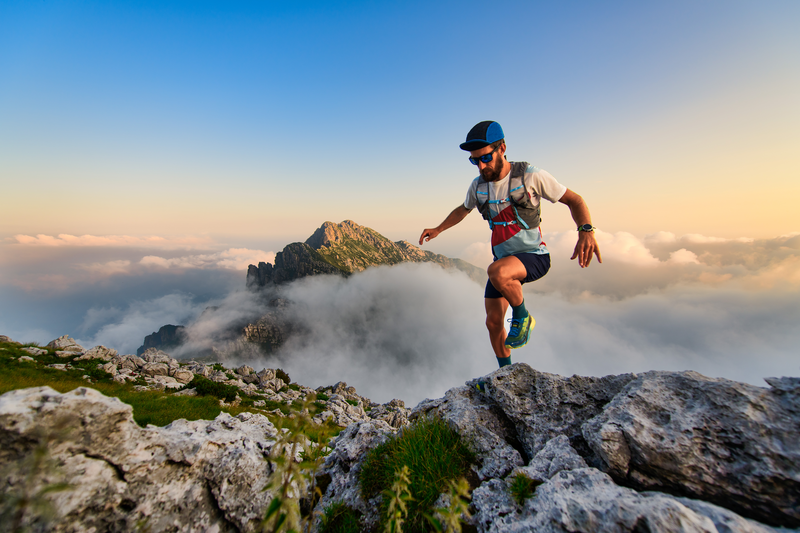
94% of researchers rate our articles as excellent or good
Learn more about the work of our research integrity team to safeguard the quality of each article we publish.
Find out more
REVIEW article
Front. Trop. Dis. , 31 January 2023
Sec. Neglected Tropical Diseases
Volume 4 - 2023 | https://doi.org/10.3389/fitd.2023.1108317
This article is part of the Research Topic Novel Therapeutics for Parasitic NTDs View all 5 articles
Background: Metabolomic based approaches are essential tools in the discovery of unique biomarkers for infectious diseases via high-throughput global assessment of metabolites and metabolite pathway dysregulation. This in-turn allows the development of diagnostic tools and provision of therapeutics. In this review, we aimed to give an overview of metabolite biomarkers and metabolic pathway alterations during Schistosoma haematobium and Schistosoma mansoni infections.
Methods: We conducted the review by systematically searching electronic databases and grey literature to identify relevant metabolomics studies on schistosomiasis. Arksey and O’Malley methodology for conducting systematic scoping reviews was applied. A narrative summary of results was conducted following the Preferred Reporting Items for Systematic reviews and Meta-Analyses extension for scoping review guidelines.
Results: Twelve articles included in the review identified 127 metabolites, whose concentrations were considerably altered during S. mansoni and S. haematobium infections. The metabolites were assigned to metabolic pathways involved in energy (34.6%), gut microbial (11.0%), amino acid (25.2%), nucleic acids (6.3%), immune proteins (8.7%) hormones (2.4%) and structural proteins/lipids (11.8%). Energy related metabolic pathways were the most affected during schistosome infections with metabolites such as succinate, citrate, aconitate and fumarate of the tricarbocylic acid cycle being significantly altered in organ, serum and plasma samples. Amino acid metabolism was also impacted during schistosome infections as phenylacetylglycine, alanine, taurine, 2-oxoisocaproate and 2-oxoisovalerate emerged as potent biomarkers. Elevated structural proteins such as actin, collagen and keratin concentrations were identified as biomarkers of liver fibrosis, a common pathological feature in chronic schistosomiasis infections. Hippurate was a major metabolite biomarker in the gut microbial related pathway.
Conclusions: The analysis of the literature revealed that energy related metabolic pathways are considerably altered during S. mansoni and S. haematobium infections. Therefore, their metabolites may provide biomarkers for diagnosis and prognosis in addition to providing therapeutics for parasitic infections. This scoping review has identified a need to replicate more schistosomiasis metabolomic studies in humans to complement animal-model based studies.
Schistosomiasis is one of the common neglected tropical diseases (NTDs) caused by sexually dimorphic helminth worms of the genus Schistosoma (1). Global estimates show a prevalence of at least 236.6 million cases in 78 countries (2). Moreover, 200 000 deaths are recorded annually, especially in Africa which accounts for 90% of global morbidity and mortality (3, 4). Of the six known human schistosome species, Schistosoma haematobium (S. haematobium) and Schistosoma mansoni (S. mansoni) are the main etiological agents of human urogenital and intestinal schistosomiasis respectively and are highly prevalent in the sub-Saharan region (5).
Early diagnosis of schistosomiasis is key for effective treatment and management of adverse symptoms which directly determine the clinical outcome of the disease, whilst development of vaccine against helminth infections is critical in the eradication of the disease (6, 7). Low sensitivity diagnostic tools in detection of early or light schistosome infections across all age groups particularly in pre-school aged children (PSAC) ≤5 years have implicated worldwide concerted efforts in schistosome surveillance and control programmes (8, 9). Furthermore, there is no approved effective vaccine for schistosomiasis (10).
In addition to providing insight into complex immunopathology during schistosomiasis (11), metabolite biomarkers of infection are critical to the rational development of therapeutic interventions (12), non-invasive diagnostic and prognostic tools (13, 14) thus it is of paramount significance to characterise schistosome induced metabolic alterations. In recent years, metabolomics has emerged as a powerful omics approach in characterisation of host metabolic responses to infections at a molecular level leading to a wide range of applications in discovery of drug targets and novel biomarkers in infectious diseases (15, 16).
Preventive chemotherapy alone is insufficient in the global control and elimination of schistosomiasis. Thus integrated approaches should include; inclusion of all age groups in control strategies, effective diagnosis, knowledge on efficacy of treatment, provision of good sanitary conditions (17). Moreover, a clear understanding of the infection pathology dynamics or health impacts in all age groups have to be established (18, 19). Diagnosis and knowledge in pathological mechanisms are the main factors that determine the success of schistosomiasis management strategies. This has led to concerted efforts in the search of new biomarkers by analysing metabolic pathway dysregulation using a metabolomics based approach (20, 21). The available standard methods specifically urine filtration technique for S. haematobium and Kato Katz technique for S. mansoni lacks sensitivity and absolute specificity. These diagnosis shortfalls are even more in areas where the disease burden is low and in patients with light schistosome infection (20, 22, 23).
The maximum impact of global control and elimination programs for schistosomiasis may be realised through addressing above challenges. Metabolomics is a more holistic approach as far as schistosomiasis diagnosis, prognosis, provision of therapeutic drug or vaccine targets. A metabolomics approach would also allow for the elucidation of infection mechanisms via analysis of metabolic pathway alterations and characterising metabolite signatures for biomarkers (24). Against this background, we highlight the metabolic alterations for biomarker discovery during urogenital and intestinal schistosomiasis.
In this scoping review we sought to summarize metabolic biomarkers associated with S. haematobium and S. mansoni infections to facilitate understanding of disease mechanisms and the discovery of unique diagnostics and therapeutic measures. The following review questions were answered:
1. Which metabolites can be utilized as biomarkers of S. haematobium and S. mansoni infections?
2. Which metabolic pathway alterations occur during S. haematobium and S. mansoni infections?
Our methodology for the study was informed by the Arksey and O’Malley (25) guidance for conducting systematic scoping reviews. A comprehensive systematic literature search relevant to the topic and review objectives was conducted following the Preferred Reporting Items for Systematic Reviews and Meta-Analyses extension for Scoping Reviews (PRISMA-ScR) guidelines (Table S1) (26). The protocol for the scoping review was registered in Open Science Framework (osf.io/py3x9).
Four electronic bibliographic databases (Pubmed, Cochrane Library, Medline and Embase) were searched using the following search terms, modified appropriately to each or for each database: (1) (metabolome OR metabolomics OR metabolomic OR metabonomics OR metabonomic OR metabolite OR metabolic OR metabolism OR lipidome OR lipidomics OR lipidomic OR proteome OR proteomics OR proteomic) and (2) (schistosomiasis OR bilharziasis OR bilharzia OR katayama fever OR snail fever OR urogenital schistosomiasis OR urinary schistosomiasis OR genital schistosomiasis OR Schistosoma OR haematobium OR Schistosoma haematobium OR S. haematobium OR Schistosoma mansoni OR S. mansoni OR mansoni OR intestinal schistosomiasis). Databases were searched from inception without any language restrictions. In addition to the primary databases we complemented the search strategy by searching of grey literature and unpublished preprints in MedRxiv and BioRxiv. The search strategies and results for all databases are illustrated in File S1.
Animal and human case cohort, case-control and prospective cohort studies of all age groups with S. haematobium or S. mansoni infections were included in the review. The studies were eligible if metabolomics methods, mainly but not limited to; nuclear magnetic resonance (NMR), gas chromatography (GS), liquid chromatography (LC), mass spectrometry (MS) or combinations of these approaches were utilised to identify biomarkers or changes of metabolites between schistosomiasis patients and controls or uninfected individuals. Articles with outcomes which clearly highlight significant alterations to specific metabolic pathways due to infection and where candidate biomarkers were defined by the researcher as any substance or its product/s that may be quantified in a predefined biological sample were also considered. Studies relating to other forms of schistosome infections (such as S. japonicum, S. intercalatum and S. mekongi), articles irrelevant to metabolomics of schistosomiasis, abstracts without full texts, text books, letters and review articles were excluded.
Zotero (version 5.0.96.3) program was used for bibliography management. Two independent authors screened the titles and abstracts retrieved by the search in the databases and excluded articles that were clearly opposed to our inclusion criteria whilst full articles were retrieved for the studies that were best aligned to the review questions. A comprehensive full text screening was conducted for eligibility of the studies and any disagreements in the final selection process were reconciled through discussion between authors and where agreement could not be reached, third reviewer was involved in the process to avoid the risk of bias in acceptance or rejection of the studies.
Authors subsequently extracted the following information from the eligible studies included (see Table S2) for the current review:
1. study profile (authors, DOI, year of publication, country),
2. study design and aims
3. targeted population
4. type of metabolomics analytical platform
5. sample size, including number of cases and controls
6. specimen type (serum, plasma, urine, organ and faecal)
7. metabolites or pathway identified (including concentration increase or decrease)
8. statistical approach for data analysis
Reasons for study exclusion was also extracted for all excluded articles (see Table S3).
For the current review, there is no critical appraisal of methodological quality or risk of bias of the included study articles in relation to guidance on scoping review conduct (27). However, quantitative descriptive analysis was carried out simultaneously with a qualitative thematic analysis whilst a comprehensive narrative summary was employed to interpret the tabulated findings within the context of the objective and questions of this review (25, 28).
The scoping review aggregated human and animal metabolomics studies with an emphasis on metabolite and metabolic pathway perturbations that have potential biomarker value in urogenital and intestinal schistosomiasis caused by S. haematobium and S. mansoni respectively. A total of 3118 articles were found from seven electronic databases. Following the removal of duplicates, 1208 articles remained for screening. Two researchers independently screened the studies using the title or abstract and 93 articles were sort for full text retrieval. Post assessment, a total of 12 articles were included in this review conforming to outlined eligibility, whilst 81 articles were excluded predominantly due to a lack of high throughput metabolomics techniques in their methodology (n=66). Figure 1 illustrates the PRISMA flow diagram for the study’s literature search and identification method.
The general characteristics of the articles included in this review are shown in Table 1. Based on literature survey, the majority of the metabolomics studies focused on S. mansoni species (n=10) whilst 2 studies concentrated on S. haematobium species. In terms of models, 7 studies used animals (mice), 4 studies used humans’ specimens and 1 study included both human and animal models. Biological samples included plasma in 3 studies, serum in 2 studies, urine in 11 studies, tissue or organ in 3 studies and faecal in 1 study. One study (33) utilized urine, plasma and faecal, three studies (31, 32, 35) utilized organs (either liver, jejunum, ileum, colon, spleen and kidney) and one study (21) utilized urine and plasma. The analytical platforms applied to detect metabolic perturbations, included nuclear magnetic resonance (NMR) based in 4 studies, ultra-high performance liquid chromatography (UHPLC) in 3 studies, liquid chromatography mass spectrometry (LC-MS) in 1 study, capillary electrophoresis time-of-flight mass spectrometry (CE-TOF-MS) in 1 study, matrix-assisted laser desorption ionization time-of-flight mass spectrometry (MALDI-TOF MS) in 2 studies, gas chromatography mass spectrometry (GC–MS) in 1 study, two-dimensional difference gel electrophoresis (2D DIGE) in 2 studies and capillary electrophoresis (CE) in 1 study. These platforms were either utilised in targeted metabolomics approach which is the comprehensive analysis of all metabolites or untargeted metabolomics approach which is a subset analysis of specifically defined metabolites. The approach for these analytical platforms in biomarker identification included 9 studies in untargeted and 3 studies in targeted metabolomics. The most frequently reported metabolic pathway was energy related in 9 studies followed by amino acids in 7 studies, gut microbial related in 6 studies, structural proteins or lipids in 5 studies, immune proteins in 2 studies and 1 study for hormone and nucleic acid metabolism respectively.
For a global metabolomics profile, the altered metabolic pathways were divided into seven different categories namely energy, gut microbial, amino acids, nucleic acids, immune proteins, structural proteins/lipids and hormone related metabolites. From the studies included in this scoping review, a total of 127 altered metabolites were extracted in response to S. haematobium and S. mansoni infections in which 90 were unique non repeated metabolites. Furthermore, 44 repeats (frequency in which the metabolite is determined to be a biomarker in a study) of 17 different metabolites were identified in these seven metabolic pathway categories. With respect to energy metabolism, a total of 44 energy related metabolites with 12 repeats of 7 different metabolites were identified while 14 gut microbial related metabolites containing 11 repeats of 3 different metabolites and 8 different nucleic acid related metabolites were identified. With respect to amino acid metabolism, 32 metabolites were found to be altered with 17 repeats of 7 different metabolites. Finally, a total of 11 metabolites, 15 metabolites and 3 metabolites were identified for immune proteins, structural lipids or protein and hormone metabolism respectively. Comparison of all extracted metabolites revealed that the energy metabolism was significantly affected as their metabolites deviate from normal concentrations in schistosomiasis infected individuals as compared to healthy controls, followed by amino acid, structural lipids or protein, gut microbial, immune proteins, nucleic acid and hormones metabolism. Adult schistosome worms utilise glucose ranging from one-sixth to one-fifth of its dry weight leading to energy related metabolites, enzyme and intermediate deviations from their homeostatic level which may explain the reason for a high number in energy related metabolism related biomarkers in schistosomiasis (40). Table 2 provides an overview of key altered metabolites from the studies pathway enrichment analysis on the extracted metabolites and manual assignment of the metabolites to pathway.
Table 2 Summary of changes in major affected metabolic pathways during urogenital and intestinal schistosomiasis.
Energy metabolic pathways are critical to all living organisms through production of ATP to effectuate the bioenergetic need of cellular functions and processes contributing to net biomass formation and homeostasis (41). In the current review, schistosome infection was reported to upregulate glycolysis which was indicated by an increase in intermediates (glucose-6-phosphate and 3-phosphoglyceric acid) and their end product pyruvate whilst the main substrate glucose and its stores declined (29, 31, 32, 37). Furthermore, glucose-6-phoshate and 3-phosphoglyceric acid were found to be major metabolite biomarkers in discriminating human S. haematobium infection in paediatrics below the age of five (37). A study conducted by Tielens and colleagues highlighted that during the helminth complex life cycle in mammalian hosts, paired adult schistosomes utilise glucose and glycogen for their maturation and to compensate for their substantial needs for the energy required to produce the large numbers of eggs laid continuously by the female worm (42). Consequently, enhanced activation of glycolysis was reported to directly stimulate the tricarboxylic acid cycle as exhibited by high levels of pathway intermediates such as alpha ketoglutarate (39). However, tricarboxylic acid intermediates, succinate and fumerate were considered poor diagnostic markers as they may be inconclusively lower or higher in concentration during schistosome infection (34, 39). Aconitate levels were reported to be significantly decreased during S. mansoni infection, thus may be a potent oxidative biomarker (34, 35). The findings of Yarian and colleagues suggest that perturbations of aconitase is associated with aging and disturbances in the tricarboxylic acid cycle activities leading to reduction in overall capability of mitochondrial bioenergetics (43).
Helminth infections contribute to the structure and composition of the gut microbiome which play a major role in the development of the immune system (44). The studies in this review showed that hippurate is a major candidate microbiome biomarker during intestinal and urogenital schistosomiasis and the reduction in the metabolite concentration in biofluids is associated with infection (29, 30, 33, 34). Furthermore, one study reported that hippurate concentration is elevated during light schistosome infection while the concentration is decreased in chronic schistosome infection hence the metabolite may be used as a marker to determine the intensity of infection (39). Elevated levels of triethylamine and p-cresol glucuronide were significantly associated with schistosome infection despite other microbiome metabolites such as trimethylamine N-oxide and aminovalerate detection being elevated in urine and plasma (29, 33, 34). The presence of microbial based metabolites such as hippurate, p-cresol glucuronide and trimethylamine derivatives in biofluids suggest a cross-talk between helminth worms in mammalian host and its gut microbiota therefore such unique biomarkers may be utilised in the development of new diagnostic tools for schistosomiasis (33). However, propionate had indeterminate pertubations as the metabolite may either be decreased or increased during schistosome infections, hindering its predictor value as a gut microbiota biomarker (29, 33).
Urogenital schistosomiasis alters nucleic acid metabolism by increasing the synthesis of purines mainly inosine, AMP and ADP (37). Two studies supported the findings as purine derived metabolites namely guanidino acetate, methylguanidine cyclic adenosine monophosphate response element binding protein were elevated during schistosomiasis (21, 34). A possible explanation for the upregulation of purine metabolism is an increase in demand for energy in the form of ATP by schistosome worms, which consequently increase ADP and AMP as products of hydrolysis (21). Additionally, the concentrations of the high energy phosphates, AMP and ADP in the blood are key regulators of energy homeostasis as they determine the initiation of alternative metabolic pathways such as fatty acid degradation and glycogenolysis to restore energy balance (37).
Amino acid functions are not only limited as structural building blocks of proteins or nutritional elements but are also involved in a myriad of biological processes which include acting as regulatory signals for gene expression, cell signalling pathways and being precursor molecules for hormone synthesis (21). Schistosome infection resulted in elevated levels of phenylacetylglycine and alanine (29, 33, 34, 39). Phenylacetylglycine is an alpha amino acid which bears an acyl group at its terminal nitrogen atom that signals a lysosomal disorder called phospholipidosis where there is an over accumulation of phospholipids in either the liver, kidney or lungs (45). The disorder can be linked to hepatosplenomegaly due to entrapment of schistosome eggs in the liver and spleen (46). An increase in glucogenic amino acids (alanine, glutamine and asparagine) was observed in both S. mansoni and S. haematobium infections (29, 34, 39). Elevated levels in glucogenic amino acids may be attributed to upregulation of gluconeogenesis due to depletion of energy substrates and elevated liver aminotransferases (47, 48). Free proline, which is a precursor of hydroxyproline, glycine, alanine and their derivatives, were elevated in S. mansoni infections in mouse models which might imply stimulated collagen synthesis activity (49).
A disturbance of amino acid metabolic pathways was also associated with an S. mansoni infection, as indicated by the depletion of taurine, 2-oxoisocaproate and 2-oxoisovalerate. Taurine is an amino sulfonic acid that has been reported to alleviate liver fibrosis by suppressing the activation of downstream inflammatory mediators and subsequent pyroptosis (50). A decrease in 2-oxoisocaproate and 2-oxoisovalerate that originates from valine and leucine respectively was reported to be associated with increase in amino acid metabolism to synthesise precursor molecules (29). Notably, one study indicated a decrease in urea which may be due to expression of arginase induced by schistosome worms during their development in the human host, which is responsible for the hydrolysis of urea (51).
S. mansoni phosphoenolpyruvate carboxykinase (SmPEPCK) is an egg antigen for schistosome infection and studies suggested its potential as a disease specific protein marker due to its immunological properties (52). SmPEPCK was detected and elevated in the liver during intestinal schistosomiasis (32, 35), therefore this protein-based antigen may be utilised as an immunological marker of infection adding to serum cytokines, chemokines and receptors (53). Lymphocyte cytosolic protein 1 (LCP1) and prohibitin 2 were significantly increased in schistosome chronic infections (32). LCP1 has been reported to be utilised as a marker in cancers such as leukaemia whilst the latter controls the growth, development and apoptosis of cells thus it can be concluded that they are not useful markers for schistosomiasis (54, 55). Major histocompatibility complex (MHC) precursors and interleukin-2 (IL-2) were found to be increased during the infection. The MHC plays an important role in adaptive immunity through presentation of both endogenous and exogenous antigens during helminths infections (56). A surge in cytokine IL-2 have been reported in both S. haematobium and S. mansoni infections to promote the growth and development of peripheral immune cells in the initiation of the immune response (57). One study reported elevated levels of venom allergen-like (VAL) 3 proteins during schistosomiasis which is a common feature of helminths species cocktail of proteins to aid invasion, initiate feeding, facilitate adaptation and mediate modulation of the host immune responses (58).
Lipids play a major role in the formation or maintenance of the integrity of the cellular membrane bilayer and act as receptors for a variety of cell signalling processes (59). Membrane associated lipids namely glycerophosphoryl choline, phosphoryl choline and phosphatidylethanolamine are elevated in the blood during schistosomiasis infections (21, 38). However, two studies were contradicting with respect to phosphatidylcholine; one study (21) indicated that the levels were elevated whilst the other showed that the levels were decreased in during schistosomiasis (33). Disturbance in phosphocholine concentration in tissues is associated with cell proliferation which additionally explains elevated heat shock proteins (HSP) as oncogene signalling molecules (60). Due to oxidative stress in a tumour microenvironment, phosphatidylethanolamine is usually expressed on endothelial cells and has been reported that alterations in both phosphatidylethanolamine and phosphatidylcholine is one of the mechanisms for carcinogenesis in chronic infections (61, 62). Secondary structure proteins; actins, collagen, cytokeratins, hydroxyproline and connective tissue growth factor were elevated during schistosomiasis infections with a possibility in playing a role in liver fibrosis in chronic schistosome infections (32, 36).
Low levels of human estrogen-related hormones were associated with urogenital schistosomiasis infection (21). There is existing evidence for the role of estrogen metabolism in host-parasite relationship where S. haematobium adult worm utilise or inhibit production of host steroids resulting in decreased sex steroid hormones (63, 64). On the other hand, adrenochrome-O-quinone, a toxic quinone metabolite of epinephrine, was elevated in plasma during schistosomiasis infection. Adrenocromes with quinone groups are capable of forming reactive oxygen species with severe pathological effects such as bladder cancer in chronic schistosomiasis (65).
One of our research goals was to be able to delineate schistosomiasis metabolomic profiles with more emphasis in human specimens. However, five of the identified articles expressly reported metabolic alterations in human biofluids whilst the rest utilised mouse as an animal model although such models are essential in determination of organ or tissue damage due to schisosomiasis. Another limitation was scarcity of relevant literature, arising from inclusion criteria we employed leading to exclusion of other schistosome species such as S. japonicum, S. mekongi, S. bovis and S. intercalatum despite that these species are geographically not common in Africa and their global prevalence is very low (66). Metabolomics studies require robust and expensive equipment such as NMR and HPLC which increases cost of research, this may further contribute to the reason why there is limited literature on metabolomics (67). Furthermore, the current review could not categorise metabolite alterations based on age groups as most of the studies were skewed towards adults and utilised animal models.
Metabolomics can contribute immensely in the discovery of biomarkers for diagnostic purposes and in elucidating pathophysiological changes due to schistosome infections. As the race to develop diagnostic and prognosis tools that are highly sensitive and specific continues, metabolomics studies for schistosomiasis are expected to be increasingly carried out (11). The aspect will contribute to early detection of schistosome infections before the manifestation of severe pathological symptoms and reduces treatment costs by directing MDA programs to populations with high disease prevalence (68).
For this new field to be positioned in the mainstream of biomarker discovery in infectious diseases specifically schistosomiasis, several issues need to be addressed including validating and reproducing candidate metabolites, methodologies in study design and advanced data analysis. For example, although non-targeted approaches that enable a comprehensive or global analysis to detect novel compounds and generate new hypotheses, the high cost of advanced equipment such as NMR and complex statistical analysis including bioinformatics are required. On the contrary, targeted metabolomics approaches are hypothesis driven, less expensive and require minimum statistical analysis but lack depth in the discovery of unknown metabolites (67, 69). Chen and colleagues (70) revealed that integrating both approaches in study designs maintained some strengths of targeted and untargeted metabolomics analysis, while neutralizing some of their weaknesses thus such holistic approaches should be considered for future studies. Furthermore, such integrative approaches should be extended to study population where all age groups namely pre-school aged children, school aged children and adults are considered in the inclusion criteria for schistosomiasis metabolomics studies as most researches are biased towards individuals above five years (71).
Despite energy metabolite being significantly altered during S. haemtobium and S. mansoni infections, gut microbial related compounds hippurate and trimethylamine together with phenylacetylglycine and alanine amino acids were the most promising metabolites for biomarker use. Of much interest was hippurate, which its concentration deviation from normal in metabolomics analysis can be used as an indicator for severity of schistosome infection in addition to diagnostic purposes (39). However, further analysis such as reproducibility, sensitivity and specificity assays should be done to ascertain the diagnostic accuracy for these biomarkers during schistosomiasis. Box 1 provides a summary of promising metabolite for biomarker use and some of the outstanding questions for the benefit of future metabolomics studies focusing on urogenital and intestinal schistosomiasis.
Metabolomics is receiving a great deal of attention amongst the omics approaches as it has displayed an immense potential in unearthing novel diagnostic biomarkers and previously unidentified vaccine targets in parasitic infections. This scoping review outlined the current understanding on dysregulation of the metabolic pathway and metabolites can be used as biomarkers in urogenital and intestinal schistosomiasis. A total of 127 metabolites were identified to be altered and energy metabolism was significantly affected, followed by amino acid, gut microbial, structural proteins/lipids, nucleic acid and hormones metabolism. In addition to providing biomarkers for diagnosis and prognosis, the findings of the review provide insight into the complex pathological symptoms and host-parasite relationship during infection. Energy related and structural lipids or proteins metabolite profiles were mainly altered during urogenital schistosomiasis. On the other hand, intestinal schistosomiais largely affected gut-microbial and amino acid metabolic profiles. Although animal models are essential in disease research, the models are often poor predictors of human reactions to infection exposure owing to poor study designs and low replicability of experiments (72). Most of metabolomics studies identified in this scoping review were animal based studies, therefore there is a need to increase efforts in human studies covering all age groups to complement high numbers in animals related studies thereby enhancing the quality and accuracy of the study and result respectively. Study characteristics revealed that the study population size for metabolomics studies ranges from a 50 to 335 samples with controls for human studies therefore it is evident that it is recommendable to utilise a large sample size to obtain statistically significant results. In contrast, a minimum of 5 mice per control group are deemed sufficient to conduct animal-based metabolomics studies for schistosomiasis. Therefore, we recommend the inclusion of animal models in all human base studies to obtain comparative results. In relation to specimen, most of schistosomiasis metabolomics studies searched for biomarkers in urine due to its simple collection and non-invasiveness which additionally facilitates continuous monitoring of biomarkers as opposed to blood samples which triggers immense pain and psychological trauma especially in paediatrics (73). Although all analytical platforms were utilised for both untargeted and targeted metabolomics, NMR based and UHPLC methodologies were widely utilised. NMR is relatively less sensitive and very expensive when compared to UHPLC, however its high reproducibility, non-selective nature and the ability to identify unknown metabolites in complex mixtures offer tremendous benefits to the metabolomics field (74).
Although further research is needed in metabolomics, this overview provides an insight into metabolic perturbations during schistosomiasis infections which is essential in the identification of useful biomarkers and explaining pathologies associated with the disease.
HM, TM, FM and AV conceived the idea. HM, AV and MK did the literature research, compiled the data and analysed the data. HM and AV wrote the first draft of the manuscript. TM-J, CS, IC and VM gave the suggestions for improvement. TM, FM and TN provided guidance, revised and completed the manuscript. All authors contributed to the article and approved the submitted version.
The proposed study ideation emanated from a study supported by the Royal Society Grants ICA_R1_201399.
Author IC is employed by Aravas Pharmaceuticals Pvt LTD.
The remaining authors declare that the research was conducted in the absence of any commercial or financial relationships that could be construed as a potential conflict of interest.
Box 1 Highlights and research gaps for urogenital and intestinal schistosomiasis biomarker discovery using metabolomics techniques
Most promising metabolites
● Energy: creatine, pyruvate, citrate and aconitate
● Gut microbial: hippurate, trimethylamine and p-cresol glucuronide
● Amino acids: phenylacetylglycine, alanine, 2-oxoisocaproate benzoic acid and asparagine
● Immune proteins: S. mansoni phosphoenolpyruvate carboxykinase (SmPEPCK)
Outstanding research questions
● What are the differences in metabolite profiles across all age groups during schistosomiasis?
● Can current metabolite biomarkers be assessed and validated for diagnostic accuracy in the detection of schistosome infections?
All claims expressed in this article are solely those of the authors and do not necessarily represent those of their affiliated organizations, or those of the publisher, the editors and the reviewers. Any product that may be evaluated in this article, or claim that may be made by its manufacturer, is not guaranteed or endorsed by the publisher.
The Supplementary Material for this article can be found online at: https://www.frontiersin.org/articles/10.3389/fitd.2023.1108317/full#supplementary-material
1. Utzinger J, Raso G, Brooker S, De Savigny D, Tanner M, Ørnbjerg N, et al. Schistosomiasis and neglected tropical diseases: towards integrated and sustainable control and a word of caution. Parasitology (2009) 136:1859–74. doi: 10.1017/S0031182009991600
2. Sacolo H, Chimbari M, Kalinda C. Knowledge, attitudes and practices on schistosomiasis in sub-Saharan Africa: a systematic review. BMC Infect Dis (2018) 18:46. doi: 10.1186/s12879-017-2923-6
3. Schistosomiasis . Available at: https://www.who.int/news-room/fact-sheets/detail/schistosomiasis?:cf_chl_captcha_tk:=pmd_3xnaeLqR14QZD1dclFF2cjTAqI6jQ0fNgzs5GkPJt3A-1634633141-0-gqNtZGzNAxCjcnBszQkR (Accessed October 19, 2021).
4. Aula OP, McManus DP, Jones MK, Gordon CA. Schistosomiasis with a focus on Africa. Trop Med Infect Dis (2021) 6:109. doi: 10.3390/tropicalmed6030109
5. Adenowo AF, Oyinloye BE, Ogunyinka BI, Kappo AP. Impact of human schistosomiasis in sub-Saharan Africa. Braz J Infect Dis (2015) 19:196–205. doi: 10.1016/j.bjid.2014.11.004
6. Utzinger J, Becker SL, van Lieshout L, van Dam GJ, Knopp S. New diagnostic tools in schistosomiasis. Clin Microbiol Infect (2015) 21:529–42. doi: 10.1016/j.cmi.2015.03.014
7. Hotez PJ, Bethony JM, Diemert DJ, Pearson M, Loukas A. Developing vaccines to combat hookworm infection and intestinal schistosomiasis. Nat Rev Microbiol (2010) 8:814–26. doi: 10.1038/nrmicro2438
8. Ajibola O, Gulumbe BH, Eze AA, Obishakin E. Tools for detection of schistosomiasis in resource limited settings. Med Sci (2018) 6(2):39–54. doi: 10.3390/medsci6020039
9. Osakunor DNM, Woolhouse MEJ, Mutapi F. Paediatric schistosomiasis: What we know and what we need to know. PloS Negl Trop Dis (2018) 12(2):e0006144–57. doi: 10.1371/journal.pntd.0006144
10. Hagan P, Sharaf O. Schistosomiasis vaccines. Expert Opin Biol Ther (2003) 3:1271–8. doi: 10.1517/14712598.3.8.1271
11. Preidis GA, Hotez PJ. The newest “Omics”–metagenomics and metabolomics–enter the battle against the neglected tropical diseases. PloS Negl Trop Dis (2015) 9:e0003382. doi: 10.1371/journal.pntd.0003382
12. Wishart DS. Applications of metabolomics in drug discovery and development. Drugs R D (2008) 9:307–22. doi: 10.2165/00126839-200809050-00002
13. Gowda GAN, Zhang S, Gu H, Asiago V, Shanaiah N, Raftery D. Metabolomics-based methods for early disease diagnostics: A review. Expert Rev Mol Diagn (2008) 8:617. doi: 10.1586/14737159.8.5.617
14. Tokarz J, Adamski J, Lanišnik Rižner T. Metabolomics for diagnosis and prognosis of uterine diseases? a systematic review. J Pers Med (2020) 10:294. doi: 10.3390/jpm10040294
15. Yang Q, Zhang A, Miao J, Sun H, Han Y, Yan G, et al. Metabolomics biotechnology, applications, and future trends: a systematic review. RSC Adv (2019) 9:37245–57. doi: 10.1039/C9RA06697G
16. Tounta V, Liu Y, Cheyne A, Larrouy-Maumus G. Metabolomics in infectious diseases and drug discovery. Mol Omics (2021) 17:376–93. doi: 10.1039/D1MO00017A
17. Inobaya MT, Olveda RM, Chau TN, Olveda DU, Ross AG. Prevention and control of schistosomiasis: a current perspective. Res Rep Trop Med (2014) 5:65. doi: 10.2147/RRTM.S44274
18. Krauth SJ, Balen J, Gobert GN, Lamberton PHL. A call for systems epidemiology to tackle the complexity of schistosomiasis, its control, and its elimination. Trop Med Infect Dis (2019) 4:21. doi: 10.3390/tropicalmed4010021
19. Sun L-P, Wang W, Zuo Y-P, Hong Q-B, Du G-L, Ma Y-C, et al. A multidisciplinary, integrated approach for the elimination of schistosomiasis: a longitudinal study in a historically hyper-endemic region in the lower reaches of the Yangtze river, China from 2005 to 2014. Infect Dis Poverty (2017) 6:56. doi: 10.1186/s40249-017-0270-x
20. Weerakoon KGAD, Gobert GN, Cai P, McManus DP. Advances in the diagnosis of human schistosomiasis. Clin Microbiol Rev (2015) 28:939–67. doi: 10.1128/CMR.00137-14
21. Adebayo AS, Mundhe SD, Awobode HO, Onile OS, Agunloye AM, Isokpehi RD, et al. Metabolite profiling for biomarkers in schistosoma haematobium infection and associated bladder pathologies. PloS Negl Trop Dis (2018) 12:e0006452. doi: 10.1371/journal.pntd.0006452
22. Bergquist J, Mv J JU. Diagnostic dilemmas in helminthology: what tools to use and when? Trends Parasitol (2009) 25(4):151–156. doi: 10.1016/j.pt.2009.01.004
23. Shiff C. Accurate diagnostics for schistosomiasis: a new role for PCR? RIP (2015) 4:23–9. doi: 10.2147/RIP.S74319
24. Alarcon-Barrera JC, Kostidis S, Ondo-Mendez A, Giera M. Recent advances in metabolomics analysis for early drug development. Drug Discovery Today (2022) 27:1763–73. doi: 10.1016/j.drudis.2022.02.018
25. Arksey H, O’Malley L. Scoping studies: towards a methodological framework. Int J Soc Res Method (2005) 8:19–32. doi: 10.1080/1364557032000119616
26. Tricco AC, Lillie E, Zarin W, O’Brien KK, Colquhoun H, Levac D, et al. PRISMA extension for scoping reviews (PRISMA-ScR): Checklist and explanation. Ann Intern Med (2018) 169:467–73. doi: 10.7326/M18-0850
27. Munn Z, Peters MDJ, Stern C, Tufanaru C, McArthur A, Aromataris E. Systematic review or scoping review? guidance for authors when choosing between a systematic or scoping review approach. BMC Med Res Methodol (2018) 18:143. doi: 10.1186/s12874-018-0611-x
28. Brien SE, Lorenzetti DL, Lewis S, Kennedy J, Ghali WA. Overview of a formal scoping review on health system report cards. Implement Sci (2010) 5:2. doi: 10.1186/1748-5908-5-2
29. Wang Y, Holmes E, Nicholson JK, Cloarec O, Chollet J, Tanner M, et al. Metabonomic investigations in mice infected with schistosoma mansoni: an approach for biomarker identification. Proc Natl Acad Sci U.S.A. (2004) 101:12676–81. doi: 10.1073/pnas.0404878101
30. Garcia-Perez I, Angulo S, Utzinger J, Holmes E, Legido-Quigley C, Barbas C. Chemometric and biological validation of a capillary electrophoresis metabolomic experiment of schistosoma mansoni infection in mice. Electrophoresis (2010) 31:2338–48. doi: 10.1002/elps.200900523
31. Li JV, Holmes E, Saric J, Keiser J, Dirnhofer S, Utzinger J, et al. Metabolic profiling of a schistosoma mansoni infection in mouse tissues using magic angle spinning-nuclear magnetic resonance spectroscopy. Int J Parasitol (2009) 39:547–58. doi: 10.1016/j.ijpara.2008.10.010
32. Manivannan B, Jordan TW, Secor WE, La Flamme AC. Proteomic changes at 8 weeks after infection are associated with chronic liver pathology in experimental schistosomiasis. J Proteomics (2012) 75:1838–48. doi: 10.1016/j.jprot.2011.12.025
33. Li JV, Saric J, Wang Y, Keiser J, Utzinger J, Holmes E. Chemometric analysis of biofluids from mice experimentally infected with schistosoma mansoni. Parasit Vectors (2011) 4:179. doi: 10.1186/1756-3305-4-179
34. Balog CIA, Meissner A, Göraler S, Bladergroen MR, Vennervald BJ, Mayboroda OA, et al. Metabonomic investigation of human schistosoma mansoni infection. Mol Biosyst (2011) 7:1473–80. doi: 10.1039/C0MB00262C
35. Manivannan B, Rawson P, Jordan TW, Karanja DMS, Mwinzi PNM, Secor WE, et al. Identification of cytokeratin 18 as a biomarker of mouse and human hepatosplenic schistosomiasis. Infect Immun (2011) 79:2051–8. doi: 10.1128/IAI.01214-10
36. Onile OS, Calder B, Soares NC, Anumudu CI, Blackburn JM. Quantitative label-free proteomic analysis of human urine to identify novel candidate protein biomarkers for schistosomiasis. PloS Negl Trop Dis (2017) 11:e0006045. doi: 10.1371/journal.pntd.0006045
37. Osakunor DNM, Mduluza T, Osei-Hyiaman D, Burgess K, Woolhouse MEJ, Mutapi F. Schistosoma haematobium infection is associated with alterations in energy and purine-related metabolism in preschool-aged children. PloS Negl Trop Dis (2020) 14:e0008866. doi: 10.1371/journal.pntd.0008866
38. Bexkens ML, van Gestel RA, van Breukelen B, Urbanus RT, Brouwers JF, Nieuwland R, et al. Schistosoma mansoni infection affects the proteome and lipidome of circulating extracellular vesicles in the host. Mol Biochem Parasitol (2020) 238:111296. doi: 10.1016/j.molbiopara.2020.111296
39. Loyo RM, Zarate E, Barbosa CS, Simoes-Barbosa A. Gas chromatography-mass spectrometry (GC/MS) reveals urine metabolites associated to light and heavy infections by schistosoma mansoni in mice. Parasitol Int (2021) 80:102239. doi: 10.1016/j.parint.2020.102239
40. Bueding E. Carbohydrate metabolism of schistosoma mansoni. J Gen Physiol (1950) 33:475–95. doi: 10.1085/jgp.33.5.475
41. Rigoulet M, Bouchez CL, Paumard P, Ransac S, Cuvellier S, Duvezin-Caubet S, et al. Cell energy metabolism: An update. Biochim Biophys Acta Bioenerg (2020) 1861:148276. doi: 10.1016/j.bbabio.2020.148276
42. Tielens AG, Celik C, Van den Heuvel JM, Elfring RH, Van den Bergh SG. Synthesis and degradation of glycogen by schistosoma mansoni worms in vitro. Parasitology (1989) 98(Pt 1):67–73. doi: 10.1017/s0031182000059692
43. Yarian C, Toroser D, Sohal R. Aconitase is the main functional target of aging in the citric acid cycle of kidney mitochondria from mice. Mech Ageing Dev (2006) 127:79–84. doi: 10.1016/j.mad.2005.09.028
44. Osakunor DNM, Munk P, Mduluza T, Petersen TN, Brinch C, Ivens A, et al. The gut microbiome but not the resistome is associated with urogenital schistosomiasis in preschool-aged children. Commun Biol (2020) 3:1–11. doi: 10.1038/s42003-020-0859-7
45. Delaney J, Neville WA, Swain A, Miles A, Leonard MS, Waterfield CJ. Phenylacetylglycine, a putative biomarker of phospholipidosis: Its origins and relevance to phospholipid accumulation using amiodarone treated rats as a model. Biomarkers (2008) 9(3):271–290. doi: 10.1080/13547500400018570
46. Wilson S, Vennervald BJ, Dunne DW. Chronic hepatosplenomegaly in African school children: A common but neglected morbidity associated with schistosomiasis and malaria. PloS Negl Trop Dis (2011) 5:e1149. doi: 10.1371/journal.pntd.0001149
47. You H, Stephenson RJ, Gobert GN, McManus DP. Revisiting glucose uptake and metabolism in schistosomes: new molecular insights for improved schistosomiasis therapies. Front Genet (2014) 5:176. doi: 10.3389/fgene.2014.00176
48. Senussi NH, Manne V. Persistently elevated liver enzymes caused by schistosoma mansoni. Clin Gastroenterol Hepatol (2022) 20:A18. doi: 10.1016/j.cgh.2020.08.050
49. Tanabe M, Kaneko N, Takeuchi T. Schistosoma mansoni: Higher free proline levels in the livers of infected mice. Exp Parasitol (1991) 72:134–44. doi: 10.1016/0014-4894(91)90131-F
50. Liu X, Zhang Y-R, Cai C, Ni X-Q, Zhu Q, Ren J-L, et al. Taurine alleviates schistosoma-induced liver injury by inhibiting the TXNIP/NLRP3 inflammasome signal pathway and pyroptosis. Infection Immun (2019) 87:e00732–19. doi: 10.1128/IAI.00732-19
51. Fitzpatrick JM, Fuentes JM, Chalmers IW, Wynn TA, Modolell M, Hoffmann KF, et al. Schistosoma mansoni arginase shares functional similarities with human orthologs but depends upon disulphide bridges for enzymatic activity. Int J Parasitol (2009) 39:267–79. doi: 10.1016/j.ijpara.2008.06.015
52. Asahi H, Osman A, Cook RM, LoVerde PT, Stadecker MJ. Schistosoma mansoni phosphoenolpyruvate carboxykinase, a novel egg antigen: immunological properties of the recombinant protein and identification of a T-cell epitope. Infect Immun (2000) 68:3385–93. doi: 10.1128/IAI.68.6.3385-3393.2000
53. Colley DG, Secor WE. Immunology of human schistosomiasis. Parasite Immunol (2014) 36:347–57. doi: 10.1111/pim.12087
54. Dubovsky JA, Chappell DL, Harrington BK, Agrawal K, Andritsos LA, Flynn JM, et al. Lymphocyte cytosolic protein 1 is a chronic lymphocytic leukemia membrane-associated antigen critical to niche homing. Blood (2013) 122:3308–16. doi: 10.1182/blood-2013-05-504597
55. Cheng W-J, Gu M-J, Ye F, Zhang Y-D, Zhong Q-P, Dong H-F, et al. Prohibitin 1 (PHB1) controls growth and development and regulates proliferation and apoptosis in schistosoma japonicum. FASEB J (2020) 34:11030–46. doi: 10.1096/fj.201902787RRR
56. Wieczorek M, Abualrous ET, Sticht J, Álvaro-Benito M, Stolzenberg S, Noé F, et al. Major histocompatibility complex (MHC) class I and MHC class II proteins: Conformational plasticity in antigen presentation. Front Immunol (2017) 8:292. doi: 10.3389/fimmu.2017.00292
57. Meurs L, Mbow M, Boon N, Vereecken K, Amoah AS, Labuda LA, et al. Cytokine responses to schistosoma mansoni and schistosoma haematobium in relation to infection in a Co-endemic focus in northern Senegal. PloS Negl Trop Dis (2014) 8:e3080. doi: 10.1371/journal.pntd.0003080
58. Chalmers IW, Hoffmann KF. Platyhelminth venom allergen-like (VAL) proteins: revealing structural diversity, class-specific features and biological associations across the phylum. Parasitology (2012) 139:1231–45. doi: 10.1017/S0031182012000704
59. Iruela-Arispe ML. Membrane lipids and cell signaling. Curr Opin Lipidol (2017) 28:408–13. doi: 10.1097/MOL.0000000000000443
60. Saito R de F, Andrade LN de S, Bustos SO, Chammas R. Phosphatidylcholine-derived lipid mediators: The crosstalk between cancer cells and immune cells. Front Immunol (2022) 13:768606. doi: 10.3389/fimmu.2022.768606
61. Cheng M, Bhujwalla ZM, Glunde K. Targeting phospholipid metabolism in cancer. Front Oncol (2016) 6:266. doi: 10.3389/fonc.2016.00266
62. Szlasa W, Zendran I, Zalesińska A, Tarek M, Kulbacka J. Lipid composition of the cancer cell membrane. J Bioenerg Biomembr (2020) 52:321–42. doi: 10.1007/s10863-020-09846-4
63. Botelho MC, Alves H, Richter J. Estrogen catechols detection as biomarkers in schistosomiasis induced cancer and infertility. Lett Drug Des Discovery (2017) 14:135–8. doi: 10.2174/1570180813666160720165057
64. Correia da Costa JM, Vale N, Gouveia MJ, Botelho MC, Sripa B, Santos LL, et al. Schistosome and liver fluke derived catechol-estrogens and helminth associated cancers. Front Genet (2014) 5:444. doi: 10.3389/fgene.2014.00444
65. Baez S, Segura-Aguilar J, Widersten M, Johansson AS, Mannervik B. Glutathione transferases catalyse the detoxication of oxidized metabolites (o-quinones) of catecholamines and may serve as an antioxidant system preventing degenerative cellular processes. Biochem J (1997) 324(Pt 1):25–8. doi: 10.1042/bj3240025
66. Chitsulo L, Engels D, Montresor A, Savioli L. The global status of schistosomiasis and its control. Acta Trop (2000) 77:41–51. doi: 10.1016/S0001-706X(00)00122-4
67. Lokhov PG, Trifonova OP, Maslov DL, Lichtenberg S, Balashova EE. Personal metabolomics: A global challenge. Metabolites (2021) 11(11):715. doi: 10.3390/metabo11110715
68. Secor WE. Early lessons from schistosomiasis mass drug administration programs. F1000Res (2015) 4:F1000 Faculty Rev–1157. doi: 10.12688/f1000research.6826.1
69. Johnson CH, Gonzalez FJ. Challenges and opportunities of metabolomics. J Cell Physiol (2012) 227:2975–81. doi: 10.1002/jcp.24002
70. Chen L, Zhong F, Zhu J. Bridging targeted and untargeted mass spectrometry-based metabolomics via hybrid approaches. Metabolites (2020) 10:348. doi: 10.3390/metabo10090348
71. Mduluza T, Mutapi F. Putting the treatment of paediatric schistosomiasis into context. Infect Dis Poverty (2017) 6:1–6. doi: 10.1186/s40249-017-0300-8
72. Bracken MB. Why animal studies are often poor predictors of human reactions to exposure. J R Soc Med (2009) 102:120–2. doi: 10.1258/jrsm.2008.08k033
73. Ialongo C, Bernardini S. Phlebotomy, a bridge between laboratory and patient. Biochem Med (Zagreb) (2016) 26:17–33. doi: 10.11613/BM.2016.002
Keywords: metabolites, biomarkers, S. haematobium, S. mansoni, schistosomiasis
Citation: Midzi H, Vengesai A, Muleya V, Kasambala M, Mduluza-Jokonya TL, Chipako I, Siamayuwa CE, Mutapi F, Naicker T and Mduluza T (2023) Metabolomics for biomarker discovery in schistosomiasis: A systematic scoping review. Front. Trop. Dis 4:1108317. doi: 10.3389/fitd.2023.1108317
Received: 25 November 2022; Accepted: 19 January 2023;
Published: 31 January 2023.
Edited by:
Srinivasa P. S. Rao, Novartis Institute for Tropical Diseases, United StatesReviewed by:
Bartholomew N. Ondigo, Egerton University, KenyaCopyright © 2023 Midzi, Vengesai, Muleya, Kasambala, Mduluza-Jokonya, Chipako, Siamayuwa, Mutapi, Naicker and Mduluza. This is an open-access article distributed under the terms of the Creative Commons Attribution License (CC BY). The use, distribution or reproduction in other forums is permitted, provided the original author(s) and the copyright owner(s) are credited and that the original publication in this journal is cited, in accordance with accepted academic practice. No use, distribution or reproduction is permitted which does not comply with these terms.
*Correspondence: Herald Midzi, bWlkemloZXJhbGRAZ21haWwuY29t
Disclaimer: All claims expressed in this article are solely those of the authors and do not necessarily represent those of their affiliated organizations, or those of the publisher, the editors and the reviewers. Any product that may be evaluated in this article or claim that may be made by its manufacturer is not guaranteed or endorsed by the publisher.
Research integrity at Frontiers
Learn more about the work of our research integrity team to safeguard the quality of each article we publish.