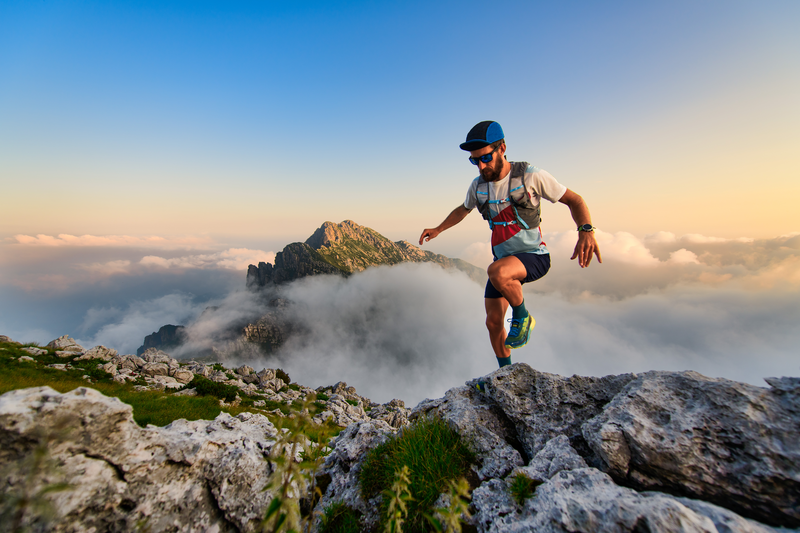
94% of researchers rate our articles as excellent or good
Learn more about the work of our research integrity team to safeguard the quality of each article we publish.
Find out more
ORIGINAL RESEARCH article
Front. Trop. Dis. , 13 March 2024
Sec. Vector Biology
Volume 5 - 2024 | https://doi.org/10.3389/fitd.2024.1235146
This article is part of the Research Topic 2022 in Review: Vector Biology View all 5 articles
Introduction: Many sampling methods are used for entomological surveillance of vector borne diseases. This paper, evaluated the performance of five methods with regard to various ecosystems encountered in Cameroon.
Material and methods: Two entomological databases generated during two study periods were examined: 2011-2014 in the North Region, and 2018-2019 in the Centre Region. Mosquitoes were collected using the (Human Landing Catches) (HLC) and four alternative methods including Clay Pots (CPs), Pyrethroid Spray Catches (PSCs), Window Exit Traps (WETs) and Centers for Disease Control-Light Traps (CDC-LTs) for which the performance was assessed in this study.
Results: A total of 29 anopheline species were identified from samples collected during the two study periods. All these anopheline species were found in North Region, with 5 species being the most abundant and prévalent, i.e. An. gambiae s.l, An. funestus, An. rufipes, An. paludis and An. pharoensis. In the Centre Region, only five species including An. gambiae s.l, An. funestus, An. coustani, An. ziemanni and An. paludis were recorded. Among these, An. gambiae s.l was the most abundant and prevalent species. Data confirmed HLC as the best in sampling outdoor and indoor mosquitoes in the surveyed HDs. The alternative methods showed variable records regarding the species richness. Based on the number of mosquitoes collected, CP was an alternative to HLC for outdoor collections in Garoua and Pitoa HDs, while WET was an alternative in Mayo Oulo HD. In the Centre Region, CDC-LT was an alternative to HLC for indoor collections in Ekié and Nkolbisson HDs, while PSC proved to be the best alternative in Nkolondom HD. Regarding the species richness WET appeared as an alternative to HLC in sampling outdoor mosquitoes in Garoua and Mayo Oulo HDs, while CP was the best alternative in Pitoa HD. In the Centre Region, CDC-LT was an alternative for outdoor and indoor collections in Nkolbisson HD, and the best alternative for outdoor collections in Ekie HD.
Conclusion : The current study revealed variable performance of the five tested adult mosquito collection methods across the prospected HDs in North and Centre Regions of Cameroon. Further investigations will be conducted on other collection methods, e.g., aspiration, mosquito electrocuting grid trap, ovitraps and human-baited double net trap.
Vector borne diseases (VBDs) represent a growing threat to human and animal health. They are a broad and varied group of diseases with the common denominator that pathogens are transmitted through contact or bites of arthropods including mosquitoes, ticks, and aquatic snails (1). In the early 20th century, VBDs were among the world’s most serious public human and animal health problems (2). Currently, they account for more than 17% of all infectious diseases, causing about 1 million deaths annually (3). Over half of the world’s human populations are at risk, and the heaviest burden is borne by the world’s poorest people living in inter-tropical zones, particularly those located in sub-Saharan Africa (SSA) (4). Vector control is the most effective weapon for mass prevention of number of these diseases both historically and today (5). Among VBDs, malaria is a life-threatening disease primarily found in tropical countries, but it represents a global health problem for human populations. The World Health Organization (WHO) reported an estimated 247 million cases and 619,000 deaths due to malaria in 2021. Countries in SSA carry the heaviest part of the global malaria burden with 95.5% cases and 96.3% deaths recorded in 2021 (6). Cameroon is among the 11 countries displaying the highest malaria burden in SSA with 2.7% (N = 6,669,000) of all global malaria cases and 2.3% (N = 14, 237) deaths recorded in 2021 (6). For mass prevention, WHO mainly recommends long-lasting insecticidal nets (LLINs) and (2) insecticide indoor residual spraying (IRS) (7).
Cameroon adopted in 2002 the use of mosquito nets i.e., insecticides treated nets (ITNs) and latterly long-lasting insecticidal nets (LLINs) as the main malaria mass prevention intervention throughout the 10 Regions of the country. Since then, four nationwide mass distribution campaigns have been delivered around 48, 613,031 LLINs, including 8,654,731 in 2011; 11,761,972 in 2015-2016; 10,440,128 in 2019 and 17, 756, 200 for the ongoing distribution in 2023, in order to achieve the universal coverage (one net for two people all over the country). Nets have been distributed in the all the country Regions across the three main malaria epidemiological zones, namely the Sahelian zone where transmission lasts 3-4 months per year, the tropical zone where transmission lasts 6-9 months and the equatorial zone where transmission is continuous all year round. These ecological zone lead to different patterns of vector species’ diversity, with various adaptative capacities, biting and resting behavior and levels of susceptibility to insecticides.
To assess the epidemiological impact of wide usage of LLINs, both parasitological and entomological surveys are to be considered. The entomological impact assessment involves routine surveillance of anopheline vector populations through adequate mosquito sampling methods at larval and/or adult stages. For a country-wide assessment, it is important to conduct routine entomological surveys in the main malaria epidemiological facies. In the framework of this study five collection methods have been tested namely “Human Landing Catches (HLC), Pyrethrum Spray Catches (PSC), Centers for Disease Control Light Traps (CDC-LT), Clay Pots (CP) and Window Exit Trap (WET) (8–11). Nevertheless, most of the data on malaria vectors in Cameroon were collected using HLC considered as the reference sampling method. However, this method is very tedious, requiring well-trained mosquito collectors, a lot of material and financial resources, and above all, it raises ethical issues. Therefore, this method is often restricted to specific research projects rather than entomological surveillance. Although the other existing mosquito sampling methods do not involve human exposure in their procedure, their performance in various malaria epidemiological contexts regularly raise debates.
This paper aims at assessing the performance of four mosquito sampling methods deployed in both tropical and equatorial malaria epidemiological facies encountered in Cameroon. The expected results will enable the National Malaria Control Programme and research institutions involved in vector control and entomological studies to select the appropriate method for mosquito sampling according to the ecological zones and the objectives of the surveys.
The data presented in this study derived from two entomological databases composed of data from longitudinal surveys carried out in a total of 6 health districts (HDs) including 3 HDs in the North region and 3 HDS in the Centre region of Cameroon. The entomological data obtained from the North region were collected in the framework of the “Impact of Resistance” project conducted between 2011 and 2014, while those from the Center region were generated from DMC-MALVEC Project conducted between 2018 and 2019. The HDs prospected in the North region included Garoua (9°30′00′′N, 13°40′00′′E), Pitoa (9°21′00′′N, 13°31′00′′E) and Mayo Oulo (9°46′00′′N, 13°44′00′′E) in urban, semi-urban and rural areas respectively. The North region lies within the Soudanian climate domain with 700–1,000 mm of annual rainfall of 4 months of rains (July to October) and 8 months of dry season (November to June) with an average annual temperature between 27°C and 28°C. The region is located in the tropical zone where malaria transmission is seasonal (4 months per year) and occurs during the rainy season. Yearly cross-sectional entomological surveys were conducted during the high transmission season between September and November for four consecutive years from 2011 to 2014. In the North Region, mosquitoes were collected in 24 locations grouped into HDs as presented by Mandeng and colleagues (12).
In the Centre region, mosquito collections were conducted in three HDs in Yaoundé city namely, Ekié (3°49’60”N, 11°33’00”E), Nkolbisson (04°35’18”N, 09°37’48”E) and Nkolondom (03°56’52”N, 11°30’18”E) located in urban area. Yaoundé, the capital city of Cameroon is located in the Guinean equatorial zone under the influence of the equatorial climate with 4 seasons throughout the year: 2 dry seasons (December-February and July-August) and 2 rainy seasons (March-June and September-November). Temperatures are mild with mean annual ranging from 23 to 25.5°C. The mean rainfall ranges between 1,500 mm to 1,800 mm. The climate is characterized by the alternation of dry and rainy seasons throughout the year, creating favorable conditions for mosquito development and malaria ongoing transmission. The Centre region is situated in the equatorial zone where malaria transmission occurs throughout the year. Yearly cross-sectional entomological surveys were conducted during the low malaria transmission season between April and May in 2018 and the high transmission season between September and October in 2019. In the Centre Region (Yaounde), mosquitoes were collected in one site of each selected HD. Details on the description of these collection sites are provided in Piameu and colleagues (13).
Adult mosquitoes were collected across 36 houses randomly selected in 6 surveyed HDs, i.e. 24 in the North region and 12 in the Centre region. In addition to the standard « Human landing catch » technique, adult mosquitoes were also collected using other conventional sampling methods. All these mosquito sampling methods are described elsewhere (14–16) and those used in the framework of this study are presented in Figure 1. In the North Region, conventional methods included window exit traps (WETs), clay-pots (CPs) and pyrethrum spray catches (PSCs). Each method was performed in 6 houses for 2 non-consecutive days. In the Centre region, the methods used were CDC-Light Trap, pyrethrum spray catches (PSCs) and window exit traps (WET), each method being performed in 3 houses for 2 non-consecutive days. Collected mosquitoes were sorted by genus and those belonging to Anopheles genus were morphologically identified down to species/species complex using standard taxonomic keys for the region (17, 18).
Figure 1 Set of the mosquito collection methods used in collecting anopheline samples in the North and Centre regions. (1) Clay-pots (CPs), (2) Pyrethrum spray catches (PSCs), (3) CDC-Light Trap, and (4) Window exit traps (WET), (S) Standard references method (Human landing catches), 1, 2, 4 and S were used in the Northern region; 2, 3, 4 and S were used in the Centre region.
The collected anopheline specimens were analysed in terms of species richness, community species relative abundance, and distribution status of species. The relative abundance (RA) and distribution status of mosquito species (Ds) were calculated as suggested in previous studies (19–22). The RA of each anopheline species in each surveyed HD considered in this case as a community, so called community species relative abundance (csRA), was calculated as, csRA = (n/N) *100, where “n” is the number of specimens of a particular species and “N” is the total number of specimens collected. Based on the values of csRA, mosquito species were categorized according to Trojan (23) into the following classes: Rare (csRA < 1%), Sub-dominant (csRA < 5%) and Dominant species (csRA > 5%). The distribution status of species was calculated as, Ds = (s/S) *100; where “s” is the number of sites where the mosquito species were found, and “S” is the total number of sites surveyed. Based on the values of Ds, species were categorized according to Dziêczkowski (24) into the following classes: Ds = 0 to 20% (sporadic); Ds = 20.1 to 40% (infrequent); Ds = 40.1 to 60% (moderate); Ds = 60.1 to 80% (frequent), and Ds = 80.1 to 100% (constant). Species richness was evaluated using Shannon-Wiener ecological index (H’) (25). This measure corresponds to the entropy concept defined as:
pi = the proportional abundance or percentage abundance of a species present (pi = ni/N).
ni = the number of individuals counted for a species present.
N = the total number of individuals counted; all species combined.
S = the total or cardinal number of the list of species present.
The value Hmax = log2(S) corresponds to a heterogeneous population for which all the individuals of all the species are equally distributed. The Piélou’s evenness index (E) was defined as E = H’/Hmax. This index therefore varies between 0 and 1, if Hmax tends towards E = 1, then the species present in the stand have identical abundances. If it tends towards E = 0, then we are in the presence of an imbalance where a single species dominates the entire stand.
The annual relative abundance of each mosquito species (aRAs) over the 2 or 4 years of collection was calculated as aRAs = (n/N) *100, where “n” is the number of specimens of a particular species collected during a given year and “N” is the total number of specimens collected during the collection period (2 years in the Region or 4 years in the North region). Descriptive statistics were performed and presented according to the type of variable. Qualitative variables were presented as frequencies and percentages, while quantitative variables were presented as means or medians (M) and standard deviations (SD). Since the mosquito-sampling data for each collection method violated the ANOVA assumptions of normality (Shapiro-Wilk test) and equal variances (Bartlett’s test), these data were compared using the non-parametric Kruskal-Wallis test. When this test was significant, the Dunn’s test was performed to compare the subgroups 2 by 2, using Bonferroni’s correction to avoid inflation of the type I error. Finally, negative binomial models were implemented through multiple regressions to explain the variability in the number of mosquitoes collected by each method. The significance of the incidence rate ratio (IRR) was used to assess the association between number of mosquitoes and explanatory variables. The magnitudes of IRR were used to define an order for the different collection methods. For species richness, the proportions of species collected were computed. For each proportion, its confidence interval was calculated using a binomial approach.
A total of 19,751 anopheline specimens including 19,042 from the North Region and 709 from the Centre Region were collected during entomological surveys conducted for the two projects. The collected anopheline specimens consisted of 29 species heterogeneously distributed within the six surveyed HDs. The species richness varied among the prospected HDs. The distributions of species collected between 2011 and 2014 across the 3 surveyed HDs in the North region and the 3 HDs of the Centre region between 2018 and 2019 are presented in Figure 2.
Figure 2 Distribution of anopheline species collected in the health districts surveyed in the North region between 2011 and 2014 (A) and the Centre region 2018 and 2019 (B).
The North region had relatively high species richness with 29 species, including 13, 17 and 22 species in the Garoua, Pitoa and Mayo-Oulo HDs respectively. These species were classified in six groups (Figure 2A): (1) a group composed of 8 species including An. coustani, An. funestus, An. gambiae s.l, An. implexus, An. paludis, An. pharoensis, An. rufipes and An. ziemanni which were found in the three HDs; (2) a group consisting of two species namely An. squamosus and An. tenebrosus found in Garoua and Mayo Oulo HDs; (3) a group composed of 5 species including An. christyi, An. maculipalpis, An. nili, An. pretoriensis and An. smithii found in Pitoa and Mayo-Oulo HDs; (4) a group composed of 3 species including An. longipalpis, An. moucheti, and An. obscurus found in Garoua HD; (5) a group composed of 4 species namely An. carnevalei, An. marshallii, An. hancocki, and An. jebudensis found only in Pitoa HD and (6) a group consisting of 7 species namely An. ardensis, An. azaniae, An. barberellus, An. domicolus, An. kingi, An. natalensis and An. rhodesiensis found only in Mayo Oulo HD.
The mosquito collections from the Centre region were characterized by a low species richness with five species recorded. Of these, 3 species were found in Ekié HD, 4 in Nkolbisson HD and only one was found in Nkolondom HD (Figure 2B). The 5 species were divided into the following 4 groups: (1) a mono-specific group consisting of An. gambiae s.l, commonly found in the three HDs, (2) a mono-specific group consisting of An. funestus found in Ekie and Nkolbisson HDs; (3) a mono-specific group consisting of An. ziemanni, found in EKié HD, and (4) a group of two species including An. coustani and An. ziemanni only found in Nkolbisson HD.
The community species relative abundance and distribution of anopheline species sampled in each HD are presented in Table 1. In the North region, 5 anopheline species including An. gambiae s.l, An. funestus, An. rufipes, An. paludis and An. pharoensis were the most abundant and ubiquitous in the three surveyed HDs. An. gambiae s.l and An. funestus were dominant (csRA > 5%) and constant (Ds > 80.1%) species in the 3 HDs. An. paludis was a sub-dominant species in the 3 HDs (csRA< 5%), but sporadic in Garoua and Pitoa HDs (Ds = 0 - 20%) and moderate in Mayo Oulo HD (Ds = 40.1-60%). An. pharoensis was a dominant (csRA > 5%) and infrequent (Ds = 20.1- 40%) species in Garoua HD, but sub-dominant (csRA< 5%) and moderate (Ds = 40.1 - 60%) in Pitoa HD, while it was sub-dominant (csRA< 5%), and sporadic (Ds = 0 - 20%) in Mayo Oulo HD. An. rufipes was a dominant (csRA > 5%) and infrequent (Ds = 20.1- 40%) species in Garoua HD, sub-dominant (csRA< 5%) and frequent (Ds = 60.1 – 80%) in Pitoa HD, dominant (csRA > 5%) and frequent (Ds = 60.1 – 80%) in Mayo -Oulo HD. Except for An. rhodesiensis and An. squamosus which were rare (csRA< 1%), and infrequent (Ds = 20.1- 40%) species in Mayo-Oulo HD, An. ziemanni and An. pretoriensis which were rare (csRA< 1%), and moderate (Ds = 40.1 - 60%) species in the same HD. The other 20 remaining species identified in at least one of the 3 surveyed HDs were rare (csRA< 1%), and sporadic (Ds = 0 - 20%).
Table 1 Community species relative abundance and distribution of anopheline species collected between 2011-2014 in the North region and between 2018-2019 in the Centre Region.
In the Centre region, An. gambiae s.l was a dominant (csRA > 5%) and constant (Ds = 80.1-100%) species in the 3 surveyed HDs. An. funestus was a dominant (RA > 5%) and frequent (Ds = 60.1 - 80%) species in Ekie and Nkolbisson HDs, but it was absent in Nkolondom HD. The species An. coustani and An. paludis found in Nkolbisson HD, and An. ziemanni in Ekié HD were distributed as rare (csRA< 1%), and sporadic species (Ds= 0 - 20%).
Table 2 shows indicators of ecological diversity for anopheline species in the surveyed HDs in the North and Centre regions. The values of the Shannon-Wiener index ranged from 0.5 to1.5 in the HDs of the North Region and were below 0.5 in the HDs of the Centre Region. Overall, the values are below 1.5, indicating a low species diversity in the surveyed HDs. However, among the HDs surveyed in each region, the species diversity was greatest in Mayo-Oulo (H’ = 1.48) and Ekie HDs (H’ = 0.69) in the North and Centre regions respectively. The species evenness index (E) within the HDs ranged between 31% and 47% in the HDs of the North region, and between 7% and 55% in the HDs of the Centre Region. These data suggest that the anopheline species collected are unequally distributed in the surveyed HDs. The presence of only a single species in the Nkolondom HD precluded investigation of species diversity in this HD.
Table 2 Ecological diversity indices of anopheline populations of the surveyed health districts in the North and Centre regions.
The species richness and annual relative abundance of each mosquito species (aRAs) varied from one year to another within the same HD and between HDs in the same region. The variation in species richness over the collection period in the North and Centre Regions are shown in Figure 3. In the North region, the species richness of anophelines from 2011 to 2014 ranged from 5 to 9 species in Garoua HD, 6 to 17 species in Pitoa HD and 10 to 15 species in the Mayo Oulo HD (Figure 3A).
Figure 3 Temporal variation in species richness of anopheline species in the surveyed health districts of the North (A) and Centre (B) regions.
In the Garoua and Pitoa HDs, the number of species contributing in anopheline biodiversity was below 10 over the four collection years, except in Pitoa HD where 17 species were recorded in 2013. In Mayo Oulo HD, the number of species composing the biodiversity was equal to or greater than 10 over the four collection years, with a maximum of 15 species recorded in 2012. In the Centre region, species diversity from 2018 to 2019 ranged from 2 to 3 species in Ekie and Nkolbisson HDs, while only a single species complex was found in Nkolondom HD over the two collection periods (Figure 3B).
The study of spatial and temporal variation in the annual relative abundance of each mosquito species (aRAs) collected in the surveyed HDs was carried out only for the 5 main malaria mosquito species/species complex including An. funestus, An. gambiae s.l, An. paludis, An. pharoensis, and An. rufipes in the North Region and for only on An. gambiae s.l in the Centre Region. The dynamics of the annual relative abundance of each species by year of collection, HD and region is shown in Figure 4. Whether in the North or Centre region, each species showed fluctuations in relative abundance from one year to the other across the surveyed HDs. Overall, the maximum relative abundance of the species collected was observed in 2013 compared to the other collection years. Considering all the collections performed for the 5 main species recorded in the three surveyed HDs between 2011 and 2014 in the North Region, the following trends were noted:
- (1) An. funestus was abundantly collected in 2013 in Garoua HD (≈ 50%) and in 2012 and 2014 in Mayo-Oulo and Pitoa HDs (≈ 35-40%). This species was less collected in 2011 in Garoua and Mayo Oulo HDs (< 12%), and in 2013 in Pitoa HD (< 20%). A significant difference was noted between the 2013 relative abundances recorded in each HD compared with that of 2011 in Garoua and Mayo Oulo HDs and 2012 in Pitoa HD (p< 0.05), (Figure 4A);
- (2) An. gambiae s.l. was more collected in 2013 in Garoua and Pitoa HDs (40-50%) and in 2011 in Mayo Oulo HD (≈ 42%). The low relative abundances of this species were recorded in 2011 in Garoua and Pitoa HDs (≈ 5-18%) and in 2012 in Mayo Oulo (≈ 10%). It was noted a significant difference between the 2013 and 2011 relative abundances in Garoua and Pitoa HDs and between the 2011 and 2012 relative abundances in Mayo Oulo HD (p< 0.05), (Figure 4B);
- (3) An. paludis was more abundant in 2013 in Pitoa and Mayo-Oulo HDs (≈ 75-80%) and in 2012 in Garoua HD (≈ 45%). It was almost absent in 2011 in the 3 HDs, but appeared at low relative abundances (< 15%) in 2014 with relative abundances significantly lower compared with that of 2013 in Pitoa and Mayo-Oulo HDs and 2012 in Garoua HD (p< 0.05) (Figure 4C);
- (4) An. pharoensis abundance was higher in 2013 in Pitoa and Mayo Oulo HDs (≈ 55-85%) and in 2011 in Garoua HD (≈ 55%). Compared to the2011 and 2013 relative abundances noted in the corresponding HDs, this species showed significantly lower relative abundances in 2012 and 2014 in the three surveyed HDs (< 10%), (p< 0.05), (Figure 4D);
- (5) An. rufipes was more abundant in 2013 in all three HDs (≈ 35-50%), but with significantly lower relative abundances noted in 2011 in Garoua HD (≈10%), 2012 in Pitoa HD (≈ 7%) and 2014 in Mayo Oulo HD (< 10%) compared with those of 2013 recorded in the corresponding HDs (Figure 4E).
Figure 4 Spatial and temporal variations in annual relative abundance of the main mosquito species collected in the surveyed health districts s of the North and Centre regions. n, number of mosquitoes species collected. Garoua;
Pitol;
Mayo Oulo: Health, districts of the North region corresponding to (A), (B), (C), (D) and (E).
Ekie;
Nkalbisson;
Nikolondom: Health, districts of the Centre region corresponding to (F).
In the Centre region, An. gambiae s.l. was the main and most abundant species collected in the 3 HDs with high mosquito number recorded in 2018 than 2019. This species was very abundant in 2018 (76.7%) in the Nkolbisson HD and less abundant (23.3%) in the same HD in 2019 (Figure 4F).
In the North region, the mean number of mosquitoes collected outdoors in the three HDs by HLC, CP and WET were 534 (SD = 354.44), 281 (SD = 251.9) and 233 (SD = 283.3) respectively. The number of mosquitoes collected by HLC was higher than those obtained by CP and WET in the 3 surveyed HDs. (Figure 5A). The association between collection methods and number of mosquitoes collected was not statistically significant (Kruskal-Wallis H = 5.622, df = 2, p = 0.0601). A 2 by 2 comparison was not necessary because there was no significant difference between mean numbers of mosquitoes collected by the different collection methods. As part of this analysis, the explanatory variables, including the collection method, the health district and the year of collection, were taken into consideration. Compared to the HLC method, the CP (IRR = 0.303, p< 0.0001) and WET methods (IRR = 0.206, p< 0.0001) collected a low number of mosquitoes when considering that collections were performed at the same conditions. Similarly, the IRR recorded with the CP method was higher than that of the WET method, but the difference was not significant because their 95% confidence intervals overlapped (Table 3). Compared to Garoua HD, a low mosquito number was collected in Mayo Oulo HD (IRR = 0.235, p< 0.0001), all other variables being equal. In Pitoa HD however, a slight increase in the number of mosquitoes collected was noted although not significant (IRR = 1.261, p = 4156) compared to Garoua HD. Likewise, compared to 2011, more mosquitoes were collected in 2013 (IRR = 2.797, p = 0.0014) and 2014 (IRR = 2.244, p = 0.0143).
Figure 5 Performance of outdoor and indoor collection methods according to the number of mosquitoes collected in the surveyed health districts in the North and Centre regions (statistical test used: Non-parametric Kruskal-Wallis test). Outdoor collection: (A) North Region; (B) Centre Region. Indoor collection: (C) North Region; (D) Centre Region.
Table 3 Relationship of explanatory variables allowing comparison of collection methods according to the relative abundance of collected mosquitoes.
In the Centre region, the mean number of mosquitoes collected outdoor in the three HDs by HLC, CDC-LT and WET was 115 (SD = 65.9), 3 (SD = 1.4) and 2 (SD = 1.0) respectively. At the level of each HD, the number of mosquitoes collected by CDC-LT was higher than that collected by WET in the 3 HDs. (Figure 5B). The association between collection methods and number of mosquitoes collected was statistically significant (Kruskal-Wallis H = 10.926, df = 2, p = 0.0042). The comparison of collection methods 2 by 2 were made and presented in Table 4. Data suggest that the number of mosquitoes recorded with HLC was greater than that obtained with CDC-LT or WET. Also, CDC-LT collections appeared little interesting than that of WET although the difference was not significant (p = 1) since their 95% confidence intervals overlapped (Table 4). For this analysis, explanatory variables including collection method, health district and year of collection were associated the number of mosquitoes. Compared to the HLC method, the CDC-LT (IRR = 0.030, p< 0.0001) and WET (IRR = 0.012, p< 0.0001) methods collected a low number of mosquitoes when considering that the collection conditions were similar. On the other hand, the IRR of CDC-LT was higher than that of WET, although the difference was not statistically significant (p = 1) due to the overlapping of their 95% confidence intervals, indicating that the two collection methods can be used interchangeably, with CDC-LT being however more suitable to maximize collections (Table 3). Also, fewer mosquitoes were collected in 2019 (IRR = 0.446; p< 0.0001) than in 2018 (Table 3, Figure 5B).
Table 4 Comparison of outdoor and indoor collection methods used in the surveyed HDs of the Centre region.
In the North Region, the mean number of mosquitoes collected indoors in the three health districts was 489 (SD = 119970.3) for HLC and 268 (SD = 37914.4) for PSC. Within the surveyed HDs, the number of mosquitoes collected indoor by HLC was higher than that collected by PSC in the Garoua and Mayo Oulo HDs. However, the difference between collection methods was not statistically significant (Kruskal-Wallis H = 2.9391, df = 1, p = 0.08646) (Figure 5C). When adjusting collection method to the others explanatory variables, the results of the multiple regression were summarized in Table 3. Compared to the HLC method, the PSC method collected significantly fewer mosquitoes (IRR = 0.446, p< 0.0001) (Figure 5C). Among HDs, fewer mosquitoes were collected in Mayo Oulo HD (IRR = 0.332, p< 0.0001) compared to Garoua HD. According to the collection year, more mosquitoes were collected in 2013 (IRR = 1.863, p = 0.0115) compared to 2011.
In the Centre Region, the mean number of mosquitoes collected by HLC, CDC-LT and PSC indoors in the three HDs were 89 (SD = 2191.6), 7 (SD = 3.8) and 4 (SD = 18.2) respectively. Overall, the mean number of mosquitoes collected by HLC was significantly higher (p< 0.0001) than that collected by CDC-LT or PSC in the three surveyed HDs. In Ekie and Nkolbisson HDs, the mean number of mosquitoes collected by CDC-LT was significantly higher (p< 0.0001) than that obtained by PSC, while in Nkolondom HD the difference not significant, the median of the distribution of the numbers of mosquitoes obtained having almost the same value (Figure 5D).
The association between collection methods and number of mosquitoes collected was statistically significant (Kruskal-Wallis H = 13.105, df = 2, p = 0.0014). The comparison of collection methods 2 by 2 is summarized in Table 4. On the whole, HLC performed better than CDC-LT and WET. When combining the explanatory variables i.e. collection method, health district, collection year and the number of mosquitoes collected the results were summarized in Table 3. Compared to the HLC method, the CDC-LT (IRR = 0.088, p< 0.0001) and PSC (IRR = 0.042, p< 0.0001) methods collected fewer mosquitoes when all other conditions were considered as being similar. On the other hand, the IRR of CDC-LT was higher than that of PSC, but the difference was not significant due to the overlap of their 95% confidence intervals. Also, fewer mosquitoes were collected in 2019 than in other years (IRR = 0.556, p = 0.0008) than in 2018 (Table 3, Figure 5D).
In terms of species richness, the performance of the tested sampling methods varied within each surveyed HD. The sampling methods used outdoors in the HDs of the North Region showed comparable performance, with however an exception in the Mayo Oulo HD where the species richness recorded with HLC was significantly higher than that obtained from CP or WET (p< 0.0001) (Figure 6). In the Centre region, no significant difference was found between the performance of HLC, CDC-LT and WET in the three surveyed HDs. For indoor collections carried out in the North Region, the species richness recorded with HLC was statistically higher than that of PSC in the Mayo Oulo and Pitoa HDs (p< 0.0001), but comparable in the Garoua HD. In the Centre region, no significant difference was found between HLC, CDC-LT and PSC (Figure 6). The classification of the collection methods according to their performance in recording a better species richness and collecting a great number of mosquitoes has been summarized in Table 5.
Figure 6 Performance of outdoor and indoor collection methods according to species richness in the surveyed health districts of the North and Centre regions (statistical method used: binomial approach to determine confidence intervals, n: number of mosquito species caught).
Table 5 Classification of the tested alternative methods to HLC according to their performance in recording a better species richness and collecting a great number of anopheline mosquitoes in the surveyed health districts of the North and Centre regions.
The current study assessed the performance of the collection methods used for sampling Anopheles mosquitoes during entomological studies in the North region during 2011-2014 and 2018 -2019 in the Centre Region in Cameroon. The performance of these methods was evaluated considering the species diversity and the relative abundance of anopheline samples collected. Considering the 29 Anopheles species recorded from these entomological databases, the species richness reported in this study was higher compared to the findings of a previous study conducted across 32 malaria endemic locations in Cameroon which reported 21 species (11). However, the diversity recorded here remains lower compared to the total 48 species previously reported (10, 26–29) and the 54 species recently reported in Cameroon (30).
The increase in the number of species reported in this study can be explained by the use of additional sampling methods such as CP and WET not previously tested as part of the inventory of anopheline fauna in Cameroon. In addition, the differences in collection year, number of collection days as well as prospected HDs could explain the difference in species diversity reported in this study compared to other previous studies. Also, as demonstrated by previous studies on species diversity (31–34), the diversity of the surveyed ecological zones (urban, semi-urban and rural) as part of this study may be perceived as a significant factor increasing the number of anopheline species reported in this study. However, this number is still lower than that reported other studies conducted in Cameroon (10, 26–30). This low species richness may be attributed to the restricted biogeographic area covered by the studies in the North and Centre Regions, contrary to the previous studies undertaken in almost all diverse biogeographical zones that exist in Cameroon. Indeed, as shown in previous studies, limited biogeographic zones are characterized by a low species richness, as might be expected when extrapolating from large biogeographical zones (35).
Among the species reported here, it should be mentioned that molecular identification of species of the An. gambiae complex was not fully completed because of the high numbers of An. gambiae s.l specimens (≈ 40% of the anophelines collected) recorded in the surveyed HDs. The results of the samples that were analysed were published by Ekoko and colleagues (36). Indeed, An. gambiae s.l is a complex of 8 species (37), of which 3 species namely An. arabiensis, An. coluzzii and An. gambiae are found in sympatry in the North (12, 36, 38) while An. coluzzii and An. gambiae coexist in the Centre region (13, 39). With the breakdown of An. gambiae complex species, we would have reported 31 species in the North region and 6 species in the Centre region, if all specimens collected belonging to the An. gambiae complex were subjected to species identification. However, even considering this breakdown, the total number of species reported in this study remains lower compared with that of the recently updated list of 54 anopheline species (30). Noteworthy, of the species reported in this study, only 25 are included among the 54 recorded by the updated list. The 4 other species, including An. ardensis, An. azaniae, An. barberellus and An. kingi are not in the updated list of 54 species (30). These new anopheline species were found in Mayo Oulo HD, a rural area, suggesting that the decrease in the urbanization gradient from urban to rural areas may have a strong influence on anopheline species diversity and distribution. In this study anopheline species richness was higher in Mayo Oulo HD, where anthropogenic activities mainly related to natural environment changes (e.g deforestation, construction of houses, extension, or construction of urban centres ….) are limited compared to Pitoa and Garoua HDs where these activities are important with the growing population pressure. Indeed, changes occurring in natural environments contribute to the destruction of mosquito habitats and their behavioral alterations (40, 41). Data presented here regarding the gradient of species diversity are in agreement with the recent published findings on mosquito diversity following human activity gradients (42–45). As mentioned in several previous studies an urban environment is unable to accommodate mosquitoes with different ecological niches as opposed to a rural environment (46, 47). The presence of these 4 new species among the samples collected during the current study prompts the need to update the list of anopheline species found in Cameroon. In addition to the 4 new species reported in this study, 2 other species, namely An. cinereus and An. demeilloni have already been reported by Fondjo and colleagues (48). Thus, 6 new anopheline species have been reported extending the list to 60 species in Cameroon. This number may further increase when combining to the existing mosquito collection methods commonly used (HLC, PSC, CDC light trap, WET…) and CP in the various biogeographical zones found in Cameroon.
The low species richness recorded in the Centre region is not in agreement with previous entomological studies conducted in this region (11, 49), In addition to the limited number of houses surveyed in the Centre region, the entomological collections performed during the DMC-MALVEC Project were only made in urban HDs in Yaoundé. These HDs are not representative of all the ecological patterns found in this Region, therefore the number of Anopheles species recorded in the prospected settings may constitute only a subset of anopheline species diversity of the Region. The HDs surveyed in the Centre Region may be compared with that of Garoua HD in the North Region since they are all located in urban areas. Nevertheless, the species richness recorded in the city of Garoua (nine species) is greater than that reported in Yaoundé c(5 species), which is the capital city of Cameroon exhibiting a long history of human influence on the environment, compared with Garoua town. These findings confirm that the changes in natural environment through pronounced human activities may influence the biodiversity of mosquitoes (40, 41). In addition, climate and vegetation in certain environments could offer better adaptive conditions to certain species of mosquitoes than to others. The various climatic and phytogeographical patterns observed across the country could have an influence on the distribution of species and consequently on the variation in species diversity and relative abundance of anophelines within the surveyed HDs and regions. This is in line with recent literature on the distribution of insect species biodiversity (50, 51), and may also explain the presence of species such as An. arabiensis, An. pharoensis and An. rhodesiensis in tropical areas.
In the other hand, as noted in this study, anopheline relative abundance follows a gradient of human activities with higher numbers observed in the Garoua urban HD compared to the semi-urban and rural HDs of Pitoa and Mayo-Oulo respectively. This result is in agreement with previous findings highlighting that mosquito relative abundance increases with intense human activities (42, 52–54). The gradient in relative abundance of anopheline species noted in the North region is opposite to that of species diversity hereafter evoked, confirming findings of previous studies (42–44).
Annual variations in mosquito population density were noted within the collection methods used and the surveyed HDs in the two regions. In the North region, the annual intra-population variations over the four years of the study may be essentially due to annual rainfall, but also to the number of mosquito species surviving in harsh conditions of the dry season. These surviving species are found in residual waterholes along seasonal rivers so called “mayos”. When conditions are restored, these species ensure the “founder effect”, i.e. the reconstitution of the population from a relatively small number of individuals from a mother population (55). In the Centre region, the collections were greater in the dry season than in the rainy season. Indeed, the study was conducted in lowlands consisting of marshy areas where market gardening is an important activity for the local human populations. During the rainy season, these areas are flooded, leading to the washing out of larval breeding sites. In the dry season, on the other hand, they are potential mosquito breeding sites, as the water stagnates and remains permanent, thus creating favorable conditions for the development and proliferation mosquitoes.
The spatial and temporal variation in the species diversity and abundances of anophelines noted in the surveyed HDs may mainly attributed to abiotic factors such as temperature, humidity, soil type, rainfall/precipitation, pH, and anthropogenic activities as mentioned in previous studies (56, 57). Although these factors were not investigated in this study their variation across surveyed regions, between seasons (rainy/dry) and over the years may affect the abundance and diversity of anopheline mosquito populations. Therefore, the spatial and temporal diversity and abundance in anopheline species observed in this study may explain the variation in malaria intensity usually noted across endemic settings.
The collection methods evaluated in the framework of this study showed variable performance within the surveyed HDs. In general, HLC appeared as the most efficient collection method compared to the tested conventional collection methods. This finding is consistent with previous studies supporting that HLC is the standard reference method for measuring human exposure to mosquito bites (14, 58), and the most efficient method in sampling anthropophilic Anopheles mosquitoes (59–62). However, given that HLC method poses ethical issues and requires a lot of resources, it is important to identify alternative methods for entomological surveillance in each epidemiological context in Cameroon. The results presented in this study demonstrate that the performance of the tested conventional methods varies from one HD to another. Overall, in the North region there was no significant difference in the number of mosquitoes collected by HLC, and the corresponding tested alternative outdoor and indoor methods. However, at the level of the HD, the difference between HLC and alternative methods was noticeable in some HDs, although it was mitigated when the collections were carried out at a larger scale, taking into account several HDs. However, this trend was not observed in the centre region, where the performance of HLC was significantly remarkable compared to the tested alternative methods as well at a limited scale (i.e. in one HD) as at a larger scale (several HDs).
The performance of PSC, CP and WET observed in the HDs of the North region could partly depend on the exophilic and exophagic behavior of certain species such as An. arabiensis, An. rufipes and An. pharoensis listed as dominant species in the Region. Since, biting and resting behavior varies from species to species, it might be assumed that the performance of the collection methods depends on the local mosquito populations. On the other hand, the arid and dry environments of the tropical/Sahelian climates generally offer harsh conditions (e.g., high temperature, intense heat, ….) unsuitable for insect resting. Therefore, CPs containing a small quantity of water as used during the sampling procedure in the North appear as a potential resting place for anophelines and other mosquito genera. This is in line with previous studies highlighting that An. arabiensis rest more frequently in granaries (63–65). Other species such as An. pharoensis, An. rufipes and An. rhodesiensis are likely to have the same behavior considering their high density observed in the CPs.
The performance of CDC-LT in collecting outdoor and indoor anophelines in HDs of the Centre region is in agreement with previous studies (66–69). This result suggests that CDC-LT is the suitable alternative method for collecting anophelines in the Centre region. Indeed, the light emitted by the trap attracts mosquitoes from a distance, increasing its performance even in areas where mosquito density is low. The low performance of PSC and WET in the centre region may be justified by the types of dwellings in which collection methods were performed. In the Centre Region, houses have many openings, most of them having no ceilings, with a space between the walls and the roof. Such a configuration of houses is not conducive to better performance of PSC and WET because mosquitoes have several escape routes. In the North region, on the other hand, houses have limited openings (one door and one window for the most) with no space between walls and roof, and consequently very few escape routes for mosquitoes. This configuration favors the performance of PSC and WET, as mentioned above.
The current study showed that the performance of collection methods on the basis of relative abundance and species richness varied according to the HDs and regions in Cameroon. The 29 species identified in this study showed highly heterogeneous distribution and fluctuations in species diversity and relative abundance across the surveyed HDs in the North and Centre regions. Indeed, HLC is confirmed in this study as the reference collection method for malaria vectors. Based on mosquito relative abundance, PSC, WET and CP are alternative methods to HLC in performing outdoor and indoor mosquito collection in the three surveyed HDs of the North region.
In the Centre region, CDC-LT is a suitable alternative to HLC for outdoor and indoor mosquito collections. Regarding species richness, HLC has proven to be more effective in collecting a large number of species outdoors and indoors in Mayo Oulo HD and indoors in Pitoa HD, in the North region. However, all the methods used in the Centre region showed similar trends in collecting both outdoor and indoor mosquito species in Ekie, Nkolbisson and Nkolondom HDs. This information is essential for the National Malaria Control Programme in the framework of malaria entomological surveillance in the surveyed HDs and Regions. Similar investigations considering other existing collection methods should be conducted in diverse ecosystems to ensure better malaria entomological surveillance in Cameroon and other African countries with similar landscapes.
The raw data supporting the conclusions of this article will be made available by the authors, without undue reservation.
For "Impact of Resistance" project, ethical clearance was provided by the National Ethics Committee of Cameroon under the reference number FWA IRB00001954 and approved through the authorization number 102/CNE/SE/09. Informed consent was obtained from heads of households or their representatives and community leaders before access to houses and bedrooms for mosquito collections. Regarding DMC-MALVEC project, the study protocol was approved by the Cameroon National Ethics Committee (N°2020/07/1573/L/CNERSH/SP) and by the Ministry of Public Health (N°D30- 633/AAR/MINSANTE/SG/DROS/NDG/). Administrative authorizations were delivered from the respective district officers of the Districts of Yaoundé I, IV and VII.
PN, JE, PA-A, JB and EF conceived and designed the study protocol. PN, MP, WEE, SEM, LRM, SP, JCT, LDA, ENB, HO, NN, RT, JB, EF, PA-A and carried out field data collection and mosquito identification. PN, MP, YNE and JE analysed and interpreted data. PN prepared the original draft. WEE, SEM, LRM, LDA, ENB, HO, NN, RT, JB, EF critically reviewed the manuscript. JE and RM validated the draft. All authors read and approved the final manuscript.
The author(s) declare that no financial support was received for the research, authorship, and/or publication of this article.
The authors declare that the research was conducted in the absence of any commercial or financial relationships that could be construed as a potential conflict of interest.
All claims expressed in this article are solely those of the authors and do not necessarily represent those of their affiliated organizations, or those of the publisher, the editors and the reviewers. Any product that may be evaluated in this article, or claim that may be made by its manufacturer, is not guaranteed or endorsed by the publisher.
1. Thomson MC. Emerging infectious diseases, vector-borne diseases, and climate change. Global Environ Change. (2014) 1:623–8. doi: 10.1007/978-94-007-5784-4-103
2. Gubler DJ. Vector borne diseases. Rev Sci Tech Off Int Epiz. (2009) 28(2):583–58. doi: 10.20506/rst.28.2.1904
3. WHO. Vector-borne diseases. (2017). Available at: http://www.who.int/mediacentre/factsheets/fs387/en/.
5. Wilson AL, Courtenay O, Kelly-Hope LA, Scott TW, Takken W, Torr SJ, et al. The importance of vector control for the control and elimination of vector-borne diseases. PloS Negl Trop Dis. (2020) 14(1):e0007831. doi: 10.1371/journal
6. WHO. World malaria report. Geneva: World Health Organization. (2022). Licence: CC BY-NC-SA 3.0 IGO.
7. WHO. Indoor residual spraying: use of indoor residual spraying for scaling up global malaria control and elimination Geneva. Geneva: Global Malaria Programme and World Health Organization (2006). WHO/HTM/MAL/2006.1112.
8. Manga L, Bouchite B, Toto JC, Froment A. La faune anophélienne et la transmission du paludisme dans une zone de transition forêt-savane au centre du Cameroun. Entomol Med. (1997) 91:4. doi: 10.12692/ijb/10.4.9-18.37
9. Manga L, Robert V, Messi J, Desfontaine M Carnevale P. Le paludisme urbain à Yaoundé Cameroun. 1. Etude entomologique dans deux quartiers centraux. Mem Soc Belge Ent. (1992) 35:155–62. doi: 10.4000/books.irdeditions.20879
10. Cohuet A, Simard F, Toto JC, Kengne P, Coetzee M, Fontenille D. Species identiÞcation within the Anopheles funestus group of malaria vectors in Cameroon and evidence for a new species. Am J Trop Med Hyg. (2003) 69:200 Ð205. doi: 10.4269/ajtmh.2003.69.200
11. Antonio-Nkondjio C, Kerah CH, Simard F, Awono-Ambene P, Chouaibou M, Tchuinkam T, et al. Complexity of the malaria vectorial system in Cameroon: contribution of secondary vectors to malaria transmission. J Med Entomol. (2006) 43(6):1215–21. doi: 10.1603/0022-2585(2006)43[1215:cotmvs]2.0.co;2
12. Mandeng SE, Awono-Ambene HP, Bigoga JD, Ekoko WE, Binyang J, Piameu M, et al. Spatial and temporal development of deltamethrin resistance in malaria vectors of the Anopheles Gambiae complex from North Cameroon. PloS One. (2019) 14(2):e0212024. doi: 10.1371/journal.pone.0212024
13. Piameu M, Nwane P, Toussile T, Mavridis K, Wipf NC, Kouadio FP, et al. Pyrethroid and Etofenprox Resistance in Anopheles Gambiae and Anopheles coluzzii from Vegetable Farms in Yaoundé, Cameroon: Dynamics, Intensity and Molecular Basis. Molecules. (2021) 26:5543. doi: 10.3390/molecules26185543
14. Service MW. A critical review of procedures for sampling populations of adult mosquitoes. Bull Entomol Res. (1977) 67:343–82. doi: 10.1017/S0007485300011184
17. Gillies MT, De Meillon BA. The anophelinae of africa south of the sahara (Ethiopian zoogeographical region). Pub S Afr Inst Med Res Jburg. (1968) 54:131–2.
18. Gillies MT, Coetzee M. A supplement to the Anophelinae of Africa South of the Sahara (Afro-tropical region). Pub S Afr Inst Med Res Jburg. (1987) 55:78–143.
19. Rydzanicz K, Lonc E. Species composition and seasonal dynamics of mosquito larvae in the Wroclaw, Poland area. J Vector Ecol. (2003) 28(2):255–66.
20. Sengil AZ, Akkaya H, Gonenc M, Gonenc D, Ozkan D. Species composition and monthly distribution of mosquito (Culicidae) larvae in the Istanbul metropolitan area, Turkey. Int J Biol Med Res. (2011) 2:415–24.
21. Ali N, Khan K, Kausar A. Study on mosquitoes of swat ranizai sub-division of malakand. Pakistan J Zool. (2013) 45(2):503–10.
22. Attaullaha M, Gula S, Bibia D, Andaleeba A, Ilahia I, Sirajb M, et al. Diversity, distribution and relative abundance of the mosquito fauna (Diptera: Culicidae) of Malakand and Dir Lower. Pakistan Braz J Biol. (2023) 83:e247374. doi: 10.1590/1519-6984.247374
24. Dziêczkowski A. Quantitative researches of the beech malacofauna in south-west of Poland. Prace Komisji Biologicznej PTPN. (1972) 35:243–332.
25. Hixon MA, Brostoff WN. Damselfish as keystone species in reverse: intermediate disturbance and diversity of reef algae. Science. (1983) 220:511–3. doi: 10.1126/science.220.4596.511
26. Hervy JF, Le Goff G, Geoffroy B, Herve JP, Manga L, Brunhes J. Les Anophe`les de la région afro-tropicale. CD-ROM. Paris, France: ORSTOM eédiitions (1998).
27. Brunhes J, Le Goff G, Bousses P. Anopheles afrotropicaux. V. Description du maˆle et des stades pre´- imaginaux d’An. deemingi et description dÕAn. eouzani n. sp. (Diptera: Culicidae). Ann Soc Entomol. (2003) Fr. 39:179 Ð 185.
28. Kengne P, Awono-Ambene P, Antonio-Nkondjio P, Simard F and Fontenille D. Molecular identification of the Anopheles nili group of African malaria vectors. Med Vet Entomol. (2003) 17:67Ð74. doi: 10.1046/j.1365-2915.2003.00411.x
29. Awono-Ambene HP, Kengne P, Simard F, Antonio-Nkondjio C, Fontenille D. Description and bio-nomics of Anopheles (Cellia) ovengensis (Diptera: Culicidae) a new malaria vector species of the Anopheles nili group from south Cameroon. J Med Entomol. (2004) 41:561Ð 568.
30. Irish SR, Kyalo D, Snow RW, Coetzee M. Updated list of Anopheles species (Diptera: Culicidae) by country in the Afrotropical Region and associated islands. Zootaxa. (2020) 4747(3):401–49. doi: 10.11646/zootaxa.4747.3.1
31. Hart DD. Diversity in stream insects: regulation by rock size and microspatial complexity. Verh Internat Verein Limnol. (1978) 20:209–35. doi: 10.1080/03680770.1977.11896700
32. Trush JWJ. The effects of area and surface complexity on the structure and formation of stream benthic communities. Blacksburg, VA: Virginia Polytechnic In- stitute (1979). Unpublished M.Sc. Thesis.
33. Williamson M. Relationship of species number to area, distance and other variables. In: Myers AA, Giller PS, editors. Analytical biogeography. An integrated approach to the study of animal and plant communities. London: Chapman and Hall (1988). p. 91–115.
34. Hart DD, Horwitz RJ. Habitat diversity and the species- area relationship: alternative models and tests. In: Bell SS, McCoy ED, Mushinsky HR, editors. Habitat structure: the physical arrangement of objects in space. London: Chapman and Hall (1991). p. 47–68.
35. Douglas M, Lake PS. Species richness of stream stones: an investigation of the mechanisms generating the species-area relationship. Oikos. (1994) 69:387–96. doi: 10.2307/3545851
36. Ekoko Eyisap W, AwonoAmbene P, Bigoga J, Mandeng S, Piameu M, Nvondo N, et al. Patterns of anopheline feeding/resting behavior and Plasmodium infections in North Cameroon, 2011-2014: implications for malaria control. Parasites Vectors. (2019) 12:297. doi: 10.1186/s13071-019-3552-2
37. Coetzee M, Hunt RH, Wilkerson R, Della Torre A, Coulibaly MB, Besansky NJ. Anopheles coluzzii and Anopheles amharicus, new members of the Anopheles Gambiae complex. Zootaxa. (2013) 3619:246–74. doi: 10.11646/zootaxa.3619.3.2
38. Chouaïbou M, Etang J, Brevault T, Nwane P, Hinzoumbé CK, Mimpfoundi R, et al. Dynamics of insecticide resistance in the malaria vector Anopheles Gambiae s.l. from an area of extensive cotton cultivation in Northern Cameroon. Trop Med Int Hlth. (2008) 13:476–86. doi: 10.1111/j.1365-3156.2008.02025.x
39. Nwane P, Etang J, Chouaibou M, Toto JC, Kerah-Hinzoumbé C, Mimpfoundi R, et al. Trends in DDT and pyrethroid resistance in Anopheles Gambiae s.s. populations from urban and agro-industrial settings in southern Cameroon. BMC Infect Dis. (2009) 9:163. doi: 10.1186/1471-2334-9-163
40. Afrane Y, Lawson B, Yan G, Githeko A, Zhou G. Effects of microclimatic changes caused by deforestationon the survivorship and reproductive fitness of Anopheles Gambiae in Western Kenya highlands. Am J Trop Med Hygiene. (2006) 74(5):772778. doi: 10.4269/ajtmh.2006.74.772
41. Olson S, Gangnon R, Silveira GA, Patz J. Deforestation and malaria in mâncio lima county, Brazil. Emerging Infect Dis. (2010) 16(7):11081115. doi: 10.3201/eid1607.091785
42. Pathy TS, Lee JM, Yek SH. Disturbance gradient and mosquito diversity pattern in areas surrounding Chini Lake - the second largest freshwater lake in Peninsular Malaysia. Biodiversity Data J. (2022) 10:e83800. doi: 10.3897/BDJ.10.e83800
43. Young KI, Buenemann M, Vasilakis N, Perera D and. Hanley KA. Shifts in mosquito diversity and abundance along a gradient from oil palm plantations to conterminous forests in Borneo. Ecosphere. (2021) 12(4):e03463. doi: 10.1002/ecs2.3463
44. Loaiza J, Dutari L, Rovira J, Sanjur O, Laporta G, Pecor J, et al. Disturbance and mosquito diversity in the lowland tropical rainforest of central Panama. Sci Rep. (2017) 7(1). doi: 10.1038/s41598-017-07476-2
45. Loaiza J, Rovira J, Sanjur O, Zepeda JA, Pecor J, Foley D, et al. Forest disturbance and vector transmitted diseases in the lowland tropical rainforest of central Panama. Trop Med Int Health. (2019) 24(7):849861. doi: 10.1111/tmi.1324
46. Steiger DM, Ritchie S, Laurance S. Mosquito communities and disease risk influenced by land use change and seasonality in the Australian tropics. Figshare. (2016) 9:387. doi: 10.6084/m9.figshare.c.3646076.v1
47. Hermanns K, Marklewitz M, Zirkel F, Kopp A, Kramer-SChadt S, Junglen S. Mosquito community composition shapes virus prevalence patterns along anthropogenic disturbance gradients. bioRxiv. (2021) 12:e66550. doi: 10.1101/2021.02.04.429754
48. Fondjo E, Toto JC, Tchouakui M, Ekoko Eyisap W, Patchoke S, Menze B, et al. High vector diversity and malaria transmission dynamics in five sentinel sites in Cameroon. Malar J. (2023) 22:123. doi: 10.1186/s12936-023-04552-z
49. Mbakop LR, Awono-Ambene P, Ekoko EW, Mandeng SE, Nwane P, Nono Feshu B, et al. Malaria transmission and vector resistance to insecticide in a changing environment: case of Simbock in Yaoundé, Cameroon. Front Trop Dis. (2019) 3. doi: 10.3389/fitd.2022.90221
50. Chester CC, Hilty JA. The yellowstone to yukon conservation initiative as an adaptive response to climate change. In: Leal Filho W, Barbir J, Preziosi R, editors. Handbook of climate change and biodiversity. Climate change management. Cham: Springer (2019). doi: 10.1007/978-3-319-98681-
51. Bush A, Rahel S, Andreas W, Kristine B, Beth C, Heiko B, et al. Connecting Earth observation to high-throughput biodiversity data. Nat Ecol Evol. (2017) 1:176. doi: 10.1038/s41559-017-0176
52. Schrama M, Hunting ER, Beechler BR, Guarido MM, Govender D, Nijland W, et al. Human practices promote presence and abundance of disease-transmitting mosquito species. Sci Rep. (2020) 10:13543. doi: 10.1038/s41598-020-69858-3
53. Lee JM, Wasserman RJ, Gan JY, Wilson RF, Rahman S, Yek SH. Human activities attract harmful mosquitoes in a tropical urban landscape. Ecohealth. (2020) 17(1):52–63. doi: 10.1007/s10393-019-01457-9
54. Medeiros-Sousa AR, de Oliveira Christe R, de Castro Duarte AMR, Mucci LF, Ceretii-Junior W, Marrelli MT. Effects of anthropogenic landscape changes on the abundance and acrodendrophily of Anopheles (Kerteszia) cruzii, the main vector of malaria parasites in the Atlantic Forest in Brazil. Malar J. (2019) 18:110. doi: 10.1186/s12936-019-2744-8
55. Barton NH, Charlesworth B. Annual review of ecology and systematics, Vol. 15. (1984). pp. 133–164 (32 pages), Published By: Annual Reviews.
56. Ahumada JA, LaPointe DA, Samuel MD. Modeling the population dynamics of Culex quinquefasciatus (Diptera: Culicidae), along an elevational gradient in Hawaii. J Med Entomol. (2004) 41:1157–70. doi: 10.1603/0022-2585-41.6.1157
57. Reisen W, Cayan D, Tyree M, Barker C, Eldridge B, Dettinger M. Impact of climate variation on mosquito abundance in California. J Vector Ecol. (2008) . 33:89–98. doi: 10.3376/1081-1710(2008)33[89:IOCVOM]2.0.CO;2
59. Lima JBP, Rosa-Freitas MG, Rodovalho CM, Santos F, Oliveira RL. Is there an efficient trap or collection method for sampling Anopheles darlingi and other malaria vectors that can describe the essential parameters affecting transmission dynamics as effectively as human landing catches? a review. Mem Inst Oswaldo Cruz. (2014) 109:685–705. doi: 10.1590/0074-0276140134
60. Mathenge EM, Misiani GO, Oulo DO, Irungu LW, Ndegwa PN, Smith TA, et al. Comparative performance of the Mbita trap, CDC light trap and the human landing catch in the sampling of Anopheles arabiensis, An. funestus and culi- cine species in a rice irrigation scheme in western Kenya. Malar J. (2005) 4:7. doi: 10.1186/1475-2875-4-7
61. Govella NJ, Chaki PP, Mpangile JM, Killeen GF. Monitoring mosquitoes in urban Dar es Salaam: evaluation of resting boxes, window exit traps, CDC light traps, Ifakara tent traps and human landing catches. Parasites Vectors. (2011) 4:40. doi: 10.1186/1756-3305-4-40
62. Dia I, Diallo D, Duchemin JB, Ba Y, Konate L, Costantini C, et al. Comparisons of human-landing catches and odor-baited entry traps for sampling malaria vectors in Senegal. J Med Entomol. (2005) 42:104–9. doi: 10.1093/jmedent/42.2.104
63. Githeko A, Service M, Mbogo C, Atieli F. Resting behavior, ecology and genetics of malaria vectors in large scale agricultural areas of Western Kenya. Parassitologia. (1996) 38:481–9.
64. Degefa T, Githeko AK, Lee MC, Yan G, Yewhalaw D. Patterns of human exposure to early evening and outdoor biting mosquitoes and residual malaria transmission in Ethiopia Acta Tropica. (2021). doi: 10.1016/j.actatropica.2021.105837
65. Kibret G, Wilson G, Ryder D, Tekie H, Petro B. Environmental and meteorological factors linked to malaria transmission around large dams at three ecological settings in Ethiopia Solomon. Malar J. (2019) 18:54. doi: 10.1186/s12936-019-2689-y
66. Wong J, Bayoh N, Olang G, Killeen GF, Hamel MJ, Vulvule JM, et al. Standardizing operational vector sampling techniques for measuring malaria transmission intensity: evaluation of six mosquito collection methods in western Kenya. Malar J. (2013) 12:143. doi: 10.1186/1475-2875-12-143
67. Onyango SA, Kitron U, Mungai P, Muchiri EM, Kokwaro E, King CH, et al. Monitoring malaria vector control interventions: effectiveness of five diferent adult mosquito sampling methods. J Med Entomol. (2013) 50(5):1140–51. doi: 10.1603/ME12206
68. Sikaala CH, Killeen GF, Chanda J, Chinula D, Miller JM, Russell TL, et al. Evaluation of alternative mosquito sampling methods for malaria vectors in Lowland South-East Zambia. Parasit Vectors. (2013) 6:91. doi: 10.1186/1756-3305-6-91
69. Briët OJ, Huho BJ, Gimnig JE, Bayoh N, Seyoum A, Sikaala CH, et al. Applications and limitations of Centers for Disease Control and Prevention miniature light traps for measuring biting densities of African malaria vector populations: a pooled-analysis of 13 comparisons with human landing catches. Malar J. (2015) 14:247. doi: 10.1186/s12936-015-0761-9
Keywords: mosquito sampling methods, performance, malaria vector surveillance, Anopheles, Cameroon
Citation: Nwane P, Piameu M, Emalio YN, Ekoko WE, Mandeng SE, Mbakop LR, Patchoke S, Toto J-C, Alenou LD, Bikoi EN, Onguina H, Nvondo N, Mimpfoundi R, Tabue R, Bigoga J, Fondjo E, Awono-Ambene P and Etang J (2024) Assessing the performance of five adult mosquito sampling methods for malaria vector surveillance in various ecosystems in Cameroon. Front. Trop. Dis 5:1235146. doi: 10.3389/fitd.2024.1235146
Received: 05 June 2023; Accepted: 12 January 2024;
Published: 13 March 2024.
Edited by:
Baldwyn Torto, International Centre of Insect Physiology and Ecology (ICIPE), KenyaReviewed by:
Derrick Mathias, University of Florida, United StatesCopyright © 2024 Nwane, Piameu, Emalio, Ekoko, Mandeng, Mbakop, Patchoke, Toto, Alenou, Bikoi, Onguina, Nvondo, Mimpfoundi, Tabue, Bigoga, Fondjo, Awono-Ambene and Etang. This is an open-access article distributed under the terms of the Creative Commons Attribution License (CC BY). The use, distribution or reproduction in other forums is permitted, provided the original author(s) and the copyright owner(s) are credited and that the original publication in this journal is cited, in accordance with accepted academic practice. No use, distribution or reproduction is permitted which does not comply with these terms.
*Correspondence: Philippe Nwane, cGhpbGlubzA3QHlhaG9vLmZy
Disclaimer: All claims expressed in this article are solely those of the authors and do not necessarily represent those of their affiliated organizations, or those of the publisher, the editors and the reviewers. Any product that may be evaluated in this article or claim that may be made by its manufacturer is not guaranteed or endorsed by the publisher.
Research integrity at Frontiers
Learn more about the work of our research integrity team to safeguard the quality of each article we publish.