- 1Institute for Medical Microbiology, Immunology and Parasitology, University Hospital Bonn, Bonn, Germany
- 2Drugs for Neglected Diseases initiative, Geneva, Switzerland
- 3German Center for Infection Research (DZIF), partner site Bonn-Cologne, Bonn, Germany
Oxfendazole is one of the lead macrofilaricidal candidates for the treatment of onchocerciasis and lymphatic filariasis. Originally, oxfendazole was developed for the veterinary market, where it is mainly used to treat intestinal helminth infections. In humans, oxfendazole was proven to be safe in multiple ascending dose studies. Furthermore, previous experimental studies demonstrated that the benzimidazoles class is active in animals and humans against filarial nematodes. In the present study, we have compared the efficacy of oxfendazole isomers with the commercially available racemic mixture Dolthene against the rodent filaria Litomosoides sigmodontis in female BALB/c mice. Treatment with either the isomers or Dolthene led to a reduction of the adult worm burden by 94-98% following the ten-day treatment and by 72% (oxfendazole (-)), 85% (oxfendazole (+)) and 91% (Dolthene) following the five-day treatment. No statistically significant differences in the macrofilaricidal efficacy against L. sigmodontis were observed for both isomers and Dolthene. Metabolites of oxfendazole are fenbendazole and fenbendazole sulfone. Two hours after treatment with Dolthene and both oxfendazole isomers, fenbendazole sulfone, but rarely fenbendazole, was detected. The oxfendazole (-) isomer was metabolised at the highest rate to fenbendazole sulfone. Furthermore, oxfendazole isomers have a comparable pharmacokinetic profile in dogs. In conclusion, our data does not point at the development of a single isomer for future use in humans.
Introduction
Lymphatic filariasis (LF) and onchocerciasis (river blindness) are two of 20 neglected tropical diseases (NTDs). Both diseases are caused by filarial nematodes. The causative agents are Onchocerca volvulus for onchocerciasis and Wuchereria bancrofti, Brugia malayi and Brugia timori for LF, respectively (1). About 51.4 million people are currently infected with LF while 859 million people live in areas requiring preventive chemotherapies through mass drug administration (MDA) (2, 3). Typical symptoms of LF are lymphoedema which can develop into hydrocoele and elephantiasis (4). Onchocerciasis manifests with palpable skin nodules harbouring the adult filariae and may cause blindness (‘river blindness’) and severe dermatitis (1). There are an estimated 21 million onchocerciasis cases as of 2017 while 218 million people are at risk of infection (3, 5). Due to the severity of both filarial diseases, the World Health Organization (WHO) outlined a path to eliminate onchocerciasis via interruption of transmission and eliminate LF as a public health problem by 2030 (3). Access to treatments for both diseases occurs mainly via MDA with ivermectin (for onchocerciasis) or a combination of ivermectin, albendazole and diethylcarbamazine (for LF). Both treatments are microfilaricidal, i.e. they only target the progeny of the filariae. Thus, elimination of onchocerciasis and LF requires a continuous (bi-)annual treatment for the entire life span of the adult worms which is estimated at 5 years for LF and up to 15 years for onchocerciasis (1, 6). As a result, one critical action to facilitate the elimination of onchocerciasis as outlined in the 2030 WHO roadmap, is to develop a macrofilaricidal treatment that could be used in MDA programs (3) or for individual case management.
A promising macrofilaricidal candidate is oxfendazole (7, 8) which belongs to the benzimidazole class and acts as an inhibitor of microtubule polymerization via binding of tubulin (9). For mebendazole, an oxfendazole derivative, inhibition of glucose uptake and transport together with its effect on tubulin depolymerisation has been suggested to irreversibly cause degenerative modifications in the gastrointestinal tract of helminths. Thus, it is assumed that oxfendazole, similar to mebendazole, depletes the energy stores of helminths, resulting in helminth death (10). Oxfendazole has been used in veterinary medicine as a broad-spectrum anthelmintic treatment for livestock and companion animals for decades (6). Recent studies have shown that oxfendazole is a potent macrofilaricidal agent following a short oral treatment of 5 days in the in vivo Litomosoides sigmodontis rodent model (11). Furthermore, oxfendazole was shown to inhibit motility of O. volvulus adult worms in vitro (11). Importantly, oxfendazole had no significant efficacy in vivo against L. sigmodontis or Loa loa microfilariae injected in mice (11, 12). This strongly suggests that oxfendazole may specifically target the adult filarial stage. Such specificity is a strong advantage as fewer drug-induced adverse events in onchocerciasis patients or people (co-)infected with Loa loa are expected due to the death of microfilariae (8).
Recently, single and multiple ascending dose clinical studies in healthy volunteers have shown that oxfendazole is safe and well tolerated at dosages expected to be efficacious against filarial infections (11, 13–15). Prior studies against filariae and other helminths have shown significant differences in the anthelmintic activity of isomers, e.g. praziquantel against Schistosoma spp., tetramisole against Ostertagia spp. and Trichostrongylus axei (16, 17). As oxfendazole has a chiral centre on its sulphur atom, one remaining question for further development is the activity against filariae of both enantiomers (oxfendazole (+) and oxfendazole (-)). Those enantiomers may be metabolized at different rates from oxfendazole (=fenbendazole sulfoxide) to fenbendazole or fenbendazole sulfone, which could impact the efficacy against filariae, as fenbendazole sulfone has been suggested to be inactive against several helminths (18–20).
Thus, the aim of this study was to compare the macro- and microfilaricidal efficacy of two different oxfendazole enantiomers (oxfendazole (+) and oxfendazole (-)) against L. sigmodontis in the BALB/c mouse model, analyse their pharmacokinetic profile, their metabolization to fenbendazole as well as fenbendazole sulfone, and determine whether the development of a racemic mixture for future use in humans is justifiable.
Methods
Separation of the oxfendazole isomers
Oxfendazole (+) (lot FS00144340) and oxfendazole (-) (lot FS00144342) isomers were obtained by chiral separation from WuXi AppTec (Shanghai). The visual appearance of both isomers is a white powder. The structure of both isomers was confirmed by 1H NMR. The chiral conformation was not elucidated, however isomers were differentiated by specific optical conformation in 0.1% DMSO solution. The purity measured by LC-MS was 99.01% and 96.88% respectively for oxfendazole (+) and oxfendazole (-). Lastly the chiral purity measured by HPLC was 99.78% and 99.29% respectively for oxfendazole (+) and oxfendazole (-). Fenbendazole (lot BCBS1202V), of chemical formula C15H13N3O2S, molecular weight 299.35 g/mol and purity 99.7% was purchased by Syngene International Limited. Fenbendazole sulfone (lot BCBW6806) of chemical formula C15H13N3O4S, molecular weight 331.35 g/mol and purity 99.9% was also purchased by Syngene International Limited.
Ethics statement
All experimental procedures were conducted in accordance with EU Directive 2010/63/EU and approved by the appropriate authorities, i.e. Landesamt für Natur, Umwelt und Verbraucherschutz (LANUV, AZ 84-02.04.2015.A507, AZ 81-02.04.2020.A244). All in vivo mouse experiments were performed at the Institute for Medical Microbiology, Immunology and Parasitology (IMMIP) of the University Hospital Bonn, Germany.
The pharmacokinetic study in the dog was conducted at WuXi AppTec Co., Ltd. in accordance with the WuXi IACUC standard animal procedures along with the IACUC guidelines compliant with the Animal Welfare Act, the Guide for the Care and Use of Laboratory Animals.
Infection of BALB/c mice with L. sigmodontis and treatment
Female BALB/c mice were obtained from Janvier Labs, Saint-Berthevin, France, and maintained at the animal facilities of the IMMIP on a 12h dark/light cycle. Animals had constant access to food and water and were controlled for well-being once per day. A score of A-C was used to assess animal well-being as previously described (21). 6-8 week old female BALB/c mice were naturally infected with L. sigmodontis via contact with Ornithonyssus bacoti, the tropical rat mite, as previously described (22, 23). Infected mice were treated per os, two times per day for 5 or 10 days starting at 35 days post infection (dpi), a timepoint at which adult filariae have developed, but microfilariae are not present yet. Mice were treated with a commercially available racemic mixture of oxfendazole (Dolthene) dissolved in corn oil (Sigma-Aldrich, Germany) or one of two oxfendazole isomers (separated by chiral chromatography by Syngene International Limited) in a 3% sodium carboxymethyl cellulose (Sigma-Aldrich, Germany) suspension in corn oil. Vehicle controls received corn oil with 3% sodium carboxymethyl cellulose. Treated animals (n=8) received 12.5 oxfendazole (PK and efficacy study, single experiment) or 25 mg/kg oxfendazole (efficacy study, single experiment) in a volume of 5 mL/kg.
Mice were euthanized with an overdose of isoflurane (AbbVie, Wiesbaden, Germany) at 70 dpi. Worms from the pleural cavity were collected by washing the pleural cavity with 6-8 ml of phosphate buffered saline (PBS). Remaining worms were collected with forceps. The recovered worms were counted.
To determine microfilaremia, 50 μL of blood was taken from the facial vein and collected in ethylenediaminetetraacetic acid (EDTA) coated tubes (Sarstedt AG & Co. KG, Nümbrecht, Germany) immediately before necropsy. 50 μL of blood was mixed with 950 μL red blood cell lysis buffer (eBioscience, USA) and incubated for 10 minutes at room temperature. Afterwards blood was centrifuged at 400 g for 5 min. The supernatant was discarded and microfilariae in the pellet were counted with light microscopy.
Pharmacokinetic analysis
40 μL of blood for pharmacokinetic analysis was taken 2 hours after the first (35 dpi) and second to last (39 dpi) treatment with oxfendazole from female BALB/c mice (n=8, single experiment) infected with L. sigmodontis. Samples were collected in EDTA tubes (Sarstedt AG & Co. KG, Nümbrecht, Germany) and stored on ice. Plasma was isolated after centrifugation at 3220 g, 10 min, 4°C.
Analysis was conducted with a Sciex LC-MS Triple Quad 6500-7. 32 μL of sample was mixed with 96 μL internal standard (100 ng/ml labetalol, 100 ng/ml tolbutamide and 200 nM nadolol in acetonitrile) and centrifuged at 4000 g for 10 min, 4°C. 60 μL of supernatant was transferred to sample plates and mixed with 120 μL water. The plate was shaken at 800 rpm for 10 min before analysis. For oxfendazole (+) and (-), a Chiral-AGP 150 x 4.0 mm, 5 µm column (Chrom Tech, Inc./ChiralPak) with a flow rate of 0.7 mL/min was used. The mobile phase consisted of 90% phase A (1% formic acid in water, pH 7.5) and 10% B (isopropyl alcohol). For fenbendazole and fenbendazole sulfone, an ACQUITY UPLC BEH C18 2.1*50 mm, 1.7 µM column with a flow rate of 0.6 ml/min was used. Mobile phase A consisted of 0.1% formic acid in water and phase B consisted of 0.1% formic acid in acetonitrile. Ratio of phases A:B was 85:15 from start until 1.10 min, 2:98 until 1.50 min and 85:15 until 2.00 min.
Electrospray positive ionization with selected ion MS/MS monitoring was used for the determination of oxfendazole (= fenbendazole sulfoxide; m/z 316.0/284.1), fenbendazole (m/z 300/267.9) and fenbendazole sulfone (m/z 332/300.1)
Single doses of 10 mg/kg of each isomer were administrated by oral gavage (PO) to non-naïve male Beagle dogs (n=3, single experiment, Marshall Bioresources, Beijing, China). Both isomers and metabolites, fenbendazole, and fenbendazole sulfone were monitored in plasma for up to 24 hours in two groups of 3 animals each. Oxfendazole (+) and oxfendazole (-) were formulated as a homogenous opaque suspension at 22.65 mg/mL in 3% sodium methylcellulose. Prior to dosing, the dose formulation concentration was verified by LC/UV. Dogs were fed prior to the day of oral dosing and water remained available ad libitum. Approximately 0.5 mL of blood were collected from a peripheral vessel from restrained, non-sedated animals into a commercial tube (Jiangsu Kangjian medical supplies co., LTD) containing Potassium (K2) EDTA·2H2O (0.85-1.15 mg) on wet ice and processed for plasma. An aliquot of 40 µL was used for protein precipitation by quenching, then vortexed, and centrifuged for 15 min at 12000 g, 4 °C. 50 µL of supernatant were transferred in a 96-well plate, centrifuged for 5 min at 3220 g and 4 °C. The supernatant was directly injected for analysis using LCMS-01-SMBA-Triple Quad 6500 Plus.
For oxfendazole (+) and (-), the metabolites fenbendazole and fenbendazole sulfone were analysed in a cassette using column CHIRALCEL OD-RH 4.6··150 mm with a flow rate of 0.8 mL/min at 30°C. The mobile phase consisted of phase A (0.1% formic acid and 2mM HCOONH4 in H2O/ACN (95/5) and B (0.1% formic acid and 2mM HCOONH4 in ACN/H2O (95/5)). Phase B was 25% from start until 11 min, 26% until 11.8 min and 100% until 16 min.
Oxfendazole, fenbendazole sulfoxide, fenbendazole and fenbendazole sulfone were sourced from Sigma Aldrich Germany and all the reagents used for the bioanalysis were provided by WuXi AppTec Shanghai.
Statistical analysis
Statistical analysis was performed with GraphPad Prism Software version 8.4.3 (San Diego, USA). Statistical significance for adult worm burden and number of microfilariae was assessed with the Kruskal-Wallis test followed by Dunn’s multiple comparison test. Differences in the plasma concentrations of oxfendazole and its metabolites in mice were evaluated for a normal distribution with the Shapiro-Wilk test and then analysed with a repeated measure two-way ANOVA with Geisser-Greenhouse correction and Tukey’s multiple comparison test. P values < 0.05 were considered significant. Values in the text are arithmetic mean with 95% confidence interval (CI).
Results
Oxfendazole isomers display a similar treatment duration-dependent macrofilaricidal efficacy against L. sigmodontis in vivo
Macrofilaricidal efficacy of a commercially available racemic mixture of oxfendazole (Dolthene) was compared to two isomers (oxfendazole (+) and oxfendazole (-)) in 6–8-week-old female BALB/c mice infected with L. sigmodontis. Mice were treated for 5 or 10 days from 35 dpi onwards, i.e. after the development of adult filariae and before the onset of microfilaremia (24) with 25 mg/kg oxfendazole twice per day and necropsy was performed 70 dpi to determine adult worm burden and development of microfilaremia (Figure 1A).
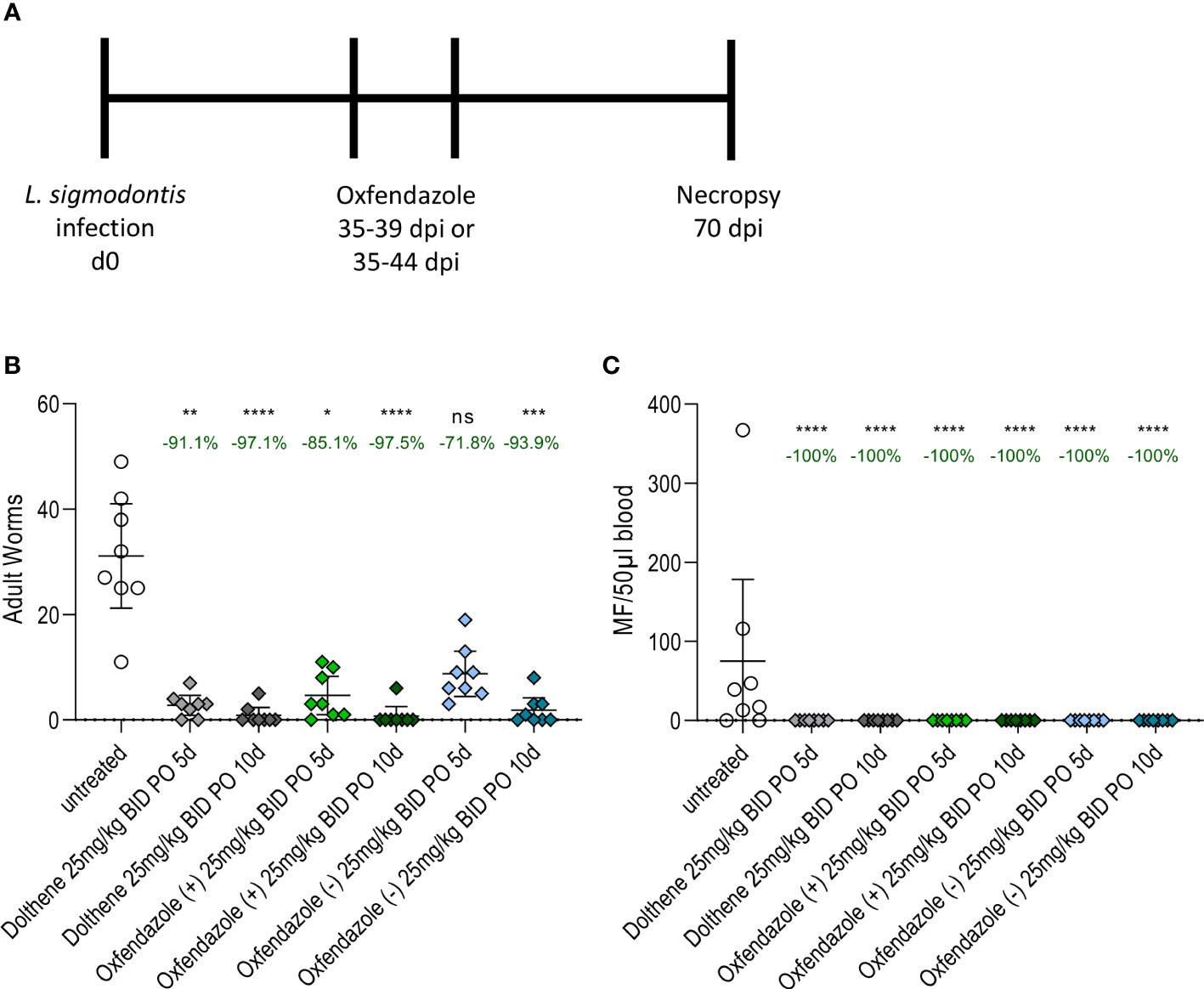
Figure 1 Isomers mediate similar macrofilaricidal efficacy against L. sigmodontis in BALB/c mice. (A) Experimental design. 6-8 week old female BALB/c mice (n=8) were naturally infected L. sigmodontis and treated orally with 25 mg/kg oxfendazole or one of two isomers for 5 or 10 days twice per day. Adult worm burden and microfilariae numbers were determined at 70 dpi. (B) Adult worm burden in the pleural cavity and (C) number of microfilariae in 50 µl peripheral blood at 70 dpi. Graphs show arithmetic mean with 95% CI from a single experiment. Treated groups were compared to vehicle controls with Kruskal-Wallis and Dunn’s posthoc test. The numbers over each group indicate the reduction of the arithmetic mean compared to the vehicle control. *p < 0.05, **p < 0.01, ***p < 0.001, ****p < 0.0001, ns = p > 0.05.
Dolthene reduced the adult worm burden by 91.1% (arithmetic mean 2.7 worms, 95% CI 0.8 – 4.6 worms) after 5 days of treatment and 97.1% (0.8 worms, 95% CI 0 – 2.3 worms) after 10 days of treatment respectively compared to vehicle controls (31.1 worms, 95% CI 21.2 – 41.1 worms; Figure 1B). By comparison, oxfendazole (+) reduced the number of adult worms by 85.1% (4.6 worms, 95% CI 0.9 – 8.2 worms) and 97.5% (0.7 worms, 95% CI 0 – 2.5 worms), whereas oxfendazole (-) caused a reduction of 71.8% (8.7 worms, 95% CI 4.4 – 13.0 worms) and 93.9% (1.8 worms, 95% CI 0 – 4.2 worms) after 5 or 10 days of treatment, respectively. All treatment conditions led to a significant reduction of the adult worm burden (p < 0.05) compared to vehicle controls except for the 5-day treatment with oxfendazole (-) (p = 0.87). There was no statistically significant difference in the adult worm burden between groups treated with oxfendazole (+), oxfendazole (-) and Dolthene.
Next, the efficacy of oxfendazole and its isomers to prevent the development of microfilaremia was analysed (Figure 1C). All treatment regimens led to a complete lack of microfilariae in the peripheral blood 70 dpi, whereas 6 out of 8 vehicle-treated mice developed microfilaremia at that time point. Prior studies have shown that oxfendazole has no strong effect on microfilariae in vitro or in vivo (11) and thus we conclude that the prevention of microfilaremia is caused by the clearance of adult worms and inhibited embryogenesis of remaining adult worms (as described in (11)). This is supported by the fact that treatments were performed before the occurrence of microfilaremia (microfilaremia starts around 8 weeks after infection, treatment was initiated 5 weeks after infection) and the short half-life of 2-3h of oxfendazole after oral treatment (11). Confirmation of a similar macrofilaricidal efficacy of Dolthene and both isomers was given by an additional study that tested a 5-day oral treatment with 12.5 mg/kg twice per day in L. sigmodontis-infected mice (Figure S1). The reduction of the adult worm burden ranged from 63% (oxfendazole (+)) over 75% (Dolthene) to 83% (oxfendazole (-), Figure S1A). All mice treated with Dolthene and oxfendazole isomers had no microfilariae in the peripheral blood at 70 dpi (Figure S1B).
Taken together, both isomers mediated a comparable macrofilaricidal efficacy against L. sigmodontis in BALB/c mice and prevented the development of microfilaremia in all animals studied. Of note, no adverse reactions to any of the substances were observed during or after treatment.
Both oxfendazole isomers are metabolised into fenbendazole sulfone at different rates
Next, plasma levels of oxfendazole (=fenbendazole sulfoxide) as well as its metabolites (Figure 2) fenbendazole and fenbendazole sulfone were measured in satellite PK compared on the first and last day of treatment (Figure 3). Mice were treated twice per day for 5 days from 35 dpi onwards with 12.5 mg/kg of Dolthene or one of two isomers. Blood from the facial vein was collected 2 hours after the first and second to last treatment.

Figure 2 Metabolism of oxfendazole. Oxfendazole (=fenbendazole sulfoxide, C15H15N3O3S) is reversibly reduced into fenbendazole (C15H15N3O2S) or oxidized into fenbendazole sulfone (C15H15N3O4S).
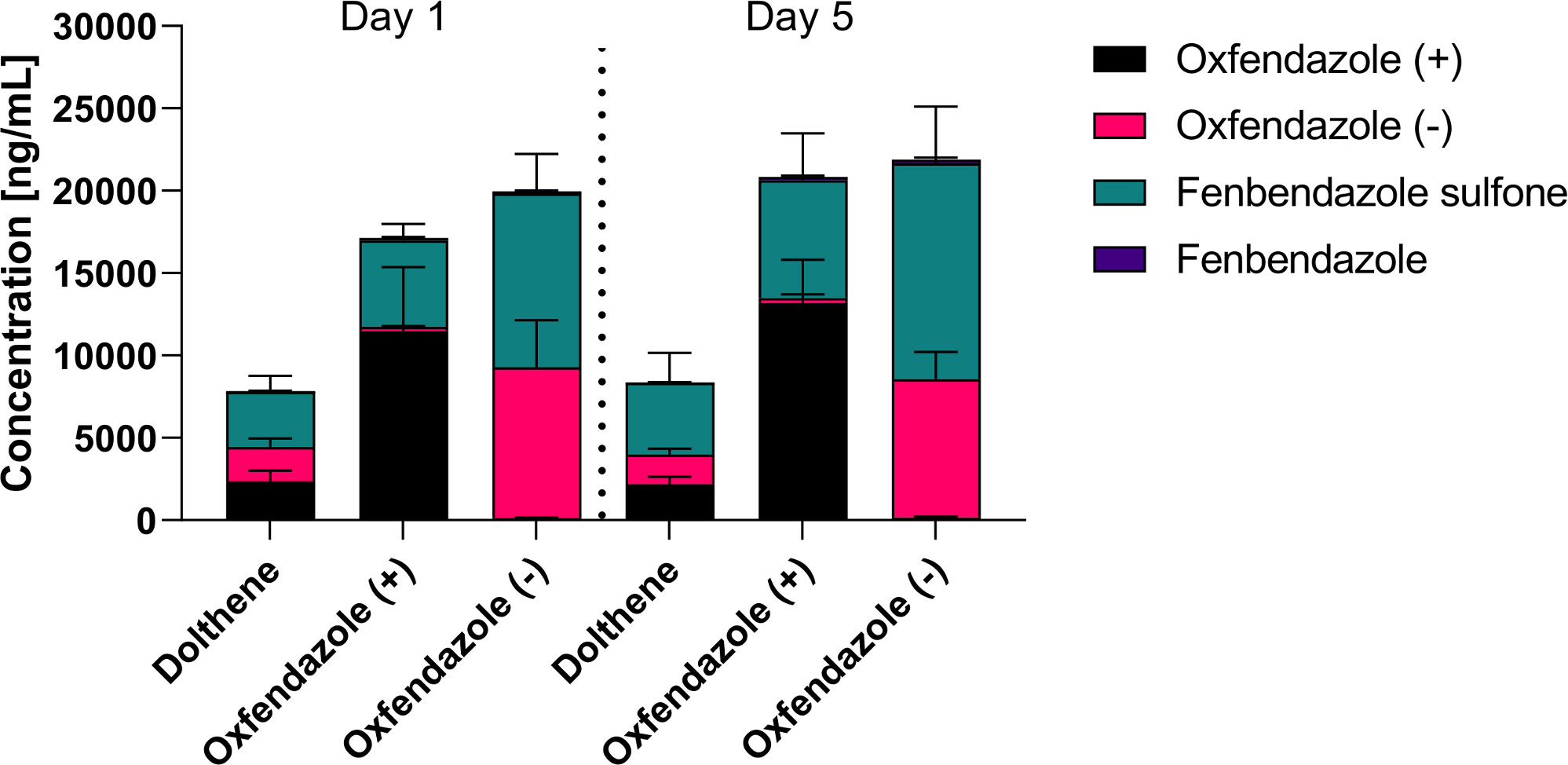
Figure 3 Plasma concentrations of oxfendazole and its metabolites on the first and last day of treatment. 6-8 week old female BALB/c mice (n=8) were treated orally with 12.5 mg/kg commercially available racemic mixture of oxfendazole (Dolthene) or one of two isomers twice per day for 5 days. Shown is the plasma level of oxfendazole, both oxfendazole isomers, as well as its metabolites fenbendazole and fenbendazole sulfone in peripheral blood 2 h after the first and second to last treatment from a single experiment.
Plasma concentrations of oxfendazole and its metabolites were comparable at both time points which suggests no significant accumulation in the plasma during the treatment (Figure 3). There were no statistically significant differences between plasma concentrations of oxfendazole (+)/(-) or fenbendazole sulfone on day 1 and day 5 in each treatment group. Interestingly, oxfendazole (-) seemed to be metabolized into fenbendazole sulfone more thoroughly than oxfendazole (+) on both the first (52.8 vs. 30.6% of oxfendazole metabolites in the plasma) and last (59.8% vs. 34.3%) day of treatment. Accordingly, total plasma levels of fenbendazole sulfone were significantly higher in mice treated with oxfendazole (-) compared to mice treated with oxfendazole (+) on both day 1 (oxfendazole (-): 10544 ng/ml, 95% CI 8840 – 12200 vs. oxfendazole (+): 5255 ng/ml, 95% CI 4570 – 5950, p = 0.0023) and day 5 (oxfendazole (-): 13100 ng/ml, 95% CI 10700 – 15500 vs. oxfendazole (+): 7148 ng/ml 95% CI 5190 – 9110, p = 0.0214). Dolthene was metabolized into fenbendazole sulfone by 42.7% (3350 ng/ml, 95% CI 2529 – 4171 ng/ml) and 52.0% (4352 ng/ml, 95% CI 2823 – 5881 ng/ml) on day 1 and 5 after treatment, respectively. Levels of fenbendazole (= reduced oxfendazole) were relatively low at < 200 ng/ml plasma and comparable across all groups and time points.
As the pharmacokinetic profile of oxfendazole and its metabolites has been described to be similar in humans and dogs (15, 25), we assessed exposure of both oxfendazole (+) and oxfendazole (-) in dogs. Male beagle dogs (n=3) were given 10 mg/kg of oxfendazole (+) or oxfendazole (-) by oral gavage. Plasma concentrations were measured at 0.083, 0.25, 0.5, 1, 2, 4, 8, 12, and 24 hours. The pharmacokinetic profiles of oxfendazole isomers and metabolites are described in Figure 4. The maximum concentration (Cmax) and the area under the curve AUC0-24 calculated by a non-compartmental model are summarized in Table 1. Oxfendazole isomers are stable in dog plasma as conversion from one isomer to another is minimal. Exposure of both isomers is comparable, although higher concentrations of fenbendazole sulfone were measured in the oxfendazole (-) group.
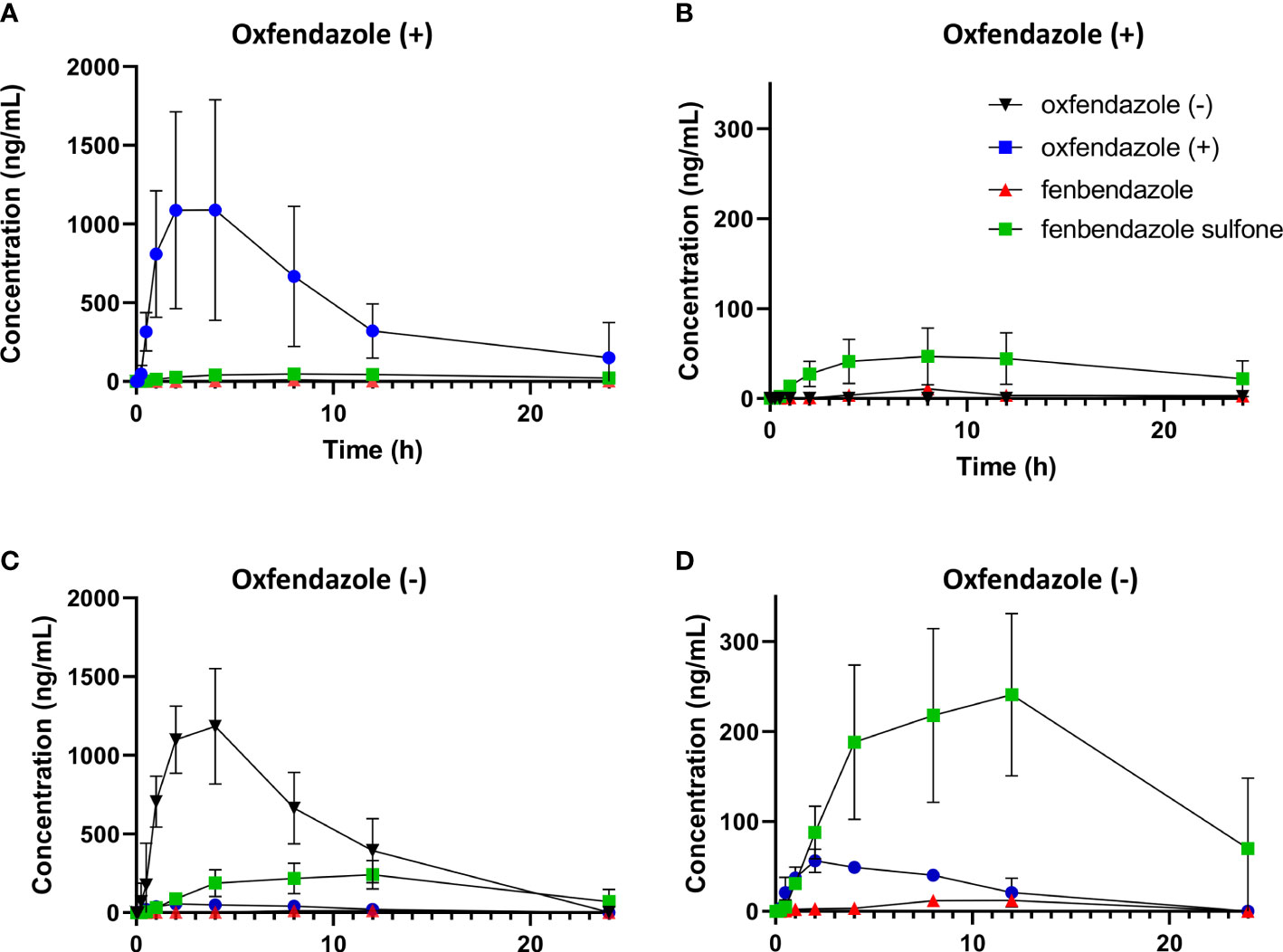
Figure 4 Pharmacokinetic profile of oxfendazole isomers and their metabolites in dogs. Male beagles (n=3) were treated orally with 10 mg/kg of each isomer. Concentration of oxfendazole (+) [blue circles], oxfendazole (-) [black inverted triangles], fenbendazole sulfone [green squares] and fenbendazole [red triangles] were assessed via HPLC-MS/MS at indicated time points after treatment. (A) Plasma level of oxfendazole metabolites after treatment with 10 mg/kg oxfendazole (+). (B) Plasma level after treatment with 10 mg/kg oxfendazole (+) without the level of oxfendazole (+). (C) Plasma level of oxfendazole metabolites after treatment with 10 mg/kg oxfendazole (-). (D) Plasma level after treatment with 10 mg/kg oxfendazole (-) without the level of oxfendazole (-). Graphs show mean with standard deviation from a single experiment. Error bars that are not visible are hidden as they are smaller than the size of the symbol.
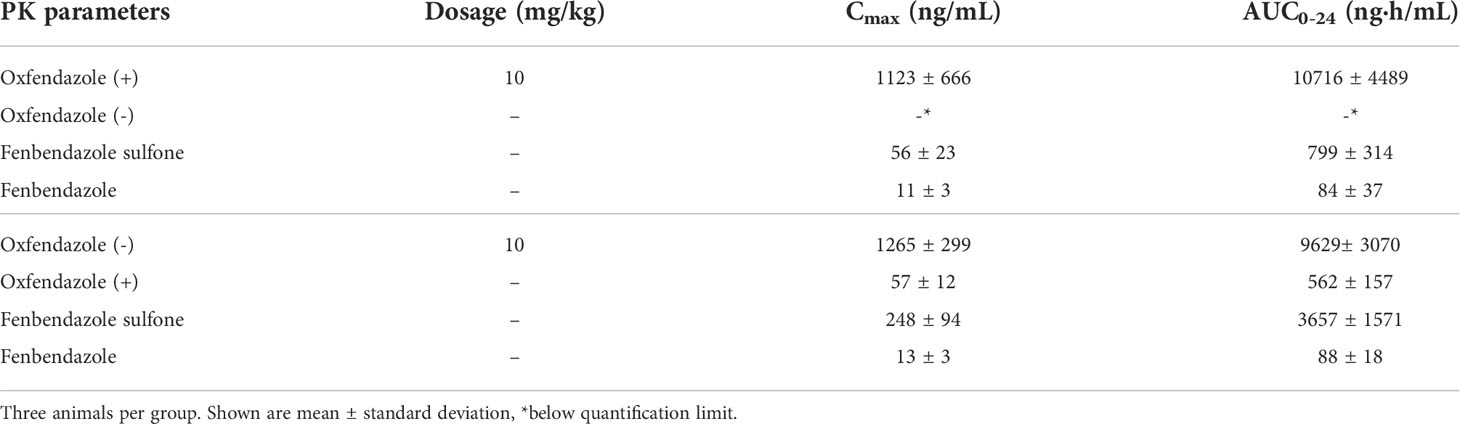
Table 1 Maximum concentration (Cmax) and area under the curve (AUC0-24) of oxfendazole isomers and metabolites in dogs orally treated with 10 mg/kg oxfendazole (+) and oxfendazole (-).
Discussion
Our study demonstrates that the two oxfendazole isomers, oxfendazole (+) and oxfendazole (-), have a similar treatment duration-dependent macrofilaricidal efficacy against L. sigmodontis as oxfendazole. In addition, oxfendazole and its isomers prevented the development of microfilaremia in all animals. Pharmacological studies showed that all three oxfendazole treatments led to elevated plasma levels of the compound itself as well as oxidized oxfendazole (=fenbendazole sulfone). Reduction into fenbendazole or metabolization into the alternative isomer did not occur in any relevant amount. These pharmacokinetic observations are in line with other recent studies in dogs (26, 27) and mice (11). The observed lack of accumulation over the course of the treatment supports the idea from previous studies (11) that treatment duration rather than high dosage is key for an effective macrofilaricidal treatment with oxfendazole.
In both dogs and mice, at equal dosages, oxfendazole (+) seems to have a lower oxidation into fenbendazole sulfone than oxfendazole (-). Although fenbendazole sulfone has been suggested to be inactive against several helminths (18–20), both isomers have a similar in vivo efficacy against L. sigmodontis. Thus, despite both isomers having different oxidation rates into fenbendazole sulfone, a racemic mixture for treatment of filarial infections in humans should be considered.
Interestingly, albendazole sulfone, a metabolite of albendazole, was reported to have anti-Wolbachia properties (28). Whether the sulfone metabolite of oxfendazole (fenbendazole sulfone) has a similar anti-Wolbachia activity is not known yet and should be analysed in the future.
Due to its excellent macrofilaricidal efficacy (6, 7, 11), oxfendazole is developed by the Drugs for Neglected Diseases initiative (DNDi) for use against onchocerciasis (29). With funds from the EU HELP project (https://eliminateworms.org/) DNDi developed a field-applicable tablet and phase 1 bioavailability studies are ongoing. Thus, phase II clinical studies in filariasis patients are in preparation. Furthermore, the selective macrofilaricidal property of oxfendazole indicates that oxfendazole is also safe for treatment in loiasis patients, independent of their microfilarial levels. Therefore, oxfendazole could be used for treatment of onchocerciasis patients in areas that are co-endemic with loiasis and current MDAs are hindered, and it presents the first safe, short-term macrofilaricidal treatment for loiasis patients.
The current study is limited in scope as it uses only a single rodent model of infection. Potentially relevant differences include the shorter life span of L. sigmodontis compared to O. volvulus, Brugia spp. and W. bancrofti as well as the site of infection. Adult worms of L. sigmodontis reside in the thoracic cavity while adult worms of O. volvulus can be found in the skin and in the case of B. malayi, B. timori and W. bancrofti in the lymphatic vessels (1, 30). However, the L. sigmodontis BALB/c mouse model is well established for the preclinical development of anti-filarial compounds, and its translation for human-pathogenic filariae was shown with drugs such as doxycycline, rifampicin, minocycline and albendazole (1, 8, 31–34).
Overall, our results suggest that both oxfendazole isomers display similar anti-filarial activity and our data do not support the development of a single isomer for future use in human patients.
Data availability statement
The original contributions presented in the study are included in the article/Supplementary Material. Further inquiries can be directed to the corresponding author.
Ethics statement
The mouse studies were reviewed and approved by Landesamt für Natur, Umwelt und Verbraucherschutz. The study in dogs was approved by WuXi IACUC guidelines.
Author contributions
Conceived and designed the experiments: SS, AH, MH, and IS. Performed the experiments: FR, MK, and BL. Analyzed the data: FR, MK, MH, and IS. Contributed reagents/materials/analysis tools: MH and AH. Wrote the manuscript: FR, MH, and IS. All authors contributed to the article and approved the submitted version.
Funding
AH and MH are members of the German Center for Infection Research (DZIF). MH received funding from the German Center for Infection Research (TTU 09.701). This work was supported by the Drugs for Neglected Diseases initiative through funding provided by USAID. The funders had no role in the study design, data collection and analysis, decision to publish, or preparation of the manuscript.
Acknowledgments
We acknowledge Dr. Mathieu Louis (formerly affiliated at DNDi) for his contribution for the separation of the oxfendazole enantiomers and Dr. Zhang Mengyue from WuXi AppTec for the bioanalytical analysis and pharmacokinetic study.
Conflict of interest
The authors declare that the research was conducted in the absence of any commercial or financial relationships that could be construed as a potential conflict of interest.
Publisher’s note
All claims expressed in this article are solely those of the authors and do not necessarily represent those of their affiliated organizations, or those of the publisher, the editors and the reviewers. Any product that may be evaluated in this article, or claim that may be made by its manufacturer, is not guaranteed or endorsed by the publisher.
Supplementary material
The Supplementary Material for this article can be found online at: https://www.frontiersin.org/articles/10.3389/fitd.2022.982421/full#supplementary-material
References
1. Taylor MJ, Hoerauf A, Bockarie M. Lymphatic filariasis and onchocerciasis. Lancet (2010) 376(9747):1175–85. doi: 10.1016/S0140-6736(10)60586-7
2. Local Burden of Disease 2019 Neglected Tropical Diseases Collaborators. The global distribution of lymphatic filariasis, 2000-18: a geospatial analysis. Lancet Glob Health (2020) 8(9):e1186–94. doi: 10.1016/S2214-109X(20)30286-2
3. World Health Organization. Ending the neglect to attain the sustainable development goals: a road map for neglected tropical diseases 2021–2030. Geneva: World Health Organization (2020) 1–80.
4. Lourens GB, Ferrell DK. Lymphatic filariasis. Nurs Clin North Am (2019) 54(2):181–92. doi: 10.1016/j.cnur.2019.02.007
5. GBD 2017 DALYs and HALE Collaborators. Global, regional, and national disability-adjusted life-years (DALYs) for 359 diseases and injuries and healthy life expectancy (HALE) for 195 countries and territories, 1990-2017: a systematic analysis for the global burden of disease study 2017. Lancet (2018) 392(10159):1859–922. doi: 10.1016/S0140-6736(18)32335-3
6. Hawryluk NA. Macrofilaricides: An unmet medical need for filarial diseases. ACS Infect Dis (2020) 6(4):662–71. doi: 10.1021/acsinfecdis.9b00469
7. Gonzalez AE, Codd EE, Horton J, Garcia HH, Gilman RH. Oxfendazole: a promising agent for the treatment and control of helminth infections in humans. Expert Rev Anti Infect Ther (2019) 17(1):51–6. doi: 10.1080/14787210.2018.1555241
8. Ehrens A, Hoerauf A, Hübner MP. Current perspective of new anti-wolbachial and direct-acting macrofilaricidal drugs as treatment strategies for human filariasis. GMS Infect Dis (2022) 10:Doc02. doi: 10.3205/id000079
9. Lacey E. Mode of action of benzimidazoles. Parasitol Today (1990) 6(4):112–5. doi: 10.1016/0169-4758(90)90227-u
10. Available at: https://www.accessdata.fda.gov/drugsatfda_docs/label/2017/017481s047lbl.pdf (Accessed 13th September, 2022).
11. Hübner MP, Martin C, Specht S, Koschel M, Dubben B, Frohberger SJ, et al. Oxfendazole mediates macrofilaricidal efficacy against the filarial nematode Litomosoides sigmodontis in vivo and inhibits Onchocerca spec. motility in vitro. PloS Negl Trop Dis (2020) 14(7):e0008427. doi: 10.1371/journal.pntd.0008427
12. Pionnier NP, Sjoberg H, Chunda VC, Fombad FF, Chounna PW, Njouendou AJ, et al. Mouse models of loa loa. Nat Commun (2019) 10(1):1429. doi: 10.1038/s41467-019-09442-0
13. Bach T, Murry DJ, Stebounova LV, Deye G, Winokur P, An G. Population pharmacokinetic model of oxfendazole and metabolites in healthy adults following single ascending doses. Antimicrob Agents Chemother (2021) 65(4):e02129–20. doi: 10.1128/AAC.02129-20
14. Bach T, Galbiati S, Kennedy JK, Deye G, Nomicos EYH, Codd EE, et al. Pharmacokinetics, safety, and tolerability of oxfendazole in healthy adults in an open-label phase 1 multiple ascending dose and food effect study. Antimicrob Agents Chemother (2020) 64(11):e01018–20. doi: 10.1128/AAC.01018-20
15. An G, Murry DJ, Gajurel K, Bach T, Deye G, Stebounova LV, et al. Pharmacokinetics, safety, and tolerability of oxfendazole in healthy volunteers: a randomized, placebo-controlled first-in-Human single-dose escalation study. Antimicrob Agents Chemother (2019) 63(4):e02255–18. doi: 10.1128/AAC.02255-18
16. Forsyth BA. The anthelmintic activity of the optical isomers of tetramisole in sheep and cattle. Aust Vet J (1968) 44(9):395–400. doi: 10.1111/j.1751-0813.1968.tb09128.x
17. Sharma LK, Cupit PM, Goronga T, Webb TR, Cunningham C. Design and synthesis of molecular probes for the determination of the target of the anthelmintic drug praziquantel. Bioorg Med Chem Lett (2014) 24(11):2469–72. doi: 10.1016/j.bmcl.2014.04.014
18. Moreno L, Lopez-Urbina MT, Farias C, Domingue G, Donadeu M, Dungu B, et al. A high oxfendazole dose to control porcine cysticercosis: pharmacokinetics and tissue residue profiles. Food Chem Toxicol (2012) 50(10):3819–25. doi: 10.1016/j.fct.2012.07.023
19. Lacey E, Brady RL, Prichard RK, Watson TR. Comparison of inhibition of polymerisation of mammalian tubulin and helminth ovicidal activity by benzimidazole carbamates. Vet Parasitol (1987) 23(1-2):105–19. doi: 10.1016/0304-4017(87)90029-x
20. Capece BP, Virkel GL, Lanusse CE. Enantiomeric behaviour of albendazole and fenbendazole sulfoxides in domestic animals: pharmacological implications. Vet J (2009) 181(3):241–50. doi: 10.1016/j.tvjl.2008.11.010
21. Schiefer A, Hübner MP, Krome A, Lämmer C, Ehrens A, Aden T, et al. Corallopyronin a for short-course anti-wolbachial, macrofilaricidal treatment of filarial infections. PloS Negl Trop Dis (2020) 14(12):e0008930. doi: 10.1371/journal.pntd.0008930
22. Risch F, Ritter M, Hoerauf A, Hübner MP. Human filariasis-contributions of the Litomosoides sigmodontis and Acanthocheilonema viteae animal model. Parasitol Res (2021) 120(12):4125–43. doi: 10.1007/s00436-020-07026-2
23. Nieguitsila A, Frutos R, Moulia C, Lhermitte-Vallarino N, Bain O, Gavotte L, et al. Fitness cost of Litomosoides sigmodontis filarial infection in mite vectors; implications of infected haematophagous arthropod excretory products in host-vector interactions. BioMed Res Int (2013) 2013:584105. doi: 10.1155/2013/584105
24. Hübner MP, Torrero MN, McCall JW, Mitre E. Litomosoides sigmodontis: a simple method to infect mice with L3 larvae obtained from the pleural space of recently infected jirds (Meriones unguiculatus). Exp Parasitol (2009) 123(1):95–8. doi: 10.1016/j.exppara.2009.05.009
25. Codd EE, Ng HH, McFarlane C, Riccio ES, Doppalapudi R, Mirsalis JC, et al. Preclinical studies on the pharmacokinetics, safety, and toxicology of oxfendazole: toward first in human studies. Int J Toxicol (2015) 34(2):129–37. doi: 10.1177/1091581815569582. Cysticercosis Working Group in Peru.
26. Colella V, Maia C, Pereira A, Gonçalves N, Caruso M, Martin C, et al. Evaluation of oxfendazole in the treatment of zoonotic Onchocerca lupi infection in dogs. PloS Negl Trop Dis (2018) 12(1):e0006218. doi: 10.1371/journal.pntd.0006218
27. Gokbulut C, Bilgili A, Hanedan B, McKellar QA. Comparative plasma disposition of fenbendazole, oxfendazole and albendazole in dogs. Vet Parasitol (2007) 148(3-4):279–87. doi: 10.1016/j.vetpar.2007.06.028
28. Serbus LR, Landmann F, Bray WM, White PM, Ruybal J, Lokey RS, et al. A cell-based screen reveals that the albendazole metabolite, albendazole sulfone, targets Wolbachia. PloS Pathog (2012) 8(9):e1002922. doi: 10.1371/journal.ppat.1002922
29. Available at: https://dndi.org/research-development/portfolio/oxfendazole/ (Accessed 19th May, 2022 12:03).
30. Petit G, Diagne M, Maréchal P, Owen D, Taylor D, Bain O. Maturation of the filaria Litomosoides sigmodontis in BALB/c mice; comparative susceptibility of nine other inbred strains. Ann Parasitol Hum Comp (1992) 67(5):144–50. doi: 10.1051/parasite/1992675144
31. Hübner MP, Koschel M, Struever D, Nikolov V, Frohberger SJ, Ehrens A, et al. In vivo kinetics of Wolbachia depletion by ABBV-4083 in. L. sigmodontis adult worms and microfilariae. (2019) 13(8):e0007636. doi: 10.1371/journal.pntd.0007636
32. Specht S, Pfarr KM, Arriens S, Hübner MP, Klarmann-Schulz U, Koschel M, et al. Combinations of registered drugs reduce treatment times required to deplete Wolbachia in the Litomosoides sigmodontis mouse model. PLos Negl Trop Dis (2018) 12(1):e0006116. doi: 10.1371/journal.pntd.0006116
33. Hoerauf A, Specht S, Büttner M, Pfarr K, Mand S, Fimmers R, et al. Wolbachia endobacteria depletion by doxycycline as antifilarial therapy has macrofilaricidal activity in onchocerciasis: a randomized placebo-controlled study. Med Microbiol Immunol (2008) 197(3):295–311. doi: 10.1007/s00430-007-0062-1
Keywords: Oxfendazole, Litomosoides sigmodontis, Microfilariae, Isomer, Filariae, Macrofilaricidal, Fenbendazole
Citation: Risch F, Koschel M, Lenz B, Specht S, Hoerauf A, Hübner MP and Scandale I (2022) Comparison of the macrofilaricidal efficacy of oxfendazole and its isomers against the rodent filaria Litomosoides sigmodontis. Front. Trop. Dis. 3:982421. doi: 10.3389/fitd.2022.982421
Received: 30 June 2022; Accepted: 20 September 2022;
Published: 19 October 2022.
Edited by:
Jeremy Foster, New England Biolabs, United StatesReviewed by:
David Abraham, Thomas Jefferson University, United StatesWilliam Sullivan, University of California, Santa Cruz, United States
Copyright © 2022 Risch, Koschel, Lenz, Specht, Hoerauf, Hübner and Scandale. This is an open-access article distributed under the terms of the Creative Commons Attribution License (CC BY). The use, distribution or reproduction in other forums is permitted, provided the original author(s) and the copyright owner(s) are credited and that the original publication in this journal is cited, in accordance with accepted academic practice. No use, distribution or reproduction is permitted which does not comply with these terms.
*Correspondence: Marc P. Hübner, huebner@uni-bonn.de
†These authors have contributed equally to this work and share senior authorship