- 1Faculty of Medicine, Department of Dermatology, University of Amsterdam, Amsterdam, Netherlands
- 2Dermatology Service, Ministry of Health, Paramaribo, Suriname
- 3Freudenthal Institute & Utrecht Institute for Pharmaceutical Sciences (UIPS), Utrecht University, Utrecht, Netherlands
- 4Department of Microbiology, Immunology and Pathology, Colorado State University, Fort Collins, CO, United States
- 5Department of Infectious Diseases, Leiden University Medical Center, Leiden, Netherlands
- 6Department of Pathology, Academic Hospital, Paramaribo, Suriname
The new world was considered free of leprosy before the arrival of Europeans. In Suriname, historical migration routes suggest that leprosy could have been introduced from West Africa by the slave trade, from Asia by indentured workers, from Europe by the colonizers, and more recently by Brazilian gold miners. Previous molecular studies on environmental and ancient samples suggested a high variability of the strains circulating in the country, possibly resulting from the various migration waves. However, a current overview of such diversity in humans still needs to be explored. The origin and spread of leprosy in Suriname are investigated from a historical point of view and by strain genotyping of Mycobacterium leprae from skin biopsies of 26 patients with multibacillary leprosy using PCR-genotyping and whole-genome sequencing. Moreover, molecular signs of resistance to the commonly used anti-leprosy drugs i.e. dapsone, rifampicin and ofloxacin, were investigated. Molecular detection was positive for M. leprae in 25 out of 26 patient samples, while M. lepromatosis was not found in any of the samples. The predominant M. leprae strain in our sample set is genotype 4P (n=8) followed by genotype 1D-2 (n=3), 4N (n=2), and 4O/P (n=1). Genotypes 4P, 4N, 4O/P are predominant in West Africa and Brazil, and could have been introduced in Suriname by the slave trade from West Africa, and more recently by gold miners from Brazil. The presence of the Asian strains 1D-2 probably reflects an introduction by contract workers from India, China and Indonesia during the late 19th and early 20th century after the abolition of slavery. There is currently no definite evidence for the occurrence of the European strain 3 in the 26 patients. Geoplotting reflects internal migration, and also shows that most patients live in and around Paramaribo. A biopsy of one patient harbored two M. leprae genotypes, 1D-2 and 4P, suggesting co-infection. A mutation in the dapsone resistance determining region of folP1 was detected in two out of 13 strains for which molecular drug susceptibility was obtained, suggesting the circulation of dapsone resistant strains.
Introduction
The Americas are considered free of leprosy prior to the arrival of Europeans (1).
In Suriname, a country that is part of the Guiana Shield located in the northern part of South America, the disease was first described in the mid-18th century. From 1790 until the second half of the 20th century, leprosy patients were legally confined in leprosaria, accounting for 1% of the population in the middle of the 19th century (2, 3). At the beginning of the 20th century, 2.5-3% of the population of the capital Paramaribo suffered from leprosy (4). Dapsone (DDS) was introduced in 1946 and resulted in a sharp decline in the incidence of the disease and the closure of leprosy asylums in the 1960s and 1970s (5). Relapses after initial improvement by dapsone therapy were reported as early as 1955, hypothesizing a possible bacterial resistance, but this has never been proven to date (6). Multiple drug therapy (MDT: a combination of dapsone, clofazimine and rifampicin) was introduced in the 1980s and contributed to the rapid decrease in the number of new cases in Suriname (7). However, leprosy is still present in the country, and a steady average of 20 to 25 new cases annually in a population of around 600,000 inhabitants is reported (8, 9).
Leprosy is mainly caused by the non-cultivable pathogen Mycobacterium leprae and, to a lesser extent, Mycobacterium lepromatosis (10, 11). M. leprae shows low genetic variability between strains from different locations. This created the basis for the establishment of a robust genotyping system characterized by four single nucleotide polymorphism (SNP) types (1–4) and 16 SNP subtypes (A–P) used to retrace local and large scale transmission of the pathogen (1, 12, 13). The close relationship between human migration and the introduction and spread of infectious diseases, a well-documented phenomenon, is particularly evident for M. leprae (14, 15). Indeed, the introduction of the pathogen in the Americas is ascribed to the first Europeans who settled in the new world (1, 16).
Suriname has a rich history of migration waves that began in the early 17th century. European settlers, predominantly Dutch and Portuguese Jews, played a significant role in establishing a plantation economy (17). The workforce on the plantations consisted of enslaved West-Africans, many of whom escaped to the hinterland, establishing Maroon communities (18). Slavery was abolished in 1863, leading to the recruitment of indentured workers from China, British India (now India) and the Dutch East Indies (now Indonesia) in the period 1860s-1920s to replace the formerly enslaved West-Africans (19). The diverse origin of the population suggests that leprosy may have been introduced in the country at different times and from different countries. Previous research reported the presence of a M. leprae strain harboring genotype 4 in DNA extracted from the bones of a West-African adolescent buried at the former leprosarium in the 19th century. Moreover, M. leprae genotype 1 or 2 was identified in DNA extracted from soil samples collected around armadillo burrows in two former leprosaria in Suriname (20, 21). These findings indicate a high variability of the M. leprae strains circulating in the country, possibly resulting from the various immigration waves. However, no information is currently available regarding the genetic diversity of M. leprae strains in contemporary human samples within the country.
Consequently, solid biological evidence supporting a multi-ethnic origin of leprosy in Suriname is lacking.
This paper aims to investigate the origin and spread of leprosy in Suriname by genotyping of M. leprae strains isolated from skin biopsies of multibacillary (MB) patients from various ethnic backgrounds. Additionally, it explores the molecular resistance to commonly used anti-leprosy drugs, namely dapsone, rifampicin and ofloxacin.
Materials and methods
Patients and clinical samples
A total of 27 formalin fixed biopsies that were collected between 2014 and 2019 from skin lesions of 26 untreated MB patients diagnosed at the Dermatology Service in Paramaribo, were recovered from the Department of Pathology of the Academic Hospital in Paramaribo. Examination of patients, clinical diagnosis and collection of epidemiological data were performed by the Dermatology Service in Paramaribo. Leprosy patients were classified according to the Ridley-Jopling classification (22). Each patient’s self-reported ethnicity that had been recorded was used for the study. Biopsies were sent to Leiden University Medical Center where DNA extraction, molecular identification of M. leprae and M. lepromatosis by qPCR and strain genotyping by PCR-sequencing were performed.
DNA extraction
DNA isolation from paraffined biopsies
DNA was isolated using QIAamp UCP Pathogen Mini Kit (Qiagen) as per the manufacturer’s instructions with additional modifications. The excess of paraffin surrounding the biopsies was removed using sterile surgical blades. To remove the remaining paraffin, tissues were transferred to 1,5 ml tubes (Eppendorf) and incubated in 1 ml of xylene for 30 minutes at room temperature. After centrifugation at 14,000 rpm for 5 minutes, supernatant was removed and xylene extraction was repeated as described above. Biopsy tissues were washed twice by adding 1 ml of absolute ethanol and centrifuged for 5 minutes at 14,000 rpm. To remove the ethanol, tubes were incubated with an opened cap at 37°C for 15 minutes. The biopsies were cut into thin slices using sterile surgical blades and transferred to new microtubes (Sarstedt) containing 400 μl buffer ATL (provided in Qiagen kit) and three sterile 3 mm glass beads (VWR). Samples were homogenized twice in a Precellys 24 tissue homogenizer (Bertin) at 5000 rpm for 45 seconds with 5 minutes of incubation on ice in between. Next, 20 μl of proteinase K (provided in the Qiagen kit) was added and tubes were incubated at 55°C with continuous stirring at 1300 rpm for 2 hours. After centrifugation for 1 minute at 14,000 rpm supernatants were transferred to new microtubes containing 250 μl of 0,1 mm Zirconia beads (Biospec) and homogenized in the tissue homogenizer as described above. To remove the beads, tubes were centrifuged at 14,000 rpm for 1 minute and supernatants were transferred to new microtubes. Subsequently, 20 µl of proteinase K was added prior to incubation at 55°C with constant stirring at 1,300 rpm for 30 minutes. Then 200 µl APL2 buffer (provided in the kit) was added and tubes were incubated at 70°C with constant stirring at 1300 rpm for 10 minutes. After absolute ethanol precipitation (300 μl), column extraction was performed according to the manufacturer’s instructions.
Molecular identification of M. leprae and M. lepromatosis
RLEP qPCR was performed on isolated DNA samples as previously described (23). The mix included 12.5 µl TaqMan Universal Master Mix II (Applied Biosystems, Foster City, CA), 0.5 µl (25 µM) forward and reverse primers, 0.5 µl (10 µM) TaqMan probe and 5 µl template DNA or water (negative control) or M. leprae DNA (positive control provided by BEI resources, Manassas, VA, strains Br4923 and Thai-53 DNA) were mixed in a final volume of 25 µl. DNA was amplified using the following protocol: 2 min at 50°C and 10 min at 95°C followed by 40 cycles of 15 s at 95°C and 1 min at 60°C with a QuantStudio 6 Flex Real-Time PCR System (Applied Biosystems). Presence of M. leprae DNA was considered if a sample was positive for RLEP qPCR with a cycle threshold (Ct) lower than 37.5.
PCR to detect M. lepromatosis was performed as described previously (24). Briefly, the 244 bp hemN sequence (primers LPM244) (25) was amplified in 50 µl of reaction by addition of 10 µl 5x Gotaq® Flexi buffer (Promega, Madison, WI), 5 µl MgCl2 (25 mM), 2 µl dNTP mix (5 mM), 0.25 µl Gotaq® G2 Flexi DNA Polymerase (5 u/µl), 5 µl (2 µM) forward and reverse primers and 5 µl template DNA, water (negative control) or M. lepromatosis DNA (kindly provided by Dr Han, Clinical Microbiology Laboratory, the University of Texas M. D. Anderson Cancer Center, Houston, TX, USA) as a positive control. PCR mixes were subjected to 2 min at 95°C followed by 40 cycles of 30 s at 95°C, 30 s at 53°C and 30 s at 72°C and a final extension of 10 min at 72°C. PCR products (15µl) were used for electrophoresis in a 3.5% agarose gel at 130V. Amplified DNA was visualized by Midori Green Advance staining (Nippon Genetics Europe, Dueren, Germany) using iBright™ FL1000 Imaging System (Invitrogen, Carlsbad, CA).
Genotyping
To determine the genotype (1, 2, 3 or 4) of M. leprae, SNP-14676 (locus 1), SNP-1642875 (locus 2) and SNP-2935685 (locus 3) were amplified and sequenced as described with minor modifications (12): PCRs were performed with 5 µl of template DNA using the aforementioned PCR mixes and forward and reverse primers for loci 1-3 in a final volume of 50 µl. DNA was denatured for 2 minutes at 95°C, following 45 cycles of 30 s at 95°C, 30 s at 58°C and 30 s at 72°C and a final extension cycle of 10 min at 72°C. PCR products were resolved by agarose gel electrophoresis as explained above. PCR products showing a band were purified prior to sequencing using the Wizard SV Gel and PCR Clean-Up System (Promega, Madison, WI). Sequencing was performed on the ABI3730xl system (Applied Biosystems, Foster City, CA) using the BigDye Terminator Cycle Sequencing Kit (Thermo Fisher Scientific, Waltham, MA).
Two set of primers covering the position 978589 and 1476525 (16) were used to differentiate between the genotypes 4N, 4O and 4P. For the PCR reaction, a total of 3 μl of each purified DNA sample, or the positive control (Thai53, BEI resources NR-19352) or the negative control (water), was added to a total PCR reaction volume of 50 μl containing 25 μl of DreamTaq Green PCR master Mix 2X (Thermofisher), and 200 nM of each forward and reverse primers. The reaction mixtures were submitted to an amplification started with an initial denaturation step of 1 min at 95°C, followed by 40 cycles of 15s at 95°C, 15s at 55°C-62°C, and 1min at 72°C followed by a final extension at 72°C for 5min. Amplification was checked on agarose gel 1% and sent for sequencing through Genewiz company. Alignment of amplicon was performed using Codon code aligner v9.0.2 underfree access.
The RLEP positive M. leprae DNA samples were then transferred to the Department of Microbiology, Pathology and Immunology, Colorado State University, for whole-genome sequencing and further genotyping. DNA was quantified using the Qubit Fluorometer instrument (Thermofisher).
Library preparation
Because of the low quality of DNA extracted from FFPE, DNA libraries were prepared without fragmentation using 1 to 100ng of starting material in 50µl of Tris-HCl 10mM. The 50µl of each extract was used for library preparation using the Kapa Hyper Prep kit (Roche, Switzerland) as per the KAPA HyperCap Workflow (v3.2). UDI primer mixes (Roche) were used to index each library as recommended in the protocol.
M. leprae enrichment of libraries
No bacterial DNA enrichment was performed during DNA extraction, so enrichment for M. leprae DNA was approached using bait capture. Based on our previous investigations, all samples with Cts around 25 were considered for capture (24).
To obtain enough M. leprae coverage for DNA extract from FFPE samples, libraries were target enriched for the M. leprae genome using the KAPA HyperExplore Max 3Mb custom bait capture kit (Roche, IRN 1000013073, M. leprae Br4923). Libraries were pooled with one to three other libraries with a similar RLEP-qPCR Ct for a total amount of 1.5µg of DNA library according to the KAPA HyperCap Workflow (v3.2). Each enrichment was followed by an amplification step using the Kapa library amplification kit following manufacturer recommendations and a purification step using AMPure beads. Libraries were quantified using the Qubit dsDNA BR (Thermofisher) kit and the quality was assessed on a TapeStation 4150 using the D1000 screen tape (Agilent). Libraries were pooled and sequenced single-end on NextSeq 500.
Genomic data analysis
Raw reads were processed as described elsewhere (26). Briefly, reads were adapter- and quality-trimmed with Trimmomatic v0.35 (27). The quality settings were “SLIDINGWINDOW:5:15 MINLEN:40”. Preprocessed reads were mapped onto the M. leprae TN reference genome (GenBank AL450380.1) with Bowtie2 v2.3.4.2 (Langmead 20120). SNP calling was done using VarScan v2.3.9 (28). To avoid false-positive SNP calls, duplicate reads were omitted from downstream analyses and the following cutoffs were applied for VarScan: minimum overall coverage of five non-duplicated reads, minimum of three non-duplicated reads supporting the SNP, mapping quality score >8, base quality score >15, and a SNP frequency above 80%. All VCF files were analyzed using snpEff v4.3 (29). Heterozygous sites (20-80% SNP frequency), repetitive regions and ribosomal RNA genes in the reference sequence were omitted. Indel calling was done using Platypus v0.8.1 followed by manual curation (30). Putative unique variants of the strains associated with genotyping and drug resistance determining regions of rpoB, folP1 and gyrA were manually checked and visualized using the Integrative Genomics Viewer (31).
Comparative genomics
The phylogenetic analysis was performed using a concatenated SNP alignment. Maximum parsimony (MP) trees were constructed in MEGA11 (32) with the 12 new genomes from this study (Table 1) and 312 previously published genomes using 500 bootstrap replicates and M. lepromatosis as an outgroup (24, 33). Sites with missing data were partially deleted (arbitrary 80% coverage cutoff), resulting in 5625 variable sites used for the tree calculation.
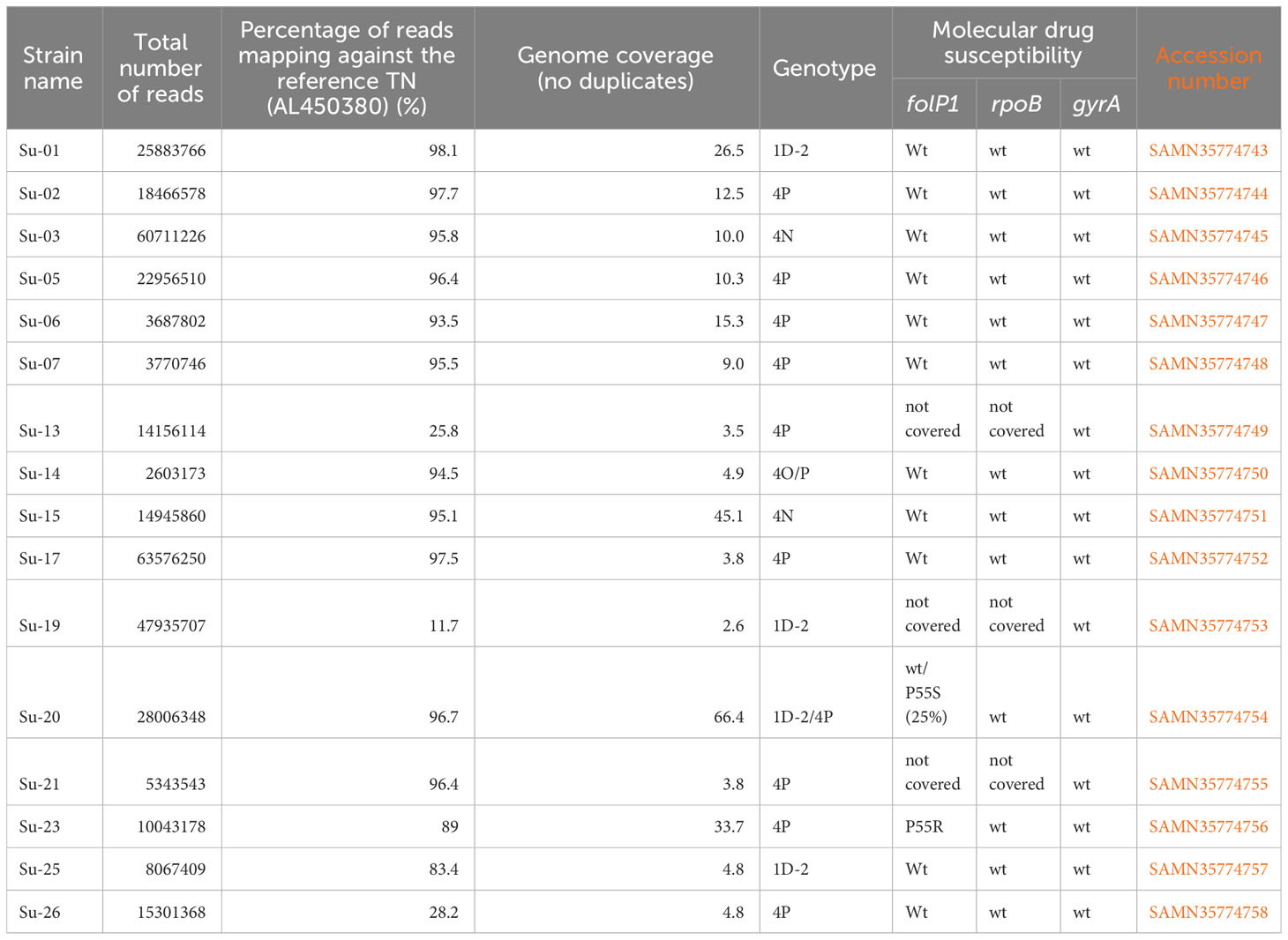
Table 1 List of the 16 newly sequenced genomes – sequencing information, genotyping, molecular drug resistance and SAM numbers wt: sequence wild-type.
Geoplotting
The geomap (Figure 1) was constructed using residence and ethnicity of the patients and the results of M. leprae genotyping, as presented in Tables 1 and 2.
Results
Patients, ethnic origin and identification of M. leprae
The 26 patients (9 females and 17 males; age ranging from 12 to 71, mean 37,4 years) included in this retrospective study belong to six ethnic groups: Maroons = African origin (n=11); Creoles/mixed (n=6); Hindustani= predominantly from the northeastern part of India (n=4), Javanese origin= from the Indonesian island of Java (n=3) and Chinese origin (n=1); Indigenous = autochthonous people (n=1) (Table 2, Figure 1). The Ridley-Jopling classification as well as the histopathological classification are given in Table 2. The 27 biopsies presented a median bacillary index of 2 (Table 2).
A total of 26 out of the 27 DNA extracts were positive for M. leprae by RLEP qPCR with a Ct ranging from 21.7 to 37.7 (Table 2). All samples were negative for M. lepromatosis in qPCR.
Genotyping and comparative genomics
Library preparation was performed on 19 samples (Ct ranging from 21.69 to 28.11, Table 2). Three samples did not pass the quality control, and 17 samples were submitted to bait enrichment and Illumina sequencing. A total of 12 genomes were obtained with coverage sufficient for comparative genomics (>4) (Table 1). The four other sequenced genomes had a coverage between 2.5 and 4 which was sufficient for manual checking of the genotype using the Integrative Genome Viewer (v2.8.13) (Table 3). For all other strains, genotyping was determined by PCR-sequencing (Table 2).
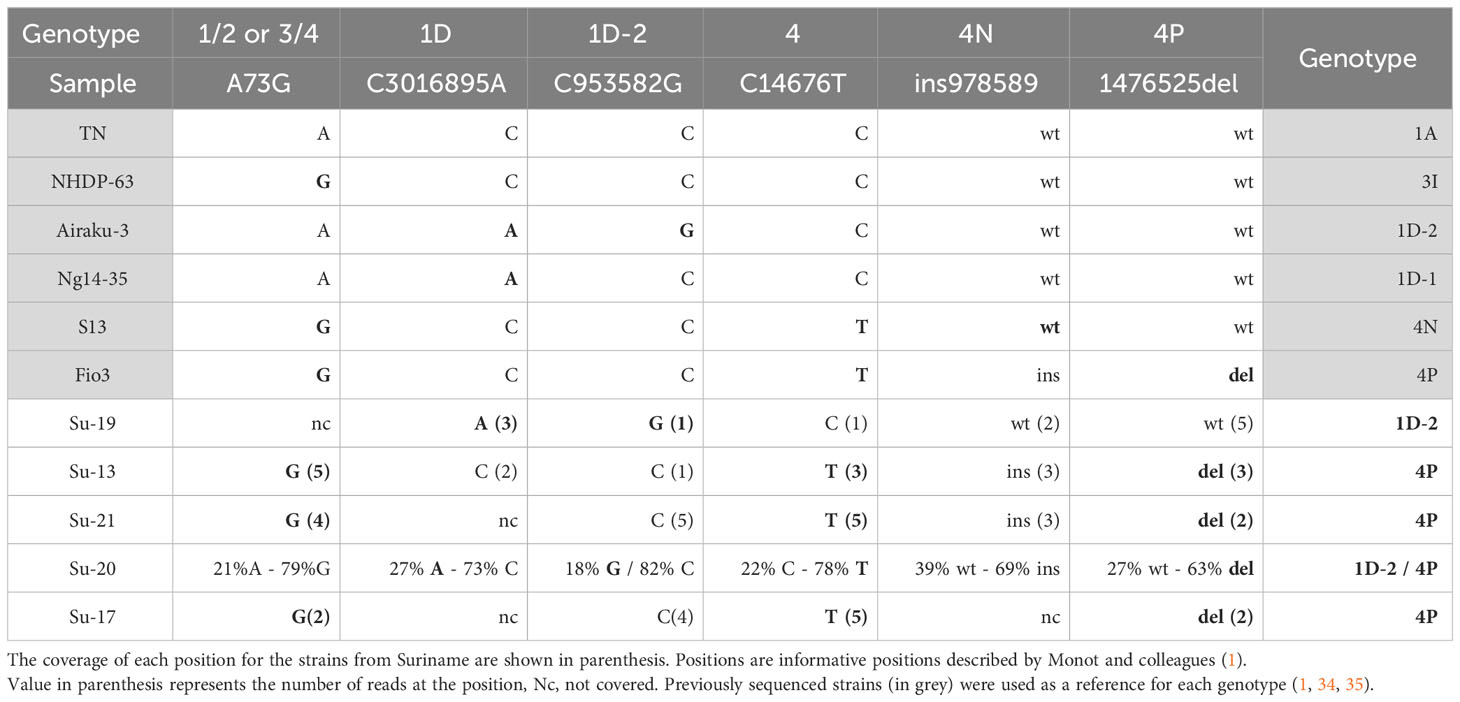
Table 3 Single nucleotide polymorphisms manually checked to decipher the M. leprae genotypes in the samples with insufficient coverage by genome sequencing and compared to already published genomes from the different genotypes (in grey).
Out of the 26 positive DNA extracts, genotyping was undetermined for two samples (Su-11 and Su-22), and inconclusive (no SNP type determined) for four samples (Su-08, Su-09, Su-12, Su-16). Partial genotyping (SNP-type alone or inconclusive SNP-subtype) was obtained for five samples (Su-4, Su-10, Su-18, Su-24, and, Su-27), while full genotyping was obtained for 16 strains (Table 2).
Among the 16 full or partial genomes available, three belong to the 1D-2 genotype commonly found in Asia (India, Nepal, Bangladesh, Japan) (24, 26) (Table 3; Figure 2A), two belong to genotype 4N, one to genotype 4O/P and twelve harbor the genotype 4P mostly found in West Africa and Brazil (Table 3; Figure 2B) (36). One sample, Su-20 presents with two strains, one from genotype 1D-2 (around 25% of the reads) and one with genotype 4P (around 75% of the reads) (Table 3).
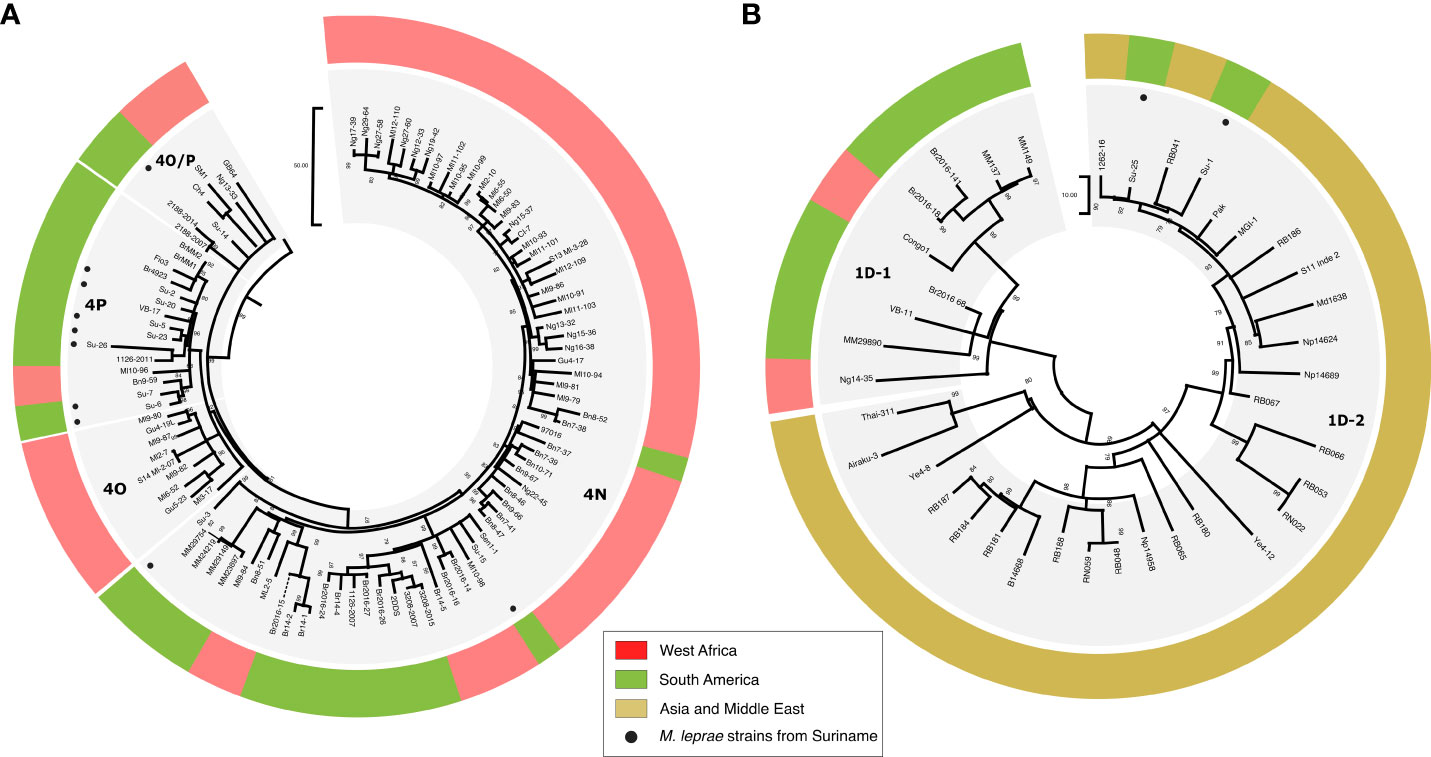
Figure 2 Maximum parsimony tree of 317 M. leprae genomes highlighting the new genomes from Suriname harboring the genotypes 4 (A) and 1D (B). The tree was initially constructed using 317 genomes, 500 bootstrap replicates, and M. lepromatosis as an outgroup. Sites with missing data were partially deleted (80% coverage cutoff), resulting in 5625 variable sites used for the tree calculation. Subtrees corresponding to branches were then retrieved in MEGA. Corresponding genotypes are indicated on the side of each subtree. Newly sequenced genomes are shown with circles, and genomes are colored-code based on the region where the isolate was identified. The branch length represents the number of SNPs. RB, Bangladesh; Np/Md, Nepal; Br or MM, Brazil; VB, Venezuela; Pak, Pakistan; 1262-16/S11/MGI-1, India; Ng, Niger; Ye, Yemen; Airaku-3, Japan; Thai, Thailand; Number (e.g, 20H02900), French Guyana; Bn, Benin; Ml, Mali.
A mutation in the resistance determining region of folP1 was detected in the sample with two strains Su-20, (P55S, 25% of the reads) and Su-23 (P55R), suggesting that the strains are resistant to dapsone (Table 1).
Geoplotting
The geoplotting (Figure 1) shows that most of the patients in this study appear to live in Paramaribo or south of this city, in districts in the catchment area along the Suriname River. Data from the Dermatology Service shows that most leprosy patients registered in recent decades also live in these areas (5). The plot in Figure 1 therefore seems to be a good reflection of the actual distribution of leprosy across Suriname
Genotyping of M. leprae strains was correlated with the ethnic group of each patient. Six of the 11 Maroons carried type 4; 3 of the 6 Creoles/Mixed carried type 4; 2 of the 4 Hindustani patients and 2 out of 3 of Javanese origin carried type 1; the only indigenous patient carried type 4 and the only Chinese type 4 (Figure 1 and Tables 1 and 2). The patient presenting with the mixed infection (type 1 and 4) is of Hindustani ethnicity.
Discussion
The origin of leprosy in Suriname has been discussed since colonial times, with blame and associated stigma being apportioned to different ethnic groups, primarily those of African and Jewish descent. This led to stigmatizing labels such as ‘nengre siki’ (‘disease of the blacks’) and ‘yu-siekie’ (‘disease of the Jews’) (2, 3). Historical migration routes suggest that leprosy could have been introduced in Suriname through various means. It may have arrived from West Africa through the slave trade, from Asia by indentured workers, from Europe by the European colonizers and Portuguese Jews, and more recently from Brazil, by wildcat gold miners (37, 38). Previous studies on ancient remains (19th century) and soil samples collected from different sites in the country have indicated the co-existence of multiple M. leprae strains in Suriname, possibly originating from different sources (20, 21).
Our study confirms this hypothesis and provides a better understanding of the different introductions of the disease into Suriname. Genotyping of modern human M. leprae strains circulating in the Surinamese population suggests multiple introductions of M. leprae from West Africa, Asia and Brazil. The predominant strain in our sample set is represented by genotype 4P, followed by two strains harboring genotype 4N and one harboring genotype 4O/P. These genotypes are predominant in West Africa and Brazil. Comparative genomics analysis revealed that the Surinamese strains are clustering with strains from West Africa (Su-6, Su-7, Su-15), from Brazil (Su-5, Su-26, Su-23), in a broader cluster including strains from Brazil and Venezuela (Su-20, Su-2) or are branching alone in the three (Su-3) (Figure 2A). These data suggest that the M. leprae genotypes were introduced during the slave trade into South America between the 17th century and the abolishment of Dutch colonial slavery in 1863, over which period approximately 550,000 enslaved West-Africans were transported to Suriname. These genotypes could also have been introduced in the 17th century from Brazil when the Dutch, along with the Portuguese Jewish plantation owners and their enslaved West-African workers, were expelled and moved to Suriname. They could also have been introduced in recent decades by Brazilian gold seekers (garimpeiros) who have been working in gold mines in the interior of Suriname.
One notable genotype encountered is genotype 1D, which is separated in three different clusters in the M. leprae phylogeny and found in different regions worldwide. One cluster, called 1D-Malagasy is found predominantly in Madagascar and the Comoros, the second cluster called 1D-1 is mostly found in Brazil and Venezuela, while the third one, called 1D-2, is mostly observed in Asian countries such as India, Bangladesh, Nepal, Thailand, and Japan (13). Some might expect that the genotype 1D circulating in Suriname would belong to the 1D-1 cluster due to the proximity with Brazil. However, all 1D strains belong to 1D-2 cluster (n=3), suggesting that the 1D strains were introduced during the 19th century and early 20th century following the large migration of Asian contract workers (India, Indonesia and China) to Suriname.
A conspicuous observation is the absence of the genotype 3I in the samples analyzed. This genotype is predominant in North and Central America and has also been reported in the north of Brazil, Colombia and in Venezuela (13, 26). Previous ancient DNA studies have hypothesized that the first European settlers brought leprosy with genotype 3I into the new world. The absence of this genotype in our biopsy samples suggests that if present, the genotype 3I is not predominant.
To get deeper into the transmission pattern in Suriname, we also seek to correlate patients’ ancestry with the strain’s genotype. Geo-plotting showed that the majority of the patients live in and around Paramaribo. Enslaved West-Africans fled from the plantations to the hinterland, establishing Maroon communities along the Suriname River (and other rivers) upstream, where they were safer from the authorities. In recent times, these Maroons have moved to the capital Paramaribo, where the majority of patients is also diagnosed. The Javanese and Indian-Hindustani migrants mainly settled on plantations in the coastal area. Strain type 4 was not only found in people of West-African descent and type 1 not only in Asians, suggesting that the current distribution of strains is not linked with ancestry, and interethnic contacts in daily life and personal relationships, including marriages between Surinamese people of different ethnicity probably explain it.
In an earlier report, 3 out of 28 samples taken at the entrance of armadillo holes in former leprosaria in Suriname were positive for M. leprae DNA (21). The positive soil samples from armadillo burrows may also indicate that armadillos play a role in the persistence of M. leprae in the environment in Suriname (39, 40). Also, armadillos are hunted and their meat is part of the food consumption of inhabitants in the interior of Suriname, especially among Maroons. Additionally, during a field trip into the interior, leprosy was diagnosed in an armadillo hunter (unpublished data). It has been suggested that contact with armadillos or environmental shedding may be risk factors in acquiring leprosy (41, 42).
A mutation in the dapsone resistance determining region of folP1 was detected in sample Su-20 with two strains (P55S, 25% of the reads) and in Su-23 (P55R). The mutation P55S is rarely reported and to our knowledge, only one M. leprae strain in Korea was identified with this mutation (43). Based on the number of reads mapping both strains, we suspect that the mutation is carried by the strain 1D-2. Both patients were new cases and showed a BI 4+ at diagnosis. They were positive for M. leprae DNA after prolonged MDT and did not show a relapse, although the follow-up period was short in both cases, in one case due to the Covid pandemic, while the second patient died while still on extended treatment. These data suggest primary transmission of dapsone-resistant cases in a high percentage of the infected population (2/13 screened) (44). These data call for additional molecular surveillance in the population to avoid transmitting resistant strains.
Overall, our data unraveled an unprecedented diversity of M. leprae circulating in Suriname directly linked with the various migration waves into the country.
Data availability statement
The datasets generated for this study can be found in the NCBI Sequence Read Archive (SRA) under accession number PRJNA984678.
Ethics statement
The studies involving humans were approved by ethics committee of the Academic Hospital Paramaribo. The studies were conducted in accordance with the local legislation and institutional requirements. The participants provided their written informed consent to participate in this study.
Author contributions
WF: Conceptualization, Funding acquisition, Investigation, Methodology, Supervision, Writing – original draft. KS: Investigation, Methodology, Writing – review & editing. HM: Conceptualization, Formal Analysis, Investigation, Methodology, Validation, Writing – original draft. CA: Data curation, Formal Analysis, Funding acquisition, Investigation, Methodology, Software, Writing – review & editing. AG: Data curation, Funding acquisition, Investigation, Methodology, Software, Writing – review & editing. EV: Investigation, Methodology, Writing – review & editing. MTC: Investigation, Methodology, Writing – review & editing. MC: Investigation, Writing – review & editing. TP: Conceptualization, Funding acquisition, Investigation, Methodology, Project administration, Resources, Supervision, Writing – review & editing.
Funding
The author(s) declare financial support was received for the research, authorship, and/or publication of this article. This work is supported by the Fondation Raoul Follereau (grants to CA), the Heiser Program of the New York Community Trust for Research in Leprosy (grant no. P21-000127 to CA), the Association de Chimiothérapie Anti-Infectieuse of the Société Française de Microbiologie (CA), the European Union’s Horizon 2020 Research and Innovation Program (Marie Sklodowska-Curie grant no. 845479 to CA) and the QM Gastmann-Wichers Foundation.
Acknowledgments
We are grateful for the support of Dr. Richard Truman, for scientific comment as well as his visit to Suriname. We want to thank Elodie da Silva for her technical assistance in constructing the geomap. CA thanks Dr. Mary Jackson for her support and mentorship. We thank Dr. Han for providing DNA control of M. lepromatosis and Dr. Bartelt de Jongh for the biopsy Suriname logistics. We would also like to thank the Dermatology Service. The Dermatology Service is an institution under the authority of the Ministry of Health, for the diagnosis and treatment of skin diseases, STD and leprosy, including leprosy health education and case finding.
Conflict of interest
The authors declare that the research was conducted in the absence of any commercial or financial relationships that could be construed as a potential conflict of interest.
The author(s) CA declared that they were an editorial board member of Frontiers, at the time of submission. This had no impact on the peer review process and the final decision.
Publisher’s note
All claims expressed in this article are solely those of the authors and do not necessarily represent those of their affiliated organizations, or those of the publisher, the editors and the reviewers. Any product that may be evaluated in this article, or claim that may be made by its manufacturer, is not guaranteed or endorsed by the publisher.
References
1. Monot M, Honoré N, Garnier T, Zidane N, Sherafi D, Paniz-Mondolfi A, et al. Comparative genomic and phylogeographic analysis of Mycobacterium leprae. Nat Genet (2009) 41(12):1282–9. doi: 10.1038/ng.477
2. Snelders S. Leprosy and slavery in Suriname: godfried schilling and the framing of a racial pathology in the eighteenth century. Soc Hist Med (2013) 26:432–50. doi: 10.1093/shm/hkt040
3. Snelders S. Leprosy and colonialism. In: Suriname under Dutch rule, vol. 12. . Manchester (UK: Manchester University Press (2017). p. 1750–950.
4. Flu PC. Verslag van een studiereis naar Suriname (Nederlandsch Guyana) Sept. 1927 - Dec. 1927, en beschouwingen dienaangaande. Utrecht: Kemink en Zoon (1928). p. 65.
5. Menke H, Pieters T, Menke J. How colonial power, colonized people and nature shaped leprosy settlements in Suriname. Societies (2020) 10(2):32. doi: 10.3390/soc10020032
6. de Mesquita B. Annual report, leprosy control service Suriname. In: National archives, paramaribo (1955) surinam.
7. WHO Study Group on Chemotherapy of Leprosy for Control Programmes & World Health Organization. Chemotherapy of leprosy for control programmes : report of a WHO study group [meeting held in Geneva from 12 to 16 October 1981]. Geneva: World Health Organization (1982). Available at: https://apps.who.int/iris/handle/10665/38984.
8. WHO. Global leprosy (Hansen disease) update, 2020: impact of COVID-19 on global leprosy control Geneva. (2021) 36:421–44.
9. WHO. Global leprosy (Hansen disease) update, 2021: moving towards interruption of transmission. In: Weekly epidemiological record global leprosy situation by WHO region, country and territories, 8 September 2022. Available at: https://www.who.int/publications/i/item/who-wer9736-429-450.
10. Ploemacher T, Faber WR, Menke H, Rutten V, Pieters T. Reservoirs and transmission routes of leprosy; A systematic review. PloS Negl Trop Dis (2020) 14(4):e0008276. doi: 10.1371/journal.pntd.0008276
11. Han XY, Seo YH, Sizer KC, Schoberle T, May GS, Spencer JS, et al. A new Mycobacterium species causing diffuse lepromatous leprosy. Am J Clin Pathol (2008) 130(6):856–64. doi: 10.1309/AJCPP72FJZZRRVMM
12. Monot M, Honoré N, Garnier T, Araoz R, Coppée J-Y, Lacroix C, et al. On the origin of leprosy. Science (2005) 308(5724):1040–2. doi: 10.1126/science/1109759
13. Avanzi C, Singh P, Truman RW, Suffys PN. Molecular epidemiology of leprosy: An update. Infect Genet Evol (2020) 86:104581. doi: 10.1016/j.meegid.2020.104581
14. Thèves C, Crubézy E, Biagini P. History of smallpox and its spread in human populations. Microbiol Spectr. (2016) 4(4). doi: 10.1128/microbiolspec.PoH-0004-2014
15. Jones SD, Atshabar B, Schmid BV, Zuk M, Amramina A, Stenseth NC. Living with plague: Lessons from the Soviet Union's antiplague system. Proc Natl Acad Sci USA (2019) 116(19):9155–63. doi: 10.1073/pnas.1817339116
16. Truman RW, Singh P, Sharma R, Busso P, Rougemont J, Paniz-Mondolfi A, et al. Probable zoonotic leprosy in the southern United States. N Engl J Med (2011) 364(17):1626–33. doi: 10.1056/NEJMoa1010536
17. Vink WA. Creole Jews, negotiating community in colonial Suriname, Acad. Erasmus University Rotterdam: Thesis (2008) p. 50–1.
18. Hoogbergen W. ‘De Bosnegers zijn gekomen!’ Slavernij en Rebellie in Suriname. Amsterdam: Prometheus (1992).
19. Suriname Migration Profile: A study on emigration from, and immigration into Suriname (2015). Available at: https://publications.iom.int/fr/books/Suriname-migration-profile-study-emigration-and-immigration-Suriname.
20. Van Dissel JT, Pieters T, Geluk A, Maat G, Menke HE, Tió-Coma M, et al. Archival, paleopathological and aDNA-based techniques in leprosy research and the case of Father Petrus Donders at the Leprosarium ‘Batavia’, Suriname. Int J Paleopathol (2019) 27:1–8. doi: 10.1016/j.ijpp.2019.08.001
21. Tió-Coma M, Wijnands T, Pierneef L, Schilling AK, Alam K, Roy JC, et al. Detection of Mycobacterium leprae DNA in soil: multiple needles in the haystack. Sci Rep (2019) 9(1):3165. doi: 10.1038/s41598-019-39746-6
22. Ridley DS, Jopling WH. Classification of leprosy according to immunity. A five group system. Int J Lepr. (1966) 34(3):255–73.
23. Martinez AN, Lahiri R, Pittman TL, Scollard D, Truman R, Moraes MO, et al. Molecular determination of Mycobacterium leprae viability by use of real-time PCR. J Clin Microbiol (2009) 47(7):2124–30. doi: 10.1128/JCM.00512-09
24. Tió-Coma M, Avanzi C, Verhard EM, Pierneef L, van Hooij A, Benjak A, et al. Genomic characterization of mycobacterium leprae to explore transmission patterns identifies new subtype in Bangladesh. Front Microbiol (2020) 11:1220. doi: 10.3389/fmicb.2020.01220
25. Vera-Cabrera L, Escalante-Fuentes W, Ocampo-Garza SS, Ocampo-Candiani J, Molina-Torres CA, Avanzi C, et al. Mycobacterium lepromatosis infections in nuevo león, Mexico. J Clin Microbiol (2015) 53(6):1945–6. doi: 10.1128/JCM.03667-14
26. Benjak A, Avanzi C, Singh P, Loiseau C, Girma S, Busso P, et al. Phylogenomics and antimicrobial resistance of the leprosy bacillus Mycobacterium leprae. Nat Commun (2018) 9(1):352. doi: 10.1038/s41467-017-02576-z
27. Bolger AM, Lohse M, Usadel B. Trimmomatic: a flexible trimmer for Illumina sequence data. Bioinformatics (2014) 30(15):2114–20. doi: 10.1093/bioinformatics/btu170
28. Koboldt DC, Zhang Q, Larson DE, Shen D, McLellan MD, Lin L, et al. VarScan 2: somatic mutation and copy number alteration discovery in cancer by exome sequencing. Genome Res (2012) 22(3):568–76. doi: 10.1101/gr.129684.111
29. Cingolani P, Platts A, Wang le L, Coon M, Nguyen T, Wang L, et al. A program for annotating and predicting the effects of single nucleotide polymorphisms, SnpEff: SNPs in the genome of Drosophila melanogaster strain w1118; iso-2; iso-3. Fly (Austin). (2012) 6(2):80–92. doi: 10.4161/fly.19695
30. Rimmer A, Phan H, Mathieson I, Iqbal Z, Twigg SRF, WGS500 Consortium, et al. Integrating mapping-, assembly- and haplotype-based approaches for calling variants in clinical sequencing applications. Nat Genet (2014) 46(8):912–8. doi: 10.1038/ng.3036
31. Robinson JT, Thorvaldsdóttir H, Wenger AM, Zehir A, Mesirov JP. Variant review with the integrative genomics viewer. Cancer Res (2017) 77(21):e31–4. doi: 10.1158/0008-5472.CAN-17-0337
32. Koichiro T, Glen S, Sudhir K. MEGA11: molecular evolutionary genetics analysis version 11. Mol Biol Evol (2021) 38:3022–7:7). doi: 10.1093/molbev/msab120
33. Furtwängler A, Neukamm J, Böhme L, Reiter E, Vollstedt M, Arora N, et al. Comparison of target enrichment strategies for ancient pathogen DNA. Biotechniques (2020) 69(6):455–9. doi: 10.2144/btn-2020-0100
34. Avanzi C, Lécorché E, Rakotomalala FA, Benjak A, Rapelanoro Rabenja F, Ramarozatovo LS, et al. Population genomics of mycobacterium leprae reveals a new genotype in Madagascar and the Comoros. Front Microbiol (2020) 11:711(11). doi: 10.3389/fmicb.2020.00711
35. Singh P, Benjak A, Carat S, Kai M, Busso P, Avanzi C, et al. Genome-wide re-sequencing of multidrug-resistant Mycobacterium leprae Airaku-3. Clin Microbiol Infect (2014) 20(10):O619–22. doi: 10.1111/1469-0691.12609
36. Hockings KJ, Mubemba B, Avanzi C, Pleh K, Düx A, Bersacola E, et al. Leprosy in wild chimpanzees. Nature (2021) 598(7882):652–6. doi: 10.1038/s41586-021-03968-4
37. Menke H, Snelders S, Pieters T. Omgang met lepra in 'de West' in de negentiende eeuw. Tegendraadse maar betekenisvolle geluiden vanuit Suriname [Coping with leprosy in the Dutch West Indies in the 19th century; opposing but meaningful views from Suriname]. Studium (2009) 2(2):65–77.
38. Menke H, Snelders S, Pieters T. Leprosy control and contagionism in Suriname. Acad J Suriname (2011) 2:168–75.
39. McDonough CM, DeLaney MJ, Le Q, Blackmore P, Mark S, & Loughry WJ. Burrow characteristics and habitat associations of armadillos in Brazil and the United States of America. Rev Biología Trop (2000) 48(1):109–20.
40. Schaub R, Avanzi C, Singh P, Paniz-Mondolfi A, Cardona Castro N, Legua P. Leprosy transmission in amazonian countries: current status and future trends. Curr Trop Med Rep (2020) 7:79–91. doi: 10.1007/s40475-020-00206-1
41. da Silva MB, Portela JM, Li W, Jackson M, Gonzalez-Juarrero M, Hidalgo AS, et al. Evidence ofzoonotic leprosy in Pará, Brazilian Amazon, and risks associated with human contact or consumption of armadillos. PloS Negl Trop Dis (2018) 12(6):e0006532. doi: 10.1371/journal.pntd.0006532
42. Pieters T, Meredith AL, Braig HR. More on autochthonous leprosy in the United States. N Engl J Med (2023) 389(10):963. doi: 10.1056/NEJMc2308758
43. You EY, Kang TJ, Kim SK, Lee SB, Chae GT. Mutations in genes related to drug resistance in Mycobacterium leprae isolates from leprosy patients in Korea. J Infection (2005) 50(1):6–11. doi: 10.1016/j.jinf.2004.03.012
44. Cambau E, Saunderson P, Matsuoka M, Cole ST, Kai M, Suffys P, et al. WHO surveillance network of antimicrobial resistance in leprosy. Antimicrobial resistance in leprosy: results of the first prospective open survey conducted by a WHO surveillance network for the period 2009-15. Clin Microbiol Infect (2018) 24(12):1305–10. doi: 10.1016/j.cmi.2018.02.022
Keywords: leprosy, genotypes, distribution, Suriname, dapsone resistance, M. leprae, historical migration
Citation: Faber WR, Sewpersad K, Menke H, Avanzi C, Geluk A, Verhard EM, Tió Coma M, Chan M and Pieters T (2023) Origin and spread of leprosy in Suriname. A historical and biomedical study. Front. Trop. Dis 4:1258006. doi: 10.3389/fitd.2023.1258006
Received: 13 July 2023; Accepted: 05 October 2023;
Published: 07 November 2023.
Edited by:
Rosane M.B. Teles, University of California, Los Angeles, United StatesReviewed by:
Rupendra Jadhav, The Institute of Science, Mumbai, IndiaMaria Pena, Health Resources and Services Administration, United States
Flavio Alves Lara, Oswaldo Cruz Foundation (Fiocruz), Brazil
Copyright © 2023 Faber, Sewpersad, Menke, Avanzi, Geluk, Verhard, Tió Coma, Chan and Pieters. This is an open-access article distributed under the terms of the Creative Commons Attribution License (CC BY). The use, distribution or reproduction in other forums is permitted, provided the original author(s) and the copyright owner(s) are credited and that the original publication in this journal is cited, in accordance with accepted academic practice. No use, distribution or reproduction is permitted which does not comply with these terms.
*Correspondence: Toine Pieters, t.pieters@uu.nl; William R. Faber, w.r.faber@amsterdamumc.nl