- 1Department of Microbiology/Immunology, Center for Cancer Cell Biology, Immunology and Infection, Chicago Medical School, Rosalind Franklin University, North Chicago, IL, United States
- 2Department of Chemistry, The Chinese University of Hong Kong, Hong Kong, Hong Kong SAR, China
- 3Department of Molecular Parasitology and Tropical Diseases, School of Medicine, College of Medicine, Taipei Medical University, Taipei, Taiwan
Singlet oxygen (1O2) is a potent biocide potentially deployable for integrated control of tropical diseases and their insect vectors. This very short-lived free radical is highly destructive of cellular molecules when generated intracellularly. Most organisms, including parasites and vectors, are defenseless against 1O2 except for plants, which produce it abundantly during photosynthesis, hence, the acquisition of specific mechanisms for its detoxification. In the presence of O2 under physiological conditions, certain dyes or photosensitizers (PS), e.g., porphyrins and phthalocyanines (PC), are excitable by light to produce biocidal 1O2. Its half-life is in the order of microseconds, necessitating its intracellular generation in order to harness its biocidal activity most effectively. This is achievable by loading cells with PS for excitation with light to produce 1O2 in situ. One example to achieve this is the genetic engineering of Leishmania to complement its inherent defects in porphyrin biosynthesis, resulting in cytosolic accumulation of abundant PS in the form of uroporphyrin 1 (URO). Another example is the chemical engineering of PC for hydrophilicity, thereby facilitating the endocytosis of such PS by cells. Leishmania loaded with cytosolic URO and endosomal PC are inactivated by the 1O2 produced via light-activation of these PS in the two different cell compartments. The inactivated Leishmania are nonviable, but have their natural vaccines and adjuvants well-preserved for prophylactic vaccination against experimental leishmaniasis. 1O2-inactivated Leishmania is potentially useful to serve as a platform for the safe and effective delivery of transgenically add-on vaccines against malignant and viral diseases in experimental models. Hydrophilic and cationic PC were also shown experimentally to act as a new type of dim light-activable insecticides, i.e., their mosquito larvicidal activities with <µM LD50 values. Similar results are expected by studying PC in additional laboratory insect models. A significant advantage has long been attributed to this type of insecticide, i.e., their aversion to a selection of genetic variants for resistance. An additional advantage of PC is their excitability to produce insecticidal 1O2 with deep-penetrating red or infrared light invisible to most insects, thereby potentially increasing the range and scope of targetable insect vectors.
1 Introduction
Singlet oxygen (1O2) is known to form when photosensitive compounds or photosensitizers (PS) are excited in solutions by light in the presence of atmospheric oxygen. Since the first discovery of 1O2 in the late 1930s (1), it has been chemically characterized as a highly reactive, but extremely short-lived free radical with a half-life in the order of microseconds. Photosensitizers are naturally occurring or synthetic ring compounds of colored substances, i.e., dyes and pigments, many of which are excitable by light optimally at specific wavelengths to produce 1O2.
The biology of 1O2 is of interest in divergent disciplinary areas. It is known to play a role in signal pathways to regulate gene expression, for example, during photosynthesis of plant cells (2, 3). Cytotoxicity of 1O2 contributes to the clinical pathology of some human diseases. The best known is perhaps porphyria due to dysregulation of heme biosynthesis leading to the overproduction of photosensitive porphyrins (4). There are also light-induced, age-related human degenerative ailments, e.g., macula degeneration of eyes and skin damage (5). Significantly, the highly destructive force of 1O2 has been exploited clinically for photodynamic therapy (PDT) of certain skin diseases and tumors (6, 7), and has been explored for use as germicidal agents (8, 9) and pesticides (10).
The focus of our attention here is 1O2-mediated inactivation of parasites and vectors. This subject has been included in previous reviews, which broadly cover all cytotoxic reactive oxygen species (ROS) produced by dye activation with light to control tropical diseases (11, 12). There are more recent articles on the generation of 1O2 as biocides, pertaining, for example, to the use of rose Bengal (13, 14) and curcumin (15–17) as the PS for light-activated tumoricidal, antimicrobial, and/or larvicidal activities at the organismal level. Here, the generation and cytotoxicity of 1O2 are delineated at cellular and molecular levels with particular reference to porphyrin- and phthalocyanine (PC)-mediated photo-inactivation of unicellular eukaryotic protozoan parasites, i.e., flagellated promastigotes of the Leishmania spp. This writing is thus not an exhaustive review of literature but intended to be a conceptual one devoted specifically to the biotechnology to produce 1O2 in relation to its biocidal efficacy and aversion to resistance (18, 19). Also discussed are the potential utility of 1O2-inactivated Leishmania cells for prophylactic/therapeutic vaccination and the utility of PC as novel light-activated insecticides. The significance of deploying biocidal 1O2 is discussed along with its potential as a new strategy to control neglected diseases.
2 Susceptibility of non-photosynthetic cells to inactivation by 1O2
It is known that the output of 1O2 is a function of PS concentrations and light intensities under normal atmospheric conditions of air with ~20% O2, although the rapid emergence and dissipation of 1O2 in microseconds make it difficult to measure in complicated biological systems. 1O2 is produced in abundance when porphyrin-loaded promastigotes are illuminated with dim light during routine observation under phase contrast microscopy, resulting in a sudden cessation of their flagellar motility as a visible readout of cell inactivation (20). The use of singlet oxygen sensor green (SOSG) showed an elevation of 1O2 levels to a large magnitude over the baseline in the controls, but this reagent is impervious to live cells and gives only crude relative values. The generation of 1O2 in trace amounts may be inferred to occur via light excitation of endogenous natural cellular metabolites to serve as the PS, such as flavin adenine dinucleotide (FAD), flavin mononucleotide (FMN, riboflavin or vitamin B2) and pterins (21) (Figure 1A). Its occurrence as a general phenomenon with functional significance remains hypothetical (22). It is clear however that most cells are not endowed with specific mechanisms to detoxify the sudden emergence of abundant 1O2, except those undergoing photosynthesis (Figure 1B). Light-mediated generation of energy in photosynthesis is well-documented to produce abundant 1O2 with chlorophyll and its intermediates to serve as the PS (2, 3). Photosynthetic cells have thus evolved a specific mechanism for quenching 1O2 as soon as it emerges by deploying abundant, multifarious, and strategically placed scavengers, i.e., ascorbate, quinols, tocopherols, and carotenoids (2, 3, 23, 24). In addition, 1O2-carotenoid complex is known to provide the signal for a retrograde pathway to dampen photosynthesis (2, 3). Clearly, 1O2 is suitable to deploy as a potent biocide against non-photosynthetic parasites and vectors.
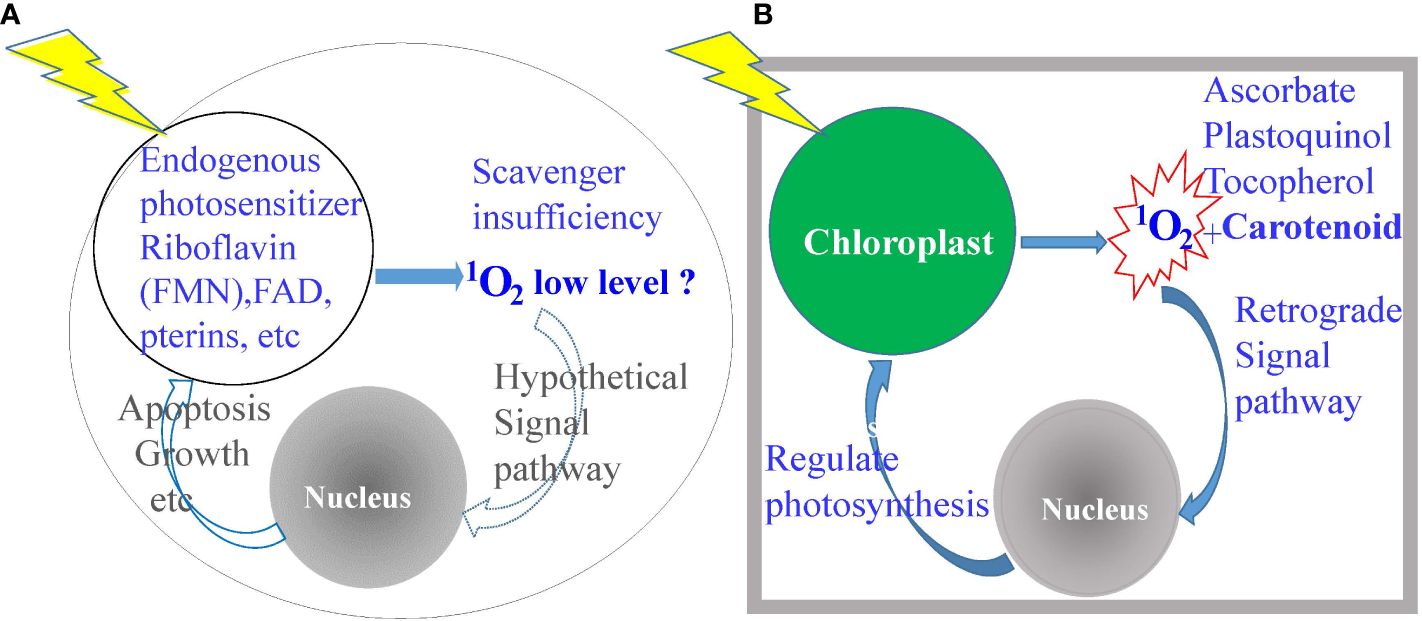
Figure 1 Diagrammatic depiction of the significant differences between photosynthetic versus non-photosynthetic cells in the elaboration and disposition of singlet oxygen (1O2). (A) Non-photosynthetic cells produce very little, if any, 1O2, hence, ill-equipped to neutralize its cytotoxicity. (B) Photosynthetic cells produce a copious amount of 1O2, hence, the possession of abundant scavengers for detoxification and for a signal to downregulate photosynthesis.
3 Transient lifespan of 1O2 necessitates its intracellular generation to optimize its biocidal activities
This is indicated by the observation that Leishmania are extremely sensitive to 1O2 when generated in its cytosol by loading them with uroporphyrin 1 followed by exposure to light of very low intensity (20). In contrast, these cells remain completely normal in appearance and totally unchanged in motility and viability when exposed to 1O2 produced abundantly in their extracellular milieu, e.g., 1-mM uroporphyrin I in PBS excited with longwave UV light. This is consistent with the general knowledge that 1O2 formed in the fluid phase dissipates quickly in microseconds, giving it insufficient time to travel across the plasma membrane of cells (25) to cause intracellular damage (Figure 2A). Additional factors may account for the indifference of Leishmania to extracellular 1O2: (A) Leishmania possess a layer of surface carbohydrates, which are 1O2-inert or -unreactive, thereby providing an additional protective barrier and (B) uroporphyrin 1 is an uncharged molecule and thus, not cell-associated with the plasma membrane. These additional factors further hamper the entry of extracellularly generated 1O2 into Leishmania cells. Positively charged PS, such as protoporphyrin XI, are known to become associated with negatively charged plasma membranes, thereby sensitizing cells to light for generating 1O2 to cause membrane damage. Clearly, the destruction is far more global by the 1O2 that is generated by light exposure of uroporphyrin I accumulated de novo in the cytosol of Leishmania. Such 1O2 are expected to react with intracellular substrates present abundantly in the immediate vicinity, i.e., the unsaturated double bands of all vital molecules of proteins, lipids, and nucleic acids (except carbohydrates) (22, 26) (Figure 2B). Cytosolically produced uroporphyrin 1 was observed to undergo efflux from the uroporphyric cells for extracellular exit (20, 27). This event is however insufficient to evacuate all cytosolic uroporphyrins. There is also no evidence for the selection of 1O2-resistance based on this mechanism (see below).
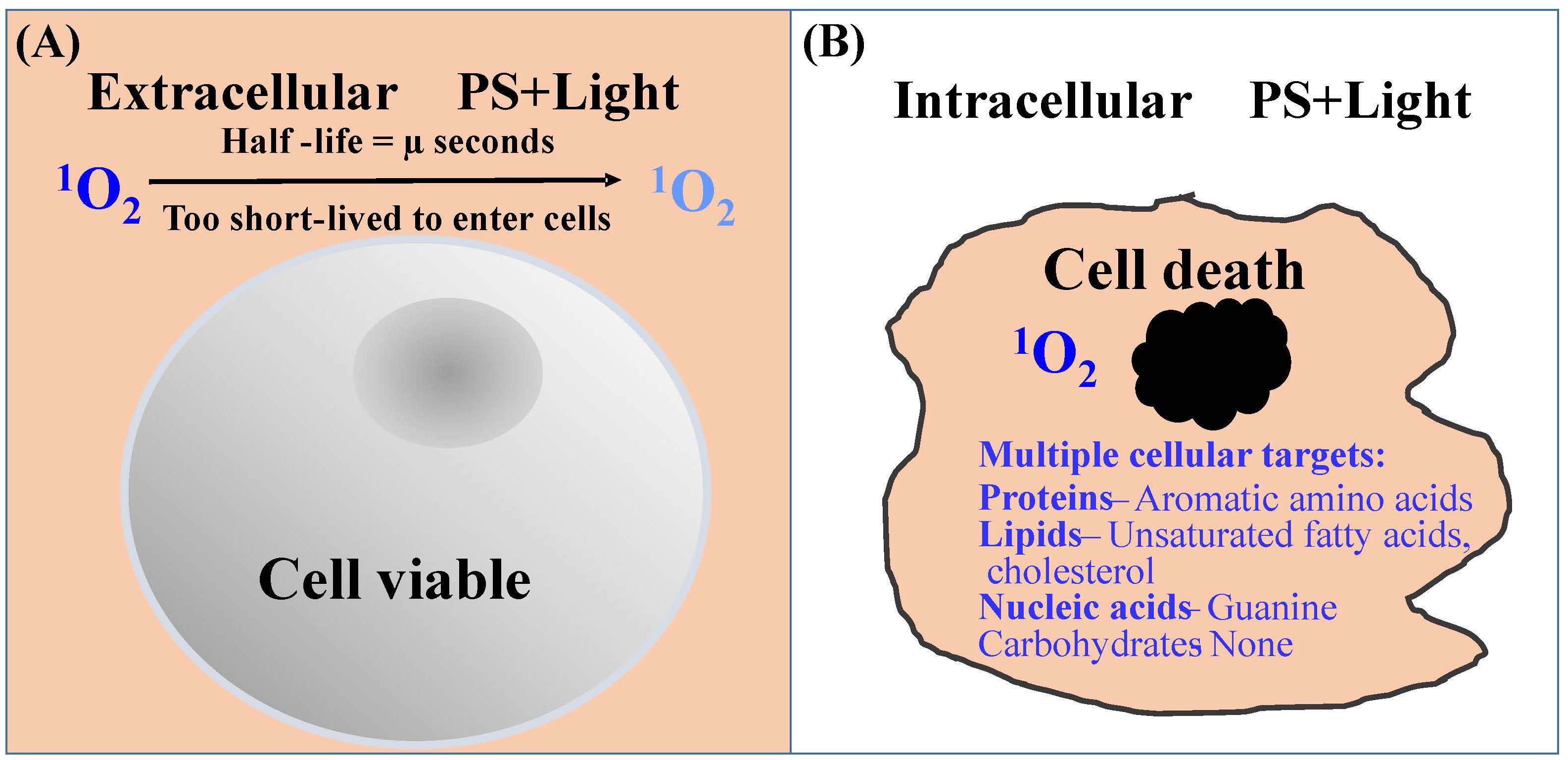
Figure 2 Diagrammatic illustration of the efficiency of 1O2 to cause cytotoxicity when produced inside of cells. (A) Extracellularly produced 1O2 (orange background) is too short-lived to travel the distance of the plasma membrane for cell entry. (B) Intracellularly produced 1O2 reacts readily with unsaturated subunits of many vital macromolecules as indicated.
4 Cytosolic loading of Leishmania with hydrophilic uroporphyrin 1 by transgenic complementation of its inherent deficiencies in the enzymes of heme biosynthetic pathway
In the foregoing sections, Leishmania were mentioned as loaded with water-soluble uroporphyrin I (URO-1) in their cytosol, rendering them sensitive to 1O2 generated after their exposure to dim light (Section 2), and also their inability to import this water-soluble porphyrin from the extracellular milieu (Section 3). This section describes the transgenic approach to loading Leishmania with URO-1 by exploiting their inherent defects in heme biosynthesis (Figure 3). In all aerobic cells, heme biosynthetic pathway is indispensable, requiring a cascade of eight different enzymes to complete (Figure 3A), of which the first five are absent in trypanosomatid protozoa (28), accounting for their requirement of heme compounds as an essential nutrient from exogenous sources for growth; exception are several trypanosome species, which acquire this nutrient from the bacterial endosymbionts they harbor (29, 30). The non-symbiont-containing species, such as Leishmania spp. possess the genes coding for the last three enzymes in this pathway (Figure 3B), presumably transferred laterally from endosymbionts that have long been lost during evolution (28). Leishmania were transfected episomally with plasmids to express mammalian cDNAs encoding aminolevulinate dehydratase (ALA-D) and porphobilinogen deaminase (PBGD), the second and third enzymes in the heme biosynthetic pathway (Figure 3C). Both transgenes were verified to express at protein levels with enzymatic activities (27, 31). These transgenic Leishmania produce cytosolic URO-1 when exposed to delta-aminolevulinic acid (ALA)—the product of the first enzyme in the heme biosynthetic pathway. ALA is readily taken up via amino acid and/or peptide transporters by the transfectants, making it available as a substrate for the transgene products (second and third enzymes). The immediate product formed is uroporphyrinogen 1, which is auto-oxidized into URO-1 in the absence of the downstream enzyme (URO-S), leading to its cellular accumulation (Figure 3D). Fluorescent microscopy clearly shows the distribution of URO-1 throughout the uroporphyric cells, including flagella, hence, its cytosolic localization accounting for their response to dim light for the generation of flagellum-immobilization 1O2.
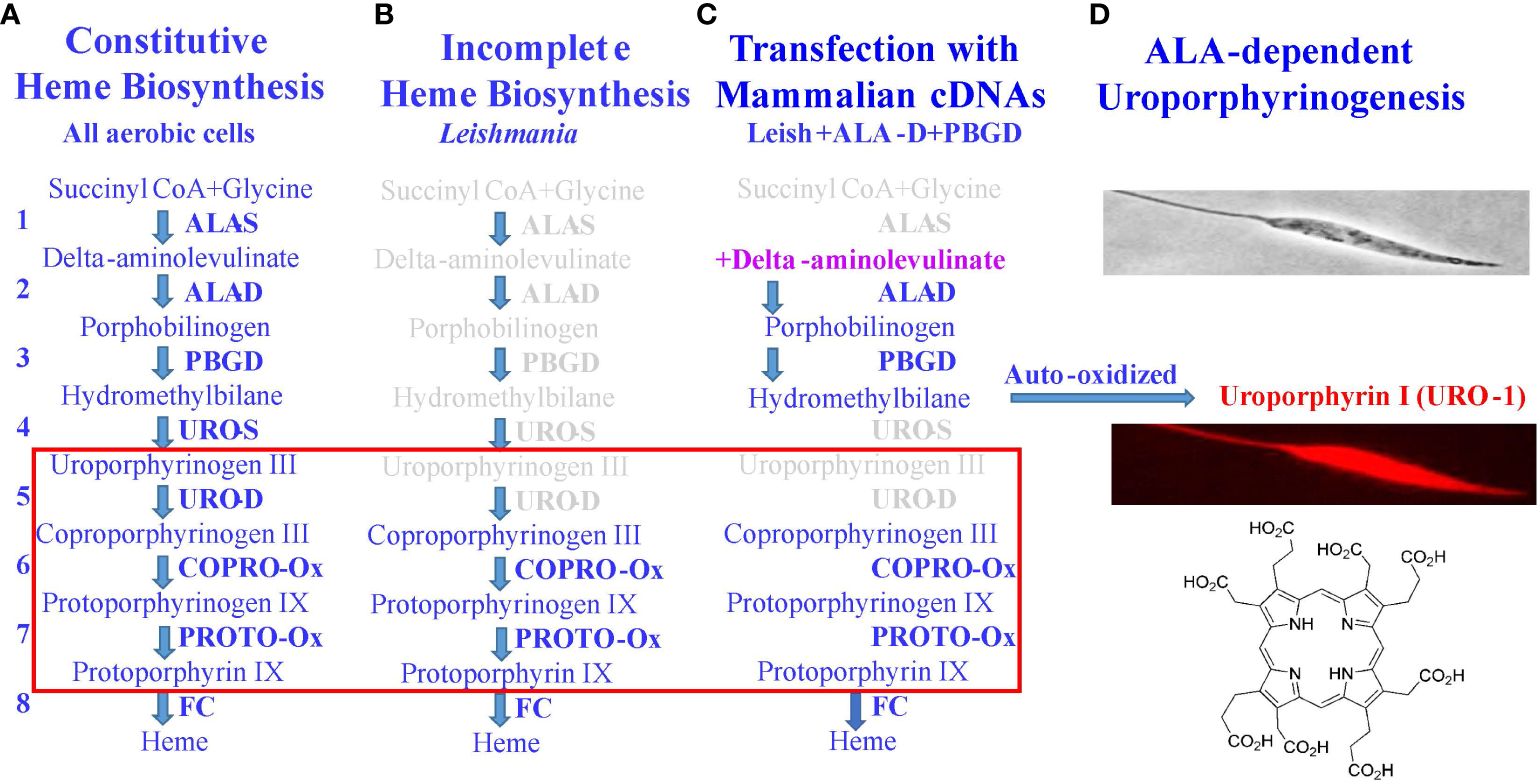
Figure 3 Molecular strategy for loading Leishmania with uroporphyrin 1. (A) Classic heme biosynthetic pathway with a cascade of eight different enzymes (1–8) and four light-sensitive porphyrin intermediates (red rectangular). (B) Defects of this pathway in Leishmania in missing the genes coding for the first 5 enzymes in its genome. (C) A partial restoration of the defects by transfection of Leishmania with mammalian cDNAs, coding for the second and third enzymes (ALA-D, PBGD), rendering the transfectants responsive to delta-aminolevulinate (ALA)—a product of the first enzyme to produce uroporphyrinogen 1. (D) In the absence of the downstream enzyme (URO-S), it is auto-oxidized to uroporphyrin 1 (URO-1) and accumulates cytosolically in Leishmania referred to as uroporphyrinogenesis. URO-1, devoid of charged side groups and highly water-soluble, is otherwise not taken up by cells from the milieu.
5 Inactivation of Leishmania by 1O2 generated after delta-aminolevulinate-induced uroporphyrinogenesis followed by UV exposure: repeated cycles of inactivation result in no emergence of 1O2 resistance
This has been established by examinations of the uroporphyrinogenic transfectants (27, 31). The transfectants, routinely passaged under drug selection, were grown under drug-free conditions for 1-cycle to the stationary phase (Figure 4A). Cells were harvested and resuspended under suitable conditions (50X107-108 cells/ml in HBSS-BSA) for exposure to a high concentration of ALA (1 mM). Uroporphyrinogenesis was allowed to proceed in the dark for up to 2 days. De novo emergence of URO-1 in these cells was verified as the sole porphyrin species by chromatography of their solvent extracts versus porphyrin standards (27, 31). Cytosolic accumulation of URO-1 was indicated by the presence of porphyrin fluorescence sedimented with cells to the pellets by centrifugation and in the cells when examined by fluorescence microscopy (20). Thin layers of the uroporphyric cell suspension (in culture wells) were exposed to UV light without a barrier (from the top for 20 min). Inspection of the cells before and after UV illumination showed a dramatic change in their flagellar behavior from active motility to complete immobilization. The inactivation was attributed to the activities of 1O2 produced by UV excitation of cytosolic URO-1 via mechanisms, as described in Sections 2 and 3. It is well-known that 1O2 is formed as the principal species of free radicals when porphyrins are excited by UV under the conditions described. While other ROS may be formed secondarily, e.g., peroxide and superoxide, they play an insignificant role, since Leishmania are known to possess abundant superoxide dismutase, peroxidases, and other anti-oxidants as intracellular parasites of macrophages (32).
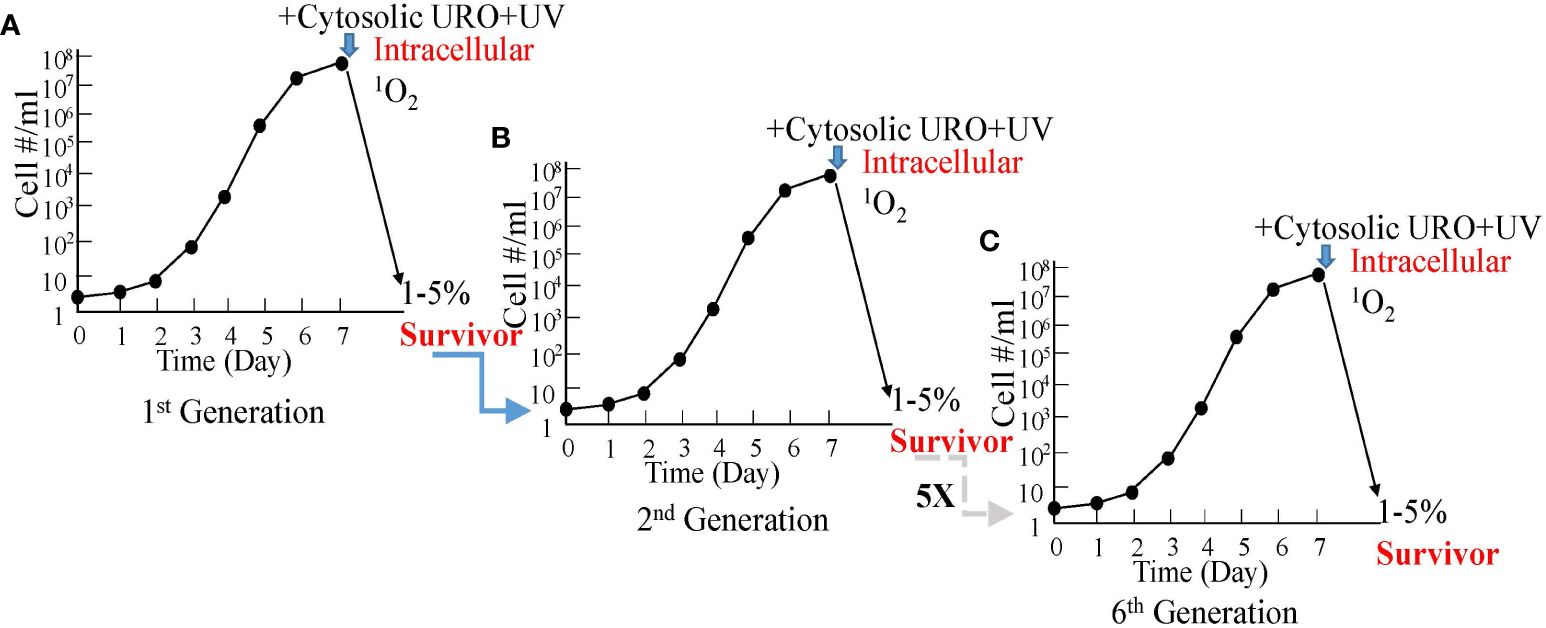
Figure 4 Graphic depiction for the absence of resistance by uroporphyric Leishmania after repeated cycles of growth and cytosolic 1O2 inactivation. (A) In vitro growth of uroporphyrinogenic cells to the stationary phase for inactivation by cytosolic 1O2 after ALA-induced uroporphyrinogenesis and longwave UV irradiation, 1%–5% being rescuable in enriched medium. (B) The second generation grown from the rescued cells remains as susceptible as the first. (C) This is the case for all the subsequent five generations examined.
All Leishmania perished in the aftermath of 1O2 inactivation, except for a small number when rescued in time with enriched cultured conditions. These survivors remain resistant to the selective drug pressures, hence, retaining their plasmids with the transgenes. When grown to the stationary phase, they remain responsive to ALA to undergo uroporphyrinogenesis for cytosolic URO-1 accumulation and are susceptible to 1O2 inactivation with UV to the same extent as before (Figure 4B). The level of this susceptibility stays the same after repeating the same treatments for another five cycles, never yielding any cultures with an increase in 1O2 resistance (Figure 4C). The recoverability of few survivors after each cycle of treatments is attributable to non-inheritable pleotropic events of population heterogeneity, e.g., reduced transgene expressions and/or ALA transport, resulting in a low level of URO-1 accumulation during uroporphyrinogenesis and inefficient 1O2 inactivation.
6 Loading of Leishmania by endocytosis with hydrophilic synthetic phthalocyanines for 1O2 inactivation via red light excitation
Phthalocyanines (PC) are photosensitizers that are amenable to laboratory synthesis with the versatilities to alter their biological properties via the substitution of different coordinating metals (Al, Cu, Si, and Zn) for ligand attachments and/or via additions/modifications of side chains. Previously, of the 17 novel hydrophilic Zn anilinium PC and Si or Zn pyridyloxy PC examined, several were found endocytosed by Leishmania, rendering them sensitive to inactivation with red light (>600 nm wavelengths) that is known to excite PC optimally to produce biocidal 1O2 (33). Subsequently, amino-PC were examined and found to have similar properties with greater potency at lower concentrations for the inactivation of Leishmania (34). In addition, the synthesis of amino-PC (PC1) is straightforward, involving reflux of the two substrates in several solvents as shown (Figure 5A). Synthesized products are purified by HPLC and authenticity is verified by NMR (35). Commercial service is available for scale-up production of PC1 in quantity (PorphyChem. Co). Figure 5B shows the avid uptake of PC1 at µM concentrations in physiological saline by Leishmania into their endosomes via endocytosis. Loading of Leishmania with PC1 is simple, effectively sensitizing these cells to 1O2 inactivation generated by deep-penetrating red light through plastic-covered culture wells (33).
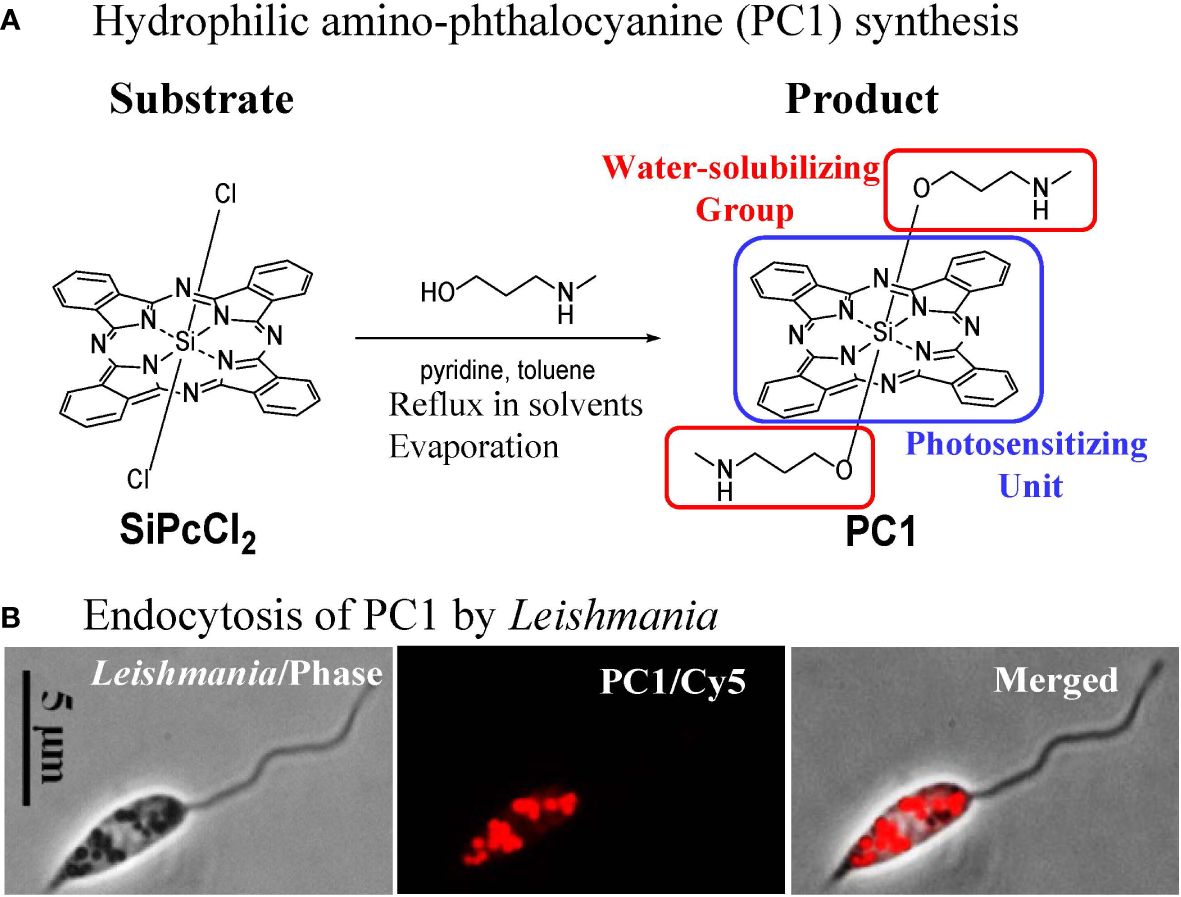
Figure 5 Molecular engineering of phthalocyanines for endosomal loading of Leishmania. (A) Chemical synthesis of amino-phthalocyanines (PC1) with hydrophilic groups (red frame). (B) Phase contrast and fluorescence images, showing uptake of PC1 into the endosomes by Leishmania.
7 1O2-inactivated Leishmania as safe and effective vaccines and platforms for vaccination against infectious and malignant diseases
Experimental evidence indicates that 1O2-inactivated Leishmania preserve the activities of both their natural vaccines and adjuvants. Antigenic peptides or proteins and adjuvants are the key components in vaccine formulations for effective immuno-prophylaxis and -therapy of many diseases (36). Leishmania are known to possess both from their prophylactic activities manifested by the development of lifelong immunity after natural infection in endemic areas. Leishmania-specific immunity has been demonstrated in convalescent patients (37, 38) from both symptomatic and asymptomatic cases (39). Immunization of animals with 1O2-inactivated Leishmania indeed protects them against challenges with virulent parasites for both cutaneous and visceral leishmaniasis (40, 41).
1O2-inactivated Leishmania are also deployable as safe and effective platforms for vaccination against other diseases when transfected to express add-on antigens. Their feasibility was initially indicated by examining Leishmania transfected to express ovalbumin (OVA)—a model T-cell antigen. Dendritic cells loaded with 1O2–inactivated transfectants were found to process their OVA effectively for presenting its T-cell epitope (SIINFEKL) and for activating the epitope-specific CD8 T cells in vitro (33). Subsequently, uroporphyrinogenic Leishmania were transfected to express a candidate lung tumor vaccine, enolase-1 (ENO1). These transfectants were 1O2-inactivated to completion via both URO-1 and PC loading in combination followed by light exposure (42). The immunotherapeutic potential of these ENO1 transfectants was demonstrated by their activities via vaccination to suppress the development of tumors from the cancer cells implanted in the peritoneal cavity of susceptible mice (43). The preliminary data obtained showed dramatic results of a significant reduction in tumor size in the experimental group versus the control. Transfection of uroporphyrinogenic Leishmania to express viral vaccine candidates, e.g., EBOLA-GP and SARS Cov-2 Spike has been completed. Immunization of mice with 1O2-inactivated Spike-transfectants showed antigen-specific T-cell responses, suggestive of their vaccination potential (44).
8 Phthalocyanines as novel red light-activated insecticides: practices and principles based on 1O2 inactivation of Leishmania
Light-activated insecticides (LAI) have been under intensive investigation, as indicated by the existence of a large body of literature in this field for an extended period, several decades ago [see 10, 11]. The pioneering work has laid much of the foundation for the general principles and practices of LAI. The light sources used, for example, included sun rays in many fieldwork and artificial lighting from incandescent bulbs to fluorescent lamps to LED for laboratory studies (Figure 6A). It is practical to use white light, inclusive of 400–700-nm wavelengths in the visible spectrum (Figure 6B), to activate chemically different PS with variable wavelengths for optimal excitation (e.g., halogenated fluoresceins and chlorophyll and porphyrin derivatives) to generate insecticidal free radicals. Not used or rarely mentioned in the previously published work are phthalocyanines (PC) as LAI. As mentioned in Section 6, PC are excitable optimally with red to infrared (IR) lights (wavelengths ≥630 nm) to produce biocidal 1O2 that have potential advantages in considering their use as LAI. One advantage is the versatility of PC for structural modifications to improve their bioavailability at the cellular level, as already mentioned in Section 6. The uptake of PS by insect cells is a prerequisite, as much as in the case of Leishmania, for their susceptibility to 1O2 generated by excitation of the PS with dim light. This was shown to be the case with the mosquito cells grown in vitro, accounting for the white light-mediated larvicidal activities of PC and other PS observed (45). The property of red light to penetrate a barrier is clearly advantageous for reaching PC, which are taken up by cells inside the insects. In comparison, cytosolically URO-1 loaded Leishmania are susceptible in cell suspensions to irradiation with far less penetrating UV (Wavelength ¾400 nm) (Figures 6D, E). Under the two different settings, while the actual levels of biocidal 1O2 generated are not known, they are sufficient to kill mosquito larvae and Leishmania, respectively. Undoubtedly, PC are favorably disposed for use as LAI in the field conditions where the penetrating red light is expected to “hit” the PC in hidden insects more effectively to produce 1O2. Another advantage of PC is related to the downward shift of the visual preference of insects for the short wavelengths toward the UV range (46) (Figure 6C). The use of IR light-excitable PC as LAI is thus more stealth against daylight active insects and makes it possible to target nocturnal species notwithstanding the complexity of the need to set up IR illumination.
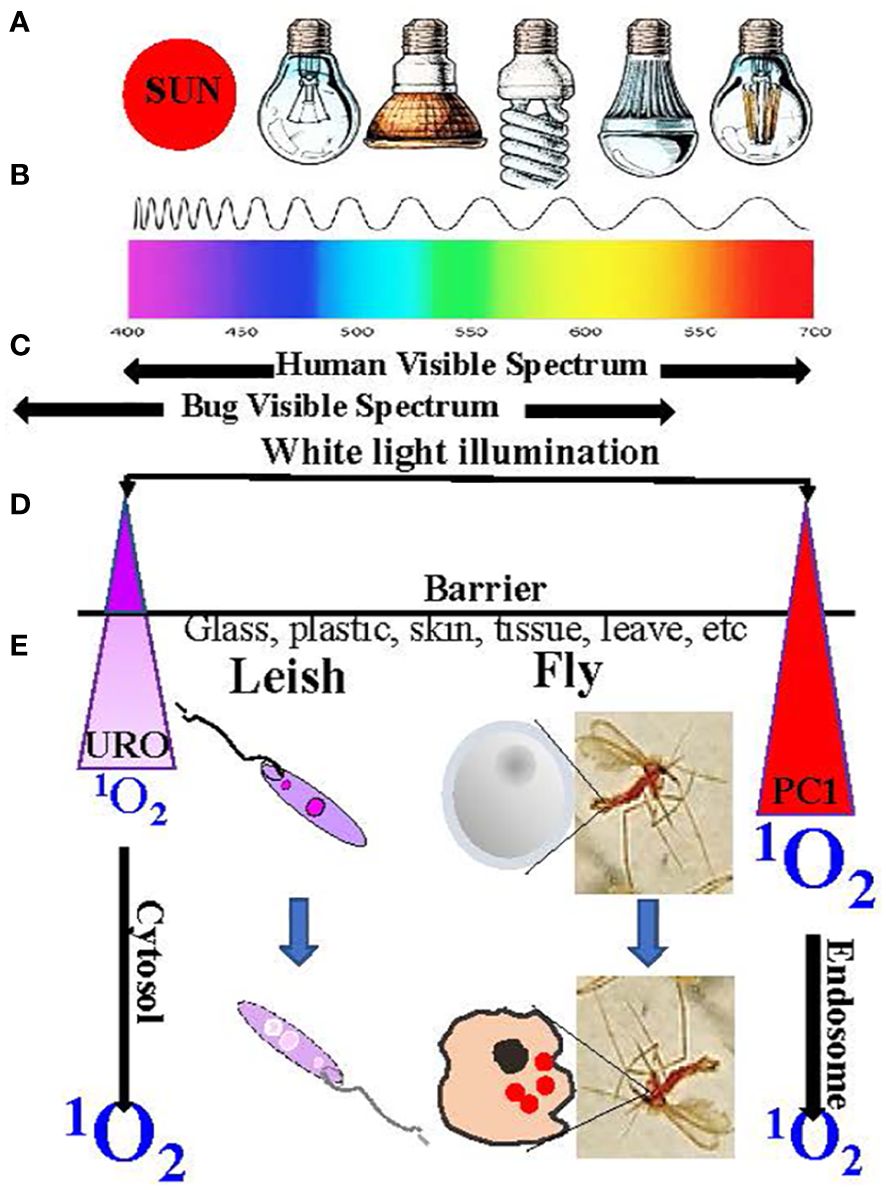
Figure 6 Illumination to excite intracellular photosensitizers to produce 1O2. (A) Light source. (B) Wavelength spectrum. (C) Human vs. insect visibility spectrum. (D) URO/PC1 peak excitation wavelengths and properties. (E) Factors affecting the biocidal activities of the generated 1O2 (See text for further details).
9 Concluding remarks
The exploitation of the highly destructive 1O2 as an effective biocide requires optimization of its cytocidal activities. This is achievable by taking into consideration of its inherent property as an extremely short-lived free radical and the selection of targets with no defense mechanism against it. Depicted in Sections 2 and 3 are the loading of non-photosynthetic cells with PS light-activable to produce 1O2 based on its effectiveness with cells from some examples of parasites and vectors, as shown in the subsequent sections. It is feasible to screen PS in vitro for their uptake by target cells and their subsequent apoptotic cell death after light activation to produce 1O2. Such in vitro screening pre-selects effective PS, thereby reducing their number for the demanding in vivo or field trials.
The major advantage of deploying 1O2 as a biocide is its aversion to the elicitation of resistance, unlike most anti-cancer drugs, microbicidal agents, and pesticides in use. The selection of pre-existing genetic mutations, responsible for many cases of drug resistance, does not apply to biocidal 1O2, since the mechanisms of its generation and disposition are non-enzymatic in all cell types, except, perhaps, human neutrophils (47), precluding the emergence of 1O2-resistant variants at protein levels from genetic mutations. The formation of 1O2 requires the simultaneous presence of light, PS, and O2 at dosages, which are individually non-toxic and thus, non-selective for spontaneously generated genetic variants of relevant phenotypes, e.g., PS transport and efflux. The biocidal activity of 1O2 itself is unlikely to generate viable target molecule-specific mutants, as it rapidly and simultaneously inactivates multiple cellular molecules of very different chemical structures. This advantage of 1O2 as a biocide has long been recognized (18, 19) and is illustrated here by experimental evidence with uroporphyric Leishmania (Section 5).
Photodynamic therapy (PDT) is a fully accepted clinical practice, but largely for rapidly eliminating fast-growing solid tumors with 1O2 and other free radicals by injecting PS into the diseased or cancerous tissues followed by laser irradiation. Other applications of PDT for germicidal, parasiticidal, and insecticidal activities have been limited. The caveat is the difficulty of its targeting specificity to avoid collateral damage to healthy cells and tissues or beneficial organisms in the natural environment. Thus, the ideal PS worthy of consideration for development toward that end are those receptive to specific tagging [see 25] or modifiable with discriminatory properties. Clearly, phthalocyanines are most amenable to such manipulation for the ease of their structural modifications, potentially allowing selective uptake, for example, by parasites and vectors, but not by their hosts and beneficial insects. The availability of hydrophilic amino-PC (Section 6) makes it possible to illustrate the necessity of PS uptake by cells for optimal biocidal activities of 1O2. Of particular interest is another observation that a shift of the O-linked phenyl side chain in two peripheral rings of a PC from the beta position to the alpha position resulted in a dramatic gain of mosquito larvicidal activity (45). Such a profound change in biological activity by a slight modification of the PC is most encouraging, suggestive of its potential utility to achieve targeting specificity. Perhaps, machine learning and artificial intelligence programs may be of help here given their emergence to facilitate drug discovery and design (48, 49).
The inactivation of Leishmania with 1O2 in vitro offers a platform for the effective and safe delivery of vaccines. Exploited for effectiveness are the natural properties of Leishmania, which are endowed with vaccinability and adjuvanticity as well as transgenic capacity to express foreign vaccines specific for other diseases. The safety is ascertained by loading Leishmania with PS for their complete inactivation with 1O2, i.e., URO-1 and PC1 in separate cell compartments for light excitation (Sections 4–6). Significantly, both vaccinability and adjuvanticity of Leishmania are well-preserved after 1O2 inactivation, favorable in comparison to treatments with chemicals, e.g., aldehydes and physical means, such as heat inactivation. The transgenic approach to produce porphyrinogenic Leishmania is applicable, in principle, to all parasites for UV-mediated 1O2 inactivation, if desirable. The same or similar transgenes are usable to achieve this for other trypanosomatid protozoa also known to be heme-auxotrophs or for parasites with no heme biosynthesis. For other parasites with a complete heme biosynthesis, it is possible to produce their porphyric mutants by genetic engineering with constructs to achieve conditional knockouts against any of the three enzymes, which utilize porphyrin intermediates in this pathway (see Figure 3A). Such 1O2-inactivated whole parasites may be advantageous for use with a full complement of vaccine molecules versus selected antigens for delivery by Leishmania or by other platforms.
There is an urgent need to develop new avenues, such as the deployment of 1O2 via the strategies outlined against the etiological agents and insect vectors of tropical diseases, which have long been neglected because of their prevalence in impoverished regions of the world.
Author contributions
KC: Conceptualization, Data curation, Methodology, Visualization, Writing – original draft. JR: Funding acquisition, Resources, Supervision, Writing – review & editing. DN: Data curation, Methodology, Validation, Writing – review & editing. Y-HT: Investigation, Writing – review & editing. C-KF: Funding acquisition, Project administration, Supervision, Writing – review & editing. S-HS: Data curation, Formal analysis, Funding acquisition, Supervision, Writing – review & editing.
Funding
The author(s) declare financial support was received for the research, authorship, and/or publication of this article. This study was supported by Center for Cancer Cell Biology, Immunology and Infection, Rosalind Franklin University of Medicine and Science, and KPC Research funds.
Acknowledgments
The authors thank the institutions to which they are affiliated, for supporting scholarly activities leading to scientific publication.
Conflict of interest
The authors declare that the research was conducted in the absence of any commercial or financial relationships that could be construed as a potential conflict of interest.
Publisher’s note
All claims expressed in this article are solely those of the authors and do not necessarily represent those of their affiliated organizations, or those of the publisher, the editors and the reviewers. Any product that may be evaluated in this article, or claim that may be made by its manufacturer, is not guaranteed or endorsed by the publisher.
References
1. Orfanopoulos M. Singlet oxygen: discovery, chemistry, C60 -sensitization. Photochem Photobiol. (2021) 97:1182–218. doi: 10.1111/php.13486
2. Triantaphylidès C, Havaux M. Singlet oxygen in plants: production, detoxification and signaling. Trends Plant Sci. (2009) 14:219–28. doi: 10.1016/j.tplants.2009.01.008
3. Dmitrieva VA, Tyutereva EV, Voitsekhovskaja OV. Singlet oxygen in plants: generation, detection, and signaling roles. Int J Mol Sci. (2020) 21:3237. doi: 10.3390/ijms21093237
4. Phillips JD. Heme biosynthesis and the porphyrias. Mol Genet Metab. (2019) 128:164–77. doi: 10.1016/j.ymgme.2019.04.008
5. Murotomi K, Umeno A, Shichiri M, Tanito M, Yoshida Y. Significance of singlet oxygen molecule in pathologies. Int J Mol Sci. (2023) 24:2739. doi: 10.3390/ijms24032739
6. Dougherty TJ, Gomer CJ, Henderson BW, Jori G, Kessel D, Korbelik M, et al. Photodynamic therapy. J Natl Cancer Inst. (1998) 90:889–905. doi: 10.1093/jnci/90.12.889
7. Algorri JF, Ochoa M, Roldán-Varona P, Rodríguez-Cobo L, López-Higuera JM. Photodynamic therapy: A compendium of latest reviews. Cancers (Basel). (2021) 13:4447. doi: 10.3390/cancers13174447
8. Hu X, Huang YY, Wang Y, Wang X, Hamblin MR. Antimicrobial photodynamic therapy to control clinically relevant biofilm infections. Front Microbiol. (2018) 9:1299. doi: 10.3389/fmicb.2018.01299
9. Buzzá HH, Alves F, Tomé AJB, Chen J, Kassab G, Bu J, et al. Porphyrin nanoemulsion for antimicrobial photodynamic therapy: effective delivery to inactivate biofilm-related infections. Proc Natl Acad Sci U.S.A. (2022) 119:e2216239119. doi: 10.1073/pnas.2216239119
10. Heitz JR. Development of photoactivated compounds as pesticides. In: Heitz JR, Downum KR, editors. Light activated pesticides. ACS Symp, Washington DC (1987). p. 1–21.
11. Chang KP, Kolli BK. New Light Group. New "light" for one-world approach toward safe and effective control of animal diseases and insect vectors from leishmaniac perspectives. Parasit Vectors. (2016) 9:396. doi: 10.1186/s13071-016-1674-3
12. Lima AR, Dias LD, Garbuio M, Inada NM, Bagnato VS. A look at photodynamic inactivation as a tool for pests and vector-borne diseases control. Laser Phys Lett. (2022) 19:025601. doi: 10.1088/1612-202X/ac4591
13. Dhaini B, Wagner L, Moinard M, Daouk J, Arnoux P, Schohn H, et al. Importance of rose Bengal loaded with nanoparticles for anti-cancer photodynamic therapy. Pharm (Basel). (2022) 15:1093. doi: 10.3390/ph15091093
14. Meier CJ, Hillyer JF. Larvicidal activity of the photosensitive insecticides, methylene blue and rose Bengal, in Aedes aegypti and Anopheles Gambiae mosquitoes. Pest Manag Sci. (2024) 80(2):296–306. doi: 10.1002/ps.7758
15. Wolnicka-Glubisz A, Olchawa M, Duda M, Pabisz P, Wisniewska-Becker A. The role of singlet oxygen in photoreactivity and phototoxicity of curcumin. Photochem Photobiol. (2023) 99:57–67. doi: 10.1111/php.13666
16. Sureshbabu A, Smirnova E, Karthikeyan A, Moniruzzaman M, Kalaiselvi S, Nam K, et al. The impact of curcumin on livestock and poultry animal's performance and management of insect pests. Front Vet Sci. (2023) 10:1048067. doi: 10.3389/fvets.2023.1048067
17. Lee HJ, Kang SM, Jeong SH, Chung KH, Kim BI. Antibacterial photodynamic therapy with curcumin and Curcuma xanthorrhiza extract against Streptococcus mutans. Photodiagnosis Photodyn Ther. (2017) 20:116–9. doi: 10.1016/j.pdpdt.2017.09.003
18. Meier CJ, Rouhier MF, Hillyer JF. Chemical control of mosquitoes and the pesticide treadmill: A case for photosensitive insecticides as larvicides. Insects. (2022) 13:1093. doi: 10.3390/insects13121093
19. Hamblin MR. Antimicrobial photodynamic inactivation: a bright new technique to kill resistant microbes. Curr Opin Microbiol. (2016) 33:67–73. doi: 10.1016/j.mib.2016.06.008
20. Dutta S, Kolli BK, Tang A, Sassa S, Chang KP. Transgenic Leishmania model for delta-aminolevulinate-inducible monospecific uroporphyria: cytolytic phototoxicity initiated by singlet oxygen-mediated inactivation of proteins and its ablation by endosomal mobilization of cytosolic uroporphyrin. Eukaryot Cell. (2008) 7:1146–57. doi: 10.1128/EC.00365-07
21. Bäumler W, Regensburger J, Knak A, Felgenträger A, Maisch T. UVA and endogenous photosensitizers–the detection of singlet oxygen by its luminescence. Photochem Photobiol Sci. (2012) 11:107–17. doi: 10.1039/c1pp05142c
22. Fujii J, Soma Y, Matsuda Y. Biological action of singlet molecular oxygen from the standpoint of cell signaling, injury and death. Molecules. (2023) 28:4085. doi: 10.3390/molecules28104085
23. Ramel F, Birtic S, Ginies C, Soubigou-Taconnat L, Triantaphylidès C, Havaux M. Carotenoid oxidation products are stress signals that mediate gene responses to singlet oxygen in plants. Proc Natl Acad Sci U.S.A. (2012) 109:5535–40. doi: 10.1073/pnas.1115982109
24. Mesa T, Munné-Bosch S. α-Tocopherol in chloroplasts: Nothing more than an antioxidant? Curr Opin Plant Biol. (2023) 74:102400. doi: 10.1016/j.pbi.2023.102400
25. Liang P, Kolodieznyi D, Creeger Y, Ballou B, Bruchez MP. Subcellular singlet oxygen and cell death: location matters. Front Chem. (2020) 8:592941. doi: 10.3389/fchem.2020.592941
26. Di Mascio P, Martinez GR, Miyamoto S, Ronsein GE, Medeiros MHG, Cadet J. Singlet molecular oxygen reactions with nucleic acids, lipids, and proteins. Chem Rev. (2019) 119:2043–86. doi: 10.1021/acs.chemrev.8b00554
27. Sah JF, Ito H, Kolli BK, Peterson DA, Sassa S, Chang KP. Genetic rescue of Leishmania deficiency in porphyrin biosynthesis creates mutants suitable for analysis of cellular events in uroporphyria and for photodynamic therapy. J Biol Chem. (2002) 277:14902–9. doi: 10.1074/jbc.M200107200
28. Opperdoes FR, Coombs GH. Metabolism of Leishmania: proven and predicted. Trends Parasitol. (2007) 23:149–58. doi: 10.1016/j.pt.2007.02.004
29. Chang KP, Trager W. Nutritional significance of symbiotic bacteria in two species of hemoflagellates. Science. (1974) 183:531–2. doi: 10.1126/science.183.4124.531
30. Chang KP, Chang CS, Sassa S. Heme biosynthesis in bacterium-protozoon symbioses: enzymic defects in host hemoflagellates and complemental role of their intracellular symbiotes. Proc Natl Acad Sci U S A. (1975) 72:2979–83. doi: 10.1073/pnas.72.8.2979
31. Dutta S, Furuyama K, Sassa S, Chang KP. Leishmania spp.: Delta-aminolevulinate-inducible neogenesis of porphyria by genetic complementation of incomplete heme biosynthesis pathway. Exp Parasitol. (2008) 118:629–36. doi: 10.1016/j.exppara.2007.11.013
32. Santi AMM, Murta SMF. Antioxidant defence system as a rational target for Chagas disease and Leishmaniasis chemotherapy. Mem Inst Oswaldo Cruz. (2022) 117:e210401. doi: 10.1590/0074-02760210401
33. Dutta S, Ongarora BG, Li H, Vicente Mda G, Kolli BK, Chang KP. Intracellular targeting specificity of novel phthalocyanines assessed in a host-parasite model for developing potential photodynamic medicine. PloS One. (2011) 6:e20786. doi: 10.1371/journal.pone.0020786
34. Al-Qahtani A, Alkahtani S, Kolli B, Tripathi P, Dutta S, Al-Kahtane AA, et al. Aminophthalocyanine-mediated photodynamic inactivation of Leishmania tropica. Antimicrob Agents Chemother. (2016) 60:2003–11. doi: 10.1128/AAC.01879-15
35. Jiang XJ, Yeung SL, Lo PC, Fong WP, Ng DK. Phthalocyanine-polyamine conjugates as highly efficient photosensitizers for photodynamic therapy. J Med Chem. (2011) 54:320–30. doi: 10.1021/jm101253v
36. Chang KP. Vaccination for disease prevention and control: The necessity of renewed emphasis and new approaches. J Immunol Immunotech. (2014) 1:10.17653/2374–9105.SSe0001. doi: 10.17653/2374-9105.SSe0001
37. Kaushal H, Bras-Gonçalves R, Negi NS, Lemesre JL, Papierok G, Salotra P. Role of CD8(+) T cells in protection against Leishmania donovani infection in healed visceral leishmaniasis individuals. BMC Infect Dis. (2014) 14:653. doi: 10.1186/s12879-014-0653-6
38. Hamrouni S, Bras-Gonçalves R, Kidar A, Aoun K, Chamakh-Ayari R, Petitdidier E, et al. Design of multi-epitope peptides containing HLA class-I and class-II-restricted epitopes derived from immunogenic Leishmania proteins, and evaluation of CD4+ and CD8+ T cell responses induced in cured cutaneous leishmaniasis subjects. . PloS Negl Trop Dis. (2020) 14:e0008093. doi: 10.1371/journal.pntd.0008093
39. Costa CHN, Chang KP, Costa DL, Cunha FVM. From infection to death: An overview of the pathogenesis of visceral leishmaniasis. Pathogens. (2023) 12:969. doi: 10.3390/pathogens12070969
40. Kumari S, Samant M, Khare P, Misra P, Dutta S, Kolli BK, et al. Photodynamic vaccination of hamsters with inducible suicidal mutants of Leishmania amazonensis elicits immunity against visceral leishmaniasis. Eur J Immunol. (2009) 39:178–91. doi: 10.1002/eji.200838389
41. Viana SM, Celes FS, Ramirez L, Kolli B, Ng DKP, Chang KP, et al. Photodynamic vaccination of BALB/c mice for prophylaxis of cutaneous leishmaniasis caused by Leishmania amazonensis. Front Microbiol. (2018) 9:165. doi: 10.3389/fmicb.2018.00165
42. Dutta S, Waki K, Chang KP. Combinational sensitization of Leishmania with uroporphyrin and aluminum phthalocyanine synergistically enhances their photodynamic inactivation in vitro and in vivo. Photochem Photobiol. (2012) 88:620–5. doi: 10.1111/j.1751-1097.2012.01076.x
43. Chang KP, Dennis KP, Ng DKP, Fan C-K, Batchu RB, Kolli BK. Effective delivery of cancer vaccines with oxidatively photo-inactivated transgenic Leishmania for tumor immunotherapy in mouse models. OBM Genet Mol Cancer Ther. (2020) 4(1): 103. doi: 10.21926/obm.genet.2001103
44. Chang KP, Reynolds JM, Liu Y, He JJ. Leishmaniac quest for developing a novel vaccine platform. Is a roadmap for its advances provided by the mad dash to produce vaccines for covid-19? Vaccines (Basel). (2022) 10:248. doi: 10.3390/vaccines10020248
45. Shiao SH, Weng SC, Luan L, Vicente MDGH, Jiang XJ, Ng DKP, et al. Novel phthalocyanines activated by dim light for mosquito larva- and cell-inactivation with inference for their potential as broad-spectrum photodynamic insecticides. PloS One. (2019) 14:e0217355. doi: 10.1371/journal.pone.0217355
46. van der Kooi CJ, Stavenga DG, Arikawa K, Belušič G, Kelber A. Evolution of insect color vision: From spectral sensitivity to visual ecology. Annu Rev Entomol. (2021) 66:435–61. doi: 10.1146/annurev-ento-061720-071644
47. Kanofsky JR, Wright J, Miles-Richardson GE, Tauber AI. Biochemical requirements for singlet oxygen production by purified human myeloperoxidase. J Clin Invest. (1984) 74:1489–95. doi: 10.1172/JCI111562
48. Vamathevan J, Clark D, Czodrowski P, Dunham I, Ferran E, Lee G, et al. Applications of machine learning in drug discovery and development. Nat Rev Drug Discovery. (2019) 18:463–77. doi: 10.1038/s41573-019-0024-5
Keywords: singlet oxygen, porphyrin, phthalocyanine, light-activation, transgenic, Leishmania, vaccine platform, resistance-aversion insecticide
Citation: Chang KP, Reynolds JM, Ng DKP, Tu Y-H, Fan C-K and Shiao S-H (2024) Molecular approaches to deploy singlet oxygen in a Leishmania model as an unassailable biocide for disease mitigation and vector control. Front. Trop. Dis 5:1306429. doi: 10.3389/fitd.2024.1306429
Received: 03 October 2023; Accepted: 14 March 2024;
Published: 07 May 2024.
Edited by:
Yoosook Lee, University of Florida, United StatesReviewed by:
Oscar David Kirstein, Israel Ministry of Health, IsraelFrancine Venturini, University of São Paulo, Brazil
Copyright © 2024 Chang, Reynolds, Ng, Tu, Fan and Shiao. This is an open-access article distributed under the terms of the Creative Commons Attribution License (CC BY). The use, distribution or reproduction in other forums is permitted, provided the original author(s) and the copyright owner(s) are credited and that the original publication in this journal is cited, in accordance with accepted academic practice. No use, distribution or reproduction is permitted which does not comply with these terms.
*Correspondence: Kwang Poo Chang, kwangpoo.chang@rosalindfranklin.edu