- Medical Entomology Laboratory, National Institute of Public Health, Praia, Cape Verde
Introduction: The transmission of pathogens by blood-sucking insects to humans and other animals depends on vector-host interactions. As yet unexplored in Cape Verde, mosquito feeding behavior plays a crucial role in pathogen transmission. Herein, we aim to explore, through blood meal analysis, the relationship between mosquito species and common hosts in Santiago Island, Cape Verde.
Methods: Engorged female mosquitoes were collected through mechanical aspiration from May 2016 to December 2017 in three municipalities of Santiago Island (Praia, Santa Cruz, and Santa Catarina). Blood-feeding behavior in each municipality was assessed through blood meal analysis using an enzyme-linked immunosorbent assay (ELISA).
Results: We were able to determine that single-host blood meals were common in Aedes aegypti, Anopheles arabiensis, and Culex pipiens sensu lato (s.l.). In general, the mosquitoes preferred to feed on humans, dogs, and chickens, and on multiple hosts, mainly two hosts. The human blood index (HBI) was highest (i.e., 1.00) in Ae. aegypti, with the lowest value (0.40) observed in An. arabiensis. It was observed that, among single-host blood meals, the likelihood of Cx. pipiens s.l. feeding on humans was significantly high, whereas the likelihood of An. arabiensis feeding on humans was significantly low (log-odds ratio (LOR) = 0.85 and –2.44, respectively). In addition, a high likelihood of Ae. aegypti feeding on humans was observed, but this was not statistically significant (LOR = 0.85).
Discussion: Overall, our findings demonstrate a lack of feeding preference in Culex pipiens s.l. compared with Ae. aegypti and An. arabiensis. These results provide insights into possible parasite transmission and pathogen spillover/spillback, which threaten human/animal health and the economy in Cape Verde.
Introduction
Vector–host interactions play a key role in pathogen transmission. More than half of the world’s population lives in areas at risk of one or more major vector-borne diseases, with mosquito-borne diseases being a major contributor (1). Mosquitoes are known to be vectors of diseases that are devastating to humans, domestic animals, and wildlife (2).
Hematophagy is an obligatory behavior for most female mosquitoes in order to reproduce and to obtain energy (3). This process is facilitated through catheterization of the skin (solenophagy), as a result of which pathogens are ingested and transmitted. Orientation toward a host is paramount for feeding success, and in the case of temporary ectoparasites, such as mosquitoes, host choice is underpinned by single or multiple factors, such as host availability (4–8), abundance (8–11), season shifting (4, 12), natural host defense (particularly in Aves) (13–17), host size (18–21), odor (22–24), and ecology (8, 25) leading to catholic tastes in a single gonotrophic cycle, which may favor parasite transmission.
The ability to precisely identify the source of blood meal in mosquitoes and to distinguish anthropophilic and zoophilic species is of prime importance for deciphering host choice and how parasites manipulate the host to promote infection and transmission, and for understanding disease epidemiology for improved entomological surveillance (2, 26–29).
Mosquito-borne pathogens, including yellow fever virus (30), Wuchereria bancrofti (31), Zika virus (32, 33), dengue virus (34), Plasmodium spp (35). Dirofilaria immitis (36), and Dirofilaria repens (37), which are of public health importance, have been reported in Cape Verde. To date, 11 species of mosquito have been described in Cape Verde, of which Anopheles arabiensis, Aedes aegypti, Culex pipiens sensu stricto (s.s.), and Culex quinquefasciatus are the most important (38–40).
Nevertheless, mosquito bites and diseases may be preventable by decreasing contact with humans through zooprophylaxis, by the use of repellant air-sprays and house improvements, and, at an individual level, by covering the skin and using insecticide-treated bed nets (1, 6, 23, 41–47). Thus, integrated vector management (IVM) with multiple strategies, included as part of the One Health Initiative, has greater potential to improve vector-borne disease control and facilitate elimination than isolated or routine approaches (48, 49). Understanding the temporal and spatial dynamics of the vector–host relationships is a key element in planning IVM.
Entomological studies in Cape Verde have focused more on mosquito density, distribution, and genetic and phenotypic composition than on feeding behaviors, although these are crucial for disease transmission. In our study, we investigated the blood feeding patterns of mosquitoes in three municipalities in Santiago Island: Praia, Santa Cruz, and Santa Catarina.
Materials and methods
Ethics statement
All mosquito collections were carried out in private spaces (i.e., homes and their vicinity). We sought consent from owners before collecting the mosquitoes on their properties. The field collections did not involve any endangered or protected species. Materials used in the study posed no health risk to researchers or owners, and no vertebrate animals were harmed.
Sampling sites and collection
As part of vector surveillance activities, led by the National Institute of Public Health, Cape Verde, mosquitoes were collected from May 2016 to December 2017 in randomly selected areas in three municipalities of Santiago Island [Praia (12 areas), Santa Cruz (four areas), and Santa Catarina (two areas)] (Figure 1). The selection was based on the history of mosquito-borne diseases, and mosquito and vertebrate composition. Both outdoor and indoor collections were carried out in the morning (7 to 11 a.m.). At each sampling site, mosquitoes were collected through 15 minutes of mechanical aspiration using a modified Centers for Disease Control and Prevention (CDC) backpack aspirator (model 1412; John W. Hock Company, Gainesville, FL, USA). All collected mosquitoes were transported alive in cups to the Medical Entomology Laboratory at the National Institute of Public Health, Cape Verde, and then killed by freezing.
Mosquito species identification
Mosquito specimens were separated according to blood digestion status (i.e., blood fed and unfed) following Reeves et al.’s recommendations (50). Only blood-fed mosquitoes were morphologically identified using dichotomous keys (39, 51). Therefore, mosquitoes with fresh blood and with an intermediary blood digestion status were used for the blood meal analysis. Each mosquito was separated into head, thorax, and abdomen. The abdomens were crushed on Whatman grade 1 qualitative filter paper (GE HealthCare Technologies Inc., Chicago, IL, USA) and preserved at –20°C for enzyme-linked immunosorbent assay (ELISA) analysis. The heads and thoraxes were individually preserved in silica gel (Merck KGaA, Darmstadt, Germany) for DNA extraction. Mosquitoes morphologically identified as Anopheles gambiae sensu lato (s.l.) were submitted to a single total DNA extraction using the NZY Tissue gDNA Isolation Kit (NZYTech genes & enzymes, Lisbon, Portugal). Sibling species of the An. gambiae complex were identified using PCR following the protocol described by Scott et al. (52).
Blood meal identification
Blood meal sources were identified through a direct ELISA adapted from Lardeaux et al. (53). Immunoglobulin G (IgG) from five vertebrate hosts of interest (human, pig, dog, goat, and chicken) was assessed. The choice of alternatives to human hosts was based on observations made during field collections at the sites. The absorbance values were obtained using an absorbance microplate reader (Stat Fax® 4200) at 450 nm. The cut-off values for each plate were calculated from the mean of three negative controls chosen randomly, plus three times their standard deviation (SD) [i.e., cut-off = mean + (3×SD)].
Statistical analysis
The human blood index (HBI) was estimated for each group of mosquitoes from each municipality in accordance with the Garrett-Jones formula (54). The probabilities of single-host blood meals on humans for each mosquito species, by municipality, and their corresponding 95% confidence intervals (CIs), were calculated. In addition, as proposed elsewhere (55), log-odds ratios (LORs) were calculated from hypergeometrically distributed data on a 2 × 2 contingency table (95% confidence interval) between each mosquito species and host (where positive and negative LORs indicate, respectively, a positive and negative feeding association, meaning that the likelihood that a blood meal of that mosquito species would originate from a given host is, respectively, higher and a lower than random chance). The greater the LOR, the stronger the feeding association. LORs close to 0 suggest no association between the mosquito species and host. In addition, RStudio version 1.4.1717 (packages gglot2, ggdendrogram, and reshape2) was used to generate a heatmap of the LORs in hierarchical clustering, which assembled mosquito species with similar feeding patterns. The percentages of blood meals by host according to season, municipality, and land use were represented graphically using JMP Pro 16.1.0.
Results
A total of 1,008 mosquito specimens freshly blood fed and with intermediary blood digestion were analyzed from the three municipalities of Santiago Island: Praia (n = 834; 83%), Santa Cruz (n = 127; 13%), and Santa Catarina (n = 47; 5%). The majority of these, 860 specimens, were Cx. pipiens s.l. (85%), followed by Ae. aegypti (n = 103; 10%) and An. arabiensis (n = 45; 4%) (Table 1). No engorged An. arabiensis or Ae. aegypti were caught in Santa Catarina.
Single-host blood meals were more common [Ae.aegypti (84.3%), An. arabiensis (64.7%), and Cx. pipiens s.l. (81.6%)] than multiple-host blood meals [Ae. aegypti (15.7%), An. arabiensis (35.3%), and Cx. pipiens s.l. (18.4%)]. Single-host blood meals were mainly represented by human blood (56 for Ae. Aegypti, nine for An. arabiensis, and 581 for Cx. pipiens s.l.). Regarding multiple-host blood meals, the mosquitoes fed on human and other animal hosts (18 for Ae. Aegypti, 15 for An. Arabiensis, and 159 for Cx. pipiens s.l.). Following humans, dogs were the most frequent host among Cx. pipiens s.l. (represented 116 times) (Table 1).
As regards the HBI, the lowest HBI (0.40) was recorded in An. arabiensis, whereas Ae. aegypti from the municipality of Santa Cruz had the highest HBI (1.00). The overall average HBIs in the Santiago Island were 0.98, 0.55, and 0.92 for Ae. aegypti, An. arabiensis, and Cx. pipiens s.l., respectively (Table 1).
The calculated probabilities of mosquito species taking a single-host blood meal from humans indicated that, for Cx. pipiens s.l., the probability was lowest in Santa Catarina (HBI = 0.88; 95% CI 0.71 to 0.96) and highest in Santa Cruz (HBI= 0.93; 95% CI 0.84 to 0.97). Whereas for An. arabiensis the chance was lower (HBI = 0.40; 95% CI 0.07 to 0.83) but higher (HBI = 1.00; 95% CI 0.63 to 1.00) for Ae. aegypti in the Santa Cruz municipality (Table 2).
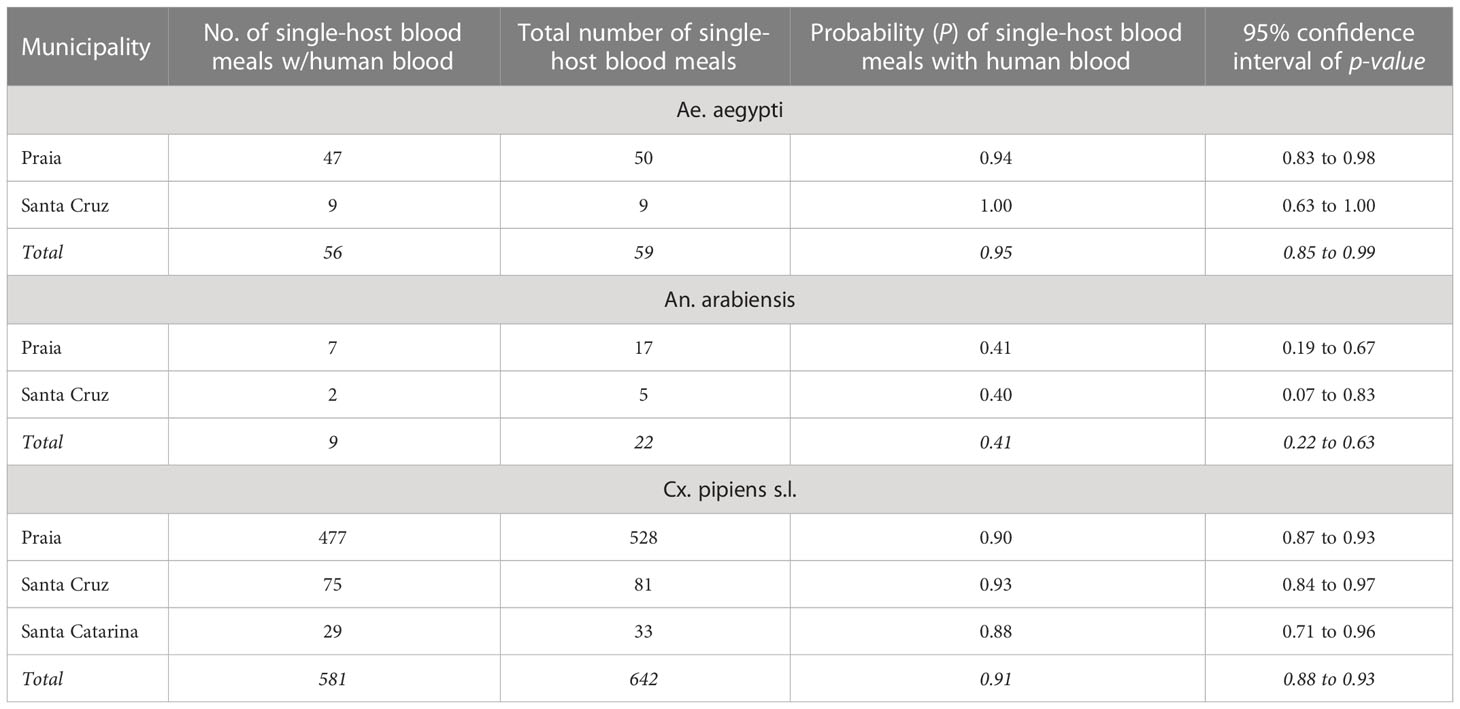
Table 2 Probability of Aedes aegypti, Anopheles arabiensis, and Culex pipiens s.l. from each municipality of Santiago Island taking a single-host blood meal from a human host.
Only An. arabiensis took single-host blood meals from all five hosts (pigs, humans, goats, dogs, and chickens) especially in urban areas like Praia during the rainy season (Figure 2). Cx. pipiens s.l. had a higher preference for human blood, followed by chicken, and this preference did not differ across seasons, municipalities, or land uses (Figure 3). Ae. aegypti also preferred to feed on humans but this preference did not differ across seasons and was not different in Praia and Santa Cruz, the two municipalities where it was possible to capture this mosquito species (Figure 4).
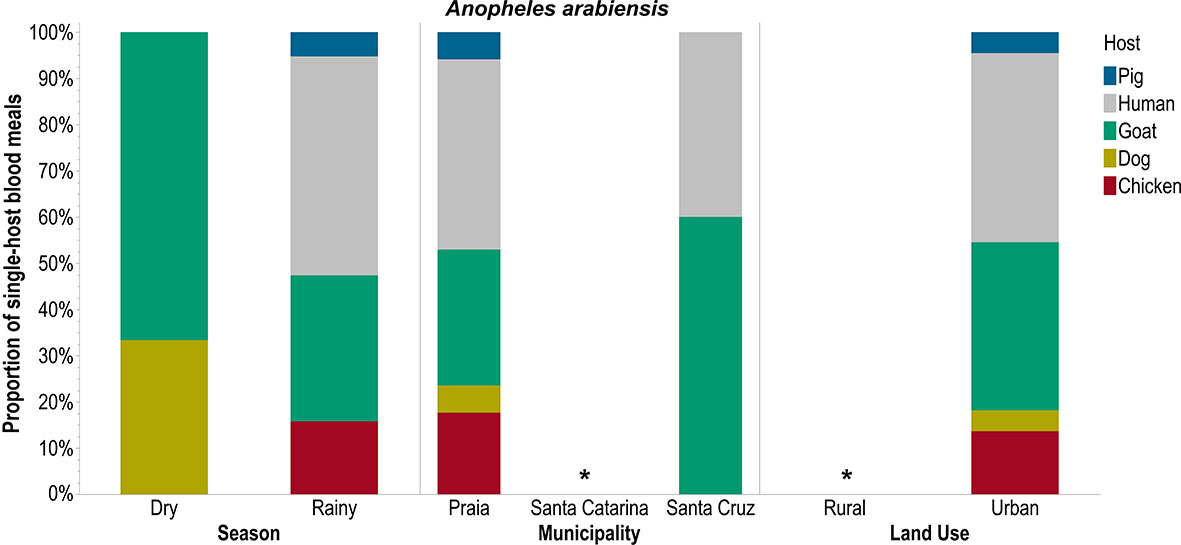
Figure 2 Single-host blood meals by Anopheles arabiensis according to season, municipality, and land use. *no mosquitoes captured.
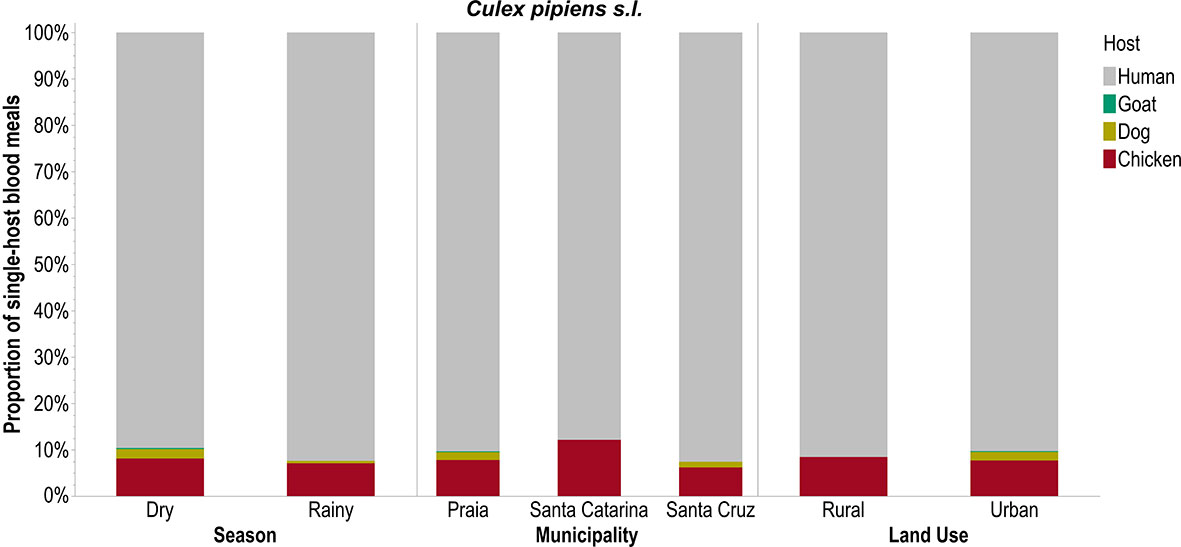
Figure 3 Single-host blood meals by Culex pipiens s.l. according to season, municipality, and land use.
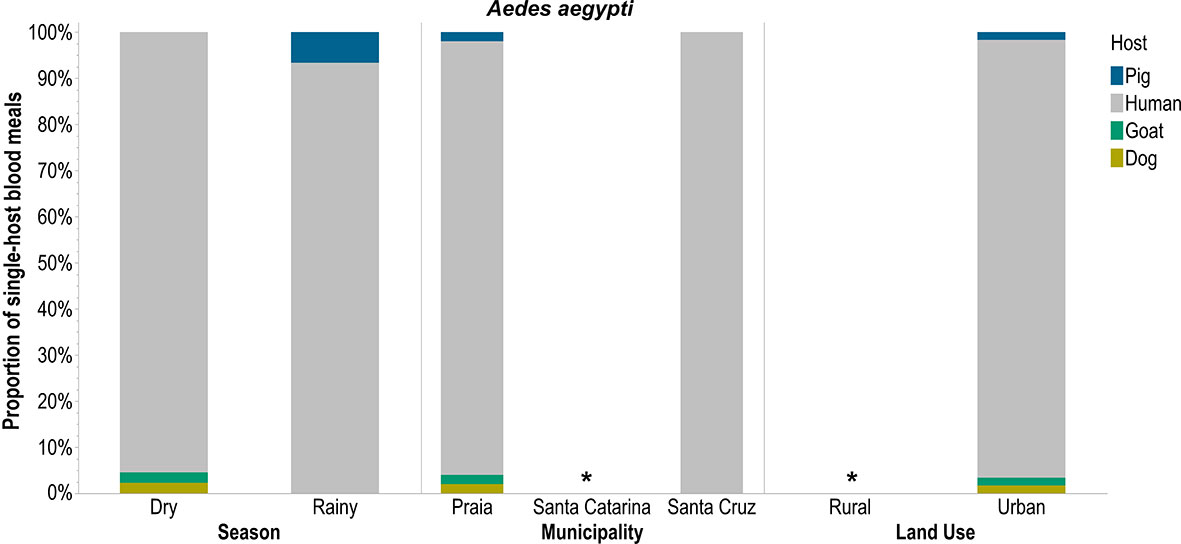
Figure 4 Single-host blood meals by Aedes aegypti according to season, municipality, and land use. *no mosquito captured.
The LOR calculated only for single-host blood meals clustered together Cx. pipiens s.l. and Ae. aegypti with similar feeding patterns. These estimations revealed positive LORs for all the mosquito–host relationships; however, the Cx. pipiens s.l.–dogs and Cx. pipiens s.l.–goats relationships show negative LORs, i.e., negative associations. This is similar to An. arabiensis–human host (Figure 5).
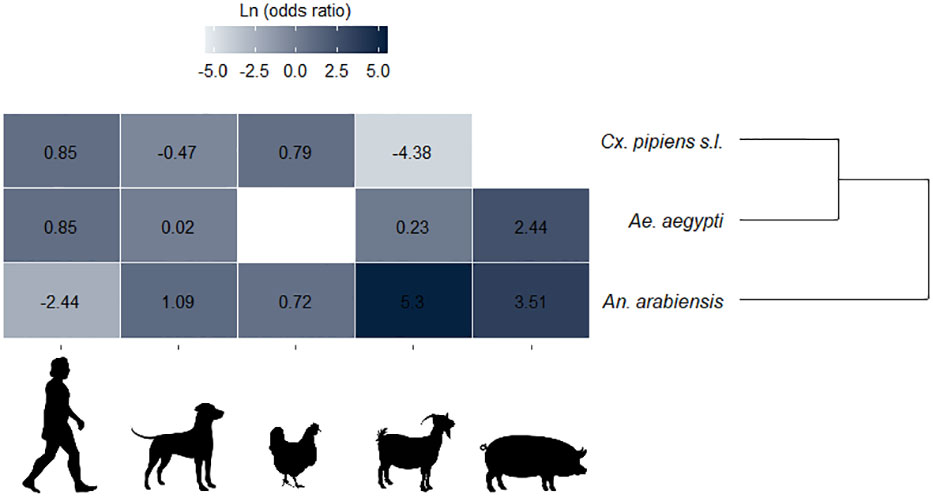
Figure 5 Single-host blood meals associations between hosts (humans, dogs, chickens, goats, and pigs) and mosquito species. Mosquito species were arranged by hierarchal clustering according to the likeness.
Significant positive or negative associations were found for two out of the five hosts (humans and goats). There was a significant clustering positive LOR (LOR = 0.85, p = 0.01) for Cx. pipiens s.l. for human hosts and a strong negative association for goats (LOR = –4.38, p < 0.0001), meaning a higher and a lower likelihood of this mosquito species taking blood from humans and goats, respectively. Similarly, positive associations between Ae. aegypti and humans (LOR = 0.85, p = 0.10) and between Ae. aegypti and pigs (LOR = 2.44, p = 0.16) were observed, but these were not statistically significant. For An. arabiensis–humans a significant strong negative correlation (LOR = –2.44, p < 0.0001) was observed, which points to a lower likelihood of this mosquito species biting humans. A strong positive association was observed between An. arabiensis and goats (LOR = 5.30, p = 7.80) and between An. arabiensis and pigs (LOR = 3.51, p = 0.06), but these associations were not statistically significant. An LOR value closer to 0 was found only for Ae. Aegypti–dogs, which suggests no relationship (LOR = 0.02, p = 0.64) (Figure 5).
Discussion
The transmission potential of a vector-borne pathogen by a competent vector is influenced to a large extent by blood feeding behavior, vector population density, and longevity (56). In our study, data gathered from multiple sites indicate that the major pathogen vectors (i.e., An. arabiensis, Ae. aegypti, and Cx. pipiens s.l.) share up to three hosts, although this may be influenced by host availability rather than by species-specific host choices. A limitation at this point is the fact that our data could be more accurate if PCR-based molecular approaches were used. Despite Praia being more urbanized, multiple-host blood meals (from four or five hosts) were also observed (Table 1). This observation is not new in mosquitoes, as shown in studies in Brazil, Honduras, and Peru (57–60).
A pertinent result that we found was the generic feeding behavior among An. arabiensis and Cx. pipiens s.l., in contrast to Ae. aegypti, which showed a specific feeding behavior with a strong predilection for human hosts (HBIsingle-host + multiple-host blood meals = 0.98; HBIsingle-host blood meals = 0.95). Work carried out in Grenada, the USA, Thailand, Puerto Rico, and Australia also found similar feeding behaviors in Ae. aegypti (61–67), as humans provide reproductive and metabolic advantages (68). This feeding behavior could be one of various factors that would explain the large number of cases of dengue fever in 2009 (34) and of Zika in 2015 (32) in Cape Verde, and this is also a point of consideration, as Ae. aegypti from Cape Verde is also able to transmit the Chikungunya virus (69).
Few (n = 45) blood-fed An. arabiensis were caught, which limits conclusions on any blood meal analyses. These low numbers could be due to the collection technique employed (70), as we collected only mosquitoes that were within range of the suction effect of the aspirator. It is likely that the use of a collecting tool with wider coverage, such as pyrethrum spray catches, would have increased the numbers collected. In any case, in our study, An. arabiensis showed low anthropophily [HBIsingle-host + multiple-host blood meals = 0.55; HBIsingle-host blood meals = 0.41(LOR = –2.44, p < 0.0001)], similar to the findings of studies in Burkina Faso, Kenya, and Ethiopia (71–73). We verified that An. Arabiensis fed from multiple hosts in the same gonotrophic cycle (from two to four hosts), which agrees with the gonotrophic discordance phenomenon previously described in other species of this genus in Ethiopia, Brazil, Kenya, Mexico, and Sri Lanka (73–78). The low anthropophily observed in this species could also explain the relatively low prevalence of malaria in Cape Verde, in contrast to other countries in Africa, where the main malaria vector, An. gambiae s.s., is, essentially, anthropophilic (79).
Although there have been reports of D. immitis and D. repens among dogs in Cape Verde (80–87), and the West Nile virus and W. bancrofti in other African countries (31, 88, 89), Cx. Pipiens s.l. has not been implicated in any human disease in Cape Verde. Two species of the Cx. pipiens complex, Cx. pipiens s.s. (ornithophilic) and Cx. quinquefasciatus (anthropophilic), and their hybrids, have been observed in Cape Verde (90). Both species are more eclectic than An. Arabiensis and Ae. Aegypti. In our study Cx. pipiens s.l. showed a preference for human blood meals (HBIsingle-host + multiple-host blood meals = 0.92; HBIsingle-host blood meals = 0.91 [LOR = 0.85, p = 0.01]) followed by blood meals in chickens and dogs. Similar trends have been found in Grenada, the USA, Ecuador, India, Brazil, Germany, Australia, and Kenya (61, 62, 91–98).
The mosquito feeding behavior observed in our study demonstrates that it is important to consider the closeness of dogs to humans and their role in parasite transmission. Although humans are dead-end hosts for D. immitis and D. repens (both found in dogs in Cape Verde), earlier stages can cause dirofilariasis and inflammatory response when parasites die in human tissues (99). Ocular and pulmonary dirofilariasis with benign pulmonary and subcutaneous nodules that may be confused with cancer can also be found in humans (99–102). In addition to Cx. pipiens s.l., Ae. aegypti has also been implicated in the transmission of both parasites, although we observed a low probability of this species biting dogs (Table 1, Figure 5) (87, 103, 104).
It is possible that our results could be a reflection more of host availability than of mosquito host preference: the high numbers of Cx. pipiens s.l. and Ae. aegypti individuals collected and their feeding behaviors (on both humans and domestic animals), as reported in this study, call for further investigations into parasites circulating in these mosquitoes and also in dogs and humans in Cape Verde. For situations where humans would behave as amplifying hosts of other dog parasites not mentioned here, from our results of simultaneous blood meals in dogs and humans, we may have an even greater amplification event due to interaction augmentation between these two hosts, which increases as their abundance also increases (105). However, as pointed out elsewhere (18, 106, 107), it is necessary to consider the specific attributes to such interactions.
Future directions from this study should therefore consider on combining data from mosquito and animal densities and richness to explore transmission risk according to season and land use. In addition, considering a larger panel of hosts, including non-domestic animals, and increasing sampling efforts with proper sampling techniques, will ensure enough representation of samples for biological relevance of data generated. Another limitation is that we did not record the total number of collected mosquitoes. Therefore, we cannot provide the percentage of blood-fed mosquitoes for our field study.
In conclusion, our study provides the first characterization of blood sources utilized by medically important mosquitoes in Santiago Island, Cape Verde. Importantly, this work has shown that feeding behavior among Cx. pipiens s.l. is more random than that demonstrated by Ae. aegypti and An. arabiensis. Our findings also provide interesting information on disease transmission between hosts and pathogens spillover or spillback phenomena. These phenomena, despite the fact that they have not yet been thoroughly investigated in the country, could threaten livestock, poultry farming, wildlife health, and the economy, and this may be even more important in view of climate change. Thus, inclusion of the One Health Initiative (involving animals) in disease surveillance could help track diseases of humans with animal ancestry and zoonosis for planning interventions. Interventions such as zooprophylaxis, in which animals are treated with mosquito-killing drugs, and mosquito bites are redirected can greatly reduce mosquito survival and lower their potential to transmit pathogens.
Data availability statement
The original contributions presented in the study are included in the article/Supplementary Material. Further enquiries can be directed to the corresponding author.
Author contributions
AG and SL conceived and directed the study. AG, DM, IV, and AD performed species identification. AG, DM, IV, and AD undertook sample processing, DNA extraction, and molecular assays. AG and AD performed data analyses. AG and AD interpreted results. AG wrote the first draft of the manuscript with inputs from SL and AD. All authors commented on and edited the draft manuscript and approved the final manuscript. AG and SL compiled the final manuscript. All authors contributed to the article and approved the submitted version.
Funding
This work was supported by the National Institute of Public Health, Cape Verde.
Acknowledgments
The authors thank the National Institute of Public Health, Cape Verde, for supporting the study; Jonas Gomes, from the National Observatory of Health (National Institute of Public Health), for the map drawing; and the health delegations from Santa Catarina and Santa Cruz, in particular anti-vectorial health agents, for their support in mosquito collection.
Conflict of interest
The authors declare that the research was conducted in the absence of any commercial or financial relationships that could be construed as a potential conflict of interest.
Publisher’s note
All claims expressed in this article are solely those of the authors and do not necessarily represent those of their affiliated organizations, or those of the publisher, the editors and the reviewers. Any product that may be evaluated in this article, or claim that may be made by its manufacturer, is not guaranteed or endorsed by the publisher.
References
1. World Health Organization. Global vector control response 2017-2030 (2017) (Accessed November 3, 2021).
2. Lehane MJ. The biology of blood-sucking in insects. 2 ed. Cambridge: Cambridge University Press (2005).
3. Wheeler D. The role of nourishment in oogenesis. Annu Rev Entomol (1996) 41:407–31. doi: 10.1146/annurev.en.41.010196.002203
4. Chandler JA, Parsons J, Boreham PF, Gill GS. Seasonal variations in the proportions of mosquitoes feeding on mammals and birds at a heronry in western kenya. J Med Entomol (1977) 14(2):233–40. doi: 10.1093/jmedent/14.2.233
5. Corbet PS. Facultative autogeny in Arctic mosquitoes. Nature (1967) 215(5101):662–3. doi: 10.1038/215662a0
6. Mayagaya VS, Nkwengulila G, Lyimo IN, Kihonda J, Mtambala H, Ngonyani H, et al. The impact of livestock on the abundance, resting behaviour and sporozoite rate of malaria vectors in southern tanzania. Malar J (2015) 14:17. doi: 10.1186/s12936-014-0536-8
7. Mwangangi JM, Mbogo CM, Nzovu JG, Githure JI, Yan G, Beier JC. Blood-meal analysis for anopheline mosquitoes sampled along the Kenyan coast. J Am Mosq Control Assoc (2003) 19(4):371–5.
8. Sousa CA, Pinto J, Almeida AP, Ferreira C, do Rosário VE, Charlwood JD. Dogs as a favored host choice of Anopheles gambiae sensu stricto (Diptera: Culicidae) of são tomé West africa. J Med Entomol (2001) 38(1):122–5. doi: 10.1603/0022-2585-38.1.12
9. Beier JC, Odago WO, Onyango FK, Asiago CM, Koech DK, Roberts CR. Relative abundance and blood feeding behavior of nocturnally active culicine mosquitoes in western kenya. J Am Mosq Control Assoc (1990) 6(2):207–12.
10. Hernandez-Colina A, Gonzalez-Olvera M, Lomax E, Townsend F, Maddox A, Hesson JC, et al. Blood-feeding ecology of mosquitoes in two zoological gardens in the united kingdom. Parasit Vectors (2021) 14(1):249. doi: 10.1186/s13071-021-04735-0
11. Petrarca V, Beier JC, Onyango F, Koros J, Asiago C, Koech DK, et al. Species composition of the Anopheles gambiae complex (diptera: Culicidae) at two sites in western kenya. J Med Entomol (1991) 28(3):307–13. doi: 10.1093/jmedent/28.3.307
12. Kilpatrick AM, Kramer LD, Jones MJ, Marra PP, Daszak P. West Nile virus epidemics in north America are driven by shifts in mosquito feeding behavior. PloS Biol (2006) 4(4):e82. doi: 10.1371/journal.pbio.0040082
13. Darbro JM, Harrington LC. Avian defensive behavior and blood-feeding success of the West Nile vector mosquito, Culex pipiens. Behav Ecol (2007) 18(4):750–7. doi: 10.1093/beheco/arm043
14. Edman JD, Kale HW II. Host behavior: Its influence on the feeding success of mosquitoes. Ann Entomol Soc Am (1971) 64(2):513–6. doi: 10.1093/aesa/64.2.513
15. Edman JD, Webber LA, Schmid AA. Effect of host defenses on the feeding pattern of Culex nigripalpus when offered a choice of blood sources. J Parasitol (1974) 60(5):874–83. doi: 10.2307/3278923
16. Walker ED, Edman JD. The influence of host defensive behavior on mosquito (Diptera: Culicidae) biting persistence. J Med Entomol (1985) 22(4):370–2. doi: 10.1093/jmedent/22.4.370
17. Webber LA, Edman JD. Anti-mosquito behaviour of ciconiiform birds. Anim Behav (1972) 20(2):228–32. doi: 10.1016/S0003-3472(72)80040-X
18. Harrington LC, Fleisher A, Ruiz-Moreno D, Vermeylen F, Wa CV, Poulson RL, et al. Heterogeneous feeding patterns of the dengue vector, Aedes aegypti, on individual human hosts in rural thailand. PloS Negl Trop Dis (2014) 8(8):e3048. doi: 10.1371/journal.pntd.0003048
19. Kweka EJ, Mwang'onde BJ, Lyaruu L, Tenu F, Mahande AM. Effect of different hosts on feeding patterns and mortality of mosquitoes (Diptera: Culicidae) and their implications on parasite transmission. J Glob Infect Dis (2010) 2(2):121–3. doi: 10.4103/0974-777X.62873
20. Liebman KA, Stoddard ST, Reiner RC Jr, Perkins TA, Astete H, Sihuincha M, et al. Determinants of heterogeneous blood feeding patterns by Aedes aegypti in iquitos, peru. PloS Negl Trop Dis (2014) 8(2):e2702. doi: 10.1371/journal.pntd.0002702
21. Port GR, Boreham PFL, Bryan JH. The relationship of host size to feeding by mosquitoes of the Anopheles gambiae Giles complex (Diptera: Culicidae). Bull Entomol Res (1980) 70(1):133–44. doi: 10.1017/S0007485300009834
22. Allan SA, Bernier UR, Kline DL. Laboratory evaluation of avian odors for mosquito (Diptera: Culicidae) attraction. J Med Entomol (2006) 43(2):225–31. doi: 10.1603/0022-2585(2006)043[0225:leoaof]2.0.co;2
23. Mahande A, Mosha F, Mahande J, Kweka E. Feeding and resting behaviour of malaria vector, Anopheles arabiensis with reference to zooprophylaxis. Malar J (2007) 6:100. doi: 10.1186/1475-2875-6-100
24. Syed Z, Leal WS. Acute olfactory response of culex mosquitoes to a human- and bird-derived attractant. Proc Natl Acad Sci U S A (2009) 106(44):18803–8. doi: 10.1073/pnas.0906932106
25. Loyola EG, González-Cerón L, Rodríguez MH, Arredondo-Jiménez JI, Bennett S, Bown DN. Anopheles albimanus (Diptera: Culicidae) host selection patterns in three ecological areas of the coastal plains of chiapas, southern mexico. J Med Entomol (1993) 30(3):518–23. doi: 10.1093/jmedent/30.3.518
26. Flores-Mendoza C, Cunha RA, Rocha DS, Lourenço-de-Oliveira R. Determinação das fontes alimentares de Anopheles aquasalis (Diptera: Culicidae) no estado do Rio de Janeiro, brasil, pelo teste de precipitina [Identification of food sources of Anopheles aquasalis (Diptera: Culicidae) by precipitin test in the state of Rio de Janeiro, brazil]. Rev Saude Publica (1996) 30(2):129–34. doi: 10.1590/s0034-89101996000200003
27. Koella JC, Sørensen FL, Anderson RA. The malaria parasite, Plasmodium falciparum, increases the frequency of multiple feeding of its mosquito vector, Anopheles gambiae. Proc Biol Sci (1998) 265(1398):763–8. doi: 10.1098/rspb.1998.0358
28. Marassá AM, Consales CA, Galati EAB. Padronização da técnica imunoenzimática do ELISA de captura, no sistema avidina-biotina para a identificação de sangue ingerido por Lutzomyia (Lutzomyia) longipalpis (Lutz & neiva, 1912). Rev Soc Bras Med Trop (2004) 37:441–6. doi: 10.1590/S0037-86822004000600003
29. Mukabana WR, Takken W, Coe R, Knols BG. Host-specific cues cause differential attractiveness of Kenyan men to the African malaria vector Anopheles gambiae. Malar J (2002) 1:17. doi: 10.1186/1475-2875-1-17
30. Patterson KD. Epidemics, famines, and population in the cape Verde islands, 1580-1900. Int J Afr Hist Stud (1988) 21(2):291–313. doi: 10.2307/219938
31. World Health Organization. Global programme to eliminate lymphatic filariasis: Progress report on mass drug administration in 2008 (2009) (Accessed January 16, 2022).
32. Lourenço J, de Lourdes Monteiro M, Valdez T, Monteiro Rodrigues J, Pybus O, Rodrigues Faria N. Epidemiology of the zika virus outbreak in the Cape Verde islands, West africa. PloS Curr (2018) 10. doi: 10.1371/currents.outbreaks.19433b1e4d007451c691f138e1e67e8c
33. Campos M, Ward D, Morales RF, Gomes AR, Silva K, Sepúlveda N, et al. Surveillance of Aedes aegypti populations in the city of Praia, cape Verde: Zika virus infection, insecticide resistance and genetic diversity. Parasit Vectors (2020) 13(1):481. doi: 10.1186/s13071-020-04356-z
34. World Health Organization. OUTBREAK NEWS: Dengue fever, cape Verde (2009) (Accessed January 16, 2022).
35. DePina AJ, Stresman G, Barros HSB, Moreira AL, Dia AK, Furtado UD, et al. Updates on malaria epidemiology and profile in Cape Verde from 2010 to 2019: the goal of elimination. Malar J (2020) 19(1):380. doi: 10.1186/s12936-020-03455-7
36. Pereira C, Almeida C, Malta M, Vilaça R, Payo-Puente P. First report of Dirofilaria immitis in the republic of cape verde. Vet Parasitol (2013) 192(1-3):290–1. doi: 10.1016/j.vetpar.2012.09.032
37. Marcos R, Pereira C, Maia JP, Santos M, Luzzago C, Lauzi S, et al. The occurrence of the filarial nematode Dirofilaria repens in canine hosts from maio island, cape verde. J Helminthol (2017) 91(1):87–90. doi: 10.1017/S0022149X16000067
38. Alves J, Gomes B, Rodrigues R, Silva J, Arez AP, Pinto J, et al. Mosquito fauna on the cape Verde islands (West africa): an update on species distribution and a new finding. J Vector Ecol (2010) 35(2):307–12. doi: 10.1111/j.1948-7134.2010.00087.x
39. Ribeiro H, Ramos H, Capela R, Pires C. Os mosquitos de Cape Verde (Diptera: Culicidae). In: Sistematica, distribuição, bioecologia e importância médica. Lisboa: Junta de Investigações Científicas do Ultramar (1980).
40. Alves J, Pina AD, Diallo M, Dia I. First report of Culex (Culex) tritaeniorhynchus Giles, 1901 (Diptera: Culicidae) in the cape Verde islands. Zool CV (2014) 5(1):14–9.
41. Foley DH, Bryan JH, Lawrence GW. The potential of ivermectin to control the malaria vector Anopheles farauti. Trans R Soc Trop Med Hyg (2000) 94(6):625–8. doi: 10.1016/s0035-9203(00)90211-6
42. Githinji S, Herbst S, Kistemann T. The human ecology of malaria in a highland region of south-West kenya. Methods Inf Med (2009) 48(5):451–3. doi: 10.3414/ME9242
43. Ghebreyesus TA, Haile M, Witten KH, Getachew A, Yohannes M, Lindsay SW, et al. Household risk factors for malaria among children in the Ethiopian highlands. Trans R Soc Trop Med Hyg (2000) 94(1):17–21. doi: 10.1016/s0035-9203(00)90424-3
44. Hewitt S, Rowland M. Control of zoophilic malaria vectors by applying pyrethroid insecticides to cattle. Trop Med Int Health (1999) 4(7):481–6. doi: 10.1046/j.1365-3156.1999.00433.x
45. Iwashita H, Dida GO, Sonye GO, Sunahara T, Futami K, Njenga SM, et al. Push by a net, pull by a cow: can zooprophylaxis enhance the impact of insecticide treated bed nets on malaria control? Parasit Vectors (2014) 7:52. doi: 10.1186/1756-3305-7-52
46. Kaburi JC, Githuto JN, Muthami L, Ngure PK, Mueke JM, Mwandawiro CS. Effects of long-lasting insecticidal nets and zooprophylaxis on mosquito feeding behaviour and density in mwea, central kenya. J Vector Borne Dis (2009) 46(3):184–90.
47. Seyoum A, Balcha F, Balkew M, Ali A, Gebre-Michael T. Impact of cattle keeping on human biting rate of anopheline mosquitoes and malaria transmission around ziway, ethiopia. East Afr Med J (2002) 79(9):485–90. doi: 10.4314/eamj.v79i9.9121
48. World Health Organization. Toolkit for integrated vector management in Sub-Saharan Africa (2016) (Accessed April 5, 2022).
49. World Health Organization. Handbook for integrated vector management (2012) (Accessed June 5, 2022).
50. Reeves LE, Gillett-Kaufman JL, Kawahara AY, Kaufman PE. Barcoding blood meals: New vertebrate-specific primer sets for assigning taxonomic identities to host DNA from mosquito blood meals. PloS Negl Trop Dis (2018) 12(8):e0006767. doi: 10.1371/journal.pntd.0006767
51. Ribeiro H, da Cunha Ramos H. Guia ilustrado para a identificação dos mosquitoes de Angola (Diptera: Culicidae). Lisboa: Sociedade Portuguesa de Entomologia (1995).
52. Scott JA, Brogdon WG, Collins FH. Identification of single specimens of the Anopheles gambiae complex by the polymerase chain reaction. Am J Trop Med Hyg (1993) 49(4):520–9. doi: 10.4269/ajtmh.1993.49.520
53. Lardeux F, Loayza P, Bouchité B, Chavez T. Host choice and human blood index of Anopheles pseudopunctipennis in a village of the Andean valleys of bolivia. Malar J (2007) 6:8. doi: 10.1186/1475-2875-6-853
54. Garrett-Jones C. The human blood index of malaria vectors in relation to epidemiological assessment. Bull World Health Org (1964) 30(2):241–61.
55. Stephenson EB, Murphy AK, Jansen CC, Peel AJ, McCallum H. Interpreting mosquito feeding patterns in Australia through an ecological lens: an analysis of blood meal studies. Parasit Vectors (2019) 12(1):156. doi: 10.1186/s13071-019-3405-z
56. Kramer LD, Ciota AT. Dissecting vectorial capacity for mosquito-borne viruses. Curr Opin Virol (2015) 15:112–8. doi: 10.1016/j.coviro.2015.10.003
57. Mucci LF, Cardoso RP, Paula MBD, Scandar SAS, Pacchioni ML, Fernandes A, et al. Feeding habits of mosquitoes (Diptera: Culicidae) in an area of sylvatic transmission of yellow fever in the state of são paulo, brazil. J Venom Anim Toxins Incl Trop Dis (2015) 21:6. doi: 10.1186/s40409-015-0005-z
58. Zimmerman RH, Galardo AK, Lounibos LP, Arruda M, Wirtz R. Bloodmeal hosts of anopheles species (Diptera: Culicidae) in a malaria-endemic area of the Brazilian amazon. J Med Entomol (2006) 43(5):947–56. doi: 10.1603/0022-2585(2006)43[947:bhoasd]2.0.co;2
59. Escobar D, Ascencio K, Ortiz A, Palma A, Sánchez A, Fontecha G. Blood meal sources of anopheles spp. in malaria endemic areas of honduras. Insects (2020) 11(7):450. doi: 10.3390/insects11070450
60. Saavedra MP, Conn JE, Alava F, Carrasco-Escobar G, Prussing C, Bickersmith SA, et al. Higher risk of malaria transmission outdoors than indoors by Nyssorhynchus darlingi in riverine communities in the Peruvian amazon. Parasit Vectors (2019) 12(1):374. doi: 10.1186/s13071-019-3619-0
61. Fitzpatrick DM, Hattaway LM, Hsueh AN, Ramos-Niño ME, Cheetham SM. PCR-based bloodmeal analysis of Aedes aegypti and Culex quinquefasciatus (Diptera: Culicidae) in st. George parish, grenada. J Med Entomol (2019) 56(4):1170–5. doi: 10.1093/jme/tjz037
62. Pruszynski CA, Stenn T, Acevedo C, Leal AL, Burkett-Cadena ND. Human blood feeding by Aedes aegypti (Diptera: Culicidae) in the Florida keys and a review of the literature. J Med Entomol (2020) 57(5):1640–7. doi: 10.1093/jme/tjaa083
63. Scott TW, Morrison AC, Lorenz LH, Clark GG, Strickman D, Kittayapong P, et al. Longitudinal studies of Aedes aegypti (Diptera: Culicidae) in Thailand and Puerto Rico: population dynamics. J Med Entomol (2000) 37(1):77–88. doi: 10.1603/0022-2585-37.1.77
64. Barrera R, Bingham AM, Hassan HK, Amador M, Mackay AJ, Unnasch TR. Vertebrate hosts of Aedes aegypti and Aedes mediovittatus (Diptera: Culicidae) in rural Puerto rico. J Med Entomol (2012) 49(4):917–21. doi: 10.1603/me12046
65. Jansen CC, Webb CE, Graham GC, Craig SB, Zborowski P, Ritchie SA, et al. Blood sources of mosquitoes collected from urban and peri-urban environments in eastern Australia with species-specific molecular analysis of avian blood meals. Am J Trop Med Hyg (2009) 81(5):849–57. doi: 10.4269/ajtmh.2009.09-0008
66. Ponlawat A, Harrington LC. Blood feeding patterns of Aedes aegypti and Aedes albopictus in thailand. J Med Entomol (2005) 42(5):844–9. doi: 10.1093/jmedent/42.5.844
67. Scott TW, Chow E, Strickman D, Kittayapong P, Wirtz RA, Lorenz LH, et al. Blood-feeding patterns of Aedes aegypti (Diptera: Culicidae) collected in a rural Thai village. J Med Entomol (1993) 30(5):922–7. doi: 10.1093/jmedent/30.5.922
68. Harrington LC, Edman JD, Scott TW. Why do female Aedes aegypti (Diptera: Culicidae) feed preferentially and frequently on human blood? J Med Entomol (2001) 38(3):411–22. doi: 10.1603/0022-2585-38.3.411
69. Vazeille M, Yébakima A, Lourenço-de-Oliveira R, Andriamahefazafy B, Correira A, Rodrigues JM, et al. Oral receptivity of Aedes aegypti from cape Verde for yellow fever, dengue, and chikungunya viruses. Vector Borne Zoonotic Dis (2013) 13(1):37–40. doi: 10.1089/vbz.2012.0982
70. Diatta M, Spiegel A, Lochouarn L, Fontenille D. Similar feeding preferences of Anopheles gambiae and a. arabiensis in senegal. Trans R Soc Trop Med Hyg (1998) 92(3):270–2. doi: 10.1016/s0035-9203(98)91005-7
71. Lefèvre T, Gouagna LC, Dabiré KR, Elguero E, Fontenille D, Renaud F, et al. Beyond nature and nurture: phenotypic plasticity in blood-feeding behavior of Anopheles gambiae s.s. when humans are not readily accessible. Am J Trop Med Hyg (2009) 81(6):1023–9. doi: 10.4269/ajtmh.2009.09-0124
72. Muriu SM, Muturi EJ, Shililu JI, Mbogo CM, Mwangangi JM, Jacob BG, et al. Host choice and multiple blood feeding behaviour of malaria vectors and other anophelines in mwea rice scheme, kenya. Malar J (2008) 7:43. doi: 10.1186/1475-2875-7-43
73. Massebo F, Balkew M, Gebre-Michael T, Lindtjørn B. Blood meal origins and insecticide susceptibility of Anopheles arabiensis from chano in south-West ethiopia. Parasit Vectors (2013) 6:44. doi: 10.1186/1756-3305-6-44
74. Forattini OP, Kakitani I, Massad E, Gomes Ade C. Studies on mosquitoes (Diptera: Culicidae) and anthropic environment. 1-parity of blood seeking anopheles (Kerteszia) in south-eastern brazil. Rev Saude Publica (1993) 27(1):1–8. doi: 10.1590/s0034-89101993000100001
75. Beier JC. Frequent blood-feeding and restrictive sugar-feeding behavior enhance the malaria vector potential of Anopheles gambiae s.l. and An. funestus (Diptera: Culicidae) in western kenya. J Med Entomol (1996) 33(4):613–8. doi: 10.1093/jmedent/33.4.613
76. Arredondo-Jiménez JI, Rodríguez MH, Washino RK. Gonotrophic cycle and survivorship of Anopheles vestitipennis (Diptera: Culicidae) in two different ecological areas of southern mexico. J Med Entomol (1998) 35(6):937–42. doi: 10.1093/jmedent/35.6.937
77. Amerasinghe PH, Amerasinghe FP. Multiple host feeding in field populations of Anopheles culicifacies and an. subpictus in Sri lanka. Med Vet Entomol (1999) 13(2):124–31. doi: 10.1046/j.1365-2915.1999.00160.x
78. Ndenga BA, Mulaya NL, Musaki SK, Shiroko JN, Dongus S, Fillinger U. Malaria vectors and their blood-meal sources in an area of high bed net ownership in the western Kenya highlands. Malar J (2016) 15:76. doi: 10.1186/s12936-016-1115-y
79. Orsborne J, Furuya-Kanamori L, Jeffries CL, Kristan M, Mohammed AR, Afrane YA, et al. Using the human blood index to investigate host biting plasticity: a systematic review and meta-regression of the three major African malaria vectors. Malar J (2018) 17(1):479. doi: 10.1186/s12936-018-2632-7
80. Bravo-Barriga D, Parreira R, Almeida AP, Calado M, Blanco-Ciudad J, Serrano-Aguilera FJ, et al. Culex pipiens as a potential vector for transmission of Dirofilaria immitis and other unclassified filarioidea in southwest spain. Vet Parasitol (2016) 223:173–80. doi: 10.1016/j.vetpar.2016.04.030
81. Cancrini G, Scaramozzino P, Gabrielli S, Di Paolo M, Toma L, Romi R. Aedes albopictus and Culex pipiens implicated as natural vectors of Dirofilaria repens in central italy. J Med Entomol (2007) 44(6):1064–6. doi: 10.1603/0022-2585(2007)44[1064:aaacpi]2.0.co;2
82. Ferreira CAC, de Pinho Mixão V, Novo MTLM, Calado MMP, Gonçalves LAP, Belo SMD, et al. First molecular identification of mosquito vectors of Dirofilaria immitis in continental portugal. Parasit Vectors (2015) 8:139. doi: 10.1186/s13071-015-0760-2
83. Kronefeld M, Kampen H, Sassnau R, Werner D. Molecular detection of dirofilaria immitis, Dirofilaria repens and Setaria tundra in mosquitoes from germany. Parasit Vectors (2014) 7:30. doi: 10.1186/1756-3305-7-30
84. Morchón R, Bargues MD, Latorre JM, Melero-Alcibar R, Pou-Barreto C, Mas-Coma S, et al. Haplotype H1 of Culex pipiens implicated as natural vector of Dirofilaria immitis in an endemic area of Western spain. Vector Borne Zoonotic Dis (2007) 7(4):653–8. doi: 10.1089/vbz.2007.0124
85. Tomazatos A, Cadar D, Török E, Maranda I, Horváth C, Keresztes L, et al. Circulation of Dirofilaria immitis and Dirofilaria repens in the Danube delta biosphere reserve, romania. Parasit Vectors (2018) 11(1):392. doi: 10.1186/s13071-018-2980-8
86. Vezzani D, Mesplet M, Eiras DF, Fontanarrosa MF, Schnittger L. PCR detection of Dirofilaria immitis in Aedes aegypti and Culex pipiens from urban temperate argentina. Parasitol Res (2011) 108(4):985–9. doi: 10.1007/s00436-010-2142-1
87. Yildirim A, Inci A, Duzlu O, Biskin Z, Ica A, Sahin I. Aedes vexans and Culex pipiens as the potential vectors of Dirofilaria immitis in central turkey. Vet Parasitol (2011) 178(1-2):143–7. doi: 10.1016/j.vetpar.2010.12.023
88. Malkinson M, Banet C. The role of birds in the ecology of West Nile virus in Europe and africa. Curr Top Microbiol Immunol (2002) 267:309–22. doi: 10.1007/978-3-642-59403-8_15
89. Murgue B, Zeller H, Deubel V. The ecology and epidemiology of West Nile virus in Africa, Europe and asia. Curr Top Microbiol Immunol (2002) 267:195–221. doi: 10.1007/978-3-642-59403-8_10
90. Gomes B, Alves J, Sousa CA, Santa‐Ana M, Vieira I, Silva TL, et al. Hybridization and population structure of the Culex pipiens complex in the islands of macaronesia. Ecol Evol (2012) 2(8):1889–902. doi: 10.1002/ece3.307
91. Asigau S, Salah S, Parker PG. Assessing the blood meal hosts of Culex quinquefasciatus and Aedes taeniorhynchus in isla Santa Cruz, galápagos. Parasit Vectors (2019) 12(1):584. doi: 10.1186/s13071-019-3835-7
92. Agrawal SK. Host selection and multiple blood feeding behavior of Culex quinquefasciatus in Raipur city, chhattisgarh state, India. Int J App Res (2015) 1(4):30–3.
93. de Carvalho GC, Malafronte Rdos S, Miti Izumisawa C, Souza Teixeira R, Natal L, Marrelli MT. Blood meal sources of mosquitoes captured in municipal parks in são paulo, brazil. J Vector Ecol (2014) 39(1):146–52. doi: 10.1111/j.1948-7134.2014.12081.x
94. Börstler J, Jöst H, Garms R, Krüger A, Tannich E, Becker N, et al. Host-feeding patterns of mosquito species in germany. Parasit Vectors (2016) 9(1):318. doi: 10.1186/s13071-016-1597-z
95. Hamer GL, Kitron UD, Brawn JD, Loss SR, Ruiz MO, Goldberg TL, et al. Culex pipiens (Diptera: Culicidae): a bridge vector of West Nile virus to humans. J Med Entomol (2008) 45(1):125–8. doi: 10.1603/0022-2585(2008)45[125:cpdcab]2.0.co;2
96. Kay BH, Boreham PFL, Williams GM. Host preferences and feeding patterns of mosquitoes (Diptera: Culicidae) at kowanyama, cape York peninsula, northern Queensland. Bull Entomol Res (1979) 69(3):441–57. doi: 10.1017/S0007485300018952
97. Samuel PP, Arunachalam N, Hiriyan J, Thenmozhi V, Gajanana A, Satyanarayana K. Host-feeding pattern of Culex quinquefasciatus Say and Mansonia annulifera (Theobald) (Diptera: Culicidae), the major vectors of filariasis in a rural area of south india. J Med Entomol (2004) 41(3):442–6. doi: 10.1603/0022-2585-41.3.442
98. Muturi EJ, Muriu S, Shililu J, Mwangangi JM, Jacob BG, Mbogo C, et al. Blood-feeding patterns of Culex quinquefasciatus and other culicines and implications for disease transmission in mwea rice scheme, kenya. Parasitol Res (2008) 102(6):1329–35. doi: 10.1007/s00436-008-0914-7
99. McCall JW, Genchi C, Kramer LH, Guerrero J, Venco L. Heartworm disease in animals and humans. Adv Parasitol (2008) 66:193–285. doi: 10.1016/S0065-308X(08)00204-2
100. Fuehrer HP, Auer H, Leschnik M, Silbermayr K, Duscher G, Joachim A. Dirofilaria in humans, dogs, and vectors in Austria (1978-2014) - from imported pathogens to the endemicity of Dirofilaria repens. PloS Negl Trop Dis (2016) 10(5):e0004547. doi: 10.1371/journal.pntd.0004547
101. Simón F, Morchón R, González-Miguel J, Marcos-Atxutegi C, Siles-Lucas M. What is new about animal and human dirofilariosis? Trends Parasitol (2009) 25(9):404–9. doi: 10.1016/j.pt.2009.06.003
102. Simón F, López-Belmonte J, Marcos-Atxutegi C, Morchón R, Martín-Pacho JR. What is happening outside north America regarding human dirofilariasis? Vet Parasitol (2005) 133(2-3):181–9. doi: 10.1016/j.vetpar.2005.03.033
103. Anyanwu IN, Agbede RI, Ajanusi OJ, Umoh JU, Ibrahim ND. The incrimination of Aedes (stegomyia) aegypti as the vector of Dirofilaria repens in nigeria. Vet Parasitol (2000) 92(4):319–27. doi: 10.1016/s0304-4017(00)00311-3
104. Hendrix CM, Brunner CJ, Bellamy LK. Natural transmission of Dirofilaria immitis by Aedes aegypti. J Am Mosq Control Assoc (1986) 2(1):48–51.
105. Keesing F, Holt RD, Ostfeld RS. Effects of species diversity on disease risk. Ecol Lett (2006) 9(4):485–98. doi: 10.1111/j.1461-0248.2006.00885.x
106. Kuno G. Review of the factors modulating dengue transmission. Epidemiol Rev (1995) 17(2):321–35. doi: 10.1093/oxfordjournals.epirev.a036196
Keywords: Cape Verde, feeding behavior, blood meal, HBI, Ae. aegypti, An. arabiensis, Cx. pipiens s.l.
Citation: Gonçalves AALM, Dias AHC, Monteiro DDS, Varela IBF and da Veiga Leal S (2023) Blood meal survey reveals insights into mosquito-borne diseases on the island of Santiago, Cape Verde. Front. Trop. Dis 4:1070172. doi: 10.3389/fitd.2023.1070172
Received: 14 October 2022; Accepted: 31 January 2023;
Published: 24 February 2023.
Edited by:
Ines Martin-Martin, Carlos III Health Institute (ISCIII), SpainReviewed by:
Constância Flávia Junqueira Ayres, Oswaldo Cruz Foundation (Fiocruz), BrazilDonald A. Yee, University of Southern Mississippi, United States
Copyright © 2023 Gonçalves, Dias, Monteiro, Varela and da Veiga Leal. This is an open-access article distributed under the terms of the Creative Commons Attribution License (CC BY). The use, distribution or reproduction in other forums is permitted, provided the original author(s) and the copyright owner(s) are credited and that the original publication in this journal is cited, in accordance with accepted academic practice. No use, distribution or reproduction is permitted which does not comply with these terms.
*Correspondence: Adéritow Augusto Lopes Macedo Gonçalves, YWRlcml0b3cuZ29uY2FsdmVzQGluc3AuZ292LmN2
†These authors have contributed equally to this work and share first authorship