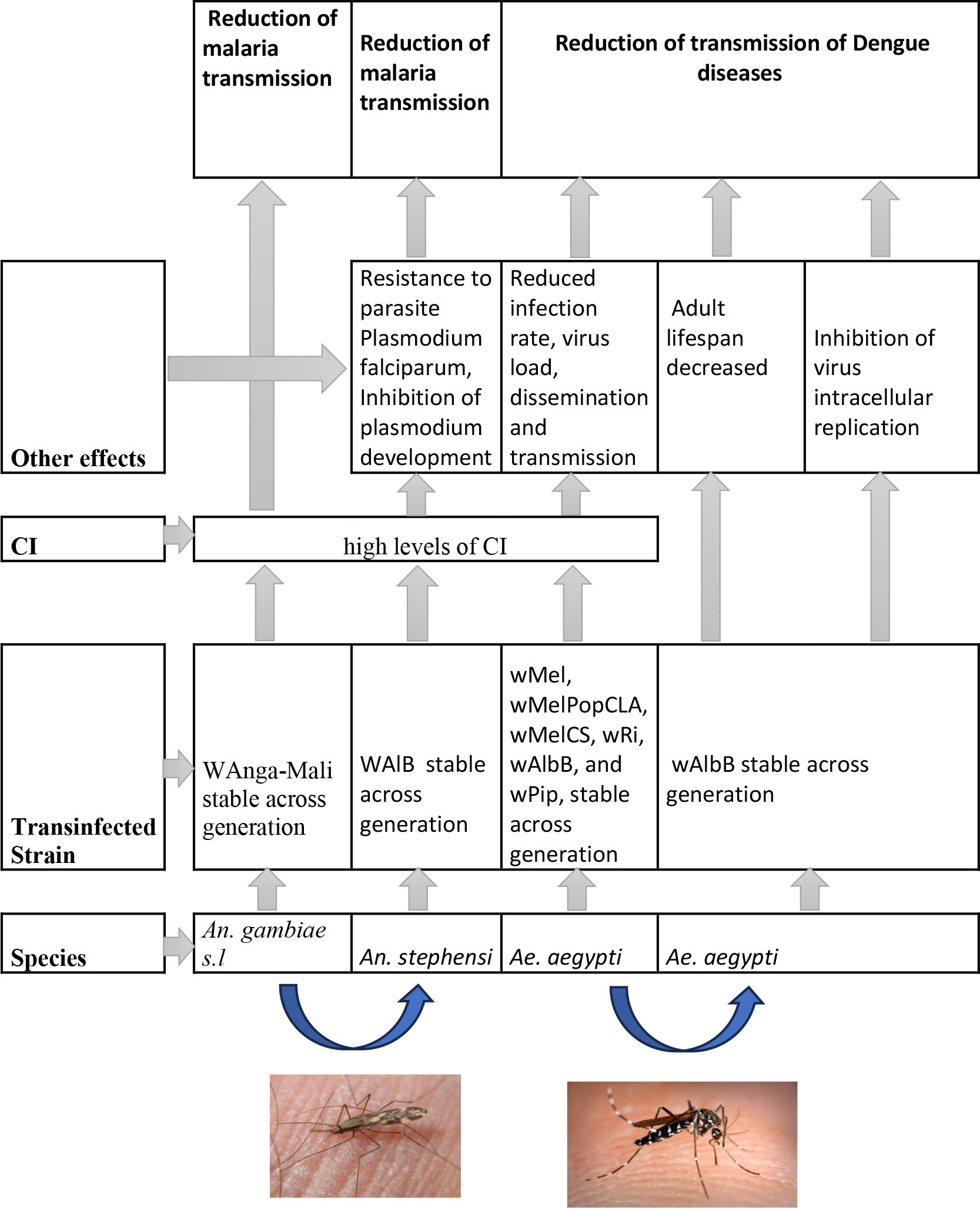
Figure 4 A synthetic view of strains of Wolbachia transinfected in mosquitoes populations and their effects on diseases control.
Moreover, the use of Wolbachia for malaria control will require creating stably infected lines of major malaria vectors, highlighting the protective effect of this bacterium against human malaria parasites such as P. falciparum and Plasmodium vivax (12, 41) (Figure 4). Finally, the release of Anopheles might be effective if further studies confirm that these Wolbachia strains are able to induce CI and express protective effects against Plasmodium species.
For the control of Aedes populations, the situation is quite different. Interestingly, following a large-scale deployment of the wAlbB strain, successful population replacement of Ae. aegypti infected with a novel Wolbachia strain has been achieved in Australia, USA, and Malaysia (68, 96, 97), coinciding with a decline in local dengue transmission.
To date, the occurrence of naturally infected Ae. aegypti requires thorough surveys for the detection of infection populations, including the choice of Wolbachia strain prior to the release of novel infections. Although most studies have reported the presence of Wolbachia in wild populations using 16S rRNA sequencing, additional studies, as described above (see Section 3.1), are needed to confirm the detection of this bacterium.
In addition to genome sequencing, the effects of natural infections (with higher prevalence) on some life history traits and vector competence must be examined as Wolbachia has useful properties that could aid in reducing virus transmission and/or decreasing population size (27).
According to Ross et al. (117), the population replacement and the suppression of Wolbachia are influenced by several factors: i) ecological effects (e.g., species composition and density in the breeding site) and the environment (i.e., temperature and competitors); ii) the Wolbachia variant (the ability to cause CI, fitness costs, and pesticide resistance); iii) disease pressure (virus incidence, serotype, and population immunity); iv) Wolbachia spread (mosquito density, the invaded area size, movement rate, and landscape structure); and v) operational issues (e.g., public engagement, quality assurance, the release technology, monitoring, and sexing, among others). These factors must be considered before the release of male-infected Wolbachia into targeted areas.
In any case, the unlikely presence of Wolbachia does not prevent the ongoing releases of this bacterium in various locations around the world, including Africa, which are aimed at reducing the transmission of vector diseases.
8 Future prospects
The prevalence and diversity of Wolbachia vary according to mosquito species. The major arboviruses vector, Ae. aegypti., is suspected to be infected by Wolbachia, while in the major malaria vector, Anopheles spp., most studies have reported their occurrence.
There is a real need for the ongoing releases to maintain control over mosquito populations. Further exploration of long-term strategies, such as genetic stability and ecological impacts, might be needed to improve the sustainability of these interventions.
In summary, future studies will consider further detailed mapping of Wolbachia strains in these two species in areas where dengue and malaria are endemic. Identifying factors such as the environmental conditions, local mosquito movement patterns (including immigration from neighboring areas with high mosquito density such as construction sites), and the nature of breeding sites need to be investigated.
Priority should be given to mosquito vectors that are the most difficult to control using the currently available methods. For this purpose, Wolbachia could be used to target outdoor-biting and outdoor-resting species that can evade insecticide-treated nets and residual insecticide sprays.
Author contributions
OG: Conceptualization, Data curation, Formal analysis, Funding acquisition, Investigation, Methodology, Project administration, Resources, Software, Supervision, Validation, Visualization, Writing – original draft, Writing – review & editing. RKD: Methodology, Project administration, Resources, Validation, Visualization, Writing – review & editing.
Funding
The author(s) declare financial support was received for the research, authorship, and/or publication of this article. Funding for this study was provided partly by the TWAS 18–163 RG/BIO/AF/AC_G – FR3240303649.
Acknowledgments
We are indebted to PODA Serge, Salifou KABRE, and DAO Zezouma for their help in drawing the figures. I also thank Casimir GNANKINE for editing this manuscript.
Conflict of interest
The authors declare that the research was conducted in the absence of any commercial or financial relationships that could be construed as a potential conflict of interest.
Publisher’s note
All claims expressed in this article are solely those of the authors and do not necessarily represent those of their affiliated organizations, or those of the publisher, the editors and the reviewers. Any product that may be evaluated in this article, or claim that may be made by its manufacturer, is not guaranteed or endorsed by the publisher.
References
1. WHO. Global plan for insect management. WHO Press, World Health Organization, 20 Avenue Appia, 1211 Geneva 27, Switzerland: World Health Organization (2012).
2. Guzman MG, Halstead SB, Artsob H, Buchy P, Farrar J, Gubler DJ, et al. Dengue: A continuing global threat. Nat Rev Microbiol. (2010) 8:S7–S16. doi: 10.1038/nrmicro2460
3. WHO. Test procedures. WHO Press, World Health Organization, 20 Avenue Appia, 1211 Geneva 27, Switzerland: World Health Organization (2013).
4. Ferguson NM. Challenges and opportunities in controlling mosquito-borne infections. Nature. (2018) 559:490–7. doi: 10.1038/s41586–018-0318–5
5. Baldacchino F, Caputo B, Chandre F, Drago A, della Torre A, Montarsi F, et al. Control methods against invasive Aedes mosquitoes in Europe: A review. Pest Manag Sci. (2015) 71:1471–85. doi: 10.1002/ps.4044
6. Benelli G, Jeffries CL, Walker T. Biological control of mosquito vectors: Past, present, and future. Insects. (2016) 7(4):52. doi: 10.3390/insects7040052
7. Balboné M, Diloma Soma D, Fogné Drabo S, Namountougou M, Konaté H, Benson Meda G, et al. Alternatives to Pyrethroid Resistance: Combinations of Cymbopogon nardus and Ocimum americanum Essential Oils Improve the Bioefficiency Control Against the Adults’ Populations of Aedes aEgypti (Diptera: Culicidae). J Med Entomol. (2022) 59:2102–9. doi: 10.1093/jme/tjac148
8. Balboné M, Sawadogo I, Soma DD, Drabo SF, Namountougou M, Bayili K, et al. Essential oils of plants and their combinations as an alternative adulticides against Anopheles Gambiae (Diptera: Culicidae) populations. Sci Rep. (2022) 12. doi: 10.1038/s41598–022-23554–6
9. Balboné M, Soma DD, Namountougou M, Drabo SF, Konaté H, Toe O, et al. Essential Oils From Five Local Plants: An Alternative Larvicide for Anopheles Gambiae s.l. (Diptera: Culicidae) and Aedes aEgypti (Diptera: Culicidae) Control in Western Burkina Faso. Front Trop Dis. (2022) 3:853405. doi: 10.3389/fitd.2022.853405
10. Balboné M, Gnankine O, Namountougou M, Soma DD, Drabo SF, Romba R, et al. The excito-repellent activity of ve essential oils extracted from local plants against dengue and malaria vectors in Burkina Faso. In press in Biologia. (2024). doi: 10.21203/rs.3.rs-2454410/v1
11. Achee NL, Grieco JP, Vatandoost H, Seixas G, Pinto J, Ching-Ng L, et al. Alternative strategies for mosquito-borne arbovirus control. PloS Negl Trop Dis. (2019) 13. doi: 10.1371/journal.pntd.0006822
12. Walker T, Moreira LA. Can Wolbachia be used to control malaria? Mem Inst Oswaldo Cruz. (2011) 106:212–7. doi: 10.1590/S0074–02762011000900026
13. Fenn K, Conlon C, Jones M, Quail MA, Holroyd NE, Parkhill J, et al. Phylogenetic relationships of the Wolbachia of nematodes and arthropods. PloS Pathog. (2006) 2:0887–99. doi: 10.1371/journal.ppat.0020094
14. Weinert LA, Araujo-Jnr EV, Ahmed MZ, Welch JJ. The incidence of bacterial endosymbionts in terrestrial arthropods. Proc R Soc B: Biol Sci. (2015) 282. doi: 10.1098/rspb.2015.0249
15. Bailly-Bechet M, Martins-Simões P, Szöllosi GJ, Mialdea G, Sagot MF, Charlat S. How long does Wolbachia remain on board? Mol Biol Evol. (2017) 34:1183–93. doi: 10.1093/molbev/msx073
16. Hurst GDD, Johnson AP, Schulenburg JHGVD, Fuyama Y. Male-killing Wolbachia in Drosophila: A temperature-sensitive trait with a threshold bacterial density. Genetics. (2000) 156(2):699–709. doi: 10.1093/genetics/156.2.699
17. Charlat S, Hurst GDD, Merçot H. Evolutionary consequences of Wolbachia infections. Trends Genet. (2003) 19:217–23. doi: 10.1016/S0168–9525(03)00024–6
18. Werren JH, Baldo L, Clark ME. Wolbachia: Master manipulators of invertebrate biology. Nat Rev Microbiol. (2008) 6:741–51. doi: 10.1038/nrmicro1969
19. Engelstädter J, Hurst GDD. The ecology and evolution of microbes that manipulate host reproduction. Annu Rev Ecol Evol Syst. (2009) 40:127–49. doi: 10.1146/annurev.ecolsys.110308.120206
20. Moriyama M, Nikoh N, Hosokawa T, Fukatsu T. Riboflavin provisioning underlies wolbachia’s fitness contribution to its insect host. mBio. (2015) 6(6):e01732-15. doi: 10.1128/mBio.01732–15
21. Hedges LM, Brownlie JC, O’Neill SL, Johnson KN. Wolbachia and virus protection in insects. Science. (2008) 322(5902):702. doi: 10.1126/science.1162418
22. Kambris Z, Cook PE, Phuc HK, Sinkins SP. Immune activation by life-shortening wolbachia and reduced filarial competence in mosquitoes. Science. (2009) 326(5949):134–6. doi: 10.1126/science.1177531
23. Rohrscheib CE, Bondy E, Josh P, Riegler M, Eyles D, van Swinderen B, et al. Wolbachia influences the production of octopamine and affects Drosophila male aggression. Appl Environ Microbiol. (2015) 81(14):4573–80. doi: 10.1128/AEM.00573–15
24. Bi J, Wang YF. The effect of the endosymbiont Wolbachia on the behavior of insect hosts. Insect Sci. (2020) 27. doi: 10.1111/1744–7917.12731
25. Ekwudu O, Devine GJ, Aaskov JG, Frentiu FD. Wolbachia strain wAlbB blocks replication of flaviviruses and alphaviruses in mosquito cell culture. Parasit Vectors. (2020) 13(1):54. doi: 10.1186/s13071–020-3936–3
26. Kulkarni A, Yu W, Jiang J, Sanchez C, Karna AK, Martinez KJL, et al. Wolbachia pipientis occurs in Aedes aEgypti populations in New Mexico and Florida, USA. Ecol Evol. (2019) 9:6148–56. doi: 10.1002/ece3.5198
27. Ross PA, Callahan AG, Yang Q, Jasper M, Arif MAK, Afizah AN, et al. An elusive endosymbiont: Does Wolbachia occur naturally in Aedes aEgypti? Ecol Evol. (2020) 10:1581–91. doi: 10.1002/ece3.6012
28. Walker T, Johnson PH, Moreira LA, Iturbe-Ormaetxe I, Frentiu FD, McMeniman CJ, et al. The wMel Wolbachia strain blocks dengue and invades caged Aedes aEgypti populations. Nature. (2011) 476:450–5. doi: 10.1038/nature10355
29. LePage D, Bordenstein SR. Wolbachia: Can we save lives with a great pandemic? Trends Parasitol. (2013) 29:385–93. doi: 10.1016/j.pt.2013.06.003
30. Lefoulon E, Clark T, Borveto F, Perriat-Sanguinet M, Moulia C, Slatko BE, et al. Pseudoscorpion Wolbachia symbionts: Diversity and evidence for a new supergroup S. BMC Microbiol. (2020) 20. doi: 10.1186/s12866-020-01863-y
31. Sanaei E, Charlat S, Engelstädter J. Wolbachia host shifts: routes, mechanisms, constraints and evolutionary consequences. Biol Rev. (2021) 96:433–53. doi: 10.1111/brv.12663
32. Moreira LA, Iturbe-Ormaetxe I, Jeffery JA, Lu G, Pyke AT, Hedges LM, et al. A Wolbachia symbiont in Aedes aEgypti limits infection with dengue, chikungunya, and plasmodium. Cell. (2009) 139:1268–78. doi: 10.1016/j.cell.2009.11.042
33. Iturbe-Ormaetxe I, Walker T, O’Neill SL. Wolbachia and the biological control of mosquito-borne disease. EMBO Rep. (2011) 12. doi: 10.1038/embor.2011.84
34. Baldini F, Segata N, Pompon J, Marcenac P, Robert Shaw W, Dabiré RK, et al. Evidence of natural Wolbachia infections in field populations of Anopheles Gambiae. Nat Commun. (2014) 5:3985. doi: 10.1038/ncomms4985
35. Bourtzis K, Dobson SL, Xi Z, Rasgon JL, Calvitti M, Moreira LA, et al. Harnessing mosquito-Wolbachia symbiosis for vector and disease control. Acta Trop. (2014) 132(Suppl):S150–63. doi: 10.1016/j.actatropica.2013.11.004
36. Walker T, Quek S, Jeffries CL, Bandibabone J, Dhokiya V, Bamou R, et al. Stable high-density and maternally inherited Wolbachia infections in Anopheles moucheti and Anopheles demeilloni mosquitoes. Curr Biol. (2021) 31:2310–2320.e5. doi: 10.1016/j.cub.2021.03.056
37. Baldini F, Rougé J, Kreppel K, Mkandawile G, Mapua SA, Sikulu-Lord M, et al. First report of natural Wolbachia infection in the malaria mosquito Anopheles arabiensis in Tanzania. Parasit Vectors. (2018) 11:635. doi: 10.1186/s13071-018-3249-y
38. Sawasdichai S, Chaumeau V, Dah T, Kulabkeeree T, Kajeechiwa L, Phanaphadungtham M, et al. Detection of diverse wolbachia 16s rrna sequences at low titers from malaria vectors in kayin state, Myanmar [version 4; peer review: 2 approved, 1 approved with reservations]. Wellcome Open Res. (2019) 4. doi: 10.12688/wellcomeopenres.15005.1
39. Waymire E, Duddu S, Yared S, Getachew D, Dengela D, Bordenstein SR, et al. Wolbachia 16S rRNA haplotypes detected in wild Anopheles stephensi in eastern Ethiopia. Parasit Vectors. (2022) 15(178). doi: 10.1186/s13071–022-05293–9
40. Niang EHA, Bassene H, Makoundou P, Fenollar F, Weill M, Mediannikov O. First report of natural Wolbachia infection in wild Anopheles funestus population in Senegal. Malar J. (2018) 17:408. doi: 10.1186/s12936-018-2559-z
41. Gomes FM, Hixson BL, Tyner MDW, Ramirez JL, Canepa GE, Alves e Silva TL, et al. Effect of naturally occurring Wolbachia in Anopheles Gambiae s.l. mosquitoes from Mali on Plasmodium falciparum malaria transmission. Proc Natl Acad Sci U.S.A. (2017) 114:12566–71. doi: 10.1073/pnas.1716181114
42. Jeffries CL, Lawrence GG, Golovko G, Kristan M, Orsborne J, Spence K, et al. Novel Wolbachia strains in Anopheles malaria vectors from Sub-Saharan Africa. Wellcome Open Res. (2018) 3:113. doi: 10.12688/wellcomeopenres.14765.1
43. Ayala D, Akone-Ella O, Rahola N, Kengne P, Ngangue MF, Mezeme F, et al. Natural Wolbachia infections are common in the major malaria vectors in Central Africa. Evol Appl. (2019) 12:1583–94. doi: 10.1111/eva.12804
44. Wong ML, Liew JWK, Wong WK, Pramasivan S, Mohamed Hassan N, Wan Sulaiman WY, et al. Natural Wolbachia infection in field-collected Anopheles and other mosquito species from Malaysia. Parasit Vectors. (2020) 13(1):414. doi: 10.1186/s13071-020-04277-x
45. Chrostek E, Gerth M. Is anopheles Gambiae a natural host of wolbachia? mBio. (2019) 10. doi: 10.1128/mBio.00784–19
46. Hughes GL, Rasgon JL. Transinfection: A method to investigate Wolbachia-host interactions and control arthropod-borne disease. Insect Mol Biol. (2014) 23:141–51. doi: 10.1111/imb.12066
47. Straub TJ, Shaw WR, Marcenac P, Sawadogo SP, Dabiré RK, Diabaté A, et al. The Anopheles coluzzii microbiome and its interaction with the intracellular parasite Wolbachia. Sci Rep. (2020) 10:13847. doi: 10.1038/s41598–020-70745–0
48. Hughes GL, Dodson BL, Johnson RM, Murdock CC, Tsujimoto H, Suzuki Y, et al. Native microbiome impedes vertical transmission of Wolbachia in Anopheles mosquitoes. Proc Natl Acad Sci U.S.A. (2014) 111:12498–503. doi: 10.1073/pnas.1408888111
49. Rossi P, Ricci I, Cappelli A, Damiani C, Ulissi U, Mancini MV, et al. Mutual exclusion of Asaia and Wolbachia in the reproductive organs of mosquito vectors. Parasit Vectors. (2015) 8:278. doi: 10.1186/s13071–015-0888–0
50. Gomes FM, Barillas-Mury C. Infection of anopheline mosquitoes with Wolbachia: implications for malaria control. PLoS Pathog. (2018) 14(11):e1007333. doi: 10.1371/journal.ppat.1007333
51. Caragata EP, Rancès E, Hedges LM, Gofton AW, Johnson KN, O’Neill SL, et al. Dietary cholesterol modulates pathogen blocking by Wolbachia. PloS Pathog. (2013) 9:e1003459. doi: 10.1371/journal.ppat.1003459
52. Gueguen G, Vavre F, Gnankine O, Peterschmitt M, Charif D, Chiel E, et al. Endosymbiont metacommunities, mtDNA diversity and the evolution of the Bemisia tabaci (Hemiptera: Aleyrodidae) species complex. Mol Ecol. (2010) 19:4365–76. doi: 10.1111/mec.2010.19.issue-19
53. Gnankiné O, Mouton L, Henri H, Terraz G, Houndeté T, Martin T, et al. Distribution of Bemisia tabaci (Homoptera: Aleyrodidae) biotypes and their associated symbiotic bacteria on host plants in West Africa. Insect Conserv Divers. (2013) 6:411–21. doi: 10.1111/j.1752-4598.2012.00206.x
54. Gowri Sankar S, Sundari TM, Prem Anand AA. Low prevalence of natural Wolbachia in major malarial vectors Anopheles culicifacies s. (2021). doi: 10.1101/2020.11.22.393652
55. Jeffries CL, Cansado-Utrilla C, Beavogui AH, Stica C, Lama EK, Kristan M, et al. Evidence for natural hybridization and novel Wolbachia strain superinfections in the Anopheles Gambiae complex from Guinea. R Soc Open Sci. (2021) 8. doi: 10.1098/rsos.202032
56. Shaw WR, Marcenac P, Childs LM, Buckee CO, Baldini F, Sawadogo SP, et al. Wolbachia infections in natural Anopheles populations affect egg laying and negatively correlate with Plasmodium development. Nat Commun. (2016) 7:11772. doi: 10.1038/ncomms11772
57. Jeffries CL, Tantely LM, Raharimalala FN, Hurn E, Boyer S, Walker T. Diverse novel resident Wolbachia strains in Culicine mosquitoes from Madagascar. Sci Rep. (2018) 8:17456. doi: 10.1038/s41598-018-35658-z
58. Adams KL, Abernathy DG, Willett BC, Selland EK, Itoe MA, Catteruccia F. Wolbachia cifB induces cytoplasmic incompatibility in the malaria mosquito vector. Nat Microbiol. (2021) (12):1575–82. doi: 10.1038/s41564-021-00998-6
59. Hemingway J, Ranson H, Magill A, Kolaczinski J, Fornadel C, Gimnig J, et al. Averting a malaria disaster: Will insecticide resistance derail malaria control? Lancet. (2016) 387:1785–8. doi: 10.1016/S0140–6736(15)00417–1
60. Sawadogo SP, Kabore DA, Tibiri EB, Hughes A, Gnankine O, Quek S, et al. Lack of robust evidence for a Wolbachia infection in Anopheles Gambiae from Burkina Faso. Med Vet Entomol. (2022) 36:301–8. doi: 10.1111/mve.12601
61. Berticat C, Rousset F, Raymond M, Berthomieu A, Weill M. High Wolbachia density in insecticide-resistant mosquitoes. Proc R Soc B: Biol Sci. (2002) 269:1413–6. doi: 10.1098/rspb.2002.2022
62. Echaubard P, Duron O, Agnew P, Sidobre C, Noël V, Weill M, et al. Rapid evolution of Wolbachia density in insecticide resistant Culex pipiens. Heredity (Edinb). (2010) 104:15–9. doi: 10.1038/hdy.2009.100
63. Bian G, Joshi D. Wolbachia invades anopheles stephensi populations and induces refractoriness to plasmodium infection. Science. (2013) 340(6133):748–51. doi: 10.1126/science.1236192
64. Hughes GL, Koga R, Xue P, Fukatsu T, Rasgon JL. Wolbachia infections are virulent and inhibit the human malaria parasite Plasmodium falciparum in Anopheles Gambiae. PloS Pathog. (2011) 7(5):e1002043. doi: 10.1371/journal.ppat.1002043
65. Zélé F, Nicot A, Berthomieu A, Weill M, Duron O, Rivero A. Wolbachia increases susceptibility to Plasmodium infection in a natural system. Proc R Soc B: Biol Sci. (2014) 281(1779):20132837. doi: 10.1098/rspb.2013.2837
66. Kambris Z, Blagborough AM, Pinto SB, Blagrove MSC, Godfray HCJ, Sinden RE, et al. Wolbachia stimulates immune gene expression and inhibits plasmodium development in anopheles Gambiae. PloS Pathog. (2010) 6(10):e1001143. doi: 10.1371/journal.ppat.1001143
67. Ross PA, Hoffmann AA. Vector control: Discovery of Wolbachia in malaria vectors. Curr Biol. (2021) 31:R738–40. doi: 10.1016/j.cub.2021.04.038
68. Nazni WA, Hoffmann AA, NoorAfizah A, Cheong YL, Mancini MV, Golding N, et al. Establishment of Wolbachia Strain wAlbB in Malaysian Populations of Aedes aEgypti for Dengue Control. Curr Biol. (2019) 29:4241–4248.e5. doi: 10.1016/j.cub.2019.11.007
69. Joshi D, McFadden MJ, Bevins D, Zhang F, Xi Z. Wolbachia strain wAlbB confers both fitness costs and benefit on Anopheles stephensi. Parasit Vectors. (2014) 7:336. doi: 10.1186/1756–3305-7–336
70. Hughes GL, Rivero A, Rasgon JL. Wolbachia can enhance plasmodium infection in mosquitoes: implications for malaria control? PloS Pathog. (2014) 10(9):e1004182. doi: 10.1371/journal.ppat.1004182
71. Coon KL, Brown MR, Strand MR. Mosquitoes host communities of bacteria that are essential for development but vary greatly between local habitats. Mol Ecol. (2016) 25:5806–26. doi: 10.1111/mec.13877
72. Teo CHJ, Lim PKC, And Mak K. Detection of dengue viruses and Wolbachia in Aedes aEgypti and Aedes albopictus larvae from four urban localities in Kuala Lumpur, Malaysia (2017). Available online at: http://www.sanofipasteur.com/en/.
73. Thongsripong P, Chandler JA, Green AB, Kittayapong P, Wilcox BA, Kapan DD, et al. Mosquito vector-associated microbiota: Metabarcoding bacteria and eukaryotic symbionts across habitat types in Thailand endemic for dengue and other arthropod-borne diseases. Ecol Evol. (2018) 8:1352–68. doi: 10.1002/ece3.3676
74. Carvajal TM, Hashimoto K, Harnandika RK, Amalin DM, Watanabe K. Detection of Wolbachia in field-collected Aedes aEgypti mosquitoes in metropolitan Manila, Philippines. Parasit Vectors. (2019) 12:361. doi: 10.1186/s13071-019-3629-y
75. Balaji S, Jayachandran S, Prabagaran SR. Evidence for the natural occurrence of Wolbachia in Aedes aEgypti mosquitoes. FEMS Microbiol Lett. (2019) 366. doi: 10.1093/femsle/fnz055
76. Bennett KL, Gómez-Martínez C, Chin Y, Saltonstall K, McMillan WO, Rovira JR, et al. Dynamics and diversity of bacteria associated with the disease vectors Aedes aEgypti and Aedes albopictus. Sci Rep. (2019) 9. doi: 10.1038/s41598–019-48414–8
77. Hegde S, Khanipov K, Albayrak L, Golovko G, Pimenova M, Saldaña MA, et al. Microbiome interaction networks and community structure from laboratory-reared and field-collected Aedes aEgypti, Aedes albopictus, and Culex quinquefasciatus mosquito vectors. Front Microbiol. (2018) 9:2160. doi: 10.3389/fmicb.2018.02160
78. Vinayagam S, Nirmolia T, Chetry S, Kumar NP, Saini P, Bhattacharyya DR, et al. Molecular Evidence of Wolbachia Species in Wild-Caught Aedes albopictus and Aedes aEgypti Mosquitoes in Four States of Northeast India. J Trop Med. (2023) 2023:6678627. doi: 10.1155/2023/6678627
79. Leta S, Beyene TJ, De Clercq EM, Amenu K, Kraemer MUG, Revie CW. Global risk mapping for major diseases transmitted by Aedes aEgypti and Aedes albopictus. Int J Infect Dis. (2018) 67:25–35. doi: 10.1016/j.ijid.2017.11.026
80. Ryan SJ, Carlson CJ, Mordecai EA, Johnson LR. Global expansion and redistribution of Aedes-borne virus transmission risk with climate change. PloS Negl Trop Dis. (2018) 13(3):e0007213. doi: 10.1371/journal.pntd.0007213
81. Xi Z, Khoo CCH, Dobson SL. Ecology: Wolbachia establishment and invasion in an Aedes AEgypti laboratory population. Sci (1979). (2005) 310:326–8. doi: 10.1126/science.1117607
82. McMeniman CJ, Lane RV, Cass BN, Fong AWC, Sidhu M, Wang Y-F, et al. Stable Introduction of a Life-Shortening Wolbachia Infection into the Mosquito Aedes aEgypti. Sci (1979). (2009) 323:141–4. doi: 10.1126/science.1165326
83. Fraser JE, De Bruyne JT, Iturbe-Ormaetxe I, Stepnell J, Burns RL, Flores HA, et al. Novel Wolbachia-transinfected Aedes aEgypti mosquitoes possess diverse fitness and vector competence phenotypes. PloS Pathog. (2017) 13(12):e1006751. doi: 10.1371/journal.ppat.1006751
84. Ant TH, Herd CS, Geoghegan V, Hoffmann AA, Sinkins SP. The Wolbachia strain wAu provides highly efficient virus transmission blocking in Aedes aEgypti. PloS Pathog. (2018) 14(1):e1006815. doi: 10.1371/journal.ppat.1006815
85. Hoffmann AA, Montgomery BL, Popovici J, Iturbe-Ormaetxe I, Johnson PH, Muzzi F, et al. Successful establishment of Wolbachia in Aedes populations to suppress dengue transmission. Nature. (2011) 476:454–9. doi: 10.1038/nature10356
86. Calvitti M, Moretti R, Lampazzi E, Bellini R, Dobson SL. Characterization of a new Aedes albopictus (Diptera: Culicidae)-Wolbachia pipientis (Rickettsiales: Rickettsiaceae) symbiotic association generated by artificial transfer of the wPip strain from Culex pipiens (Diptera: Culicida). J Med Entomol. (2010) 47:179–87. doi: 10.1603/ME09140
87. Blagrove MSC, Arias-Goeta C, Failloux AB, Sinkins SP. Wolbachia strain wMel induces cytoplasmic incompatibility and blocks dengue transmission in Aedes albopictus. Proc Natl Acad Sci U.S.A. (2012) 109:255–60. doi: 10.1073/pnas.1112021108
88. Dutra HLC, Rocha MN, Dias FBS, Mansur SB, Caragata EP, Moreira LA. Wolbachia blocks currently circulating zika virus isolates in Brazilian aedes aEgypti mosquitoes. Cell Host Microbe. (2016) 19:771–4. doi: 10.1016/j.chom.2016.04.021
89. Bian G, Xu Y, Lu P, Xie Y, Xi Z. The endosymbiotic bacterium Wolbachia induces resistance to dengue virus in Aedes aEgypti. PloS Pathog. (2010) 6:1–10. doi: 10.1371/journal.ppat.1000833
90. van den Hurk AF, Hall-Mendelin S, Pyke AT, Frentiu FD, McElroy K, Day A, et al. Impact of Wolbachia on infection with chikungunya and yellow fever viruses in the mosquito vector aedes aEgypti. PloS Negl Trop Dis. (2012) 6(11):e1892. doi: 10.1371/journal.pntd.0001892
91. Alkuriji MA, Al-Fageeh MB, Shaher FM, Almutairi BF. Dengue vector control: A review for Wolbachia-based strategies. Biosci Biotechnol Res Asia. (2020) 17:507–15. doi: 10.13005/bbra/2854
92. Hussain M, Lu G, Torres S, Edmonds JH, Kay BH, Khromykh AA, et al. Effect of Wolbachia on replication of west nile virus in a mosquito cell line and adult mosquitoes. J Virol. (2013) 87:851–8. doi: 10.1128/jvi.01837–12
93. Carrington LB, Tran BCN, Le NTH, Luong TTH, Nguyen TT, Nguyen PT, et al. Field- and clinically derived estimates of Wolbachia-mediated blocking of dengue virus transmission potential in Aedes aEgypti mosquitoes. Proc Natl Acad Sci U.S.A. (2017) 115:361–6. doi: 10.1073/pnas.1715788115
94. Flores HA, Taneja de Bruyne J, O’Donnell TB, Tuyet Nhu V, Thi Giang N, Thi Xuan Trang H, et al. Multiple Wolbachia strains provide comparative levels of protection against dengue virus infection in Aedes aEgypti. PloS Pathog. (2020) 16:e1008433. doi: 10.1371/journal.ppat.1008433
95. Edenborough KM, Flores HA, Simmons CP, Fraser JE. Using Wolbachia to eliminate dengue: will the virus fight back? J Virol. (2021) 95:e02203-20. doi: 10.1128/JVI.02203-20
96. O’Neill SL, Ryan PA, Turley AP, Wilson G, Retzki K, Iturbe-Ormaetxe I, et al. Scaled deployment of Wolbachia to protect the community from dengue and other aedes transmitted arboviruses. Gates Open Res. (2018) 2. doi: 10.12688/gatesopenres.12844.3
97. Ryan PA, Turley AP, Wilson G, Hurst TP, Retzki K, Brown-Kenyon J, et al. Establishment of wMel Wolbachia in Aedes aEgypti mosquitoes and reduction of local dengue transmission in Cairns and surrounding locations in northern Queensland, Australia. Gates Open Res. (2020) 3:1547. doi: 10.12688/gatesopenres.13061.2
98. Moreira LA, Costa GB, Smithyman R, O’Neill SL. How to engage communities on a large scale? Lessons from World Mosquito Program in Rio de Janeiro, Brazil. Gates Open Res. (2020) 4. doi: 10.12688/gatesopenres.13153.1
99. Zheng X, Zhang D, Li Y, Yang C, Wu Y, Liang X, et al. Incompatible and sterile insect techniques combined eliminate mosquitoes. Nature. (2019) 572:56–61. doi: 10.1038/s41586–019-1407–9
100. Crawford JE, Clarke DW, Criswell V, Desnoyer M, Cornel D, Deegan B, et al. Efficient production of male Wolbachia-infected Aedes aEgypti mosquitoes enables large-scale suppression of wild populations. Nat Biotechnol. (2020) 38:482–92. doi: 10.1038/s41587-020-0471-x
101. Soh S, Ho SH, Ong J, Seah A, Dickens BS, Tan KW, et al. Strategies to mitigate establishment using the Wolbachia incompatible insect technique. Viruses. (2022) 14(6):1132. doi: 10.3390/v14061132
102. O’Connor L, Plichart C, Sang AC, Brelsfoard CL, Bossin HC, Dobson SL. Open release of male mosquitoes infected with a Wolbachia biopesticide: field performance and infection containment. PloS Negl Trop Dis. (2012) 6(11):e1797. doi: 10.1371/journal.pntd.0001797
103. Mains JW, Brelsfoard CL, Rose RI, Dobson SL. Female adult aedes Albopictus suppression by Wolbachia-infected male mosquitoes. Sci Rep. (2016) 6:33846. doi: 10.1038/srep33846
104. Mains JW, Kelly PH, Dobson KL, Petrie WD, Dobson SL. Localized Control of Aedes aEgypti (Diptera: Culicidae) in Miami, FL, via Inundative Releases of Wolbachia-Infected Male Mosquitoes. J Med Entomol. (2019) 56:1296–303. doi: 10.1093/jme/tjz051
105. Liu WL, Yund HY, Chen YX, Chen BY, Ning Leaw S, Linm CH, et al. Lab-scale characterization and semi-field trials of Wolbachia Strain wAlbB in a Taiwan Wolbachia introgressed Ae. aEgypti strain. PloS Negl Trop Dis. (2022) 16(1):e0010084. doi: 10.1371/journal.pntd.0010084
106. Project Wolbachia – Singapore Consortium. Wolbachia-mediated sterility suppresses Aedes aEgypti populations in the urban tropics. (2021). doi: 10.1101/2021.06.16.21257922
107. Slatko BE, Taylor MJ, Foster JM. The Wolbachia endosymbiont as an anti-filarial nematode target. Symbiosis. (2010) 51:55–65. doi: 10.1007/s13199–010-0067–1
108. Caragata EP, Dutra HLC, Sucupira PHF, Ferreira AGA, Moreira LA. Wolbachia as translational science: controlling mosquito-borne pathogens. Trends Parasitol. (2021) 37:1050–67. doi: 10.1016/j.pt.2021.06.007
109. Zhang D, Li Y, Sun Q, Zheng X, Gilles JRL, Yamada H, et al. Establishment of a medium-scale mosquito facility: Tests on mass production cages for Aedes albopictus (Diptera: Culicidae). Parasit Vectors. (2018) 11:189. doi: 10.1186/s13071–018-2750–7
110. Pinto SB, Riback TIS, Sylvestre G, Costa G, Peixoto J, Dias FBS, et al. Effectiveness of wolbachia-infected mosquito deployments in reducing the incidence of dengue and other aedes-borne diseases in niterói, Brazil: A quasi-experimental study. PloS Negl Trop Dis. (2021) 15(7):e0009556. doi: 10.1371/journal.pntd.0009556
111. Anders KL, Durovni B, Saraceni V, Eppinghaus A, Riback TIS, Moreira LA, et al. The impact of large-scale deployment of Wolbachia mosquitoes on dengue and other Aedes-borne diseases in Rio de Janeiro and Niterói, Brazil: Study protocol for a controlled interrupted time series analysis using routine disease surveillance data. F1000Res. (2020) 8. doi: 10.12688/f1000research.19859.2
112. Garcia G, de A, Sylvestre G, Aguiar R, da Costa GB, Martins AJ, et al. Matching the genetics of released and local Aedes aEgypti populations is critical to assure Wolbachia invasion. PloS Negl Trop Dis. (2019) 13(1):e0007023. doi: 10.1371/journal.pntd.0007023
113. Utarini A, Indriani C, Ahmad RA, Tantowijoyo W, Arguni E, Ansari MR, et al. Efficacy of Wolbachia-infected mosquito deployments for the control of dengue. New Engl J Med. (2021) 384:2177–86. doi: 10.1056/nejmoa2030243
114. Nguyen TH, Nguyen H, Nguyen TY, Vu SN, Tran ND, Le TN, et al. Field evaluation of the establishment potential of wmelpop Wolbachia in Australia and Vietnam for dengue control. Parasit Vectors. (2015) 8:563. doi: 10.1186/s13071-015-1174-x
115. Turelli M. Cytoplasmic incompatibility in populations with overlapping generations. Evol (N Y). (2010) 64:232–41. doi: 10.1111/evo.2010.64.issue-1
116. O’Neill S, Hoffmann AA, Werren JH. Influential passengers: Inherited microorganisms and arthropod reproduction. In: . Oxford University Press (1997). doi: 10.1093/oso/9780198577867.001.0001
Keywords: Wolbachia, Aedes aegypti, Anopheles sp. prevalence, cytoplasmic incompatibility, vector control
Citation: Gnankine O and Dabiré RK (2024) Natural occurrence of Wolbachia in Anopheles sp. and Aedes aegypti populations could compromise the success of vector control strategies. Front. Trop. Dis 5:1329015. doi: 10.3389/fitd.2024.1329015
Received: 27 October 2023; Accepted: 02 May 2024;
Published: 22 July 2024.
Edited by:
Jatin Shrinet, Florida State University, United StatesReviewed by:
Priyanshu Srivastava, University of Texas MD Anderson Cancer Center, United StatesRaja Babu Singh Kushwah, Texas A and M University, United States
Copyright © 2024 Gnankine and Dabiré. This is an open-access article distributed under the terms of the Creative Commons Attribution License (CC BY). The use, distribution or reproduction in other forums is permitted, provided the original author(s) and the copyright owner(s) are credited and that the original publication in this journal is cited, in accordance with accepted academic practice. No use, distribution or reproduction is permitted which does not comply with these terms.
*Correspondence: Olivier Gnankine, b2xpZ25hbmtpbmVAZ21haWwuY29t; b2xpdmllci5nbmFua2luZUB1amt6LmJm