- 1Department of Biological Sciences, University of North Carolina at Charlotte, Charlotte, NC, United States
- 2School of Medical Laboratory Diseases, Faculty of Health Sciences, Institute of Health, Jimma University, Jimma, Ethiopia
- 3Department of Bioinformatics and Genomics, University of North Carolina at Charlotte, Charlotte, NC, United States
- 4School of Data Science, University of North Carolina, Charlotte, NC, United States
- 5Tropical and Infectious Diseases Research Center, Jimma University, Jimma, Ethiopia
The identification and management of low parasitemia infections have become increasingly challenging for malaria control and elimination. Submicroscopic Plasmodium infections and G6PD deficiency among febrile patients require more sensitive diagnostic methods to improve detection and careful treatment regime of these infections. In Ethiopia, information on the low density submicroscopic malarial infections and frequency of G6PD deficiency (G6PDd) is scarce. In this study, 297 malaria suspected febrile patient samples were collected from health facilities of Bonga town in southwestern Ethiopia. The positivity rates of Plasmodium infection were determined by microscopy and quantitative PCR. G6PD activity level was determined by careSTART™ G6PD biosensor and the frequency of three common variants: G6PD*A (A376G), G6PD*A− (G202A) and Mediterranean (C563T) were investigated. G6PD gene sequencing was performed to detect mutations in exons 2–11 for both G6PD normal and deficient samples based on the phenotypic assay. More than twice Plasmodium infected samples was detected by qPCR (52/297; 17.4%) than microscopy (21/297; 7.0%). About 31 (10%) of the infections were submicroscopic. Bednet usage and age had a significant association with Plasmodium infection. Of the 271 participants who were tested for G6PD phenotype, 19 (7.0%) had low G6PD level. No mutations were observed in A376G, G202A, and C563T in the G6PDd samples, but three novel non-synonymous mutations in exon 2 including a C to T transition at position ChrX:6504 (Arg to Thr), G to T at ChrX:6369 (Ser to IIe), and G to C at ChrX:6664 (Gln to His) were detected. A high number of submicroscopic Plasmodium infections observed in this study pose a challenge for accurate and timely diagnosis, which could hinder malaria control efforts. G6PD deficiency in malaria patients pose danger when treating patients with primaquine. The three novel mutations detected in exon 2 of the G6PD gene merit further investigation on the hemolytic risk when exposed to oxidative antimalarials, their prevalence, and clinical significance.
Background
Malaria is one of the major tropical diseases that poses a threat to public health and adversely affects the economic development of countries in sub-Sahara Africa (1). In Ethiopia, malaria accounts for about 60% of all hospital admission. Much of malaria-related illness and death occur in the most remote, rural areas of the country where there is insufficient health care coverage, poverty, and other socio-economic issues (2–4). In 2019, a total of 904,496 malaria cases responsible for 213 deaths were recorded. Ethiopia is one of the few African countries where Plasmodium falciparum and P. vivax coexist (5, 6). Plasmodium falciparum accounted for 81% of the total cases, and the remaining cases were attributed to P. vivax (7, 8). As malaria prevalence has shown slight decline in the past few years, transmission appears to be heterogeneous across the country, with some areas being relatively free whereas others are still facing a high malaria burden (5, 6, 9).
Malaria control and elimination strategies heavily depend on timely, accurate diagnosis and effective treatment (10, 11). Microscopy is a gold standard and common malaria diagnostic test in several African countries because of its high specificity, convenient with a rapid turnaround time, and is inexpensive. However, it has limited sensitivity especially for low parasitemia infections, which require highly competent microscopists complemented by molecular assays. An improved point-of-care detection method is critical because transmission caused by low parasitemia infections hinders the progress and goal of malaria elimination (10). Systematic identification and treatment of individuals with submicroscopic Plasmodium infections as part of the surveillance and intervention strategy would reduce and eliminate the parasite reservoirs that lead to transmission (11, 12).
Another factor that impacts the risk of malaria infections is glucose-6-phosphate dehydrogenase deficiency (G6PDd), which is the most common enzymatic disorder of red blood cells, affecting 400 million people worldwide (13). G6PDd is an X-linked disorder with high prevalence particularly in people of African, Asian, and Mediterranean descent. The gene encoding the G6PD protein is polymorphic, with more than 400 variants. In Sub-Saharan Africa, G6PD* B, G6PD*A and G6PD*A- were the most common variants (14). The G6PD variants were relative homogeneous in America, Africa, and western Asia, sharply contrasted with the heterogeneity of variants in China and Southeast Asia. While individuals with G6PD-deficient alleles have selective advantage against severe malaria, they can still be infected with low plasmodium densities and contribute to the transmission reservoirs. The prevalence of G6PDd is high in areas where malaria is endemic (15). In Ethiopia, G6PDd was estimated to be 1-14% (16), but G6PD information is still lacking in most part of southwestern Ethiopia where malaria transmission is high.
The use of single dose primaquine has been recently adopted by the Ethiopian Federal Ministry of Health (FMoH) as a radical treatment regime in 239 districts that are targeted for elimination. However, such treatment regime will be provided without prior G6PD testing. This could pose a high risk to malaria patients who are G6PD deficient and treated with primaquine. Thus, information on the prevalence of submicroscopic infections and G6PD deficiency in malaria patents is necessary for accurate diagnosis and risk-free treatment. This study determined the prevalence and factors associated with submicroscopic malaria infections, the frequency of G6PD deficiency, and the association between G6PD phenotype and genotype among malaria suspected febrile individuals in Southwest part of Ethiopia.
Materials and methods
Ethics approval and consent to participate
The study protocol was reviewed and approved by Institutional Review Board (IRB) of Institute of Health, Jimma University (Ref. No. IHRPGD/711/20) and written informed consent/assent was obtained from all consenting heads of households, parents/guardians, and individuals who were willing to participate in the study. All experimental procedures were performed following the IRB approved protocol.
Study site and sample collection
A total of 297 malaria suspected febrile patients were collected at the Bonga Gebretsadiek Shawo general hospital of Bonga town, Southwestern Ethiopia (7°16’N 36°14’E with an average elevation of 1,714 meters; Figure 1) from July to October 2020 based on a cross-sectional study design. Socio-demographic data including gender, age, occupation, records of antimalarial drug treatment, medical history, hemoglobin and G6PD levels (Tables 1, 2) was collected from each study participant. For participants aged below 18 years old, the questionnaire was completed by their guardians or parents. Finger-pricked blood sample of approximately 300µl was collected and preserved in a EDTA tube following standard WHO protocols (17). Thin and thick blood smears were prepared for microscopic examination of malaria parasites (18). A sample was considered as malaria-negative if no parasites were detected after examining 200 fields of the thick smear following standard WHO protocol (19–21). All slides were read in duplicate by two microscopists at the time of sample collection. For samples that showed discrepancy with qPCR results, we repeated the slide read for verification.
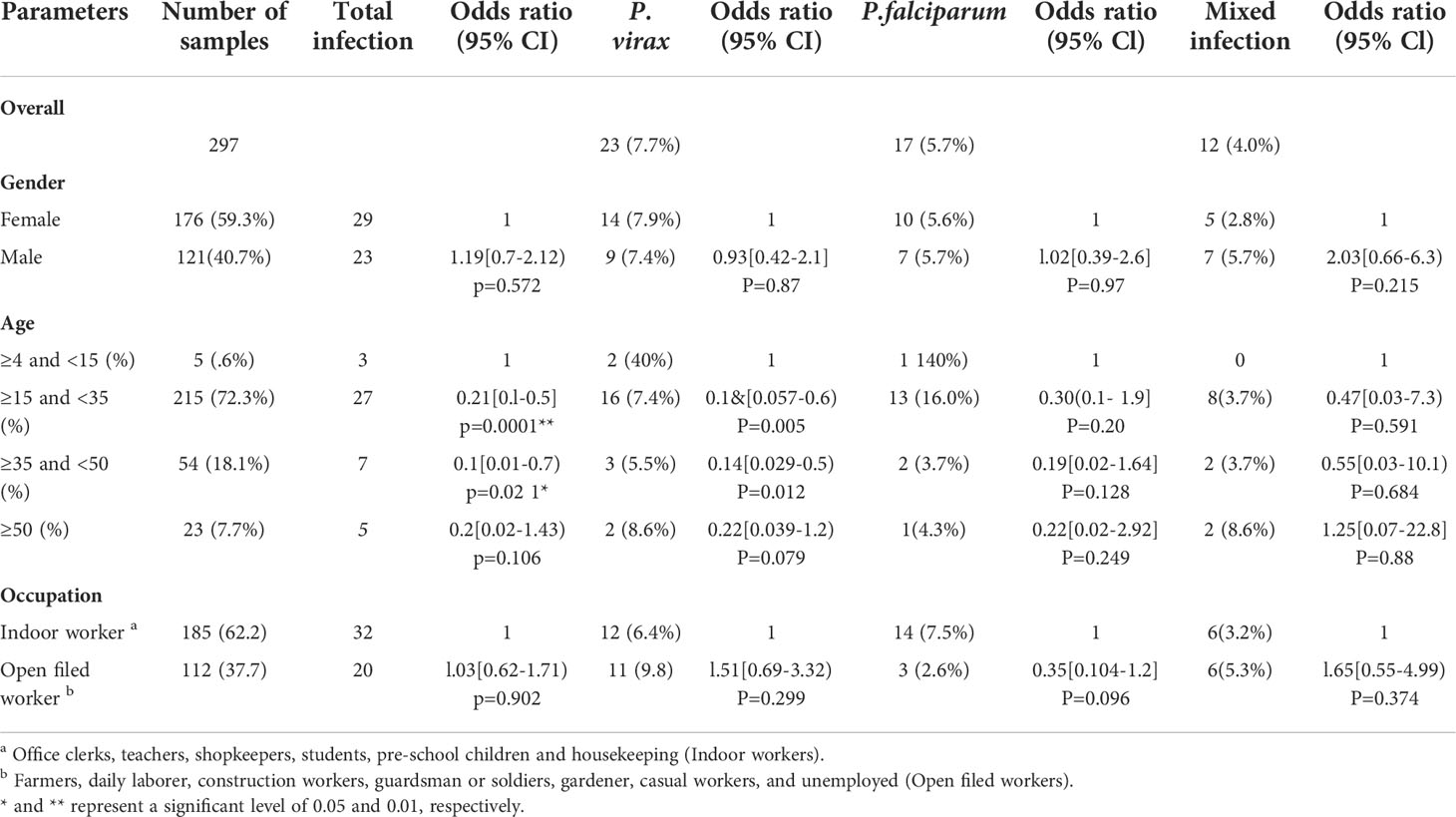
Table 1 Demographic characteristics of clinical malaria among suspected febrile individuals in Bonga, southwestern Ethiopia.

Table 2 Prevalence of submicroscopic infections among 297 malaria suspected febrile individuals in Bonga, southwestern Ethiopia.
Parasite DNA extraction and molecular screening
For each sample, parasite DNA was extracted from a dried blood spot (~50 µl) using the Saponin/Chelex method (22). Plasmodium species was examined by quantitative PCR of the 18S rDNA gene using species-specific primers, for P. falciparum and P. vivax, respectively (23, 24). Amplification was performed in a 20 μl reaction mixture containing 2 μl of genomic DNA, 10 μl 2×SYBR Green qPCR Master Mix (Thermo Scientific), and 0.5 µM primer with an initial denaturation at 95°C for 3 min, followed by 45 cycles at 94°C for 30 sec, 55°C for 30 sec, and 68°C for 1 min with a final 95°C for 10 sec. This was followed by a melting curve step of temperature ranging from 65°C to 95°C with 0.5°C increments to determine the melting temperature of each amplified product. Each assay included positive controls of P. falciparum HB-2 767G (MRA-667) and P. vivax E. coli (MRA-178), in addition to negative controls including uninfected samples and nuclease-free water. A standard curve was produced from a 10-fold serial dilution of the control plasmids to determine the efficiency of qPCR. Melting curve analyses were performed for each amplified sample to confirm specific amplifications of the target sequence. Samples yielding threshold cycle (Ct value) higher than 38 (as indicated in the negative controls) were considered negative for Plasmodium species. Parasite density in a sample was quantified with the follow equation: GCN sample = 2 E×(38-Ct sample); where GCN stands for gene copy number, Ct for the threshold cycle of the sample, and E for amplification efficiency (25). The differences in the log-transformed parasite GCN between the microscopic-positive and -negative samples were assessed for significance at the level of P<0.05 by one-tailed t-tests.
Measuring G6PD phenotype
Blood samples from each participant were tested for G6PD enzyme levels by the careSTART™ POCT S1 system (Access Bio Inc.) following the manufacturer’s instructions. Two drops (20–30 μl) of finger-prick whole blood was transferred by micro-pipettes to a strip and inserted into the biosensor at room temperature. The G6PD level was displayed within four minutes and automatically recorded. G6PD normal and deficient individuals were categorized into two classes in male and three classes in female following the WHO classification (26, 27). The adjusted male median (AMM) G6PD activity, defined as the median G6PD activity of all male participants after excluding samples with less than 10% of the overall median activity, was calculated. For male, class I is G6PD deficient with < 30% of the AMM activity and class II is normal with > 30% of the AMM activity. For female, G6PD activity < 30%, 30–80%, and > 80% of the AMM activity are considered as G6PD deficient, intermediate, and normal, respectively (27).
G6PD genotyping
Four PCR assays were conducted using previously published primers to determine mutations in exons 2–11 of the G6PD gene (Supplementary Table 1). For each assay, water was used as a negative control. PCR amplification was conducted in a 20μl reaction mixture containing 2 μl of genomic DNA (~50 ng/μl), 10 μl of 2×Maxima SYBR Green PCR Master Mix (Thermo Fisher) and 0.3uM of each forward and reverse primers. Amplifications were performed with an initial denaturation at 94°C for 3 min, followed by 38 cycles at 94°C for 30 sec, 55°C for 30 sec and 72°C for 60 sec, with a final 6 min extension at 72°C. Amplified PCR products were separated by gel electrophoresis with 1.5% agarose gel at 120 V for 2.00 hours. Amplified PCR products were purified and sequenced on an ABI 3730xl DNA analyzer (28). Sequences were analyzed using ClustalX v2.1 and BioEdit v7.2 (29). All sequences were aligned to the NCBI reference sequence (NG_009015.2) to verify the specificity of the PCR products. Samples with poor sequencing quality or showing singleton mutations were re-amplified and sequenced.
Statistical analyses
All data were analyzed using SPSS software package version 21.0. Descriptive statistics was used to summarize the socio-demographic characteristics of the study participants. Bivariate logistic regression was used to determine association of malaria infection with independent variables. Odds ratio and the corresponding 95% CI was calculated to determine the strength of the association. P-value < 0.05 was considered as statistically significant.
Results
Demographic characteristics of study participants
Among all patient samples, 121 (40.7%) were males and 176 (59.3%) were females (Table 1). About 112 (37.2%) participants were engaged in agriculture and open field work (farmers, construction workers, guardsmen and soldiers, gardeners); the remaining 185 (62.2%) were involved in work that is indoors (office clerks, teachers, shopkeepers, students, pre-school children, and housekeepers). The number of Plasmodium infection detected by qPCR (52 out of 297; 17.4%) was more than two-fold higher than that detected by microscopy (21 out of 297; 7.0%). Among the qPCR-positive samples, 23 (7.7%) were identified as P. vivax, 17 (5.7%) as P. falciparum, and 12 (4.0%) as mixed infection (Table 3). Age group and bednet usage were significantly associated with Plasmodium infection (P<0.05). For those who aged ≥15 - <35 (AOR=0.21, 95% CI: 0.02–0.59, P=0.0001) and ≥35 - <50 (AOR=0.1, 95% CI: 0.01–0.7, P=0.021) were significantly more likely to have malaria infection than those aged below 15 and above 50. Also, those who did not use bednet had significantly higher odds for malaria infection (AOR=0.54, 95% CI: 0.34–0.86, P=0.01) than those who routinely used bednet. All the other variables did not show significant association with Plasmodium infection (P>0.05; Table 2).
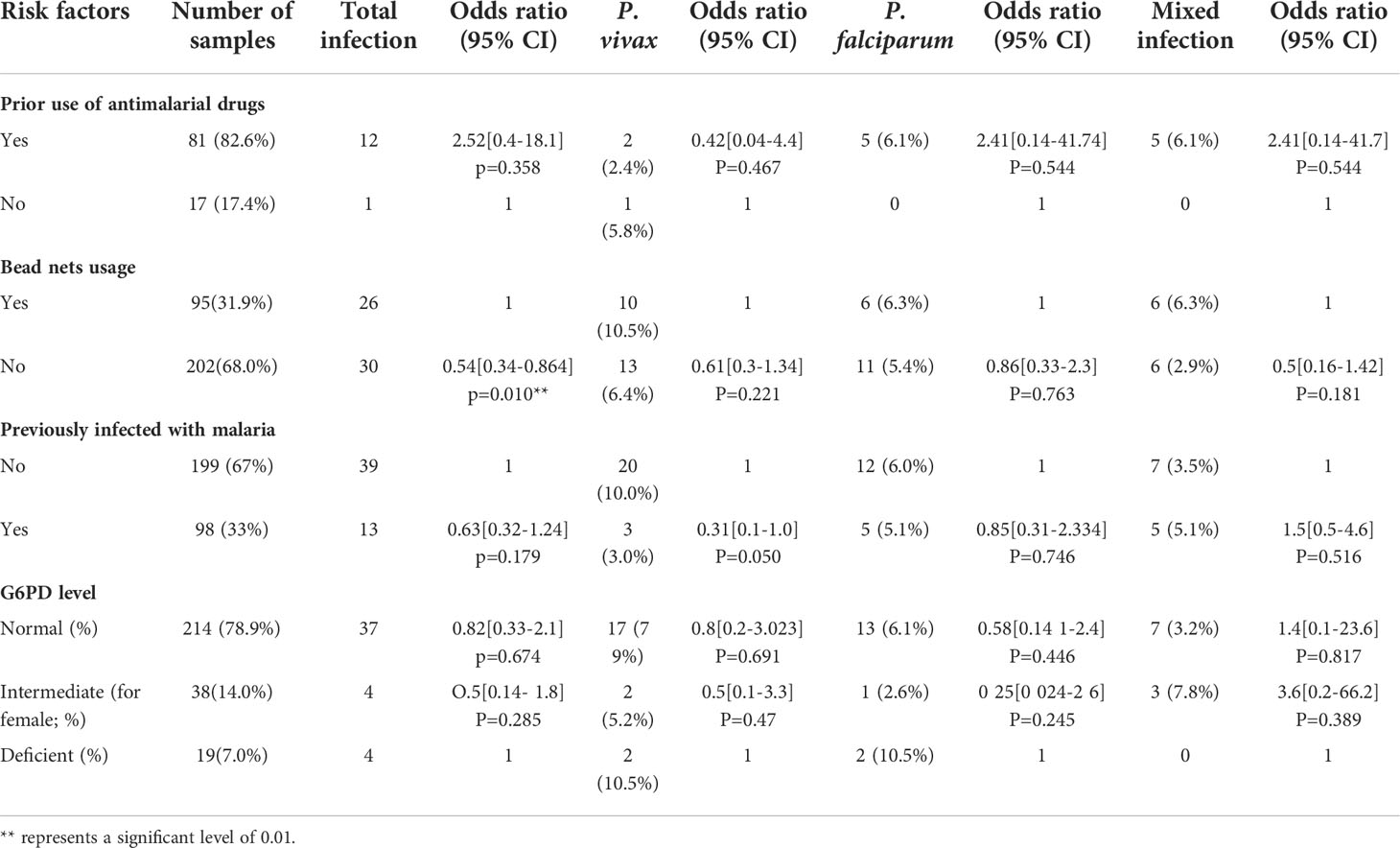
Table 3 Risk factors associated with clinical malaria among febrile individuals in Bonga, southwestern Ethiopia.
Frequency of G6PD deficiency based on phenotypic assay
The adjusted median G6PD enzyme activity for male participants was calculated by excluding 26 participants who had enzyme activity less than 10% (i.e., below 0.405 U/g Hb) of the median value obtained for all male participants. The median G6PD activity for all study participants was 4.0 U/g Hb (range: <0.4–13.5 U/g Hb). The adjusted male median enzyme activity (4.05 U/g Hb) was considered 100% enzyme activity (Figure 2). Participants with enzyme activity between 0.4-1.215 U/g Hb (10%-30% of adjusted male median) were considered as deficient. Based on this cut-off value, 19 (6 males and 13 females; 7%) out of 271 participants were identified as deficient for G6PD enzyme activity, 38 (14.0%) had intermediate (for female) and 214 (78.9%) are normal (Table 4). For the six G6PD deficient males, four of them were between 4-35 years old, and two of them were between 35-≤50 years old. For the 13 G6PD deficient females, five of them were between 40-50 years old, and the remaining were younger than 18 years of age. Both P. vivax and P. falciparum infections were observed in G6PD deficient patients (Figure 3). No significant association was observed between G6PD level and Plasmodium infection (P>0.05; Table 2).
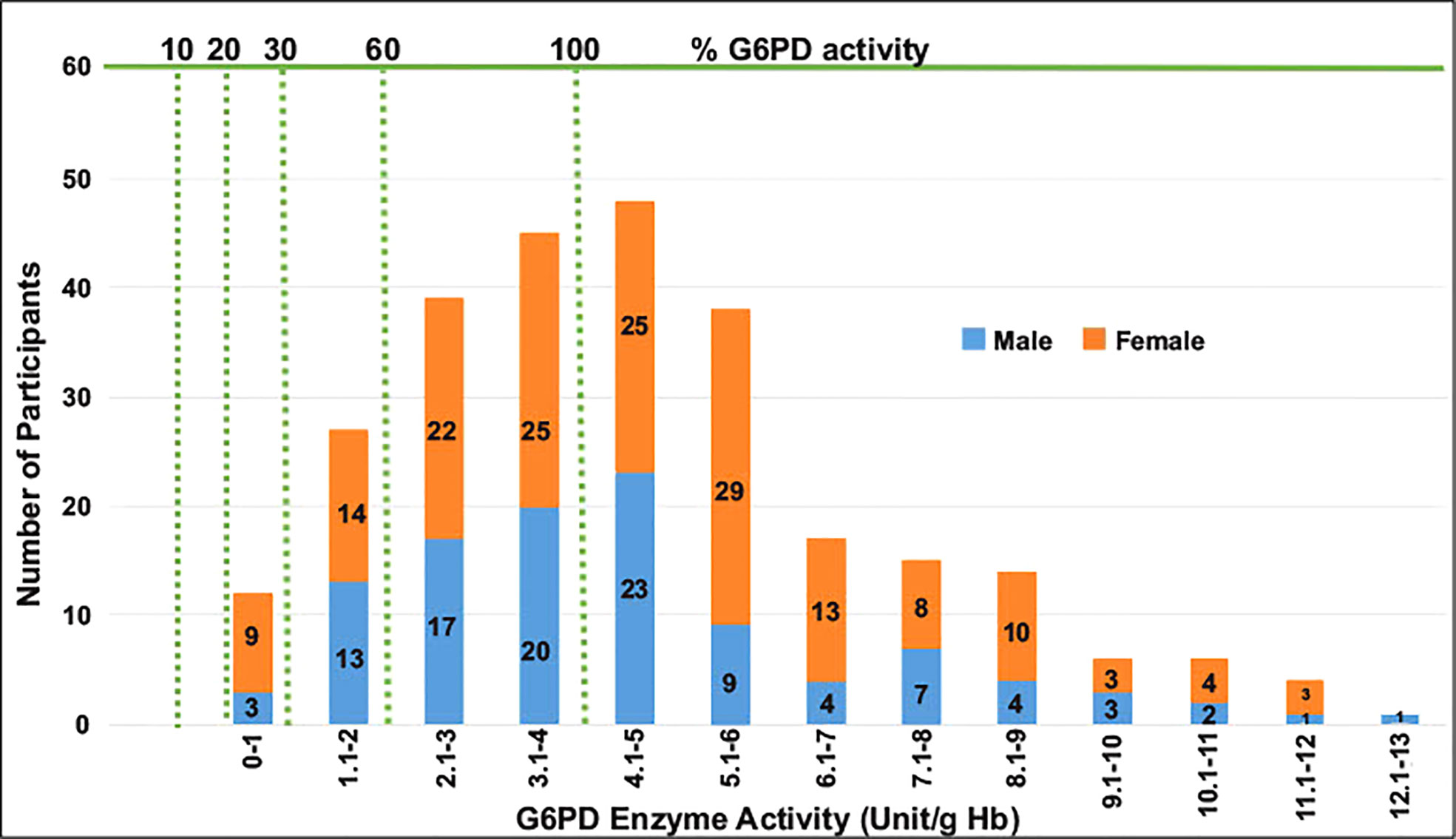
Figure 2 A histogram to show G6PD enzyme activity levels for male and female participants compared to adjusted male median value.

Table 4 G6PD phenotype distributions among male and female individuals in Bonga, southwestern Ethiopia.
Association between G6PD phenotype and genotype
No mutations were detected in C563T, G202A, A376G, nor ChrX:154535443 across all samples (Table 5). However, sequence analysis revealed three new mutations in exon 2 namely ChrX:6504 C→T (resulting in the substitution Arg → Thr), ChrX:6369 G→T (resulting in the substitution Ser → IIe), and ChrX:6664 G→C (resulting in the substitution Gln → His). Three of the individuals had normal G6PD activity and the others were G6PD deficient (Table 6).
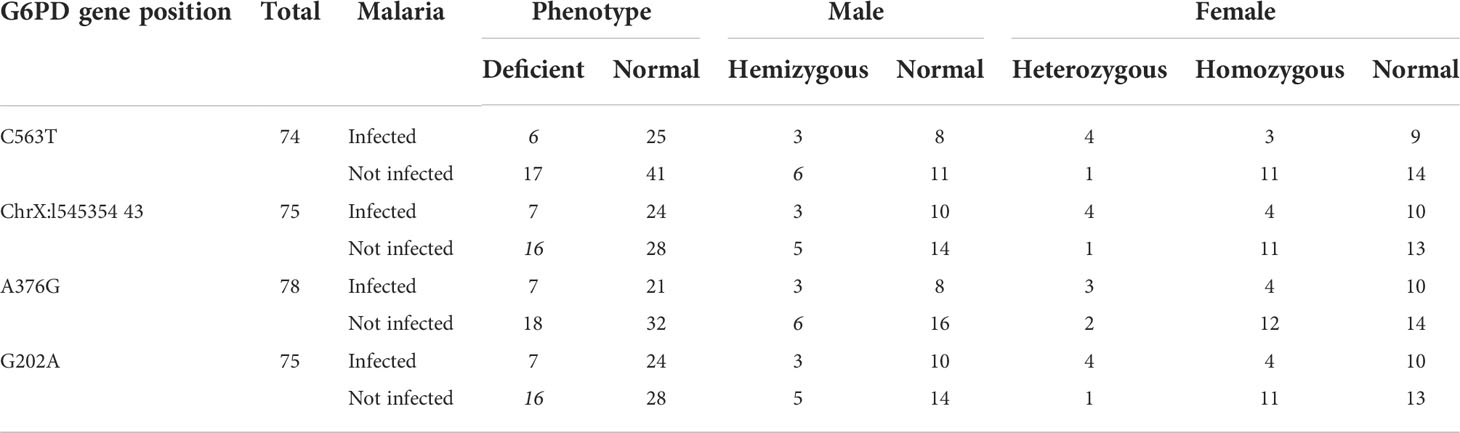
Table 5 Distributions of malaria cases by gender and G6PD genotype among malaria suspected febrile individuals in Bonga southwestern Ethiopia.

Table 6 Distribution of the three new G6PD genotypes where mutations were detected among male and female individuals in Bonga, southwestern Ethiopia.
Discussion
The Ethiopian government aims to eliminate malaria by 2030 (30) but submicroscopic infections, relapses of P. vivax, and residual transmission pose major challenges to this goal (31, 32). Submicroscopic malaria infections are hidden parasite reservoirs as infected individuals were not identified and treated with antimalarial drugs. They can serve as the source of infection and contribute to transmission in the community and/or other malaria-free areas (33–35), thereby hindering malaria control and elimination. The present study showed that submicroscopic infections were high among malaria suspected febrile patients in the southwestern part of Ethiopia. The prevalence of submicroscopic malaria was comparable to previous studies in low-endemic settings such as Malo in southwestern Ethiopia, which reported a prevalence of 9.7% (36, 37); but much lower than that in southwestern Saudi Arabia, Senegal, and Uganda where submicroscopic infection was more than 23% (38–40). According to a systematic review of P. falciparum prevalence measured by both microscopy and PCR, the prevalence of infection by microscopy was on average 50.8% of that by PCR (30). This substantial discordance highlights the unreliability of microscopic diagnosis particularly in low transmission settings where infections are sparse and parasitemia is low. Among various age groups, individuals aged 15 - 50 were found with significantly higher risk for malaria infection than the others. Such pattern could be related to underlying medical conditions of the study patients, recent travel to high transmission areas, occupation, bednet usage, and/or relapse from previous infections (41).
Across the globe, the prevalence of G6PD deficiency varies by countries, regions, and localities, ranging from 5% to 30% in the Middle East, certain parts of the Mediterranean, and Asia (15,) and 0.5 to 4% in Gambella, Oromiya, and Benishangule Gumuz of Ethiopia (42, 43). The frequency of G6PDd among malaria suspected individuals in Bonga areas as well as other parts of southwestern Ethiopia (42) was relatively low compared to other parts of Africa (as high as 35%) (44, 45). There are several reasons for such differences. In Ethiopia, multiple ethnic groups coexist and G6PDd varies by ethnic groups, ranging from 1.4 to 14.3% in the Nuer and Anuak (southwest Ethiopia) and Aregobas (northeast Ethiopia) regions (42, 43). In southern European countries, such as Greece and Italy, G6PDd prevalence ranged from 1-30% and 0-3% (46), respectively, much lower than that reported in Cameroon, Yemen, and Saudi Arabia (7.9-9%) (17, 18, 38). Based on our findings, the frequency of G6PDd was higher in females (7.9%) than in males (5.6%), similar to another study in southeast Iran that showed G6PDd in 18.9% of females and 10.8% of males (47). There could be several reasons for such a difference, such as the fact that X-chromosome inactivation tends to be more prevalent in elderly women than in young women. Our data indicated that nearly half of the G6PDd females were between 40-50 years old, and the remaining were 18 years old or younger. The higher frequency of X-chromosome inactivation in females may associate with an increase in malaria risk (48).
Several G6PD variants have been previously identified, and the severity of the disorder varies from variant to variant. There are two major types of G6PD variants: the Mediterranean variant that occurs throughout Europe, Asia, and northern India, and is considered as the most severe form (49). The other type is the African variant, which is prevalent among Africans and is a mild form (50). Though it is yet unclear which of these G6PD variants is particularly associated with hemolytic anemia, our analyses did not indicate any association between G6PD mutations and hemolytic anemia in the Ethiopian P. vivax samples. There is a number of factors that determine the level of hemolysis, including the age of the RBC (with lower G6PD activity in older cells), the total dose of primaquine, and the type of G6PD variants (51). Previous studies have been shown that individuals with a non-severe variant of the G6PD gene are more likely to experience reduced hemoglobin level when the old RBCs with low G6PD activity are lysed upon exposure to primaquine (52). As a result, erythropoiesis increases and the lysed red blood cells are replaced by younger ones with higher levels of G6PD activity, which help stabilize and reverse anemia, as well as avoid further drop of hemoglobin after additional doses of PQ (52). By contrast, RBCs affected by a severe G6PD variant will display hemolysis regardless of whether RBCs are young or old; sustained exposure to an oxidizing agent will result in hemolysis. Continuous uptake of PQ by these patients will result in severe anemia and can be fatal if not treated promptly.
Among G6PD deficient individuals, the number of Plasmodium infection was comparable to G6PD normal individuals. Variation in G6PD level had no effect on the number of clinical cases of malaria. Previous study suggested that low level G6PD may increase sensitivity of P. vivax to oxidative stress (44). P. vivax parasites preferred to invade younger erythrocytes that possess high levels of G6PD enzyme (53, 54). Though enzyme levels are diminished in older erythrocytes, these erythrocytes are less preferable for P. vivax parasite invasion. Thus, there could be a protective effect of G6PD deficiency against parasite invasion (55, 56). The African form of G6PD deficiency has been recently shown to decrease malaria cases by 46% to 58% in endemic regions (57). Ninokata et al. (2006) (58) investigated 345 healthy adults and found that 10% of them were G6PD deficient but none had malaria infection (59). It has been shown that some G6PD variants could have no effect on the number of clinical cases of malaria but on P. vivax parasitemia (60). The mechanism by which this protection is conferred is still unclear. In this study, we found three novel non-synonymous mutations in exon 2 of the G6PD gene in both G6PD normal and deficient individuals. Future study should investigate the prevalence and impact of these mutations on malarial infections.
Conclusion
This study shows a higher prevalence of submicroscopic infections among individuals who did not use ITN and a significantly higher risk of malaria infections in adults. Submicroscopic malaria infections are hurdles against ongoing malaria control programs as they influence transmission dynamics and impose negative health and economic impacts. Sensitive diagnostic methods are needed to improve detection and treatment of these infections in health facilities especially in rural areas of Ethiopia. The use of a single low dose of primaquine to reduce malaria transmission and relapse has been recently adopted by the Ethiopian Federal Ministry of Health as a radical treatment regime for P. vivax in 239 districts that are targeted for elimination. This treatment regime requires wide-scale G6PD assessment given that Bonga, one of the targeted areas, showed a high prevalence of G6PD deficiency but no G6PD testing is available. This could pose a high hemolytic risk to malaria patients who are G6PD deficient and administered with primaquine. It is imperative to re-evaluate the implementation of this treatment regime and provide resources for G6PD assessment broadly across the country to mitigate the potential risk. The prevalence and clinical significance of novel mutations identified in exon 2 of the G6PD gene among G6PD deficient individuals merit further investigations.
Data availability statement
The original contributions presented in the study are included in the article/Supplementary Materials. Further inquiries can be directed to the corresponding authors.
Ethics statement
The studies involving human participants were reviewed and approved by Institutional Review Board (IRB) of Institute of Health, Jimma University. Written informed consent to participate in this study was provided by the participants’ legal guardian/next of kin.
Author contributions
BRA, AB, DAJ, EL, and DY conceived and designed the study; BRA, FB, AA collected the samples; BRA, DK, KP, LW, SH, KL, EL, and DY collected and analyzed the data; BRA, DK, EL, and DY wrote and edited the paper. All authors have read and approved the final manuscript.
Funding
This research was funded by National Institutes of Health, grant number R01 AI162947.
Acknowledgments
We are greatly indebted to technicians and the staffs from Bonga Hospital for sample collection, the communities and Kefa zone health office for their support and willingness to participate in this research.
Conflict of interest
The authors declare that the research was conducted in the absence of any commercial or financial relationships that could be construed as a potential conflict of interest.
Publisher’s note
All claims expressed in this article are solely those of the authors and do not necessarily represent those of their affiliated organizations, or those of the publisher, the editors and the reviewers. Any product that may be evaluated in this article, or claim that may be made by its manufacturer, is not guaranteed or endorsed by the publisher.
Supplementary material
The Supplementary Material for this article can be found online at: https://www.frontiersin.org/articles/10.3389/fitd.2022.966930/full#supplementary-material
Abbreviations
G6PD, Glucose-6-phosphate dehydrogenase; PQ, Primaquine; CQ, Chloroquine; AMM, Adjusted Male Median.
References
1. Legesse Y, Tegegn A, Belachew T, Tushune K. Knowledge, attitude and practice about malaria transmission and its preventive measures among households in urban areas of assosa zone, Western ethiopia. Ethiop J Heal Dev (2007) 21(2):157–65. doi: 10.4314/ejhd.v21i2.10044
2. Howard CT, McKakpo US, Quakyi IA, Bosompem KM, Addison EA, Sun K, et al. Relationship of hepcidin with parasitemia and anemia among patients with uncomplicated plasmodium falciparum malaria in Ghana. Am J Trop Med Hyg (2007) 77(4):623–6. doi: 10.4269/AJTMH.2007.77.623
3. Ghebreyesus TA, Haile M, Witten KH, Getachew A, Yohannes M, Lindsay SW, et al. Household risk factors for malaria among children in the Ethiopian highlands. Trans R Soc Trop Med Hyg (2000) 94(1):17–21. doi: 10.1016/s0035-9203(00)90424-3
4. Peterson I, Borrell LN, El-Sadr W, Teklehaimanot A. Individual and household level factors associated with malaria incidence in a highland region of Ethiopia: a multilevel analysis. Am J Trop Med Hyg (2009) 80(1):103–11. doi: 10.1186/s12936-017-2048-9
5. Negash K, Kebede A, Medhin A, Argaw D, Babaniyi O, Guintran JO, et al. Malaria epidemics in the highlands of Ethiopia. East Afr Med J (2005) 82(4):186–92. doi: 10.4314/eamj.v82i4.9279
6. Guthmann JP, Bonnet M, Ahoua L, Dantoine F, Balkan S, van Herp M, et al. Death rates from malaria epidemics, Burundi and Ethiopia. Emerging Infect diseases (2007) 13(1):140–3. doi: 10.3201/eid1301.060546
8. Murray CJL. Choosing indicators for the health-related SDG targets. Lancet (2015) 386(10001):1314–7. doi: 10.1016/S0140-6736(15)00382-7
9. Abraha MW, Nigatu TH. Modeling trends of health and health related indicators in Ethiopia (1995-2008): a time-series study. Health Res Policy Sys (2009) 7:29. doi: 10.1186/1478-4505-7-29
11. Nayyar GM, Breman JG, Newton PN, Herrington J. Poor-quality antimalarial drugs in southeast Asia and sub-Saharan Africa. Lancet Infect Dis (2012) 12(6):488–96. doi: 10.1016/S1473-3099(12)70064-6.
12. Lin JT, Saunders DL, Meshnick SR. The role of submicroscopic parasitemia in malaria transmission: what is the evidence? Trends Parasitol (2014) 30(4):183–90. doi: 10.1016/j.pt.2014.02.004
13. Nkhoma ET, Poole C, Vannappagari V, Hall SA, Beutler E. The global prevalence of glucose-6-phosphate dehydrogenase deficiency: A systematic review and meta-analysis. Blood Cells Mol Dis (2009) 42(3):267–78. doi: 10.1016/j.bcmd.2008.12.005
14. Olusanya BO, Osibanjo FB, Slusher TM. Risk factors for severe neonatal hyperbilirubinemia in low and middle-income countries: A systematic review and meta-analysis. PloS One (2015) 10(2):e0117229. doi: 10.1371/journal.pone.0117229
15. Howes RE, Battle KE, Satyagraha AW, Baird JK, Hay SI. G6PD deficiency: global distribution, genetic variants and primaquine therapy. Adv Parasitol (2013) 81:133–201. doi: 10.1016/B978-0-12-407826-0.00004-7
16. World Health Organization. Policy brief on single-dose primaquine as gametocytocide in plasmodium falciparum malaria. World Health Organization (2015). Available at: https://apps.who.int/iris/handle/10665/338498.
17. Al-Nood HA, Bazara FA, Al-Absi R, Al-Habori M. Glucose-6-Phosphate dehydrogenase deficiency among Male blood donors inSana’a city, Yemen. Oman Med J (2012) 27(1):46–9. doi: 10.5001/omj.2012.09
18. Lauden SM, Chongwain S, Achidi A, Helm E, Cusick SE, Krug A, et al. Prevalence of glucose-6-phosphate dehydrogenase deficiency in cameroonian blood donors. BMC Res Notes (2019) 12:195. doi: 10.1186/s13104-019-4226-z
19. Cheesbrough M. District laboratory practice in tropical countries–part 2. 2nd Edition. New York: Cambridge University Press (2006). doi: 10.1017/CBO9780511543470
20. Gillespie SH, Pearson RD. Principles and practice of clinical parasitology principles and practice of clinical parasitology. New York: ISBN 0-471-97729-2 (2001). P775, 616.9.
21. Ogbodo SO, Nwagha UI, Okaka AN, Ogenyi SC, Okoko RO, Nwagha TU. Malaria parasitaemia among pregnant women in a rural community of eastern nigeria; need for combined measures. Niger J Physiol Sci (2009) 24(2):95–100. doi: 10.4314/njps.v24i2.52923
22. Walsh PS, Metzger DA, Higuchi R. Chelex 100 as a medium for simple extraction of DNA for PCR-based typing from forensic material. Biotechniques (1991) 10(4):506–13. doi: 10.2144/000114018
23. Snounou G, Viriyakosol S, Zhu XP, Jarra W, Pinheiro L, do Rosario VE, et al. High sensitivity of detection of human malaria parasites by the use of nested polymerase chain reaction. Mol Biochem Parasitol (1993) 61(2):315–20. doi: 10.1016/0166-6851(93)90077-b
24. Johnston SP, Pieniazek NJ, Xayavong MV, Slemenda SB, Wilkins PP, da Silva AJ. PCR as a confirmatory technique for laboratory diagnosis of malaria. J Clin Microbiol (2006) 44(3):1087–9. doi: 10.1128/JCM.44.3.1087-1089.2006
25. Lo E, Yewhalaw D, Zhong D, Zemene E, Degefa T, Tushune K, et al. Molecular epidemiology of plasmodium vivax and plasmodium falciparum malaria among Duffy-positive and Duffy-negative populations in Ethiopia. Malar J (2015) 14:84. doi: 10.1186/s12936-015-0596-4
26. World Health Organization. Testing for G6PD deficiency for safe use of primaquine in radical cure of p. vivax and p. ovale: Policy brief. Geneva, Switzerland: World Health Organization (2016).
27. World Health Organization. In vitro diagnostics medical devices to identify glucose-6-phosphate dehydrogenase (G6PD) activity. Geneva, Switzerland: World Health Organization (2016).
28. Lo E, Zhong D, Raya B, Pestana K, Koepfli C, Lee M-C, et al. Prevalence and distribution of G6PD deficiency: Implication for the use of primaquine in malaria treatment in Ethiopia. Malar J (2019) 18:340. doi: 10.1186/s12936-019-2981-x
29. Clustal X2.1 is a windows interface for the multiple sequence alignment program. Available at: https://clustalx.software.informer.com/2.1/https://bioedit.software.informer.com/7.2/.
30. Okell LC, Ghani AC, Lyons E, Drakeley CJ. Submicroscopic infection in plasmodium falciparum-endemic populations: A systematic review and meta-analysis. J Infect Dis (2009) 200(10):1509–17. doi: 10.1086/644781
31. Graves PM, Richards FO, Ngondi J, Emerson PM, Shargie EB, Endeshaw T, et al. Individual, household and environmental risk factors for malaria infection in amhara, oromia and SNNP regions of Ethiopia. Trans R Soc Trop Med Hyg (2009) 103(12):1211–20. doi: 10.1016/j.trstmh.2008.11.016
32. Minucci A, Gentile L, Zuppi C, Giardina B, Capoluongo E. Rapid and simple identification of the commonest glucose-6-phosphate dehydrogenase (G6PD) Italian mutations: From DNA extraction to genotyping. Clin Chim Acta (2012) 413(11-12):1018–9. doi: 10.1016/j.cca.2012.01.032
33. Idris Z, Chan C, Kongere J, Gitaka J, Logedi J, Omar A, et al. High and heterogeneous prevalence of asymptomatic and Sub-microscopic malaria infections on islands in lake Victoria, Kenya. Sci Rep (2016) 6:36958. doi: 10.1038/srep36958
34. Kaura T, Kaur J, Sharma A, Dhiman A, Pangotra M, Upadhyay AK, et al. Prevalence of submicroscopic malaria in low transmission state of punjab: A potential threat to malaria elimination. J Vector Borne Dis (2019) 56(1):78–84. doi: 10.4103/0972-9062.257780
35. Arwati H, Yotopranoto S, Rohmah EA, Syafruddin D. Submicroscopic malaria cases play role in local transmission in trenggalek district, East Java province, Indonesia. Malar J (2018) 17:2. doi: 10.1186/s12936-017-2147-7
36. Tadesse FG, van den Hoogen L, Lanke K, Schildkraut J, Tetteh K, Aseffa A, et al. The shape of the iceberg: quantification of submicroscopic plasmodium falciparum and plasmodium vivax parasitaemia and gametocytaemia in five low endemic settings in Ethiopia. Malar J (2017) 16(1):99. doi: 10.1186/s12936-017-1749-4
37. Baum E, Sattabongkot J, Sirichaisinthop J, Kiattibutr K, Jain A, Taghavian O, et al. Common asymptomatic and submicroscopic malaria infections in Western Thailand revealed in longitudinal molecular and serological studies: a challenge to malaria elimination. Malar J (2016) 15:333. doi: 10.1186/s12936-016-1393-4
38. Hawash Y, Ismail K, Alsharif K, Alsanie W. Malaria prevalence in a low transmission area, jazan district of southwestern Saudi Arabia. Korean J Parasitol (2019) 57(3):233–42. doi: 10.3347/kjp.2019.57.3.233
39. Vallejo AF, Chaparro PE, Benavides Y, Álvarez Á, Quintero JP, Padilla J, et al. High prevalence of sub-microscopic infections in Colombia. Malar J (2015) 14:201. doi: 10.1186/s12936-015-0711-6
40. Katrak S, Nayebare P, Rek J, Arinaitwe E, Nankabirwa JI, Kamya M, et al. Clinical consequences of submicroscopic malaria parasitaemia in Uganda. Malar J (2018) 17(1):67. doi: 10.1186/s12936-018-2221-9
41. Schwartz E, Sadetzki S, Murad H, Raveh D. Age as a risk factor for severe Plasmodium falciparum Malaria in nonimmune patients. Clin Infect Dis (2001) 33(10):Pages 1774–1777. doi: 10.1086/322522
42. Tsegaye A, Golassa L, Mamo H, Erko B. Glucose-6-phosphate dehydrogenase deficiency among malaria suspects attending gambella hospital, southwest Ethiopia. Malar J (2014) 13:438. doi: 10.1186/1475-2875-13-438
43. Assefa A, Ali A, Deressa W, Tsegaye W, Abebe G, Sime H, et al. Glucose-6-phosphate dehydrogenase (G6PD) deficiency in Ethiopia: Absence of common African and Mediterranean allelic variants in a nationwide study. Malar J (2018) 17:388. doi: 10.1186/s12936-018-2538-4
44. World Health Organization. Glucose-6-phosphate dehydrogenase deficiency. WHO working group. Bull World Health Organ (1989) 67(6):601–11. Available at: https://apps.who.int/iris/handle/10665/264721.
45. Sathupak S, Leecharoenkiat K, Kampuansai J. Prevalence and molecular characterization of glucose-6-phosphate dehydrogenase deficiency in the lue ethnic group of northern Thailand. Sci Rep (2021) 11:2956. doi: 10.1038/s41598-021-82477-w
46. Missiou-Tsagaraki S. Screening for glucose-6-phosphate dehydrogenase deficiency as a preventive measure: Prevalence among 1,286,000 Greek newborn infants. J Pediatr (1991) 119(2):293–9. doi: 10.1016/s0022-3476(05)80747-4
47. McDonagh EM, Thorn CF, Bautista JM, Youngster I, Altman RB, Klein TE. PharmGKB summary: very important pharmacogene information for G6PD. Pharmacogenetics Genomics (2012) 22(3):219–28. doi: 10.1097/FPC.0b013e32834eb313
48. Au WY, Lam V, Pang A, Lee WM, Chan JL, Song YQ, et al. Glucose-6-phosphate dehydrogenase deficiency in female octogenarians, nanogenarians, and centenarians. J Gerontol A Biol Sci Med Sci (2006) 61(10):1086–9. doi: 10.1093/gerona/61.10.1086
49. Beutler E. G6PD: population genetics and clinical manifestations. Blood Rev (1996) 10(1):45–52. doi: 10.1016/s0268-960x(96)90019-3
50. Ashley EA, Recht J, White NJ. Primaquine: The risks and the benefits. Malar J (2014) 13:418. doi: 10.1186/1475-2875-13-418
51. John GK, Douglas NM, von Seidlein L, Nosten F, Baird JK, White NJ, et al. Primaquine radical cure of plasmodium vivax: A critical review of the literature. Malar J (2012) 11:280. doi: 10.1186/1475-2875-11-280
52. Ashley EA, Recht J, White NJ. Primaquine: The risks and the benefits. Malar J (2014) 13:418. doi: 10.1186/1475-2875-13-418
53. Guindo A, Fairhurst RM, Doumbo OK, Wellems TE, Diallo DA. X-Linked G6PD deficiency protects hemizygous males but not heterozygous females against severe malaria. PLoS Med (2007) 4(3):e66. doi: 10.1371/journal.pmed.0040066
54. Brown M. Manson's tropical diseases. Lancet Infect Dis (2009) 9(7):407–8. doi: 10.1016/S1473-3099(09)70170-7
56. Allison AC, Clyde DF. Malaria in African children with deficient erythrocyte glucose-6-phosphate dehydrogenase. Br Med J (1961) 1(5236):1346–9. doi: 10.1136/bmj.1.5236.1346
57. Mbanefo EC, Ahmed AM, Titouna A, Elmaraezy A, Trang NT, Phuoc Long N, et al. Association of glucose-6-phosphate dehydrogenase deficiency and malaria: a systematic review and meta-analysis. Sci Rep (2017) 7:45963. doi: 10.1038/srep45963
58. Ninokata A, Kimura R, Samakkarn U, Settheetham-Ishida W, Ishida T. Coexistence of five G6PD variants indicates ethnic complexity of phuket islanders, southern Thailand. J Hum Genet (2006) 51(5):424–8. doi: 10.1007/s10038-006-0380-y
59. Ruwende C, Khoo SC, Snow RW, Yates SN, Kwiatkowski D, Gupta S, et al. Natural selection of hemi- and heterozygotes for G6PD deficiency in Africa by resistance to severe malaria. Nature (1995) 376(6537):246–9. doi: 10.1038/376246a0
Keywords: submicroscopic, Plasmodium infection, malaria elimination, G6PD deficiency, Southwestern Ethiopia
Citation: Abagero BR, Kepple D, Pestana K, Witherspoon L, Biruksew A, Adanew A, Baharu F, Hansel S, Lopez K, Janies DA, Lo E and Yewhalaw D (2022) Low density Plasmodium infections and G6PD deficiency among malaria suspected febrile individuals in Ethiopia. Front. Trop. Dis 3:966930. doi: 10.3389/fitd.2022.966930
Received: 11 June 2022; Accepted: 30 September 2022;
Published: 21 October 2022.
Edited by:
Moses Samje, The University of Bamenda, CameroonReviewed by:
Carol Hunja, South Eastern Kenya University, KenyaSusanta Kumar Ghosh, National Institute of Malaria Research (ICMR), India
Copyright © 2022 Abagero, Kepple, Pestana, Witherspoon, Biruksew, Adanew, Baharu, Hansel, Lopez, Janies, Lo and Yewhalaw. This is an open-access article distributed under the terms of the Creative Commons Attribution License (CC BY). The use, distribution or reproduction in other forums is permitted, provided the original author(s) and the copyright owner(s) are credited and that the original publication in this journal is cited, in accordance with accepted academic practice. No use, distribution or reproduction is permitted which does not comply with these terms.
*Correspondence: Delenasaw Yewhalaw, delenasawye@yahoo.com; Eugenia Lo, Eugenia.Lo@uncc.edu