- 1Department of Disease Control, London School of Hygiene and Tropical Medicine, London, United Kingdom
- 2Innovative Vector Control Consortium, Liverpool School of Tropical Medicine, Liverpool, United Kingdom
- 3Pan-African Malaria Vector Research Consortium, Kilimanjaro Christian Medical University College, Moshi, Kilimanjaro, Tanzania
Introduction: Broflanilide is a novel meta-diamide insecticide, which has a distinct mode of action compared to other active ingredients used for Indoor Residual Spraying (IRS). This study details a laboratory evaluation of broflanilide, of putative discriminating concentrations, potential cross-resistance, and residual efficacy on two substrates.
Methods: Mosquitoes were exposed to broflanilide in bottle bioassays to determine lethal concentrations (LC50 and LC95). These were used to calculate resistance ratios between the susceptible Kisumu and the pyrethroid-resistant Muleba-Kis strains of Anopheles gambiae s.s. Prototype wettable powder formulations of broflanilide were applied to mud and concrete to determine the optimal observation period for determining delayed mortality, and a Cox proportional hazards model was fitted to determine a potential dose response effect. Subsequently, residual efficacy of application rates ranging between 25 mg/m2 and 300 mg/m2 were monitored monthly.
Results: LC values of tested strains were not significantly different; therefore, the polyfactorial resistance mechanisms possessed by the resistant strain did not confer resistance to broflanilide. A significant effect of concentration and time since spraying was found on mosquito survival, indicating that higher broflanilide concentrations are more effective and that this effect was strongest immediately after spraying. Knockdown at 60min post exposure was negligible, with on average only 1% of all mosquitoes knocked down. On the mud surface, but not on concrete, there was a delayed killing effect, with mortality increasing until 72 hours after exposure. The residual efficacy test indicated that on concrete the 100 mg/m2 and 200 mg/m2 concentrations of both broflanilide 50WP formulations remained efficacious for 9 months post spraying. On mud, there were large variations in mosquito mortality from month to month. Generally, higher concentrations resulted in higher mortality, despite variation over time.
Discussion: Cross-resistance to broflanilide was not detected in mosquitoes with multiple resistance mechanisms. This opens up the possibility for wide-scale use of broflanilide, especially in areas with established pyrethroid resistance. Like some other insecticide classes, delayed mortality up to 72 hours post exposure was found for broflanilide. Promising residual efficacy was found with broflanilide 50WP formulations on concrete. On mud, efficacy varied and further testing with a refined formulation is recommended.
Introduction
Mosquito vector control currently relies heavily on a limited number of insecticides in even fewer insecticidal classes. Pyrethroids, a class of insecticides used in all insecticide treated nets and, to a lesser extent, for indoor residual sprays (IRS), have been the foundation of mosquito control for over 30 years. The overuse of insecticides from this class has resulted in the evolution and spread of resistance in mosquitoes (1–4), and in some locations pyrethroid-only treated nets are failing to control malaria mosquitoes (5, 6). For IRS a wider range of insecticidal classes are WHO prequalified, and large IRS spray campaigns, like the PMI VectorLink Project, are currently conducted using formulations containing either an organophosphate or a neonicotinoid (7). To delay the emergence and spread of insecticide resistance, the World Health Organisation (WHO) ‘Global plan for insecticide resistance management in malaria vectors’ (GPIRM) recommends annual rotation of at least three insecticides with different modes of action (8). With the current available IRS products this goal cannot be achieved, and there is thus a dire need for novel insecticides that are effective against mosquitoes with multiple resistance mechanisms to maintain the momentum towards malaria eradication over the next 20 years (9, 10).
Broflanilide [N-[2-bromo-4-(perfluoropropan-2-yl)-6-(trifluoromethyl)phenyl]-2-fluoro-3-(N-methylbenzamido)benzamide] is a novel meta-diamide insecticide discovered by Mitsui Chemicals Agro, Inc. (MCAG) and further developed in collaboration with BASF (11). Broflanilide targets the gamma-aminobutyric acid (GABA) gated chloride channel in the insect nervous system (12, 13), and has been classified by the Insecticide Resistance Action Committee in the new group 30; GABA-gated chloride channel allosteric modulators (14). In insects GABA is a major inhibitory neurotransmitter both in the central nervous system and in the muscular system. The GABA neurotransmitter is received by the GABA-receptors that gate chloride channels in the target cell’s (postsynaptic) membrane. Opening of the chloride channels leads to the influx of negatively charged chloride (Cl-) ions into the postsynaptic cell, thereby inhibiting the activity of the cell. Interference with the GABA-gated chloride channel leads to hyperexcitation and convulsions in insects (15).
Broflanilide has shown efficacy against various agricultural pests such as wireworm (Agriotes obscurus, L. 1758) (16), cotton bollworm (Helicoverpa armigera, Hübner 1808), two‐spotted spider mite (Tetranychus urticae, Koch 1836) (17), and beet armyworm (Spodoptera exigua, Hübner 1808) (18). A human safety assessment, ecological risk assessment, and tolerances for residues of broflanilide were published by the Environmental Protection Agency (EPA) (19, 20) and broflanilide was registered for use by the EPA in 2021 (21).
In parallel to the development of broflanilide (tradename TENEBENAL™) for the agricultural market, its potential as an insecticide for public health has been investigated. Recently the President Malaria Initiative published their programmatic environmental assessment and concluded that no adverse human health effects are expected from the use of broflanilide in IRS (22). The development of broflanilide for use in malaria vector control was initiated by a collaboration between MCAG and the Innovative Vector Control Consortium (23). The efficacy of broflanilide for use against mosquitoes was evaluated at the Pan-African Vector Control Research Consortium at the Kilimanjaro Christina Medical University College in Moshi, Tanzania, in collaboration with the London School for Hygiene and Tropical Medicine. To determine the characteristics of a novel insecticide for vector control, a series of tests are recommended in guidelines published by the WHO (24). These initial laboratory tests (formerly known as Phase I tests) include determining the discriminating concentration of a novel insecticide, evaluating potential cross-resistance through mechanisms of resistance to other insecticides, and determining the residual efficacy on typical house wall substrates.
Resistance to pyrethroids is widespread and as many as 87% of countries monitoring resistance reported it in at least one malaria vector species in 2020 (25). Determining potential cross-resistance to novel insecticides offered by mechanisms of resistance to pyrethroids is crucial to predicting operational success of the novel products. Bottle bioassays with a range of concentrations of active ingredient is the commonly used method to evaluate potential cross-resistance between insecticide classes (26). To detect emerging resistance in mosquito populations it is necessary to first define baseline susceptibility by determining the discriminating concentration (27). Here we report a) determination of the lethal concentrations that kill 50% and 95% of mosquitoes, and b) exploration of possible cross-resistance against these values using mosquito strains with different genotypic and phenotypic resistance profiles. All experiments were conducted with a prototype formulation of broflanilide.
Historically, the residual efficacy of novel compounds was tested using standardized cone bioassays (24, 28), which were developed to evaluate fast-acting neurotoxic insecticides like pyrethroids. However, with recently developed public health insecticides which act on alternative target sites in the mosquito, cone assays have not always been the optimal method to measure efficacy (29). Slower-acting (>24h mortality) insecticides that have recently been developed include the non-pyrethroid insecticides clothianidin (30–33) and chlorfenapyr IRS (34–36). Broflanilide, like chlorfenapyr, is a pro-insecticide that is metabolised to its active form inside the mosquito and the time to kill may extend beyond 24 hours (13). The optimal observation period to determine killing effect post-exposure was determined using a range of low concentrations of a broflanilide formulation sprayed on concrete and mud blocks.
The duration of effective action of an insecticide depends on several factors that influence insecticide decay and bioavailability on wall surfaces. Activity of insecticides may decrease when degrading to inactive forms, and bioavailability is reduced if insecticide is lost from the surface through volatilization, absorption or run-off. Interactions between insecticides and surfaces to which they are applied has been shown to have a large impact on efficacy (37, 38). In our study the residual efficacy of the wettable powder formulation of broflanilide at various concentrations was determined under laboratory conditions on two common wall substrates, concrete and mud. Data from this study was used to decide on a restricted number of target doses for experimental hut evaluations (39).
Materials and methods
Mosquitoes
Three mosquito strains were used: Anopheles (An.) gambiae s.s. Kisumu, An. gambiae s.s. Muleba-Kis, and An. gambiae s.l. wildtype. Pyrethroid resistance in the An. gambiae s.s. Muleba-Kis strain is polyfactorial: the strain is homozygous for the L1014S kdr mutation (kdr-east) and the cytochrome P450 CYP6P3 is overexpressed (40). An. gambiae s.s. Kisumu is fully susceptible to all insecticides. The Ace-1 (G119S) mutation is absent from the insectary-reared strains and from the wildtype An. gambiae s.l. The insectary-reared strains are routinely characterised in terms of body weight, wing length, resistance status (phenotypic and genotypic) and species identification. Routine tests using WHO test papers (41) indicated susceptibility to carbamates and organophosphates for both strains, and resistance of the Muleba-Kis strain to organochlorines and pyrethroids (40). The wildtype mosquitoes were collected as larvae from rice fields near the Harusini field station (3°40’S, 37°36’E) and reared to adults in the field insectary prior to testing. All mosquitoes were used as unfed 2-5d old adult females.
Insecticide formulations
Both the technical grade and the 50WP formulations of broflanilide were supplied by Mitsui Chemicals Agro, Inc. (MCAG; Tokyo, Japan). The technical grade active ingredient (TG-AI) had a purity of 98% (batch no. A150640B505). The wettable powder formulations had a concentration of 50% active ingredient and were supplied in two recipes: B1 (batch no. 16I-3148) and B2 (batch no. 16I-3147).
Bottle bioassays
A working solution of broflanilide was prepared by dissolving 5.1 mg technical grade in 25 mL acetone to give a concentration of 200 µg/mL. This working solution was further diluted in acetone to create six additional concentrations, 121.39 µg/mL, 73.68 µg/mL, 44.72 µg/mL, 27.14 µg/mL, 16.48 µg/mL and 10.00 µg/mL. Bottles coated with 1mL acetone only were used as a negative control. New 250mL Wheaton bottles were coated with insecticide for the assays and allowed to dry overnight at room temperature. Coated bottles were kept in the laboratory at room conditions until use and all tests were completed within 5 days of coating.
Mosquitoes were held under assay conditions (target range: 27 ± 2°C and 75 ± 10%RH) pre-exposure for a minimum of one hour. One hour before exposure, glucose-soaked cotton wool was removed from the mosquito holding cups. Separate aspirators were used to transfer 25 ± 5 mosquitoes into each negative control (acetone only) bottle and each insecticide-treated bottle. All seven concentrations and the control treatment were tested simultaneously, and a 1-hour exposure time was used throughout. Mosquitoes were then transferred back to the holding cups, were given ad libitum access to 10% glucose-soaked cotton wool, and mortality was recorded at 24h intervals, up to 72 hours post-exposure.
Insecticide application on substrates in the laboratory
Broflanilide 50WP B1 and B2 formulations were sprayed on mud and concrete blocks of 8.5 centimetre in diameter, using an auto-load precision spray Potter tower (Burkard Manufacturing Co Ltd, Rickmansworth, United Kingdom). Prior to spraying the Potter tower was centralised and calibrated using tap water at room temperature. Calibration was accepted when the volume sprayed was within 10% of the target volume for each position. Mud blocks were prepared using a ratio of 2 parts soil, 3 parts sand and 1.25 parts water and were left to dry at 27 ± 2°C and 80 ± 10%RH for at least one week before use. Concrete blocks were made using a ratio of 2 parts cement, 4 parts sand and 1.2 parts water and were left to dry under the same conditions for a minimum of one month.
Concentrations of broflanilide 50WP formulations that were at least 10-fold lower than those originally tested by Lees et al. (42) were used to determine the optimal observation period post-exposure and potential dose response of the insecticide. These low application rates, 0.5 mg/m2, 1.25 mg/m2, 2.5 mg/m2 and 5.0 mg/m2, were anticipated to result in less than 100% mortality allowing for comparisons between observation times and different concentrations. The negative controls consisted of unsprayed mud and concrete blocks.
Two mosquito strains, Kisumu and Muleba-Kis, were exposed to treated and untreated blocks 24h and 1 week after treatment. Blocks were stored after spraying and between tests at 30 ± 2°C and 80 ± 10%RH (24).
For the residual efficacy experiment, additional mud and concrete blocks were prepared and stored as described above. Application rates of 25 mg/m2, 50 mg/m2, 100 mg/m2, 200 mg/m2 and 300 mg/m2 were chosen to replicate work done by Lees et al. (42). The negative controls consisted of unsprayed mud and concrete blocks. Blocks were monitored for at least 6 months post spraying, and the monitoring period was extended, if possible, for those application rates that continued to show high mortality beyond 6 months.
Cone bioassays
Cone assays were conducted following WHOPES guidelines (24). Mosquitoes were held in paper cups, 5-10 mosquitoes per cup, for a pre-exposure period of at least one hour at 27 ± 2°C and 75 ± 10%RH. Ten mosquitoes were aspirated into each cone and exposed to the insecticide-treated and control blocks for 30 minutes under the same conditions. Two replicates were conducted per block for each mosquito strain. Post-exposure, mosquitoes were returned to the holding cups with ad libitum access to 10% glucose. Knockdown at 60 minutes after the end of the exposure period was recorded, and mortality was observed at 24h, 48h, 72h and 96h after exposure. Assay conditions were recorded every 10 minutes throughout the study using a Tinytag® View 2 Data Logger (Gemini Data Loggers Ltd, Chichester, United Kingdom).
Data management and analysis
Raw data hard-copy record sheets were double entered by a data entry clerk into a database in MS Access 2016. The two entries were compared using a query function, and where inconsistencies were detected the hard-copy data record sheets were consulted for the true value. Mosquito mortality in cone assays was corrected using Abbott’s formula if control mortality was higher than 5%.
The lethal doses killing 50% and 95% of mosquitoes (LC50 and LC95) in bottle bioassays were generated using the BioRssay package (43) in RStudio (Version 4.0.5) using a probit model and concentration on the log scale. The lethal concentration values were then used to calculate resistance ratios relative to the susceptible Kisumu strain. The dose response curves of the three strains were compared using a likelihood ratio test, using the glm function in R, with the quasi-binomial family to account for overdispersion.
A Cox proportional hazards model was fitted to determine the mosquito dose response in the cone assays. A failure event was defined as a mosquito dying during observation, and time was set as number of hours post-exposure, from 24 hours up to 96 hours. The following variables were included in the model: concentration and recipe (B1 or B2), substrate (concrete or mud), mosquito species/strain, time since spraying (1 day or 1 week). The Cox proportional hazards model was conducted in Stata I/C v.15 (StataCorp LLC, Texas, USA).
Residual efficacy was evaluated as the number of months that mosquito mortality was equal to or greater than 80% (24). When mosquito mortality dropped below 80% the latest timepoint at which the threshold was reached was used as the duration of effective action. For application rates that yielded mortality higher than 80% at the time monitoring was stopped, the residual efficacy was stated as at least the number of months that data was collected.
Results
Determining cross-resistance and lethal concentrations using bottle bioassays
The number of replicates per strain depended on mosquito availability: For the susceptible An. gambiae s.s. Kisumu strain 10 replicates with 25 mosquitoes were conducted; for the resistant An. gambiae s.s. Muleba-Kis strain 7 replicates were conducted. For the wild-type An. gambiae s.l. strain only 4 replicates were conducted due to challenges collecting sufficient larvae from the rice fields (Table 1).
Mosquito mortality at 72h was plotted against the log scale of concentrations of broflanilide for each strain, Figure 1. All three strains showed a dose-response curve; exposure to higher doses resulted in higher mortality. For the four replicates of wild type An. gambiae s.l. mosquitoes, one replicate produced results which showed much lower mortality for the 16.48 µg/mL and 27.14 µg/mL doses compared to the other 3 replicates, which resulted in large error bars for these datapoints. A Chi test indicated that for both the Kisumu and the Muleba-Kis strain a linear regression model could be fitted, however for the An. gambiae s.l. strain heterogeneity in the data was substantially higher and the data did not follow a linear regression. The An. gambiae s.l. data was thus excluded from further analysis.
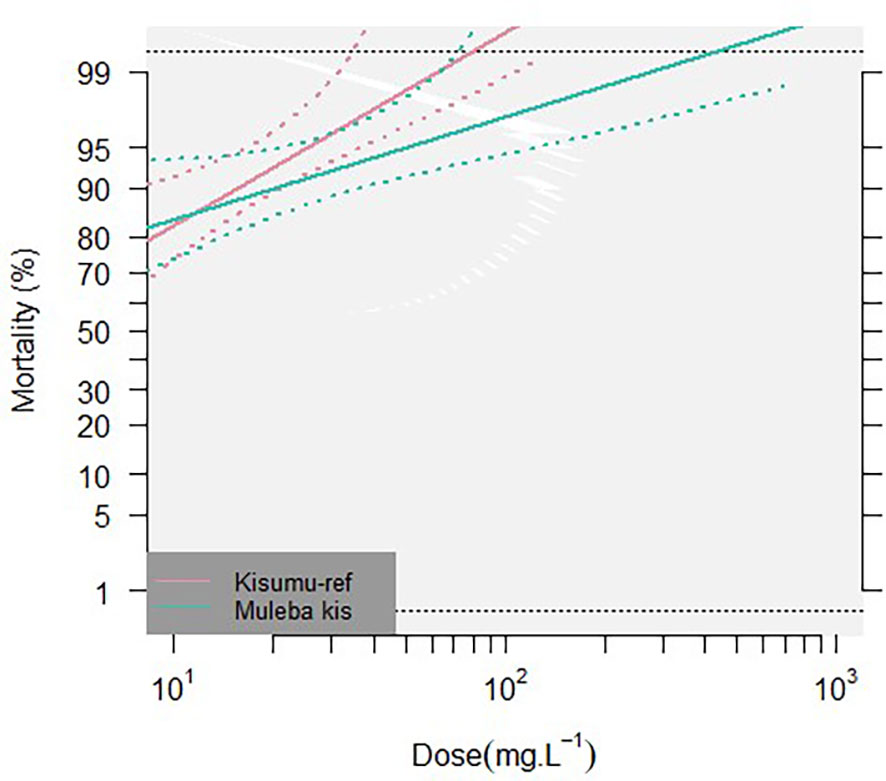
Figure 1 Percentage 72h mortality in bottle bioassays. Two insectary-reared strains, Kisumu and Muleba-Kis, were exposed to 7 concentrations of broflanilide (BRF). Dose response curves (solid lines) ± 95% confidence intervals (dotted lines) are given for both strains.
Lethal doses that are predicted to kill 50% and 95% were calculated for both insectary strains (Table 2). Resistance ratios of the Muleba-Kis strain relative to the susceptible reference strain (Kisumu) were RR50: 0.29 (0.00, 1.64e5), and RR95: 2.01 (1.50, 2.70). The dose response curves of the two strains were compared using a likelihood ratio test, which did not result in significant differences (p=0.1449), indicating no cross resistance was conferred by the polyfactorial resistance mechanisms of the Muleba-Kis strain.
Evaluation of delayed mortality and dose response using cone assays
Mosquito survival was analysed using a Cox proportional hazards model. The Schoenfeld residuals test indicated that the proportional-hazards assumption was not met, so the model was then stratified by substrate and re-run.
Mosquito strain (Muleba-Kis vs Kisumu) and broflanilide recipes (B1 vs B2) did not have a significant effect on survival, therefore were grouped for further analysis. A significant effect of concentration [HR] 1.24, (95% CI 1.22-1.26), p<0.001 and time since spraying [HR] 1.08 (95% CI 1.01-1.16), p<0.05 was found on mosquito survival, indicating that higher concentrations are more effective in killing mosquitoes and that this effect was stronger 1 day after spraying compared to 1 week post spraying, Figure 2.
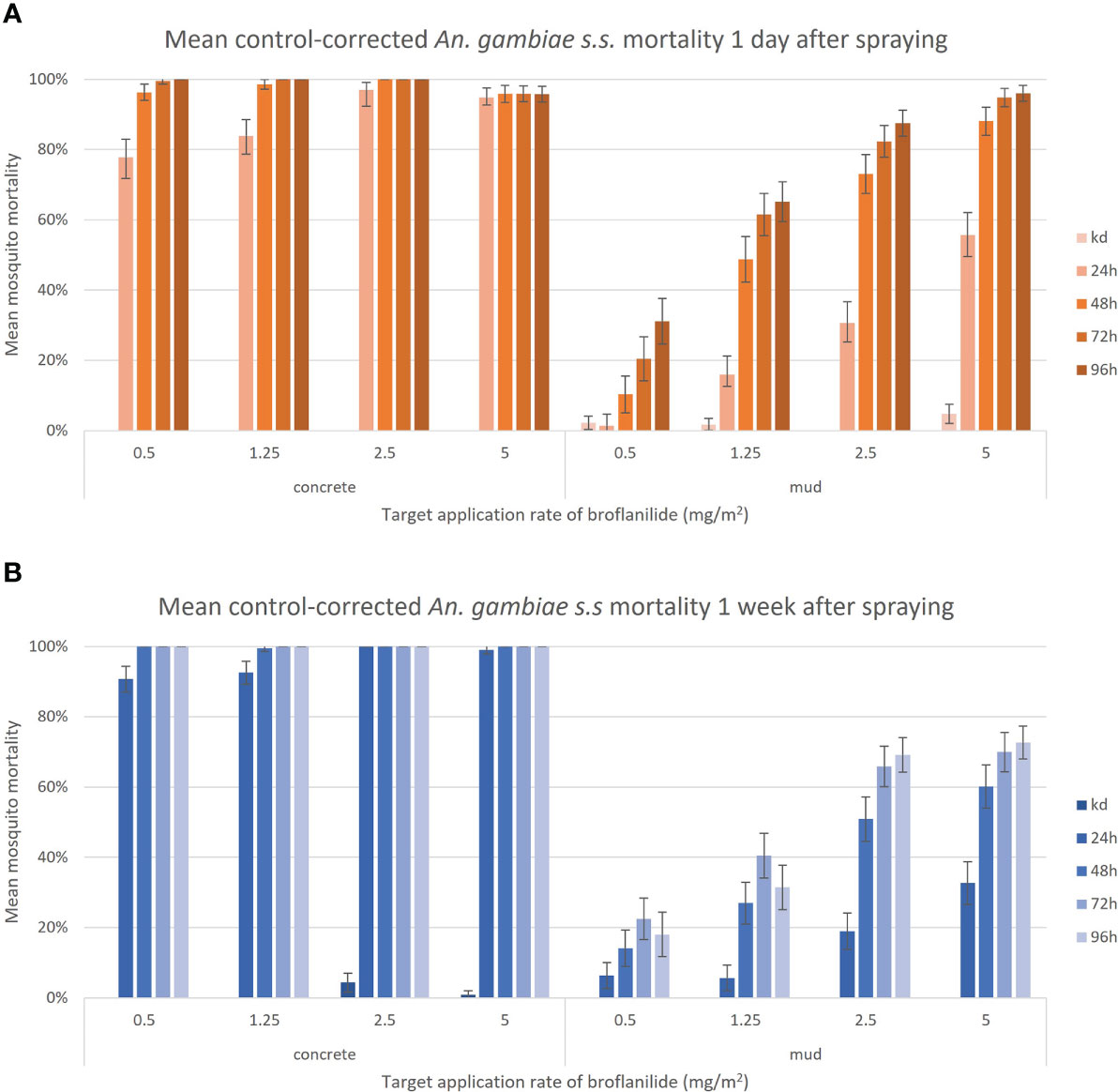
Figure 2 Control-corrected mean mortality of insectary-reared An. gambiae s.s. against a range of low concentrations of broflanilide 50WP, exposed 1 day (A) or 1 week (B) post application. Graphs show grouped data from the An. gambiae s.s. Kisumu and An. gambiae s.s. Muleba-Kis strains, and the two broflanilide recipes. All mosquitoes were monitored up to 96 hours post exposure on two substrates in a laboratory setting. Each bar represents the mean mortality (± 95% CI).
Knockdown at 60min post exposure was negligible, with on average only 1% of all mosquitoes being knocked down. Broflanilide sprayed on concrete surfaces was highly efficacious at all tested concentrations, resulting in >90% of mosquitoes dead after 24h and >99% mortality 48h after exposure. On the mud surface there was a delayed mortality effect, with control-corrected mortality increasing until 72 hours after exposure. There was some evidence of further mortality beyond 72h, although this was masked by increasing control mortality in some cases.
Residual efficacy of broflanilide 50WP by recipe, concentration, and block substrate
On a concrete surface the two highest concentrations, 100 mg/m2 and 200 mg/m2, of broflanilide 50WP remained efficacious, with mortality higher than 80%, for at least 9 months post-exposure, Figure 3. The 25 mg/m2 dose was effective for 3 months for both recipes and dropped below 80% mortality afterwards. Exposure to the 50 mg/m2 dose resulted in an 89% mean mortality (recipe B2) and 76% mortality (recipe B1) after 6 months.
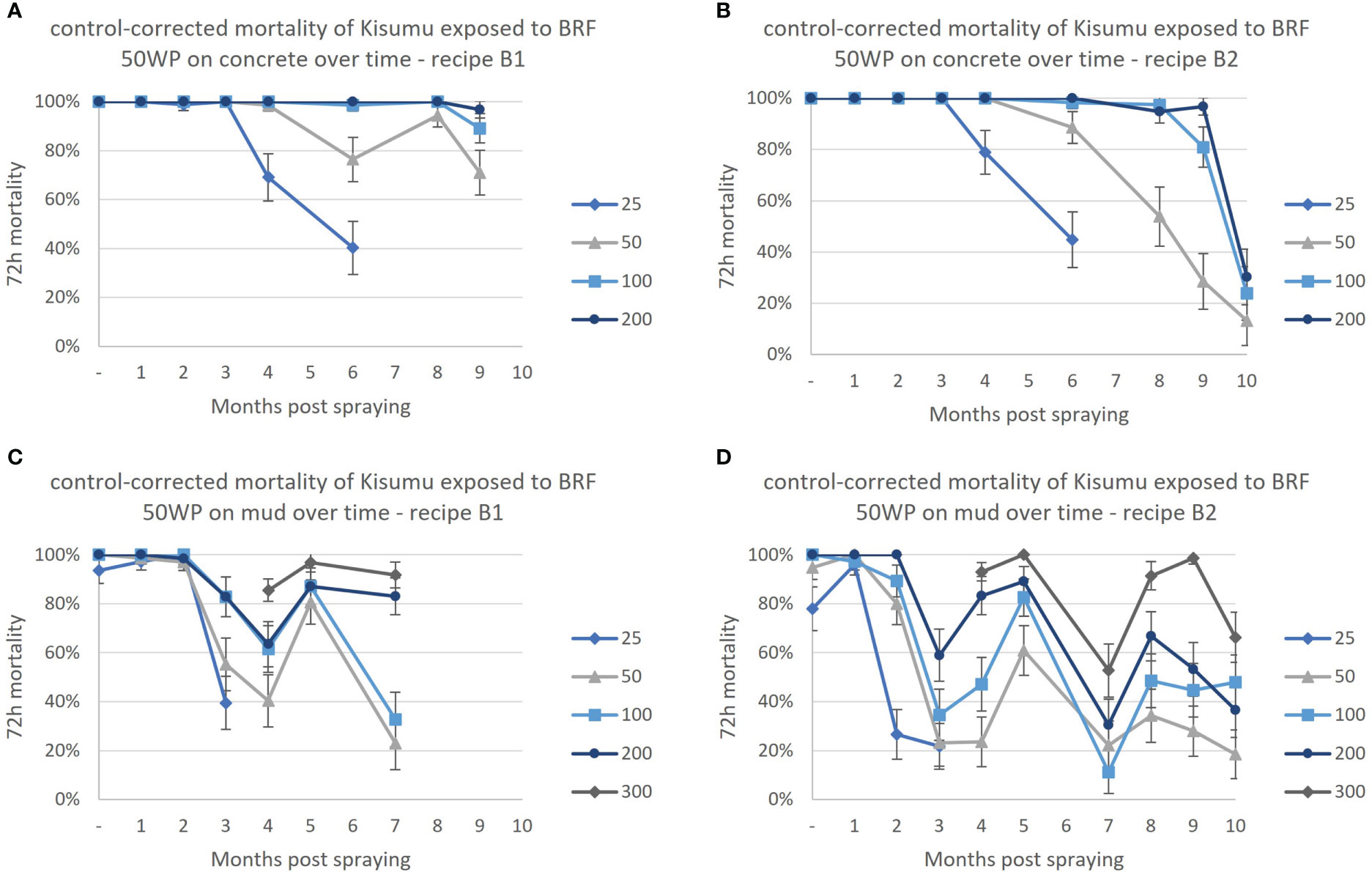
Figure 3 Residual efficacy of broflanilide (BRF) at a range of concentrations in mg a.i./m2 against the susceptible Kisumu strain. (A) BRF recipe B1 on a concrete surface, (B) BRF recipe B2 on a concrete surface, (C) BRF recipe B1 on a mud surface, (D) BRF recipe B2 on a mud surface. Monthly cone assays were conducted up to 10 months post-application. Two recipes of broflanilide 50WP; B1 (graph A, C) and B2 (graph B, D) were evaluated on two substrates; concrete (graph A, B) and mud (graph C, D). Line graphs indicate the mean mortality per month (± 95% CI). The lowest concentration of 25 mg/m2 was discontinued after 3 months on the mud surface, after which the 300 mg/m2 concentration was introduced.
Mosquito mortality showed large variation from month to month on mud substrate (Figure 3). In general, the higher concentrations resulted in a higher mortality, despite the variation over time. The lowest concentration, 25 mg/m2, resulted in mortality higher than 80% for 1 month (Recipe B2) or 2 months (recipe B1) only and was discontinued after 3 months and the highest concentration 300 mg/m2 was introduced at month 4.
On mud surfaces residual efficacy of broflanilide 50WP recipe B1 was 2 months at 25 mg/m2 and 50 mg/m2, 5 months at 100 mg/m2, and 7 or more months for the two highest concentrations 200 mg/m2 and 300 mg/m2.
For the second recipe, B2, residual efficacy of broflanilide 50WP on mud surfaces was 1 month for 25 mg/m2, 2 months for 50 mg/m2 and 100 mg/m2, 5 months for the 200 mg/m2 concentration, and 9 months for the highest concentration tested, 300 mg/m2.
Discussion
Broflanilide is a novel insecticide for malaria vector control that has undergone laboratory testing for the first time in East Africa using various Anopheline strains. During 1-hour bottle bioassays, even 10-fold lower concentrations of broflanilide were sufficient to generate high mortality of all three mosquito strains. There was no significant difference in mortality between the susceptible An. gambiae s.s. Kisumu strain and the resistant An. gambiae s.s. Muleba-Kis strain, a polyfactorial resistant strain with the L1014S kdr mutation and upregulated P450s. Similarly, in other studies, broflanilide was shown to be effective against Anopheles strains with >200 fold resistance to pyrethroids (44), and polyfactorial resistant strains with the L1014F and ace-1 mutations and upregulated P450 CYP6M2, CYP6P3 and CYP6P4 (42, 45).
Broflanilide acts on the GABA-receptor of mosquitoes, which is also the target site for cyclodienes such as dieldrin. Dieldrin was once widely used as an insecticide but, because of its persistence in the environment and relatively high mammalian toxicity, its use was prohibited following the 2004 Stockholm Convention of Persistent Organic Pollutants. Resistance to dieldrin, following a point mutation in the GABA receptor, was reported in wild An. gambiae s.s. populations as early as 1956 (46). Cross-resistance between RDL (resistant to dieldrin) mutant mosquitoes and other insecticides targeting the GABA-receptor can significantly reduce effectiveness (47–50). Nakao et al. (2013) discovered that meta-diamides have a distinct mode of action compared to other insecticides targeting the GABA-receptor in that it binds to a different site (51), and that broflanilide was thus effective against cyclodiene- and fipronil-resistant insects (13). This was confirmed by Lees et al. where broflanilide was effective against a dieldrin selected strain; Kisumu RDL (42). Cross-resistance against dieldrin was not tested in this study due to absence of a strain possessing the RDL mutation at KCMUCo, but a recent study investigating the effect of dieldrin resistance on the efficacy of broflanilide confirmed that no association between the presence of the RDL mutation and survival in broflanilide bioassays could be found (52).
Determining the discriminating concentration for a novel insecticide is key to subsequent differentiation of susceptible and resistant populations, so that emerging resistance can be monitored, and next steps determined according to strategies outlined in the countries’ insecticide resistance management plans. In this study the LC95 for the susceptible Kisumu strain was found to be 25 µg/bottle, which is lower than results found in West Africa by Ngufor et al. where the LC95 for the susceptible Kisumu strain was 70 µg/bottle (44). Whilst the Kisumu strains colonised at many research facilities was derived from the same field sampled An. gambiae s.s. from Kisumu, Kenya in 1953, variation in environmental conditions and rearing techniques at these facilities may give rise to a concomitant variability in fitness and, therefore, in tolerance to insecticides. Recent studies have also mixed broflanilide with the adjuvant Mero® (81% rapeseed oil methyl ester) in bottle assays to reduce the crystallisation of broflanilide after the acetone solvent has evaporated (42, 53). Including Mero at 400ppm resulted in a lower LC95 of 3.97 µg/bottle, corresponding to a discriminating concentration of 11.91 µg/bottle (42). Current studies use a higher concentration of 500 or 800ppm Mero, with a tentative discriminating concentration of 6 µg/bottle in Benin (54), and a range of discriminating concentrations between 0.74 μg/ml and 17.82 μg/ml in a recent multicentre study (52). Further research is recommended to reach consensus on the optimal discriminating concentration of broflanilide, including the optimal concentration of any adjuvant used.
Broflanilide was formulated as a 50% wettable powder IRS product, and evaluations on mud and concrete substrate were conducted using two recipes, B1 and B2. Using low concentrations of broflanilide showed delayed mortality on the mud surface, with an optimal post-exposure period of 72 hours. This result aligned with experiments performed in Benin, where it was also found that 72 hours is an optimal post-exposure observation period (44). Observation of delayed mortality has become standard practice for next generation insecticides, which are typically slower-acting than pyrethroids, including 120 hours for clothianidin formulated as SumiShield® 50WG (32, 55), 72 hours for chlorfenapyr formulated as Sylando® 240CS (34) and 72 or 120 hours for clothianidin (and deltamethrin) formulated as Fludora® Fusion (56–59).
Analysis of the cone assay data demonstrated a significant effect of broflanilide 50WP application rate on mosquito survival indicating a dose-response effect. This effect was found both for the residual efficacy test and for the experiment with lower application rates of broflanilide. Residual efficacy of broflanilide ranged from 1 ≥ 9 months, depending on surface substrate, recipe, and application rate. Broflanilide performed better on concrete surfaces compared to mud surfaces. Similar results have been reported with other insecticides used for IRS, where duration of effective action is typically longer on concrete surfaces compared to mud and dung surfaces (37, 60–62). On the concrete surface the 100mg/m2 and 200mg/m2 concentrations resulted in a residual life of at least 9 months post-application. On the mud surface efficacy was considerably variable but continued to show a dose-dependent effect. Residual efficacy on mud did not reach a similar duration to that reported from other laboratory studies, where mortality of the susceptible Kisumu strain was maintained >80% up to 5 months in Liverpool (42) and up to 14 months in Burkina Faso (63). It is important to note that properties of mud can vary strongly between different locations, and efficacy of an insecticide can thus vary based on where it is tested. The results reported here triggered MCAG to develop an improved recipe (B3), which was designed to improve performance on a mud surface, specifically surfaces with a high porosity as found in Tanzania. This B3 recipe was subsequently tested in semi-field experiments with broflanilide WP in Tanzania, where 7 months of efficacy was recorded at an application rate of 150mg/m2 (39) Similarly, in Benin, Ngufor et al. reported at least 6 months of residual efficacy (44), and current experiments suggest a duration of effective action of up to 18 months on a mud surface in some locations (54). Overall, initial testing with broflanilide 50WP demonstrated that it meets the target product profile established for IRS products (64).
Conclusion
At least three insecticide classes, in annual rotation, are recommended to decrease and slow down the spread and development of resistance in malaria endemic countries. In addition to the current neonicotinoid and organophosphate products, broflanilide 50WP has potential for use in such IRS rotations. This study showed that broflanilide has good efficacy against mosquitoes with multiple resistance mechanisms, providing no evidence of cross-resistance to broflanilide via the L1014S kdr mutation or upregulated P450s (CYP6P3) leading to pyrethroid resistance. This opens the possibility for wide-scale use of this insecticide for indoor residual spraying, especially in areas where pyrethroid-resistance is impacting on malaria vector control. Like some insecticides formulated into other WHO-PQT listed products, delayed mortality up to 72 hours post exposure was found for broflanilide. Residual efficacy testing showed promising results of two prototype formulations on a concrete surface, resulting in a duration of effective action of at least 9 months post exposure. On mud efficacy varied and further testing with the refined B3 recipe is recommended to determine what application rate of broflanilide 50WP should be used to give good residual efficacy on a range of substrate types.
Data availability statement
The raw data supporting the conclusions of this article will be made available by the authors, without undue reservation.
Author contributions
JS wrote the first draft of the manuscript, analysed and interpreted results, and visualised the data. MR and MK supervised the work and contributed to analysis interpretation. SA and BM supported with the conduct of the study and contributed to the final draft of the manuscript. DM contributed to the conception and design of the study. MK revised the final draft manuscript. All authors have read and approved the manuscript.
Funding
This study is funded by the Bill and Melinda Gates Foundation (grant no. OPP1148615) (https://www.gatesfoundation.org/) through the Innovative Vector Control Consortium. The funders had no role in study design, collection and analysis of data, decision to publish, or preparation of the manuscript.
Acknowledgments
The authors would like to thank all colleagues at the KCMUCo-PAMVERC Test Facility for their support with the conduct of the study. We are grateful to Kuni Mori (MCAG) for providing broflanilide samples and technical support, and to Angus Spiers, Graham Small and Derric Nimmo for providing useful comments and suggestions on an early draft. We also thank Prof. Franklin Mosha of the Kilimanjaro Christian Medical University College for contributing to setting up of the study, and Welbeck Achille Oumbouke for our useful discussions on statistical analysis.
Conflict of interest
The authors declare that the research was conducted in the absence of any commercial or financial relationships that could be construed as a potential conflict of interest.
Publisher’s note
All claims expressed in this article are solely those of the authors and do not necessarily represent those of their affiliated organizations, or those of the publisher, the editors and the reviewers. Any product that may be evaluated in this article, or claim that may be made by its manufacturer, is not guaranteed or endorsed by the publisher.
Abbreviations
An., Anopheles; BRF, Broflanilide; CI, Confidence Interval; GABA, gamma-aminobutyric acid; IRS, Indoor Residual Spraying; kdr, knockdown resistance; LC50, Lethal concentration 50%; LC95, Lethal concentration 95%; MCAG, Mitsui Chemicals Agro, Inc.; RDL, resistant to dieldrin; s.l., sensu lato; s.s., sensu stricto; WHO, World Health Organisation; WP, Wettable Powder.
References
1. Mathias DK, Ochomo E, Atieli F, Ombok M, Bayoh MN, Olang G, et al. Spatial and temporal variation in the kdr allele L1014S in anopheles gambiae s.s. and phenotypic variability in susceptibility to insecticides in Western Kenya. Malar J (2011) 10:10. doi: 10.1186/1475-2875-10-10
2. Main BJ, Lee Y, Collier TC, Norris LC, Brisco K, Fofana A, et al. Complex genome evolution in anopheles coluzzii associated with increased insecticide usage in Mali. Mol Ecol (2015) 24(20):5145–57. doi: 10.1111/mec.13382
3. Barnes KG, Weedall GD, Ndula M, Irving H, Mzihalowa T, Hemingway J, et al. Genomic footprints of selective sweeps from metabolic resistance to pyrethroids in African malaria vectors are driven by scale up of insecticide-based vector control. PloS Genet (2017) 13(2):e1006539. doi: 10.1371/journal.pgen.1006539
4. Oxborough RM. Trends in US president's malaria initiative-funded indoor residual spray coverage and insecticide choice in sub-Saharan Africa (2008-2015): urgent need for affordable, long-lasting insecticides. Malaria J (2016) 15:146. doi: 10.1186/s12936-016-1201-1
5. Asidi A, N'Guessan R, Akogbeto M, Curtis C, Rowland M. Loss of household protection from use of insecticide-treated nets against pyrethroid-resistant mosquitoes, benin. Emerging Infect Dis (2012) 18(7):1101–6. doi: 10.3201/eid1807.120218
6. Ochomo EO, Bayoh NM, Walker ED, Abongo BO, Ombok MO, Ouma C, et al. The efficacy of long-lasting nets with declining physical integrity may be compromised in areas with high levels of pyrethroid resistance. Malaria J (2013) 12:368. doi: 10.1186/1475-2875-12-368
7. Indoor residual spray operations calendar.: PMI VectorLink (2021). Available at: https://pmivectorlink.org/results/spray-calendar/.
8. WHO. Global plan for insecticide resistance management in malaria vectors. Geneva: World Health Organization (2012).
9. Hemingway J, Ranson H, Magill A, Kolaczinski J, Fornadel C, Gimnig J. Averting a malaria disaster: Will insecticide resistance derail malaria control? Lancet (2016) 387:1785–8. doi: 10.1016/S0140-6736(15)00417-1
10. Roberts L, Enserink M. Malaria. did they really say . eradication? Science (2007) 318(5856):1544–5.
11. Mitsui Chemicals. Mitsui and BASF agreement for new insecticide with IRAC novel mode of action. Int Pest Control (2018) 60(1):8–9.
12. Katsuta H, Nomura M, Wakita T, Daido H, Kobayashi Y, Kawahara A, et al. Discovery of broflanilide, a novel insecticide. J Pestic Sci (2019) 44(2):120–8. doi: 10.1584/jpestics.D18-088
13. Nakao T, Banba S. Broflanilide: A meta-diamide insecticide with a novel mode of action. Bioorganic Medicinal Chem (2016) 24(3):372–7. doi: 10.1016/j.bmc.2015.08.008
14. IRAC. IRAC mode of action classification scheme. CropLife International (2020). Version 9.3 at https://irac-online.org/documents/moa-classification/.
15. Osborne RH. Insect neurotransmission: neurotransmitters and their receptors. Pharmacol Ther (1996) 69(2):117–42. doi: 10.1016/0163-7258(95)02054-3
16. van Herk WG, Vernon RS, Goudis L, Mitchell T. Broflanilide, a meta-diamide insecticide seed treatment for protection of wheat and mortality of wireworms (Agriotes obscurus) in the field. J Econ Entomol (2021) 114(1):161–73. doi: 10.1093/jee/toaa239
17. Shen N, Li Y, Leviticus K, Chang XL, Tang T, Cui L, et al. Effect of broflanilide on the phytophagous mite tetranychus urticae and the predatory mite typhlodromips swirskii. Pest Manag Sci (2021) 77(6):2964–70. doi: 10.1002/ps.6335
18. Tang T, Hu F, Wang P, Fu W, Liu X. Broflanilide effectively controls helicoverpa armigera and spodoptera exigua exhibiting diverse susceptibilities to chlorantraniliprole and emamectin benzoate. Pest Manag Sci (2021) 77(3):1262–72. doi: 10.1002/ps.6139
19. EPA. Broflanilide; pesticide tolerances. Washington, DC: Environmental Protection Agency (2020). Available at: https://www.govinfo.gov/content/pkg/FR-2020-12-17/pdf/2020-27906.pdf.
20. Khan F, Farruggia F. Broflanilide: Comparison of hazard and Chemical/Fate properties for several alternative insecticides (2020). Available at: https://www.regulations.gov/document/EPA-HQ-OPP-2018-0053-0009.
21. EPA. Notice of pesticide registration. Washington, DC: Environmental Protection Agency (2021). Available at: https://www3.epa.gov/pesticides/chem_search/ppls/086203-00028-20210114.pdf.
22. Integrated Vector Management Programs for Malaria Vector Control. Programmatic environmental assessment. Fairfax, VA 22031: The environmental compliance support (ECOS). Available at: https://d1u4sg1s9ptc4z.cloudfront.net/uploads/2023/01/Environmental-Assessment-Integrated-2022.pdf.
23. Hemingway J, Beaty BJ, Rowland M, Scott TW, Sharp BL. The innovative vector control consortium: Improved control of mosquito-borne diseases. Trends Parasitol (2006) 22:308–12. doi: 10.1016/j.pt.2006.05.003
24. WHOPES. Guidelines for testing mosquito adulticides for indoor residual spraying and treatment of mosquito nets. Geneva: World Health Organization (2006).
26. CDC. Guideline for evaluating insecticide resistance in vectors using the CDC bottle bioassay. Centers for Disease Control and Prevention (2010). Available at https://www.cdc.gov/malaria/resources/pdf/fsp/ir_manual/ir_cdc_bioassay_en.pdf.
27. WHO. Test procedures for insecticide resistance monitoring in malaria vector mosquitoes. 2nd ed. Geneva: World Health Organisation (2016).
28. WHOPES. Guidelines for laboratory and field testing of long-lasting insecticidal nets. Geneva: World Health Organization (2013).
29. Oxborough RM, N'Guessan R, Jones R, Kitau J, Ngufor C, Malone D, et al. The activity of the pyrrole insecticide chlorfenapyr in mosquito bioassay: towards a more rational testing and screening of non-neurotoxic insecticides for malaria vector control. Malar J (2015) 14:124. doi: 10.1186/s12936-015-0639-x
30. Kweka E, Mahande A, Ouma J, Karanja W, Msangi S, Temba V, et al. Novel indoor residual spray insecticide with extended mortality effect: A case of SumiShield 50WG against wild resistant populations of anopheles arabiensis in northern Tanzania. Glob Health Sci Pract (2018) 6(4):758–65. doi: 10.9745/GHSP-D-18-00213
31. Sreehari U, Raghavendra K, Tiwari SN, Sreedharan S, Ghosh SK, Valecha N. Small-scale (Phase II) evaluation of the efficacy and residual activity of SumiShield((R)) 50 WG (clothianidin 50%, w/w) for indoor residual spraying in comparison to deltamethrin, bendiocarb and pirimiphos-methyl for malaria vector control in karnataka state, India. J Vector Borne Dis (2018) 55(2):122–9. doi: 10.4103/0972-9062.242559
32. Uragayala S, Kamaraju R, Tiwari SN, Sreedharan S, Ghosh SK, Valecha N. Village-scale (Phase III) evaluation of the efficacy and residual activity of SumiShield((R)) 50 WG (Clothianidin 50%, w/w) for indoor spraying for the control of pyrethroid-resistant anopheles culicifacies Giles in karnataka state, India. Trop Med Int Health (2018) 23(6):605–15. doi: 10.1111/tmi.13056
33. Ngwej LM, Hattingh I, Mlambo G, Mashat EM, Kashala JK, Malonga FK, et al. Indoor residual spray bio-efficacy and residual activity of a clothianidin-based formulation (SumiShield((R)) 50WG) provides long persistence on various wall surfaces for malaria control in the democratic republic of the Congo. Malar J (2019) 18(1):72. doi: 10.1186/s12936-019-2710-5
34. Ngufor C, Fongnikin A, Hobbs N, Gbegbo M, Kiki L, Odjo A, et al. Indoor spraying with chlorfenapyr (a pyrrole insecticide) provides residual control of pyrethroid-resistant malaria vectors in southern Benin. Malar J (2020) 19(1):249. doi: 10.1186/s12936-020-03325-2
35. Ngufor C, Critchley J, Fagbohoun J, N'Guessan R, Todjinou D, Rowland M. Chlorfenapyr (A pyrrole insecticide) applied alone or as a mixture with alpha-cypermethrin for indoor residual spraying against pyrethroid resistant anopheles gambiae sl: An experimental hut study in cove, Benin. PloS One (2016) 11(9):e0162210. doi: 10.1371/journal.pone.0162210
36. Ngufor C, N'Guessan R, Boko P, Odjo A, Vigninou E, Asidi A, et al. Combining indoor residual spraying with chlorfenapyr and long-lasting insecticidal bed nets for improved control of pyrethroid-resistant anopheles gambiae: an experimental hut trial in Benin. Malar J (2011) 10:343. doi: 10.1186/1475-2875-10-343
37. Dengela D, Seyoum A, Lucas B, Johns B, George K, Belemvire A, et al. Multi-country assessment of residual bio-efficacy of insecticides used for indoor residual spraying in malaria control on different surface types: results from program monitoring in 17 PMI/USAID-supported IRS countries. Parasites Vectors (2018) 11(1):71. doi: 10.1186/s13071-017-2608-4
38. Mutagahywa J, Ijumba JN, Pratap HB, Molteni F, Mugarula FE, Magesa SM, et al. The impact of different sprayable surfaces on the effectiveness of indoor residual spraying using a micro encapsulated formulation of lambda-cyhalothrin against anopheles gambiae s.s. Parasites Vectors (2015) 8(1):203. doi: 10.1186/s13071-015-0795-4
39. Snetselaar J, Rowland MW, Manunda BJ, Kisengwa EM, Small GJ, Malone DJ, et al. Efficacy of indoor residual spraying with broflanilide (TENEBENAL), a novel meta-diamide insecticide, against pyrethroid-resistant anopheline vectors in northern Tanzania: An experimental hut trial. PloS One (2021) 16(3):e0248026. doi: 10.1371/journal.pone.0248026
40. Azizi S, Snetselaar J, Wright A, Matowo J, Shirima B, Kaaya R, et al. Colonization and authentication of the pyrethroid-resistant anopheles gambiae s.s. muleba-kis strain; An important test system for laboratory screening of new insecticides. Insects (2021) 12(8):710. doi: 10.3390/insects12080710
41. WHO. Test procedures for insecticide resistance monitoring in malaria vector mosquitoes. Geneva: World Health Organization (2013).
42. Lees RS, Ambrose P, Williams J, Morgan J, Praulins G, Ingham VA, et al. Tenebenal: a meta-diamide with potential for use as a novel mode of action insecticide for public health. Malar J (2020) 19(1):398. doi: 10.1186/s12936-020-03466-4
43. Karunarathne P, Pocquet N, Labbe P, Milesi P. BioRssay: an r package for analyses of bioassays and probit graphs. Parasit Vectors (2022) 15(1):35. doi: 10.1186/s13071-021-05146-x
44. Ngufor C, Govoetchan R, Fongnikin A, Vigninou E, Syme T, Akogbeto M, et al. Efficacy of broflanilide (VECTRON T500), a new meta-diamide insecticide, for indoor residual spraying against pyrethroid-resistant malaria vectors. Sci Rep (2021) 11(1):7976. doi: 10.1038/s41598-021-86935-3
45. Williams J, Flood L, Praulins G, Ingham VA, Morgan J, Lees RS, et al. Characterisation of anopheles strains used for laboratory screening of new vector control products. Parasit Vectors (2019) 12(1):522. doi: 10.1186/s13071-019-3774-3
46. Davidson G. Insecticide resistance in anopheles gambiae giles. Nature. (1956) 178(4535):705–6. doi: 10.1038/178705a0
47. Kristensen M, Hansen KK, Jensen K-m. Cross-resistance between dieldrin and fipronil in German cockroach (Dictyoptera: Blattellidae). J Economic Entomol (2005) 98(4):1305–10, 6. doi: 10.1603/0022-0493-98.4.1305
48. Scott JG, Wen Z. Toxicity of fipronil to susceptible and resistant strains of German cockroaches (Dictyoptera: Blattellidae) and house flies (Diptera: Muscidae). J Economic Entomol (1997) 90(5):1152–6. doi: 10.1093/jee/90.5.1152
49. Kolaczinski J, Curtis C. Laboratory evaluation of fipronil, a phenylpyrazole insecticide, against adult anopheles (Diptera: Culicidae) and investigation of its possible cross-resistance with dieldrin in anopheles stephensi. Pest Manag Sci (2001) 57(1):41–5. doi: 10.1002/1526-4998(200101)57:1<41::AID-PS260>3.0.CO;2-N
50. Davari B, Vatandoost H, Oshaghi MA, Ladonni H, Enayati AA, Shaeghi M, et al. Selection of anopheles stephensi with DDT and dieldrin and cross-resistance spectrum to pyrethroids and fipronil. Pesticide Biochem Physiol (2007) 89(2):97–103. doi: 10.1016/j.pestbp.2007.04.003
51. Nakao T, Banba S, Nomura M, Hirase K. Meta-diamide insecticides acting on distinct sites of RDL GABA receptor from those for conventional noncompetitive antagonists. Insect Biochem Mol Biol (2013) 43(4):366–75. doi: 10.1016/j.ibmb.2013.02.002
52. Portwood NM, Shayo MF, Tungu PK, Mbewe NJ, Mlay G, Small G, et al. Multi-centre discriminating concentration determination of broflanilide and potential for cross-resistance to other public health insecticides in anopheles vector populations. Sci Rep (2022) 12(1):22359. doi: 10.1038/s41598-022-26990-6
53. Lees R, Praulins G, Davies R, Brown F, Parsons G, White A, et al. A testing cascade to identify repurposed insecticides for next-generation vector control tools: screening a panel of chemistries with novel modes of action against a malaria vector. Gates Open Res (2019) 3:1464. doi: 10.12688/gatesopenres.12957.2
54. Govoetchan R, Fongnikin A, Syme T, Small G, Gbegbo M, Todjinou D, et al. VECTRON™ T500, a new broflanilide insecticide for indoor residual spraying, provides prolonged control of pyrethroid-resistant malaria vectors. Malaria J (2022) 21(1):324. doi: 10.1186/s12936-022-04336-x
55. Agossa FR, Padonou GG, Koukpo CZ, Zola-Sahossi J, Azondekon R, Akuoko OK, et al. Efficacy of a novel mode of action of an indoor residual spraying product, SumiShield® 50WG against susceptible and resistant populations of anopheles gambiae (sl) in Benin, West Africa. Parasites vectors (2018) 11(1):293. doi: 10.1186/s13071-018-2869-6
56. Kamaraju R, Pant CS, Uragayala S, Baharia RK, Srivastava HC, Yadav RS. Small-scale field evaluation of the entomological efficacy and the residual activity of Fludora((R)) fusion WP-SB indoor residual spraying against anopheles culicifacies s.l. in Gujarat, India. Trop Med Int Health (2021) 26(4):469–77. doi: 10.1111/tmi.13549
57. Fuseini G, Phiri WP, von Fricken ME, Smith J, Garcia GA. Evaluation of the residual effectiveness of fludora fusion WP-SB, a combination of clothianidin and deltamethrin, for the control of pyrethroid-resistant malaria vectors on bioko island, equatorial Guinea. Acta Trop (2019) 196:42–7. doi: 10.1016/j.actatropica.2019.05.006
58. Agossa FR, Padonou GG, Fassinou A, Odjo EM, Akuoko OK, Salako A, et al. Small-scale field evaluation of the efficacy and residual effect of Fludora((R)) fusion (mixture of clothianidin and deltamethrin) against susceptible and resistant anopheles gambiae populations from Benin, West Africa. Malar J (2018) 17(1):484. doi: 10.1186/s12936-018-2633-6
59. Fongnikin A, Houeto N, Agbevo A, Odjo A, Syme T, N'Guessan R, et al. Efficacy of Fludora(R) fusion (a mixture of deltamethrin and clothianidin) for indoor residual spraying against pyrethroid-resistant malaria vectors: laboratory and experimental hut evaluation. Parasit Vectors (2020) 13(1):466. doi: 10.1186/s13071-020-04341-6
60. Etang J, Nwane P, Mbida JA, Piameu M, Manga B, Souop D, et al. Variations of insecticide residual bio-efficacy on different types of walls: results from a community-based trial in south Cameroon. Malar J (2011) 10:333. doi: 10.1186/1475-2875-10-333
61. Yewhalaw D, Balkew M, Shililu J, Suleman S, Getachew A, Ashenbo G, et al. Determination of the residual efficacy of carbamate and organophosphate insecticides used for indoor residual spraying for malaria control in Ethiopia. Malar J (2017) 16(1):471. doi: 10.1186/s12936-017-2122-3
62. Tangena JA, Adiamoh M, D'Alessandro U, Jarju L, Jawara M, Jeffries D, et al. Alternative treatments for indoor residual spraying for malaria control in a village with pyrethroid- and DDT-resistant vectors in the Gambia. PloS One (2013) 8(9):e74351. doi: 10.1371/journal.pone.0074351
63. Bayili K, Ki HD, Bayili B, Sow B, Ouattara A, Small G, et al. Laboratory and experimental hut trial evaluation of VECTRON T500 for indoor residual spraying (IRS) against insecticide resistant malaria vectors in Burkina Faso. Gates Open Res (2022) 6:57. doi: 10.12688/gatesopenres.13578.2
64. IVCC. Indoor residual spray - target product profile. Available at: https://www.ivcc.com/research-development/target-product-profiles-tpps/.
Keywords: Anopheles gambiae, indoor residual spraying, broflanilide, TENEBENALTM, cross resistance, residual efficacy
Citation: Snetselaar J, Rowland MW, Azizi S, Mawa B, Malone DJ and Kirby MJ (2023) Laboratory evaluation of broflanilide (TENEBENAL™) against Anopheles gambiae in Moshi, Tanzania – delayed mortality, cross-resistance, and residual efficacy. Front. Trop. Dis 4:1097189. doi: 10.3389/fitd.2023.1097189
Received: 13 November 2022; Accepted: 20 February 2023;
Published: 21 March 2023.
Edited by:
David P. Tchouassi, International Centre of Insect Physiology and Ecology (ICIPE), KenyaReviewed by:
Cyrille Ndo, Centre for Research in Infectious Diseases (CRID), CameroonKingsley Badu, Kwame Nkrumah University of Science and Technology, Ghana
Copyright © 2023 Snetselaar, Rowland, Azizi, Mawa, Malone and Kirby. This is an open-access article distributed under the terms of the Creative Commons Attribution License (CC BY). The use, distribution or reproduction in other forums is permitted, provided the original author(s) and the copyright owner(s) are credited and that the original publication in this journal is cited, in accordance with accepted academic practice. No use, distribution or reproduction is permitted which does not comply with these terms.
*Correspondence: Janneke Snetselaar, SmFubmVrZS5TbmV0c2VsYWFyQGxzaHRtLmFjLnVr