- 1Department of Veterinary Parasitology and Entomology, University of Maiduguri, Maiduguri, Nigeria
- 2Department of Preventive Veterinary Medicine and Animal Health, Faculty of Veterinary Medicine, University of São Paulo, São Paulo, Brazil
- 3Laboratory of Bacteriology, Division of Intramural Research, National Institute of Allergy and Infectious Diseases - National Institutes of Health (NIAID-NIH), Hamilton, MT, United States
Tick-borne rickettsioses are emerging and re-emerging diseases of public health concern caused by over 30 species of Rickettsia. Ticks are obligate hematophagous arthropods with over 700 species of Ixodid ticks known worldwide. The escalating geographical dispersal of tick vectors and concomitant increase in the incidences of tick-borne diseases have fueled interest in the ecology of tick-borne pathogens. This review focuses on aspects of the Rickettsia pathogen, including biology, taxonomy, phylogeny, genetic diversity, epidemiology of the disease, and the role of vertebrate host in the perpetuation of rickettsioses in Africa. Our review also highlights some of the species of Rickettsia that are responsible for disease, the role of tick vectors (both hard and soft ticks) and the species of Rickettsia associated with diverse tick species across the continent. Additionally, this article emphasizes the evolutionary perspective of rickettsiae perpetuation and the possible role of amplifying vertebrate host and other small mammals, domestic animals and wildlife in the epidemiology of Rickettsia species. We also specifically, discussed the role of avian population in the epidemiology of SFG rickettsiae. Furthermore, we highlighted tick-borne rickettsioses among travelers due to African tick-bite fever (ATBF) and the challenges to surveillance of rickettsial infection, and research on rickettsiology in Africa. Our review canvasses the need for more rickettsiologists of African origin based within the continent to further research towards understanding the biology, characterization, and species distribution, including the competent tick vectors involved in their transmission of rickettsiae across the continent in collaboration with established researchers in western countries. We further highlighted the need for proper funding to encourage research despite competing demands for resources across the various sectors. We finalize by discussing the similarities between rickettsial diseases around the world and which steps need to be taken to help foster our understanding on the eco-epidemiology of rickettsioses by bridging the gap between the growing epidemiological data and the molecular characterization of Rickettsia species.
Introduction
Vector-borne diseases (VBDs) comprise an expanded group of diseases that are caused by a collection of pathogens transmitted by arthropod vectors, such as fleas, mosquitoes, ticks, and sand flies (1). In recent decades, vector-borne zoonotic pathogens have gained increasing attentions globally, with several reports of emerging and/or re-emerging pathogens registered in both vertebrate and invertebrate hosts. Some vector-borne bacterial pathogens are responsible for acute febrile illnesses, with potentially fatal or severe outcomes. Vector-borne rickettsiosis (VBR) is a disease caused by a group of gram-negative bacteria transmitted by lice, mites, fleas, and ticks (2). The genus Rickettsia (Rickettsiales: Rickettsiaceae) comprises obligate intracellular Alphaproteobacteria responsible for diseases that affect diverse eukaryotic hosts (3). Rickettsial species differ considerably with respect to their association with arthropods, pathophysiology, behavior of the vector to infection, and disease outcome (4). In the tropical and subtropical regions of the world, VBD is most often prevalent and affects poor communities with the least access to healthcare, with significant health threats to the inhabitants of these areas. The life cycle of VBR is complex, involving hematophagous arthropod vectors and vertebrate hosts, with the former also serving as the main reservoirs. The majority of emerging VBR are tick-borne and are caused by rickettsial species belonging to the spotted fever group (SFG) (5).
Ticks are hematophagous arthropods, second only to mosquitoes, which are the leading vectors of human pathogens (6). It is worth noting that ticks from all regions are carriers of specific pathogens that may be unknown to healthcare providers in other parts of the world. Numerous tick species of the genera Hyalomma (Hy), Amblyomma (Am), Rhipicephalus (Rh), Ixodes (I), Haemaphysalis (Hae), and Dermacentor (D) have been implicated as vectors of rickettsial pathogens (7–9). Hard ticks (Acari: Ixodidae) are considered the most important arthropod group that harbors rickettsial organisms (10). At least 27 of the more than 30 published rickettsial species are intricately linked with hard ticks (11). Approximately 17 of these species have been isolated or detected in African arthropods (12, 13). Rickettsiae associated with ticks are usually transmitted vertically (transovarial transmission) from engorged females to their offspring. This phenomenon is speculated to be the single most important mechanism driving permanent perpetuation of rickettsiae among tick populations (14). Conversely, some species of Rickettsia can deleteriously affect tick population size by decreasing female reproductive performance (15–17). Long-term perpetuation of rickettsial species largely depends on the availability of amplifying vertebrate hosts, which serve as infection sources (horizontal transmission), thereby creating new cohorts of Rickettsia-infected ticks (18, 19). Ticks can spread into any territory that provides favorable conditions for their survival; under optimal temperature and relative humidity, ticks can reproduce and spread their bacterial pathogens.
Tick-borne rickettsioses (TBRs) are distributed worldwide and are considered among the most frequently identified etiologies of systemic febrile illness, after malaria, among international travelers. Therefore, they play an important role in public health (20, 21). The epidemiology of any type of rickettsial disease transmitted by ticks mirrors the ecological distribution and seasonal activity of implicating tick vectors and their hosts involved in the transmission of these pathogens. Additionally, human behaviors that expose people to tick attachment and subsequent infections also play a role (22). Each tick species has a particular set of optimal environmental conditions and biotopes that ultimately influences the geographical distribution of the ticks; therefore, the type of TBR, as only a handful of tick species, is distributed globally.
Rickettsioses are emerging and re-emerging VBD of global importance (11). Several SFG Rickettsia species associated with human and animal diseases have been reported worldwide (23). These species include Rickettsia africae [the etiological agent of African tick bite fever (ATBF)], Rickettsia conorii [Mediterranean spotted fever (MSF)], Rickettsia aeschlimannii (innominate rickettsioses), and Rickettsia sibirica (lymphangitis-associated rickettsioses) (24). There are also non-pathogenic endosymbiont species in Rickettsia. SFG rickettsial pathogens are transovarially transmitted depending on vertebrate hosts as pathogen reservoirs (24). Thus, ticks are regarded not only as efficient vectors but also as competent reservoirs. Clinical signs may include fever and rash that may be maculopapular, vesicular, or purpuric in severe cases, and may be absent, as seen in Rocky Mountain spotted fever (RMSF) (6). These rickettsial diseases can be mild, severe, or even fatal. One of the hallmarks of most SFG rickettsioses is the typical inoculation eschar at the site of tick bite (25).
In the last decades, some species of Rickettsia that were otherwise thought to be not prevalent and distributed in Africa have been reported by detection of their genetic material using molecular based diagnostics including sequencing. Also, rickettsioses are seldom considered when patients with undifferentiated febrile illness are evaluated, and due to the overlapping symptoms with other illness endemic in parts of Africa such as malaria, yellow and dengue fevers, clinical diagnosis is difficult in the absence of confirmatory laboratory tests. Therefore, it is expedient that this information is brought to the front-burner to enlighten healthcare providers and government on this emerging but neglected disease. Also, they lack adequate surveillance of ticks, livestock, wildlife, and companion animals for tick-borne zoonotic pathogens, specifically Rickettsia species. Aside from the Mediterranean region comprising North Africa, most of the sub-Saharan African (SSA) nations do not have dedicated research centers or institutes for research on Rickettsia. Many studies conducted in most African countries are based on scientific collaboration involving ferrying of samples to developed western nations for molecular characterization and confirmation. Due to underfunding and/or paucity of well-trained manpower, surveillance is lagging compared to other continents of the world. We hope the scientific content of this review will further bring attention to the urgent need of budgetary allocations to fund research and clinical diagnostics to better improve our scientific understanding and healthcare associated with these important diseases. In a nutshell, this review aims to gather, summarize, and present an updated knowledge about epidemiological aspects of members of the genus Rickettsia with particular emphasis on the SFG in Africa.
Methods
We performed a deep bibliographic search of the CABS Abstracts database (accessed 29th November 2021). Keywords used for the search were “Rickettsia,” “Ticks,” and “Africa”. These keywords were mapped to the subjects’ headings. In addition, we used the Boolean operator “AND” in combination with these words as the major focus in our review, which focused on the epidemiology and relationship between ticks and rickettsial infection, with emphasis on SFG rickettsiae in Africa. The search yielded 216 references. The list of papers was checked manually by screening the title and abstract of each reference as part of preliminary filtering. Papers that did not directly address the topic were excluded. All relevant papers were downloaded and subjected to full-text evaluation, and the relevant text was extracted from each paper and used for preparing this review. Text word searches were performed on multiple additional terms that were tailored to specific questions. We also checked the reference lists of the included articles and searched for additional relevant citations. In some instances, textbook references were used to support statements considered as known knowledge in the field. The selected articles were in English only. The 200 papers used in this review were cut across several article types, including original research, short communication, letters to the editor, review(s), and opinion papers. The earliest article was published in 1910, whereas the most recent article was published in 2022. The geographical origins of the bulk of the papers covering the epidemiological aspects of the review were prevalence studies, principally conducted across all regions of Africa. Additionally, we observed that most of the laboratory research work might have been carried out in laboratories in developed countries after field surveys in Africa. Therefore, the authorship of published papers cut across both Africans and experts in Western nations. Some topics covered in our review, but not exclusive to the published papers used in writing this review on spotted fever group rickettsiae and associated tick vectors, include genetics, epidemiology, diagnostics, vectorial capacity, and clinical and travel medicine.
Rickettsiae properties, taxonomy, phylogeny, and genotyping
With sizes ranging from 0.3–2.0 µm, rickettsiae are strictly intracellular bacteria and they multiply inside the cytoplasm of eukaryotic host cells by binary fission (26). The genus Rickettsia can be divided into four different phylogenetic groups: (i) the spotted fever group (SFG), with over 20 known species, all closely associated with ticks; species of Rickettsia belonging to this group affect human health. Examples within the SFG include R. conorii, Rickettsia rickettsii, R. africae, R. sibirica, and Rickettsia parkeri. (ii) Typhus group (TG), containing two species, Rickettsia typhi and Rickettsia prowazekii, which are transmitted by fleas and louses and cause diseases that affect human health. (iii) The transitional group (TRG) contains rickettsial pathogens responsible for human disease and is primarily associated with diverse arthropods, including ticks (Rickettsia australis), fleas (Rickettsia felis), and mites (Rickettsia akari). (iv) The ancestral group (AG), represented by two tick-associated organisms, namely Rickettsia bellii and Rickettsia canadensis (3, 11, 27).
To date, the genus Rickettsia comprises over 30 formally recognized species, among other uncharacterized strains. Taxonomic guidelines for species identification and description of new isolates have been proposed, utilizing sequences of the 16S rRNA (rrs) gene and four protein-coding genes: gltA, ompA, ompB, and gene D (28). Rickettsiae phylogeny has enabled precise identification and classification of rickettsiae. Based on 16S rRNA (rrs) gene sequences, the genus Rickettsia belongs to group 1 of a subclass of Proteobacteria (29). High levels of conservation in the 16S rRNA gene sequences among members of the genus Rickettsia constitute a setback for significant inferences about intragenus phylogeny (30). The genes encoding outer membrane proteins, surface cell antigens (ompA, ompB, sca1, sca2, and sca4), and citrate synthase (gltA) have been regarded as sufficiently variable to infer dependable phylogenetic relationships among rickettsiae (31–34). Furthermore, the availability of complete genome sequences has enabled phylogenomic approaches, which have provided clarity to some extent regarding the phylogenetic relationships among rickettsiae (35, 36). Large amounts of data have been generated owing to genomic sequencing, and these data are useful for evaluating the reliability of recognized groups. Furthermore, by sequencing rickettsial species, phylogenetic studies should be reinforced.
Rickettsial infection in Africa
Several Rickettsia species amplified in ticks collected from livestock and domestic animals across different geographical regions of Africa are summarized in Table 1. Many Rickettsia species have been shown to cause acute infectious diseases in humans, as described below.
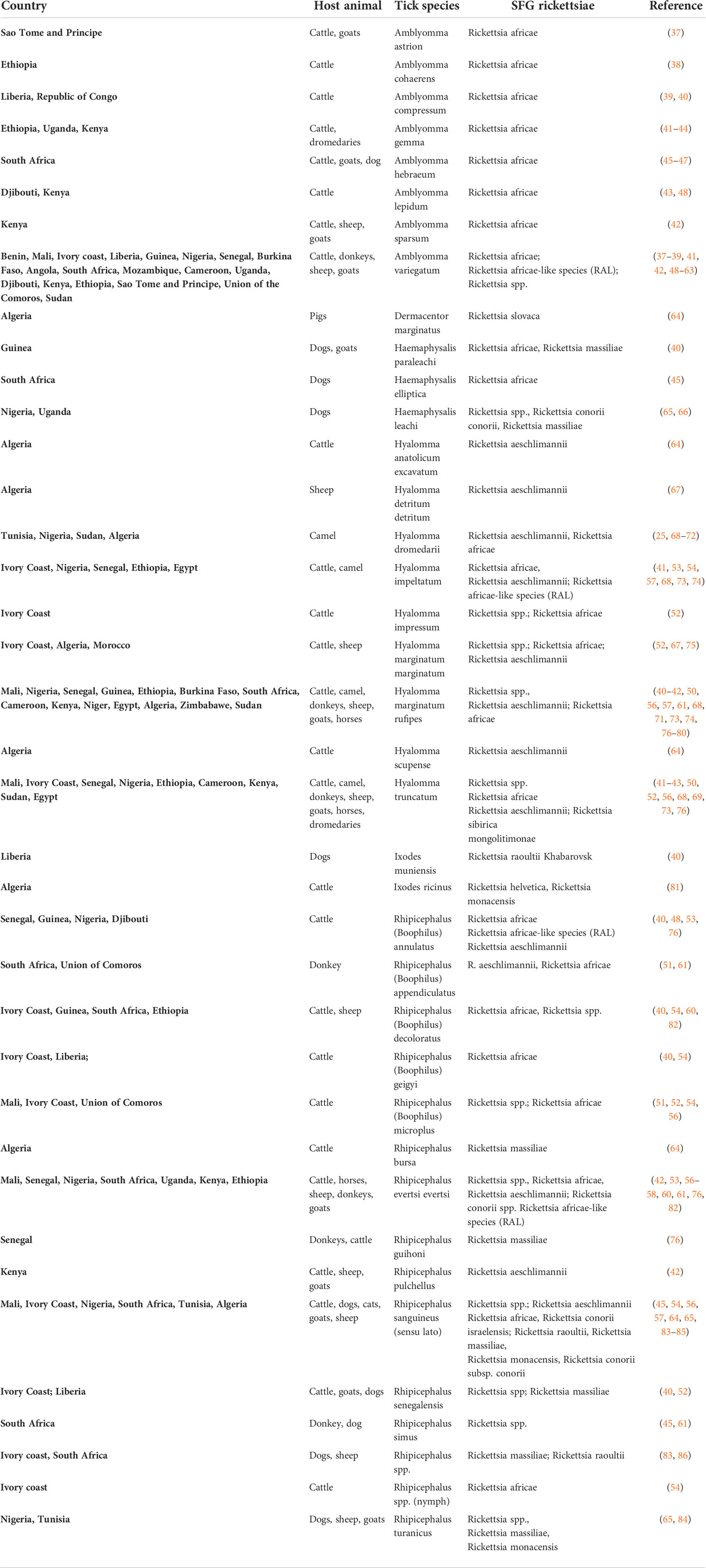
Table 1 Overview of Spotted Fever Group Rickettsia detected in ticks collected from livestock and domestic animals across Africa.
African tick-bite fever
Rickettsia africae is the etiological agent of ATBF and is transmitted via the bite of ticks belonging to the genus Amblyomma (specifically, Am. hebraeum and Am. variegatum) (87–89). These tick species are common among rural communities in parts of SSA and pose a threat to local populations. In Zimbabwe, the annual incidence rate of ATBF is estimated to be approximately 60–80 cases per 100,000, where the tick vector is endemic (9). Nonetheless, the most documented cases are among travelers from Europe, while reports from native populations are scarce. The ATBF was discovered by Pijper in 1930 (90). However, the isolate was lost, but it was subsequently isolated from humans in 1990; by 1996, it was named R. africae (87). This condition presents as a sudden onset of fever (59–100%), nausea, headache (62–83%), and neck myalgia (81%), and begins 5–10 days after tick bite (9, 91). Inoculation eschar (53–100%) develops in most patients at the spot of a tick bite, which is predominantly localized in the lower limbs and accompanied by regional lymphadenopathy (inguinal) (92). Multiple eschars can be observed in approximately 54% of the affected patients (9). Further, a generalized maculocutaneous rash (15–46%) has been observed close to the eschar. Neurological involvement, including encephalopathy and neuropathy, has also been reported (91). Other clinical manifestations include painful sacral syndrome, retinitis, and pan-uveitis (93, 94). Humans are considered accidental hosts, and no known fatal cases have been recorded (95). In febrile patients (travelers) returning from the tropics, ATBF should be considered along with malaria as a differential diagnosis. In SSA, ATBF has been reported with a high incidence in Senegal (21.4–51%) (76) and Cameroon (11.9–51.8%) (96). Rickettsia africae has been detected in ticks and, in some cases, in humans among travellers by PCR in several countries in Africa, including Chad, Sudan, Ethiopia, Niger, Mali, South Africa, and many other countries across the continent (76, 92). The prevalence of R. africae in ticks is > 50% in Uganda and Nigeria (49), Cameroon (50), the Union of the Comoros (51), and Cote d’Ivoire (52).
Mediterranean spotted fever
One of the oldest VBD is MSF, which is endemic to the Mediterranean area (comprising northern Africa and southern Europe), although sporadic cases have been reported in southern Africa and central Europe (9). MSF is the most common human tick-borne rickettsiosis in North Africa (97). MSF was caused by R. conorii subspecies conorii and was relatively unknown until 1909, when the first case was described in Tunisia (98). The first clinical case of MSF rickettsiosis was described by Conor and Brush in 1910 (98). The vector was unidentified until 1930 when, in Tunisia, crushed infected Rh. sanguineus ticks were inoculated into patients, and the patient contracted MSF (99). Patients with MSF, also referred as “boutonneuse” fever, typically have high fever (100%), influenza-like symptoms (chills, headache and arthromyalgias), generalized maculopapular rash (97%)—usually on the extremities and subsequently on the trunk involving the soles, palms, and to a lesser extent on the face. An eschar or “tache noire” (local necrotic inflammation characterized by a black crust) at the site of the tick bite may be observed in affected patients (8, 100). The eschar may not be found in some cases and is rarely observed at multiple sites (100). Other clinical features of MSF in affected patients include hepatomegaly (44%), splenomegaly (19%), and gastrointestinal symptoms (30%). The presence of either unilateral or bilateral conjunctivitis may represent an eye inoculation site for the bacterium (9). Brown dog ticks (Rh. sanguineus) is the main vector and reservoir, and this pathogen has also been reported in other tick species such as Rh. evertsi evertsi, Rh. muhsamae, Rh. simus, Hae. leachi, and Hae. punctaleachi (7, 8, 101). Most cases of MSF occur in summer (between July and October), similar to reports from southern Europe (8, 97). This period coincided with warm climatic conditions that were favorable to the vector, with increased aggressiveness, propensity, and expansion of Rh. sanguineus (102). The major risk factor for MSF is direct contact with dogs and domestic animals (97, 103, 104). Due to the influence of climate change, it was hypothesized that the host-seeking and feeding behaviors of Rh. sanguineus tick vectors could have been altered by these climatic circumstances (97), as observed for other tick species (105, 106). MSF has been reported in Algeria (97), Morocco (103), and Tunisia (104). Rickettsia conorii subspecies conorii has also been detected in ticks in Chad, Kenya, Somalia, and South Africa (100).
Israeli spotted fever
Rickettsia conorii subspecies israelensis, the causative agent of Israeli spotted fever (ISF), belongs to the R. conorii complex, and was first isolated in Israel in 1974 by Goldwasser (107). This subspecies is genetically related to the etiological agent of MSF, R. conorii subsp. conorii (108). It is one of the most severe and life-threatening rickettsial infections worldwide. Rhipicephalus sanguineus is a vector and potential reservoir for R. conorii subsp. israelensis (8). This tick species has a low affinity for hosts other than dogs (109). Just like MSF, human cases of ISF are sporadic (110, 111). These cases have been described in Portugal and Italy (112). Clinical manifestations are similar to those of other SFG except for the absence of eschar (90%) at the site of tick bite, and when visible, it resembles a small, pinkish papule (4, 9). Additionally, a history of tick exposure is not always present (111, 112). Several forms and fatal cases of ISF have been documented, mostly among travelers and people with deficiency in glucose-6-phosphate dehydrogenase (9). In one of the cases reported in southern Tunisia, the patient had a history of previous travel to Libya (two weeks) and had worked in the livestock industry (113). Another confirmed reported case of ISF involved a Swiss tourist who took a seven-day cruise on the Mediterranean Sea and sailed along the coast of Libya, Malta, and Crete (109). During the trip, he visited a handful of archaeological sites in Libya, and unfortunately, he lost his life (109). As there is no documented case of ISF in other locations (Malta & Crete) visited by tourists, we can say that the geographical distribution of ISF extends to North Africa and Libya. The similarity in vector types for both ISF and MSF denotes evidence of geographical overlap between the two diseases. R. conorii subsp. israelensis has also been detected in blood DNA and ticks (Rh. sanguineus) in an epidemiological survey of tick-borne pathogens in dogs in Nigeria (65), and in Rh. appendiculatus ticks in Cameroon (50).
Rickettsia sibirica mongolitimonae infections
Rickettsia sibirica mongolitimonae was initially named HA-91 based on serotyping (114) and was first isolated from Hy. asiaticum ticks collected from Mongolia in 1991 (115). This organism is regarded as a subspecies of the R. sibirica group and exhibits ecological and serotypic specificity (116). This Rickettsia species causes lymphangitis-associated rickettsiosis (LAR) due to lymphangitis and lymphadenopathy that occur with this infection (4). The clinical features of the reviewed cases included headache (100%), myalgia (100%), fever, chills (19%), eschar on the upper or lower limbs, enlarged lymph nodes, lymphangitis (46.1%), and generalized maculopapular rash, particularly on the palms and soles (116, 117). Recently, a patient returning from Cameroon with a confirmed case of rickettsiosis due to R. sibirica mongolitimonae did not show any signs of lymphadenopathy or lymphangitis (118). Lymphangitis has also been described in ATBF, although it is less pronounced (95). In general, R. sibirica mongolitimonae infection is mild, with complications such as disseminated intravascular coagulation, shock, acute renal failure, neurological disorders, retinal vasculitis, and atrial fibrillation (118). The clinical features presented herein may prove to be helpful, but they are not exhaustive for the diagnosis of LAR. Therefore, it is likely that their distribution is more widespread than geographically reported. LAR primarily occurs between March and September. Several cases have been confirmed in patients from different nationalities in Southern Europe, with the majority of cases recorded in patients from France (116). Therefore, Rickettsia sibirica mongolotimonae is endemic in southern France, Africa, and Asia (116). The competent tick vector for this pathogen in Southern Europe, including France, is currently unknown. Although Hyalomma ticks are extensively distributed in these areas, Hy. asiaticum has been implicated as a competent vector in China (Asia) (115) and Algeria (North Africa, a part of the Mediterranean) (119). In other parts of Africa—Mali and Senegal—R. sibirica mongolitimonae has been detected in Hy. truncatum (33, 76). Therefore, further studies including transmission experiments are needed to clarify the role of this tick vector as the possible vector in the Mediterranean region comprising southern France and other parts of northern Africa.
Four human cases have been documented in Algeria, Egypt, Cameroon, and South Africa (118, 120). Cases occur frequently during the spring season (116); however, the clinical spectrum and epidemiology of infection with R. sibirica mongolitimonae must be further analyzed to understand the disease. It has also been detected in ticks collected from the Niger Republic and Senegal (118). It appears that Hyalomma spp. ticks are associated with R. sibirica mongolitimonae, with DNA detected in Hy. truncatum in Mali and Senegal (33, 76) and Hy. aegyptium ticks in Algeria (119); however, transmission experiments are necessary to clarify the role of these ticks as vectors or reservoirs for this rickettsiae.
Innominate rickettsioses (R. aeschlimannii infection)
The infection is caused by R. aeschlimannii, which was first isolated in Morocco in 1992 from Hy. marginatum ticks (8, 75). Further, R. aeschlimannii has been detected in this tick species in North Africa and southern Europe (67). The first documented human infection was in a French patient who became ill after a trip to Morocco. The symptoms were similar to those of MSF, including high fever, eschar on the ankle, and generalized maculopapular rash (121). In Tunisia, R. aeschlimannii has been confirmed by serology in a human patient (122) as well as in two human subjects in Algeria using immunofluorescence (IF) and western blot (WB) assays (123). It has also been reported in humans from different locations in Africa, with the majority being in North Africa. For instance, it has been confirmed in a South African citizen returning from a hunting trip (124). In most published studies, R. aeschlimannii strains have been documented in ticks belonging to the genus Hyalomma collected from vegetation, humans, and various animal hosts (25, 53, 68, 125, 126). Transmission is believed to be perpetuated via transovarial and transstadial means (127). Largely, molecular tools have been useful in the detection of R. aeschlimannii in Hy. marginatum Aegyptus collected from Algeria (67) and Morocco (128), and this is one of the common ticks found on cattle in Morocco. Furthermore, this rickettsia has been detected in Hy. aegyptium ticks feeding on Algeria tortoise, Hy. dromedarii, Hy. marginatum rufipes, Hy. impeltatum, and Hy. truncatum collected from cows or camels from Algeria, Sudan, Egypt, and Tunisia (25, 69, 73, 77, 125). Aside from North Africa, R. aeschlimannii has been widely detected in blood-fed ticks in West Africa (Mali, Niger, Senegal, Nigeria, Senegal, and Ivory Coast), with a prevalence ranging from 15–95% in several subspecies of Hyalomma ticks, including Hy. marginatum rufipes and Hy. truncatum (52, 68, 74, 76, 78).
Astrakhan fever
Rickettsia conorii subsp. caspia is responsible for Astrakhan fever. It was first isolated from a patient in the Astrakhan region located near the Caspian Sea in Russia (9). The clinical manifestation of the disease is similar to that of MSF, except for the absence of a fatal form and a lower incidence of inoculation eschar (9). The first documented isolate of this rickettsia in Africa was obtained from a patient in Chad. The patient had fever, dyspnea, maculopapular rash, inoculation eschar on the leg, and conjunctivitis of the right eye (28). Rhipicephalus sanguineus and Rh. pumilio have been shown to harbor this rickettsia. In Zambia, the first documented report of R. conorii subsp. caspia engorged Rh. sanguineus ticks collected from dog (129). Therefore, further testing of ticks with an emphasis on unfed/un-engorged ticks is needed to clarify whether Rh. sanguineus can be infected (by transstadial persistence) and the likelihood of it being a potential vector of R. conorii subsp. caspia.
Rickettsia massiliae infection
This is another SFG rickettsiae that is infectious to humans, and has been confirmed in Europe, South America, and Africa (8, 130). Patients present with clinical signs similar to MSF (131). In some tropical African countries, R. massiliae is associated with several species of Rhipicephalus, including Rh. spp. from the Ivory Coast (86), Rh. senegalensis from Guinea (40), Rh. guihoni from Senegal (76), and Rh. evertsi evertsi in Nigeria (53).
New rickettsial species infection
Despite the growing number of new species of Rickettsia worldwide, data on their complete genomic sequences and annotations are scarce, with only a few species available (3). In recent decades, researchers have characterized several species of bacteria within the genus Rickettsia using molecular-based techniques before they can be cultured (41). Uncultured rickettsiae with proper molecular characterization were assigned the nomenclature Candidatus (Ca.) as proposed by experts according to taxonomic criteria, and is thus considered a potentially new species of Rickettsia (132). Numerous cell lines, media with supplements, and culture conditions have been tested for in vitro cultivation of Rickettsia spp (133). However, the isolation of these bacteria is challenging (41).
The number of bacteria within the genus Rickettsia that fulfilled the criteria for Ca. “Rickettsia” spp. continues to increase globally, particularly in Africa. It is important to note that before designating a novel rickettsial species as a strain, additional rickettsial gene analyses are needed. For instance, Rickettsia sp. strain Davousti, which was first detected in Am. tholloni ticks collected from African elephants in 2007 (134), was further characterized as Ca. ”Rickettsia davousti,” which was conducted—using a tick sample (one Amblyomma nymph) from a tourist who travelled from Gabon to Spain in 2015—by targeting five genetic gene fragments used to classify rickettsia partial gene sequences namely ompB, ompA, 16S rRNA, sca4, and gltA (135). Figure 1 provides an overview of the geographical distribution of the SFG Rickettsia species reported across Africa.
Furthermore, according to Buysse and Duron (136), before a species can be designated as a novel Rickettsia species, there must be a substantial magnitude of difference in their allelic profiles, and there must be a unique relative to all other recognized and putative species within the genus Rickettsia. These criteria must be met when a novel pathogen is typed using at least four gene-coding fragments already enumerated (137, 138). It remains to be seen if most studies reporting the existence of novel putative species of Rickettsia have fulfilled these criteria. From our literature review, some of these lapses were not fulfilled; however, it is beyond the scope of this review to discuss the downsides of these studies. Based on this evidence, it is likely that most of these new species may be a new genotype of the already characterized species. Reports of new species of Rickettsia are mostly based on inadequately performed phylogenetic analyses.
Ticks as competent vectors and reservoirs of SFG rickettsia in Africa
The concept “vector competence” can be defined as the intrinsic ability of an arthropod to transmit infection, comprising the efficiency to become infected and to maintain and transmit a pathogen (139). The vector competence of an arthropod can only be determined by feeding the supposed vector on a vertebrate host with the circulating pathogen; thereafter, allowing the arthropod to molt and re-feed on an uninfected susceptible vertebrate host, and finally examining the presence of infection in the latter host (140). Therefore, the mere report of Rickettsia DNA detection in blood-fed ticks does not denote vector competence but may simply imply that the genetic material was acquired from the fed blood of the vertebrate host. In addition, pathogen detection in adult ticks may be the result of previous infections at an immature stage. However, this reflects the successful transmission of the pathogen, which, to my knowledge, is the first step of transmission to be tested when investigating vectorial competence in ticks. It is important to emphasize that reports of ticks harboring Rickettsia species must not be interpreted as competent vectors. Additionally, pathogen detection in adult ticks collected from non-pathogen-carrying hosts could still be acquired from hosts at immature stages and not from the vertebrate host from which the adult ticks were harvested (10).
Hematophagous arthropods such as lice, mites, and fleas are responsible for the transmission of rickettsial diseases through their infectious feces, unlike ticks, where the bacteria are transmitted primarily via salivary secretions (141). These arthropods acquire bacteria either by co-feeding, that is, when uninfected and infected arthropods feed together on the same host (usually bacteremic), or by vertical transmission (142, 143). For an arthropod vector called a bacterial reservoir, rickettsiae in the arthropod can be perpetuated efficiently both transovarially and transstadially (144). As our review focuses mainly on ticks, our discussion will be limited to this group of arthropods. Therefore, transmission occurs through transdermal inoculation via tick saliva (4). These ticks can act both as vectors and sometimes as reservoirs of SFG rickettsiae and transmit the infection to domestic animals, humans, and wildlife (145). Most ticks require optimal environmental conditions, hosts, and biotopes. These factors determine the geographical dispersal of ticks and, consequently, the risk of the disease (8).
More than 900 species of ticks are currently recognized, which can be divided into three families: Ixodidae (hard ticks), Argasidae (soft ticks), and Nuttalliellidae (144, 146). The use of several hosts and different life stages, their long life span, and the use of intracellular digestion facilitate the penetration of rickettsia into tick tissues, which enhances the vectorial capacity of ticks. Recently, Rickettsia spp. has been discovered in different hosts, among which hard ticks (Ixodidae) remain the main arthropod hosts (18, 147). However, only a few Rickettsia species have been documented from a handful of soft ticks (Argasidae). Rickettsia species, including R. bellii and Rickettsia hoogstraalii, are regularly identified in Argas and Ornithodoros spp (136). Other species, including “Candidatus Rickettsia nicoyana,” “Candidatus Rickettsia wissemanii,” Rickettsia japonica strain argasii, and Rickettsia lusitaniae are known from a few Ornithodoros and Argas species (137, 148–151). Numerous reports on the discovery of new putative species of Rickettsia in soft ticks have been documented across Africa. Some of these new species may be closely related to well-known species (152).
The geographical distribution of tick species implicated in the dissemination of the spotted fever group rickettsiae across Africa is shown in Figure 2.
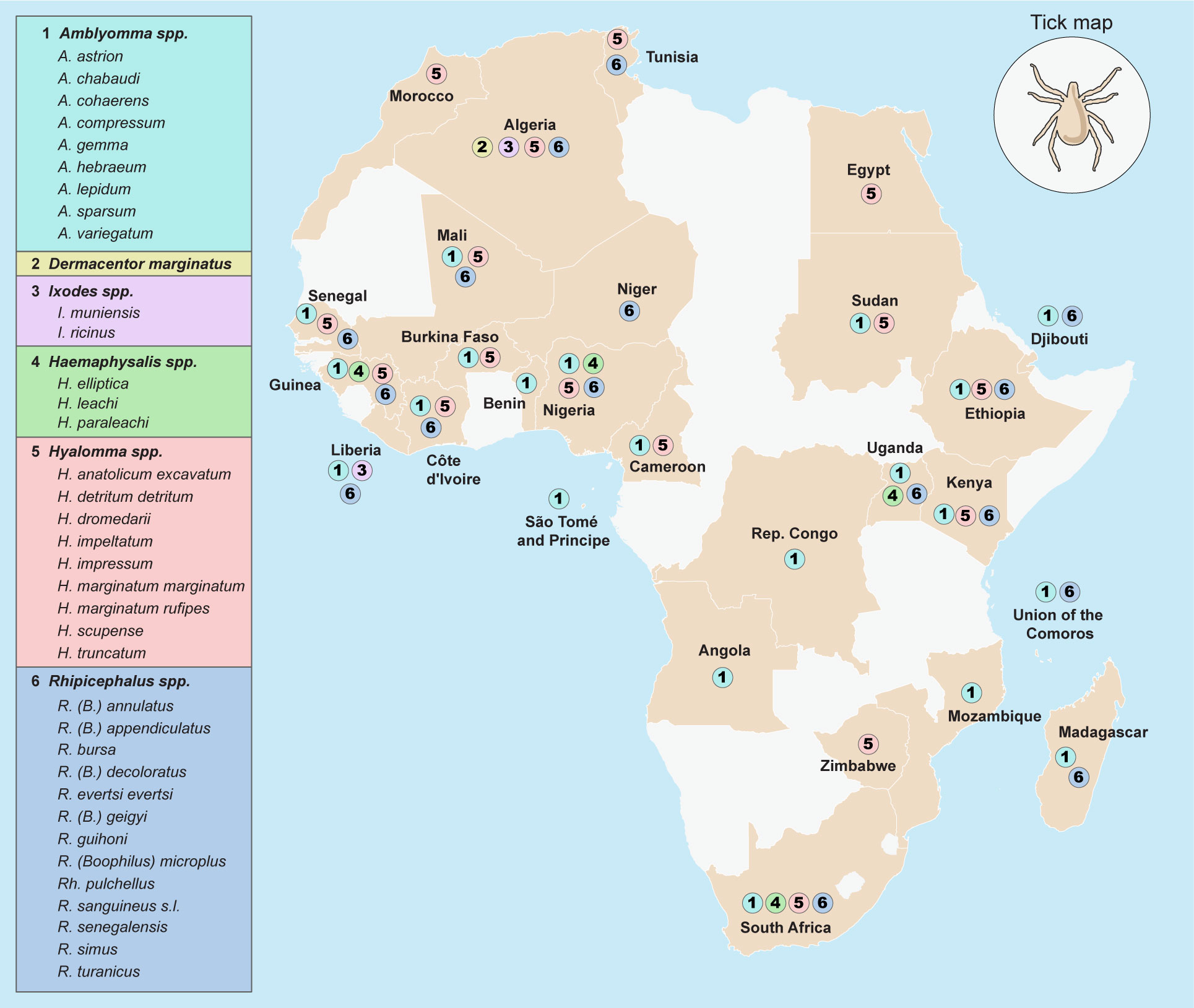
Figure 2 Geographical distribution of tick species implicated in the dissemination of Spotted Fever Group rickettsiae across Africa.
Ixodidae (hard ticks)
Amblyomma
Amblyomma variegatum and Am. hebraeum are the main competent tick vectors that transmit R. africae and are well-distributed in SSA (78). All parasitic stages (adults, nymphs, and larvae) may transmit R. africae. Adult ticks rarely feed on humans, although nymphs attach more frequently, whereas larvae are serious pests (76, 153). The adult stage of A. variegatum frequently attacks cattle, but other domestic hosts include sheep, donkeys, horses, pigs, buffaloes, antelopes, and giraffes (153). Unlike other ticks, Am. spp. does not just wait passively for their victims on vegetation but are more aggressive, emerging, and running toward a nearby host (153). These tick species are highly responsive to CO2 concentration, humidity, NH3, aromatic chemicals, body temperature, and airborne vibrations (95). Amblyomma variegatum ticks are life-long reservoirs of R. africae (88) because of their transovarial and transstadial perpetuations (142), high transovarial and transstadial transmission rates (100%), and a filial infection rate of 93% in transmission experiments (142). Rickettsia africae has also been reported in other species of Amblyomma including Am. compressum in the Democratic Republic of Congo and Liberia (39, 40), Am. cohaerens in Ethiopia (126, 154), Am. gemma in Kenya, Burkina Faso, and Ethiopia (41, 126, 155), and Am. lepidum in Djibouti, Sudan, and Ethiopia (69, 154, 156). It has been speculated that Am. cohaerens may play a role in maintaining the natural transmission cycle of R. africae in areas with low prevalence of Am. variegatum (38).
Hyalomma
Tick species within this genus, especially Hy. dromedarii (157, 158), Hy. impeltatum, Hy. excavatum (159, 160), Hy. aegyptium (119), Hy. impressum, and Hy. marginatum rufipes (77), are vectors as well as potential reservoirs for spotted fever Rickettsia including R. aeschlimannii, R. africae and R. sibirica mongolitimonae. Hyalomma spp. are aggressive and bite humans (76). Data from numerous epidemiological studies may support the theory that Hy. is the vector and reservoir of R. aeschlimannii (25, 53, 68, 125, 126). This characteristic could facilitate the transmission of R. aeschlimannii to camel handlers and other livestock workers. Several species exist across Africa, with major concentrations occurring in the Mediterranean region and North Africa. Hyalomma marginatum marginatum, known as the Mediterranean Hyalomma, accounts for 42% of ticks collected from cattle in Morocco (4). This tick species readily bites people using an attack strategy by emerging from their habitat and running toward their perceived hosts when they appear nearby (127). The geographical distribution of tick species may coincide with the distribution of R. aeschlimannii because this bacterium is transmitted transovarially with huge epidemiological significance, as Hy. marginatum marginatum may be a reservoir and vector (127). Hyalomma marginatum rufipes ticks are widespread and exist only in SSA, and their geographic distribution coincides with R. aeschlimannii, which has been shown to be transstadially perpetuated in this tick. Hy. dromedarii is widely distributed in Asia and Africa. This tick species can be found in multiple biotopes and a wide variety of hosts (25). Other species of Hyalomma that are considered probable vectors include Hy. truncatum, Hy. impeltatum, and Hy. impressum. Rickettsia africae DNA has been reported in some species of Hyalomma ticks including Hy. marginatum rufipes in Guinea (40) and Hy. impeltatum in Nigeria (53).
Rhipicephalus
One of the most widespread ticks with specialized feeding on domestic dogs worldwide is Rh. sanguineus sensu lato. This tick is considered a significant vector, as many pathogens, including bacterial and parasitic microorganisms, can replicate inside it (161). This tick species is adapted to warm climates but thrives well in human homes, dog kennels, and cooler regions (162). Rhipicephalus sanguineus, a brown dog tick, is thought to be a vector of MSF (163). This hypothesis is supported when humans are inoculated with crushed Rh. sanguineus ticks, and contracted MSF (99). The infectious agent in the tick was described by Brumpt with the name R. conorii given in honor of the work of Conor (145, 164). In Western Africa, R. africae has been reported in several species of Rhipicephalus including Rh. annulatus in Nigeria, Guinea, and Senegal (40, 53, 76); Rh. decoloratus in Nigeria (165); Rh. geigyi in Liberia (40). The vectorial capacity of Rhipicephalus ticks for the transmission of R. africae remains unclear. However, Rh. guihoni is commonly observed in the drier areas of SSA. It is a member of Rh. sanguineus group and infests a wide range of hosts, including birds. In addition, ticks of the Boophilus subgenus Rhipicephalus exhibit monotropic behavior. Rickettsia africae has been detected in a handful of closely related species, such as Rh. (B.) microplus, Rh. (B.) decoloratus, Rh. (B.) annulatus, and Rh. Geigyi (48, 51, 54).
Argasidae (soft ticks)
Some SFG and TGR rickettsiae have been reported in argasid ticks (166). However, the host range, pathogenicity, and distribution of Rickettsia spp. Associated with argasid ticks are poorly understood. In Zambia, R. lusitaniae was detected in Ornithodoros faini recovered from bat guano in Lusaka, and R. hoogstraalii in Argas (Ar) walkerae ticks obtained from a chicken coop in Isoka (167). Interestingly, R. lusitaniae has also been detected in three other continents namely Europe, Asia, and the United States. Ornithodoros faini is a bat-associated tick in Africa; however, the role of vertebrate hosts in the maintenance cycle of R. lusitaniae remains largely unknown. Nonetheless, a human case of borreliosis transmitted by O. faini was recently reported in Zambia (167). Therefore, transmission studies are needed to investigate the vectorial capacity of O. faini and pathogenicity of R. lusitaniae in humans and animals. On the contrary, Ar. walkerae, a known poultry tick, is closely associated with R. hoogstraalii, a suspected member of SFG rickettsiae with unknown pathogenicity, and is closely related to R. felis, an emerging pathogen transmitted by fleas (168). Currently, R. hoogstraalii is not known to cause diseases in vertebrates, but it has been observed to exhibit cytopathic effects on mammalian cells, similar to pathogenic SFG rickettsia (169). Originally, R. hoogstraalii was reported in Croatia in Hae. sulcata, an ixodid tick (170). Additionally, other argasid ticks, including Ornithodoros capensis and Ar. persicus, have been shown to harbor rickettsial bacteria across diverse geographical spaces (167). In general, Hae. sulcata, O. capensis, and Ar. persicus are bird-associated ticks. The migratory birds may have contributed to the wide distribution of R. hoogstraalii in southern Africa, including Zambia. In Namibia and South Africa, R. hoogstraalii has also been reported in Argas transgariepinus ticks collected from bats (149, 171). In Zambia, warthog-feeding soft ticks (Ornithodoros porcinus) were found to harbor R. lusitaniae after analyzing the gltA and htrA nucleotide sequences (172). In Ethiopia, R. hoogstraalii DNA was detected in Ar. persicus collected from cracks and crevices of human dwellings and under the bark of trees in the vicinity of livestock areas (173). Its high infection rate in ticks suggests that it may be a tick symbiont. Soft ticks belonging to the Ornithodoros erraticus complex, including Ornithodoros occidentalis, O. erraticus, and Ornithodoros normandi, have been reported in northern Africa (136). Both O. occidentalis and O. normandi are known only from North Africa and have been reported from Morocco and Algeria, respectively, whereas the last species, O. erraticus, is more widespread across the Mediterranean regions (136). Small mammals are the preferred hosts for this tick, but they can also bite humans (174). These ticks have been found to carry some potential pathogenic Rickettsia namely “Candidatus Rickettsia africaseptentrionalis” and “Candidatus Rickettsia mauretanica.” These novel Rickettsia species belong to a transitional phylogenetic group and are closely related to Rickettsia asembonensis (175).
Geographical distribution and epidemiological relationship between SFG rickettsiae and tick vectors across different regions in Africa
Several species of SFG rickettsiae infect both humans and animals worldwide. Some rickettsial pathogens are prevalent in a particular geographic region and are largely dependent on the distribution of competent tick vectors. SFG rickettsiae, which are considered human pathogens, have been reported in Africa and include R. africae, R. aeschlimannii, R. conorii, R. sibirica mongolitimonae, Rickettsia slovaca, Rickettsia helvetica, R. massiliae, Rickettsia monacensis, and Rickettsia raoultii (176). A bibliographic list of some SFG rickettsiae in different tick vectors distributed across different countries in Africa is summarized in Table 2.
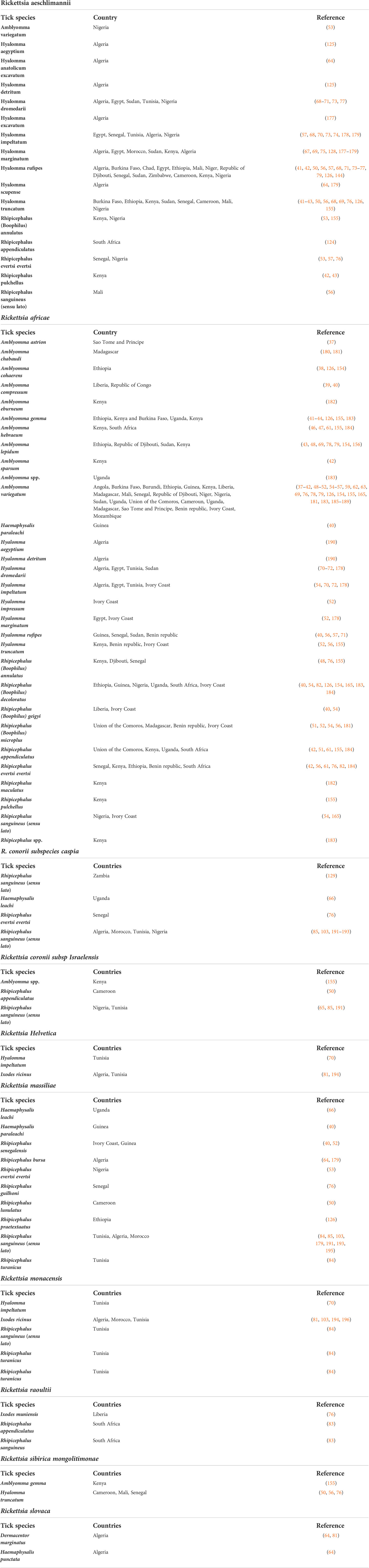
Table 2 Bibliographic reports of rickettsiae in hard ticks in Africa according to tick species and country.
West Africa
The Economic Community of West African States (ECOWAS) comprises 15 member states, including Benin, Burkina Faso, Cape Verde, Cote d’Ivoire, Gambia, Ghana, Guinea, Guinea-Bissau, Liberia, Mali, Niger, Nigeria, Senegal, Sierra Leone, and Togo. Several nations within the ECOWAS sub-region have reported the presence of SFG rickettsiae, such as Nigeria (53, 165), the Benin Republic (55), Niger and Mali (56, 78), Liberia and Guinea (40), Cote d’Ivoire (52), and Senegal (57). Most tick species harboring SFG rickettsiae in West Africa belong to the genera Amblyomma (55, 56), Rhipicephalus (56, 86), and Hyalomma (56, 68, 74).
Rickettsia africae was highly prevalent in A. variegatum ticks across the sub-region (40, 52, 57). Other tick genera where R. africae has been reported with lower prevalence include Hyalomma and Rhipicephalus (52, 56, 76), which suggests that they are probably not competent vectors (52). In contrast, Rickettsia aeschlimannii has been observed in Hyalomma ticks, with a higher prevalence in Hy. marginatum rufipes than in Hy. truncatum in Mali (56, 78), Senegal (76), and the Ivory coast (52). Similarly, R. sibirica mongolitimonae was observed in Hy. truncatum from Niger (78), Senegal (76), and Mali (56). Interestingly, fragments of R. massiliae DNA have been detected in Rh. spp. ticks collected on the Ivory Coast (86) and Mali (78), as well as in Rh. senegalensis in Liberia (40), Rh. guihoni in Senegal (76), and Hae. paraleachi ticks collected in the Republic of Guinea (40). R. conorii israelensis was also observed in Rh. sanguineus sensu lato (s.l.) in Nigeria (65), whereas R. conorii ssp. has been reported in Rh. evertsi evertsi ticks from Senegal (76). Sporadic reports of R. raoultii have been documented in South Africa in Rh. sanguineus s.l. (45, 83), and Rh. spp. (83), and only one report in I. muniensis ticks from Liberia (40).
North Africa
According to the United Nations, North Africa includes eight territories or countries (Algeria, Libya, Sudan, South Sudan, Egypt, Tunisia, Morocco, and Western Sahara) that are found in the northernmost area of the African continent. Since the beginning of the 21st century, the SFG Rickettsia has been found in North Africa. Since then, several SFG rickettsiae have been detected in arthropods thanks to the use of PCR and sequencing methods leading to new questions with regards to the geographical distribution of rickettsiae in this region.
To date, eight tick-borne rickettsial species have been detected in North Africa, mainly in human cases or ticks. These included R. conorii subsp. conorii, R. conorii subsp. israelensis, R. aeschlimannii, R. sibirica mongolitimonae, R. massiliae, R. slovaca, R. raoultii, and R. monacensis (81, 84, 125, 181). In Algeria, both R. conorii subsp. conorii (MSF) (97) and R. aeschlimannii (123) have been associated with human rickettsiosis. Rickettsia conorii subsp. israelensis was detected in Rh. sanguineus ticks in Tunisia (197), suggesting that the geographical distribution of this species is broader than expected. Additionally, R. aeschlimannii infection has been confirmed in Hy. dromedarii ticks in Tunisia (25), Egypt (73), and Sudan (69). In Algeria, R. aeschlimannii has been reported in Hy. aegyptium (125), Hy. anatolicum excavatum, and Hy. scupense (64). Documented human cases of R. aeschlimannii have been reported in Morocco (121), Tunisia (122), and Algeria (123). MSF has been reported in human patients in Algeria (181).
Rickettsia slovaca, a member of the SFG rickettsiae common in Europe, has also been isolated from ticks, such as D. marginatus (64, 81) and Hae. punctata (64). Rickettsia massiliae has been detected in Rh. turanicus and Rh. sanguineus s.l. ticks collected from livestock in Tunisia (70, 84, 197), Morocco (103), and Algeria (67). Similarly, R. monacensis DNA was detected in Rh. sanguineus s.l. tick specimens were collected from goats in Tunisia (84).
East Africa and Central Africa
Numerous tick-borne zoonotic and veterinary diseases have been documented in East Africa, including SFG rickettsiaeca used by R. africae, R. conorii, and R. aeschlimanii (58, 126, 182, 186, 198). Extensive studies have been conducted in Kenya on R. africae in ticks collected from vertebrate animals, particularly cattle. The results indicated a high prevalence of R. africae across several counties within the country (42, 43, 155, 186, 187). High prevalence of Rickettsia spp. infection in Kenya was attributed to the presence of a high population of domestic (cattle, sheep, goats, and camels) and wild (giraffes, buffaloes, and rhinoceroses) animals, which predominantly feed on these animals and acquire Rickettsia spp. infections (43). Furthermore, PCR and sequencing revealed the presence of R. africae DNA in several tick species within the genera Amblyomma, Rhipicephalus, and Hyalomma (43, 155, 185–187). Furthermore, R. massilliae was registered in Rh. annulatus, R. conorii subsp. Israelensis in Amblyomma ticks, and R. mongolitimonae in Am. gemma (155). Using PCR and sequencing of three target genes (gltA, ompA, and ompB), R. aeschlimannii has been detected in Hy. truncatum and Hy. marginatum (43).
The first documented report of R. africae in Djibouti was in 2007 on Am. lepidum ticks collected from cattle imported from Ethiopia (78). In addition, R. africae was detected in Am. lepidum, Am. variegatum, and Rh. annulatus from Djibouti (48). Additionally, R. aeschlimanii was amplified in Hy. truncatum and Hy. marginatum ticks (48). In Ethiopia, R. aeschlimannii has been reported in Hy. rufipes and Hy. truncatum ticks (79, 126). R. massiliae has also been documented in Rh. praetextatus ticks (126). R. africae DNA has been reported in Am. lepidum (79), Am. variegatum (38, 79, 126), Am. cohaerens (126), and Rh. decoloratus in Ethiopia (82, 126). Additionally, in pools of Amblyomma and Rhipicephalus ticks, Rickettsia africae DNA was detected from those collected in Ethiopia (173). R. conorii was detected in Hae. punctaleachi ticks (156), whereas both R. conorii conorii and R. massiliae were detected in Hae. leachi and Rh. praetextatus ticks (66), with a concomitant high prevalence of R. africae in A. variegatum ticks (182). In Central Africa, several species of SFG Rickettsia have been recorded in ticks collected from this region. For instance, R. africae has been reported in Am. compressum ticks from the DRC (39). Similarly, R. africae has been documented in Amblyomma ticks from Cameroon (96) and the (CAR) (188). In Cameroon, different SFG rickettsiae, including R. africae, R. aeschlimannii, R. sibirica, and R. massiliae, were detected in Amblyomma, Hyalomma, and Rhipicephalus ticks (50). Finally, in Chad, R. aeschlimannii has been documented in Hyalomma ticks (79).
Southern Africa
Southern African Development Community (SADC) member states includes Angola, Botswana, Eswatini, Lesotho, Madagascar, Malawi, Mauritius, Mozambique, Namibia, South Africa, Tanzania, Zambia, and Zimbabwe. Four major tick-borne SFG rickettsiae associated with human diseases have been reported in southern Africa. These included R. africae (87), R. raoultii (83), R. conorii (164), R. aeschlimannii (75), and R sibirica subsp. mongolitimonae (199). In this region, R. africae is primarily transmitted by Am. hebraeum (88). Other tick species have been found to harbor R. africae (40, 78); however, this bacterium was detected for the first time in Hae. elliptica, thereby possibly extending the range of known vectors. The detection of R. africae in Am. hebraeum, a tick known to readily bite humans, and Hae. elliptica, one of the most common ticks infesting domestic dogs in South Africa, underpins the potential risk of human infection with this pathogen in the southern Africa region. Rickettsia aeschlimannii was registered as a South African patient after returning from hunting and fishing trips (124). Additionally, Hy. truncatum ticks were collected from cattle in Angola (200). Using PCR, R. africae and R. aeschlimannii were reported in Am. variegatum and Hy. truncatum ticks collected from dogs and cattle in Shangombo, a town at the Zambia–Angola border (201). Furthermore, both R. africae and R. aeschlimannii were documented in Hyalomma ticks collected from cattle in Zambia (202). In Lesotho, R. africae was detected in Hy. rufipes and Rh. evertsi evertsi ticks collected from domestic animals including cattle, goats, sheep, and horses (203). In addition, R. africae was detected in Am. variegatum adult ticks from cattle and their questing larvae in South Africa (204), and in Am. variegatum and Am. heabreum ticks from cattle in Mozambique (205). Rickettsia conorii subsp. caspia was recorded in Rh. sanguineus ticks collected from Zambia (129). Additionally, Rickettsia raoultii has been detected in Rh. sanguineus ticks collected from cattle and Rh. appendiculatus in sheep (83). The detection of this bacteriumin South Africa and in Rh. spp. ticks suggest a widening of its host and geographical range, with epidemiological implications.
Island nations
Studies investigating the prevalence of rickettsial DNA in ticks collected from domestic and wild animals have been conducted in the Indian Ocean. In Madagascar, Amblyomma ticks are regarded as the predominant tick species, with a high prevalence of R. africae (183, 191, 206). Human infections caused by SFG rickettsiae in Madagascar have been previously documented (207). In the Union of Comoros, a prevalence of 90% of R. africae in A. variegatum ticks has been reported (51). Additionally, other tick species, such as Rh. appendiculatus and Rh. (B.) microplus, were found to harbor R. africae DNA (51). Interestingly, R. monacensis, an emerging human pathogen of SFG rickettsiae, has been reported in dogs from Cape Verde (208). This pathogen has been detected in ticks from North Africa, Europe, and Asia, as well as in humans with MSF-like illnesses from Spain and Italy (209–211). Rickettsial DNA has also been detected in Ar. echinops ticks in Madagascar (191). The relationship between different SFG rickettsiae and tick species across Africa is represented in the heat map shown in Figure 3.
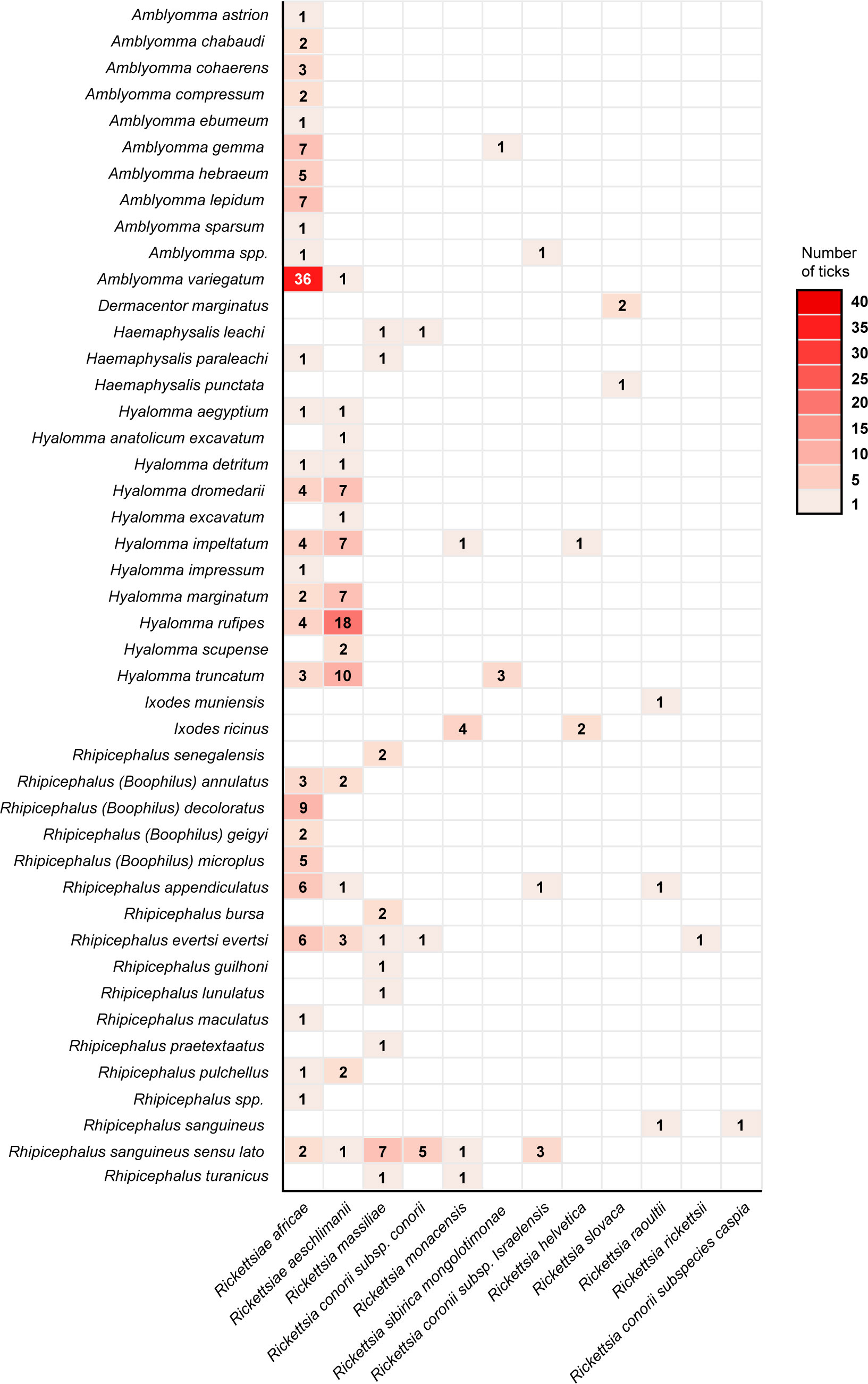
Figure 3 Heat Map showing the relationship between tick species and Spotted Fever Group rickettsiae across Africa.
Epidemiological aspects and challenges
Evolutionary perspective of Rickettsia perpetuation
From an evolutionary perspective, if the ancestral group of rickettsial organisms is a symbiont, it is possible that evolution played a major role that led to Rickettsia microorganisms finding a way (aside from tick transovarial-transmission) to perpetuate in permanent cycles in ticks (10). The finding of Rickettsia species in phylogenetically distant tick species suggests possible horizontal transmission (10). The transmission of Rickettsia could also occur via tick parasitoids or hyperparasitism (a tick feeding on another tick), as validated for other tick-associated bacteria (212–214). Parasitoids of the genus Ixodiphagus (Ix) (Hymenoptera: Chalcidoidea: Encyrtidae) are well-known natural enemies of ticks and have been described in many tick species worldwide (215, 216). However, the biology and ecology of these parasitoids remain unclear (217). Furthermore, recent studies have demonstrated the potential role of Ix. hookeri in the circulation of certain bacteria, including rickettsiae (Rickettsia helvetica and Rickettsia monacensis), in ticks (216). Regrettably, there is a critical lack of data on the presence of parasitoid wasps in ticks in Africa. Recently, the existence of Ix. hookeri, a parasitic wasp of ticks, was demonstrated in Rh. s (B.) microplus ticks collected in Côte d’Ivoire, west Africa, using molecular based technique (216). The results showed that infestation by parasitoid wasps can occur in adult ticks, with a higher prevalence than previously reported. Many rickettsiae species are endosymbionts; consequently, the level of their dependence on ticks is variable (10). An obligate symbiont may be present in most tick populations; thus, co-cladogenesis between ticks and symbionts should be expected. However, numerous Rickettsia species worldwide affect human health, and Africa is no exception.
Role of small mammals, domestic animals, and wildlife in the epidemiology of SFG Rickettsiae
Tick-borne rickettsiosis is among the oldest known vector-borne diseases. In Africa, several species of Rickettsia have been detected in vertebrate animals (178). Many domestic (sheep, goat, cattle, and dogs) and wild (rodents, hedgehogs, wild rabbits, and opossums) mammals act as hosts and contribute to the dispersal of Rickettsia-carrying ticks in different geographical areas. The role of these mammals in Africa as amplifying hosts for the SFG Rickettsia spp. requires further investigation. Domestic animals, including livestock, may play a role in perpetuation of pathogenic rickettsiae (218). Domestic ruminants in endemic areas have demonstrated evidence of exposure to SFG rickettsiae through detection of antibodies using serological tests (192, 219, 220). However, it remains unclear whether these animals show signs of infection or whether they only act as temporary bacterial amplifiers to re-infect ticks. If they do show any signs of bacteremia, further studies are needed to unravel how long this bacteremia lasts before seroconversion. Furthermore, immunological experiments are needed to clarify whether the immune response persists and is protective against reinfection. The prevalence across vertebrate animals ranges from 0–65% in Africa. Variations in seroprevalence rates may reflect geography, ecology, the method of animal handling, and climatic differences that affect the diversity, abundance, and distribution of ticks in particular areas. Due to cross-reactivity, the discrimination of Rickettsia spp. using serology is difficult. Serological screening for SFG rickettsiae has been carried out for small ruminants in Nigeria and dogs in Angola using the indirect fluorescent antibody test (IFAT) (59, 192). Dogs may develop rickettsiemia due to R. conorii with the development of clinical signs but may not be considered reservoirs of Rickettsia spp (189). Therefore, we could consider these animals sentinels to SFG rickettsiae in a particular geographical region (221). Camelids (with particular reference to one-humped camels) appear to be potential hosts for rickettsial species, particularly in Africa. The estimated molecular prevalence was 18.8% in Nigeria (74), 41.0% in Egypt (157), and 2.7% in Tunisia (70). Dogs may play an important role in the epidemiology of SFG rickettsioses in Africa, as they are important hosts of several African tick vectors (13), and could bring infected ticks close to humans, as shown in other parts of the world (222–224). For example, domestic dogs in South America have been shown to act as amplifying hosts of R. rickettsii for Am. aureolatum ticks (225). Furthermore, advances in molecular diagnostics have illuminated the possible role of small mammals as reservoirs or hosts for numerous emerging and re-emerging infectious agents. This finding is important for understanding the epidemiology of the disease and formulating preventive measures against it (226). Two Am. variegatum ticks collected from small mammals in Mali were positive for R. africae by PCR (227). Additionally, R. conorii DNA has been reported in a pool of Rh. sanguineus collected from domestic rodents in Nigeria (226). In another study, rickettsial DNA was detected in 39.8% of the spleens of vervet monkeys (Chlorocebus pygerythrus) from Luangwa National Park, Zambia (58). In another study in South Africa, R. massiliae was amplified in Am. sylvaticum ticks collected from leopard tortoise (Chersina angulate) and Rh. simus ticks from Bushveld gerbil (Gerbilliscus leucogaster) (228). Furthermore, of the 12 Am. compressum ticks collected from pangolins (Manis gigantea) in the Republic of Congo, 50% were positive for SFG rickettsiae with 100% homology to R. africae (39). Rickettsia africae was detected at a high prevalence of 100% in Am. chabaudi ticks collected from Malagasy spider tortoises (Pyxis arachnoides) (206). In Algeria, Rh. sanguineus ticks collected from wild mammals [boars (Sus scrofa algira), jackals (Canis aureus), and mongoose (Echinomon herpestis)], and screened for Rickettsia, revealing the presence of R. massiliae (64). In addition, R. sibirica mongolitimonae was found in adult Hy. aegyptium ticks collected from spur-thighed tortoises (Testudo graeca) in Algeria (119).
Role of avian populations in the epidemiology of SFG Rickettsia
Research on the possible role of avian populations in the epidemiology of rickettsiosis in Africa is still in its early stages. Surveys for Rickettsia infection in birds in Africa are scarce, with a focus on the detection of rickettsiae in ticks collected from birds. In Africa, antibodies against SFG rickettsiae were detected in farmed ostriches (Struthio camelus) in Zimbabwe (87). It was speculated that the detected antibodies could be specific to R. africae, as this rickettsial species was identified in Am. hebraeum ticks that usually parasitize ostriches in Zimbabwe. However, it is still possible that it could be other SFG rickettsiae, especially R. conorii, as it was reported circulating in that area at that time. Most studies on Rickettsia on wild birds have been conducted in Europe and America (154, 229, 230). Of the 140 ticks collected from one dunnock (Prunella modularis) and five robins (Erithacus rubecula), 61 Hae. concinna and I. ricinus ticks were PCR-positive for R. helvetica and 11 I. ricinus ticks were PCR-positive for R. monacensis (154). Furthermore, no correlation was observed between the positive PCR ticks tested and blood DNA from the birds. In addition, rickettsemia in avian hosts persists after the ticks have been detached; however, the possible role of birds as amplifying hosts was dismissed, as transmission of rickettsia is possible from ticks to birds, but the reverse rarely occurs (154, 231). As most of these birds are migratory, most of which pass through many parts of Africa to avoid the harsh winter and breed, further studies should be advocated to possibly trap some of these birds and screen both the birds and accompanying vector ticks for SFG rickettsiae.
The potential of domestic dogs to act as primary hosts for the adult stage of Am. aureolatum in Brazilian spotted fever (BSF) endemic areas has been evaluated (225). In South America, Binder et al. (225) did not consider passerine birds to play any role in amplifying the hosts of R. rickettsii for Am. aureolatum ticks, although this group of birds is a major host for immature stages of the tick vector. This statement relies on previous studies that failed to detect rickettsemia or tick-rickettsial acquisition among R. rickettsii-exposed passerines (225). Indeed, the high body temperature of birds (> 40°C) should be interpreted as a natural barrier for rickettsial survival in this vertebrate group, as there are no reports of the in vitro growth of any Rickettsia species at temperatures above 37°C.
Tick-borne rickettsioses among travelers and challenges in surveillance of spotted fever rickettsial infection
Owing to globalization, people can cross borders worldwide, and this phenomenon has led to interchanging the epidemiologic burden from each region (232). Tourism-related activities represent a source of income for developing countries, and international adventure tourism has increased astronomically in recent years. Data available from the Geosentinel surveillance network showed that approximately 280 returning travelers from 1996 to 2008 had reported rickettsial diseases, with a considerable proportion of these individuals with SFG rickettsioses (21). Measures to protect wild fauna and biodiversity in combination with land rehabilitation and management practices, including forestry, increase the potential for exposure to arthropods and the risk of rickettsial transmission (27). During travel, humans may wander into previously untouched tick habitats, resulting in an increased risk of tick bites, predisposing them to tick-borne infections (92). SFG rickettsiae transmitted by ticks account for the majority of ricketsioses among travelers, with several cases of ATBF (R. africae) among travelers from SSA (92). The two main tick vectors are Am. variegatum and Am. hebraeum, which are usually found to have high rates of infection (> 50%) in nature (11, 233).
Travelers returning from SSA are at an increased risk of rickettsial infections caused by tick-borne spotted fever than those caused by dengue or typhoid fever (20). In SSA, rickettsial diseases in international travelers are the second most commonly diagnosed diseases associated with patients with systemic febrile illnesses (20, 232). A recent systematic review showed that over 100 confirmed clinical cases of ATBF (due to R. africae) have been documented in European travel, accounting for over 80% of the registered cases (234). Notably, at least 70% of clinical R. africae cases have altered laboratory parameters, with an increase in transaminases and C-reactive protein being the most important changes (234).
In the last ten years, cases of ATBF have been documented in travelers returning from southern Africa, with more cases from patients returning from South Africa (235–237) and Zimbabwe (93, 235). Other reports include travelers returning from Uganda (238), Kenya (239), and Tanzania (240). The majority of the patients were men with a mean age of 40 years, while there were children with ages ranging from 7–16 years (92). Additionally, the majority of the patients were originally from Europe (95, 232, 241), while patients from the United States (93, 241, 242), South America (243, 244), and Asia (245, 246) have also been reported. An overview of some of the published cases of ATBF from 2010 to date is summarized in Table 3. The clinical symptoms displayed by these patients were consistent with those previously reported for ATBF, including eschars and maculopapular rash. Notably, the affected patients shared the risk factors of either game-hunting safari or walking in a rural game-hunting reserve. This observation was made following a seroepidemiological study on returning Norwegian travelers (88). Nonetheless, the diagnosis of most patients with clinical ATBF was performed by PCR detection of R. africae using tissue biopsy or swabs (92).
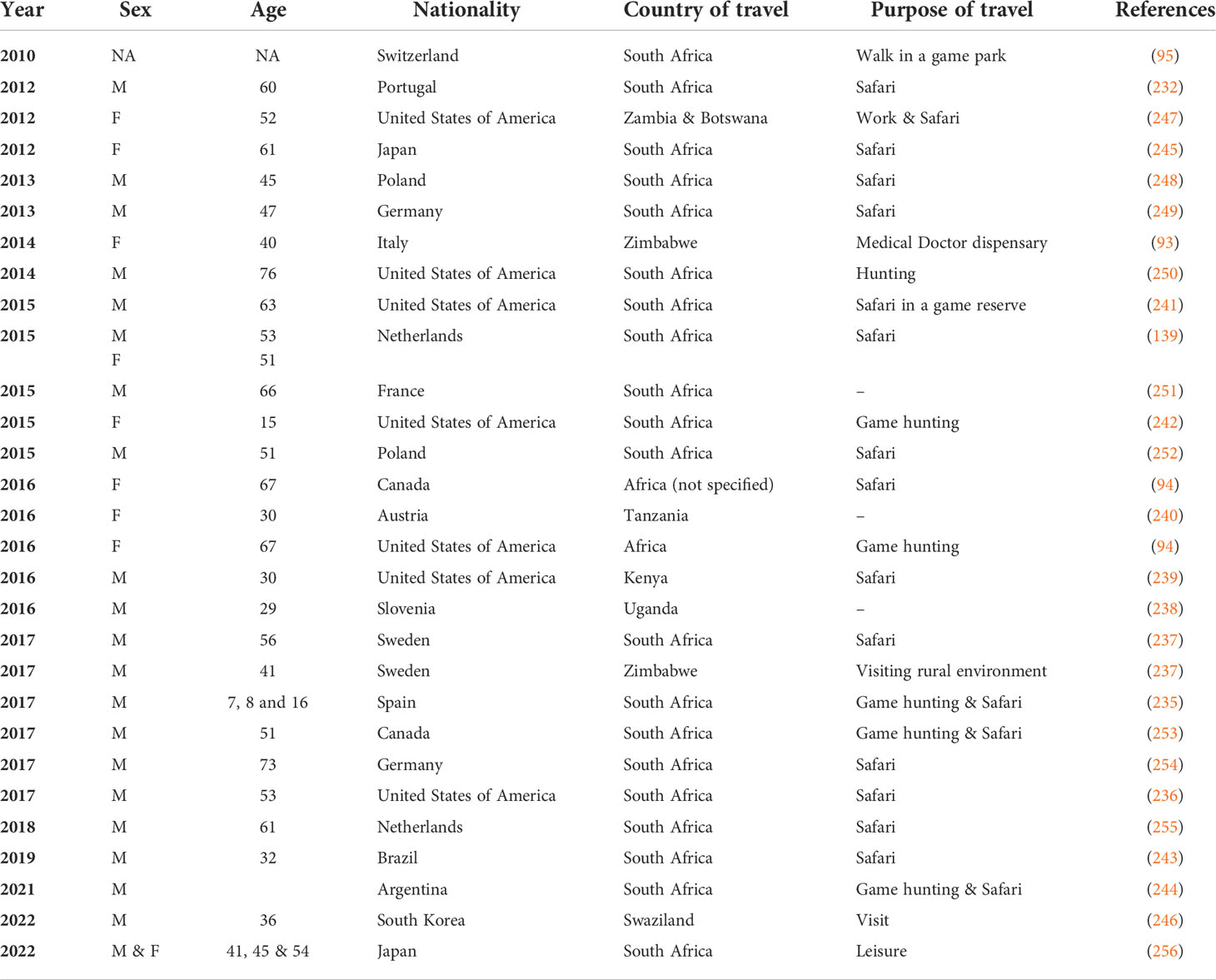
Table 3 Summary of case reports describing African Tick Bite Fever (ATBF) among travelers from 2010 to date.
The clinical presentation and severity of SFG rickettsiosis are highly variable (257), but clinical diagnosis can be easy in patients from endemic regions with a history of tick bites and other accompanying symptoms such as fever, skin rash, and headache during summer and spring months (258). Current methods for rickettsial diagnosis and disease surveillance require major overhaul, because even experienced epidemiologists and clinicians have challenges in consistently and accurately confirming or eliminating the diagnosis of SFG rickettsioses. Importantly, some cases of SFG rickettsiosis may occur during off-season periods in endemic areas (259), in locations where SFG rickettsiosis is unknown to medical practitioners (260), or even in travelers who have not declared their recent travel history (258).
The diagnosis of clinical infections due to SFG rickettsiae is still based on serological testing, despite advances in molecular technologies, because of the need for convalescent antibody titer testing, which is rarely undertaken (261). Early treatment of patients might decrease the expected rise in convalescent IFAT endpoint titers or stop the appearance of skin rash or other symptoms that are peculiar to suspected SFG rickettsiosis and consequently reduce the severity and duration of illness (262). In addition, rickettsiae microorganisms do not multiply in the blood (they do multiply in the endothelial cells). Due to the low sensitivity of molecular tests on blood samples for the detection of rickettsial pathogens, reliable amplification of the pathogen can only be performed in tissues at the time of autopsy (263). However, some patients may die of fulminant disease just before the development of convalescent antibodies. Thus, a substantial number of SFG rickettsioses have never been reported or have remain unnoticed, further complicating the surveillance of SFG rickettsioses. To circumvent these challenges, there is an urgent need to develop sensitive, specific, and accessible diagnostic tests. Serologic assays have some merits, but the disadvantages due to reliance on single time-point serologies limit their application.
Furthermore, PCR testing of blood offers high specificity but low sensitivity because of the low number of rickettsial organisms in the blood during acute illness with R. rickettsii (264). Metagenomic sequencing of blood samples offers an alternative for the broad detection of circulating pathogens; however, it remains unclear whether this approach will have sufficient sensitivity compared with nucleic acid amplification. The PCR test of skin or eschar biopsy samples is specific and sensitive, but biopsies can be difficult to obtain outside the clinical setting. In addition, diagnostic methods utilizing monoclonal antibodies that detect proteins produced by Rickettsia-infected cells require further investigation. If this method proves to be specific and sensitive, it could be utilized for quick testing at the point of care in the early course of illness (196). In conclusion, the current state of rickettsial diagnostics is a constraint for both surveillance and clinical efforts. Therefore, there is an urgent need for primary research focusing on rickettsial diagnostics.
Milestone in rickettsiology research in the continent and the importance of more epidemiological studies in Africa
Rickettsiology encompasses a large body of knowledge including systematics, epidemiology, genomics, molecular diagnostics, microbial interactions, immunopathology, vector biology, and transmission studies. A major focus of research in Africa has been molecular diagnostics using PCR and sequencing to amplify short DNA sequences of Rickettsia species in ticks and blood specimens from vertebrate hosts, including humans (46, 52, 68, 74, 165). Additionally, serology has been sparsely employed, with IFAT being the most widely used serological technique to screen serum samples for antibodies against SFG Rickettsia within the continent (59, 76, 265).
A major emphasis of ATBF in humans in SSA has been on foreign travelers on trips to Africa (95, 236, 240, 244, 254), with little concern for indigenous communities (76, 266). Serological data have indicated high seroprevalence in the native population across several regions of Africa, with records registering 5–51% in western and central Africa and 16–55% in eastern and southern Africa (76, 96, 218), but few acute cases have been documented. Perhaps, this observation could be due to exposure at an early age with possible clinical cases that were ignored due to the paucity of diagnostic instrumentation at health facilities in most communities or poor visibility of inoculation eschars on pigmented skin in black Africans (88). It has also been documented that some R. africae variants may be more virulent than others. These variations are linked to differences in the nucleotide composition (deletion or substitution mutations) of ompA and ompB, which code for the immune-dominant surface cell antigens of Rickettsia, and this could affect the virulence of R. africae variants (185, 186). This could explain the absence of acute ATBF cases among native communities, even with a high documented seroprevalence (185). Furthermore, it has been shown in some pathogens, including Rickettsia, that genome reduction may lead to enhanced virulence (207). It remains to be determined if these variants of R. africae can be found in febrile patients within the indigenous communities.
Insufficient human resources and the required skills in molecular biology are one of the problems of science and health research in Africa. Generally, there is the problem of low numbers, uneven distribution, and immigration of experts of African origin to northern nations, including Europe and North America, for obvious reasons, including poor renumeration, weak government policies for civil servants, and incessant strikes and/or industrial disputes.
Broadly, research on vectors and VBD in Africa is gaining momentum with an increasing number of young professionals with requisite training in molecular biology and genomics, applying their knowledge and skills to comprehend pathogen-vector-host interactions, especially as it pertains to infectious diseases. Owing to the general lack of resources, it is very difficult to see a researcher/scientist with research interests that focus solely on rickettsiae or other vector-borne bacteria. In an attempt to survive in their careers, the majority of researchers are involved in surveillance for multiple pathogens across different classes. Hence, many scientists combine their research on vector-borne microorganisms (including protozoans and bacterial and viral pathogens). Nevertheless, extensive research on rickettsia has been conducted by a handful of scientists from North Africa, mostly from the Mediterranean region. This is partly supported by research collaborators from Europe cutting across Spain and France, which are part of the Mediterranean region. On the contrary, molecular surveillance has been carried out in other parts of Africa, but more focused research is needed to understand the eco-epidemiology of TBR in the continent. Specific aspects of the epidemiology that warrant further investigation include characterization of Rickettsia species from the blood of domestic vertebrate hosts, wild animals, and tick vectors infesting these animals on a large scale. In addition, knowledge of the roles of small rodents and avian species in the epidemiology of rickettsial pathogens in Africa is lacking. A large-scale survey of Rickettsiae in host-seeking ticks is lacking in some parts of the continent. Other aspects include the kinetics of infection in vertebrate hosts and the vectorial capacity of common and geographically dispersed tick species to transmit rickettsial pathogens to animals and humans. Lastly, whole genome sequencing (WGS) of some of the detected rickettsial species needs to be performed to obtain high-level information on the bacteria circulating within the continent. To circumvent all these challenges, we propose that a multidisciplinary alliance of skilled experts from a broad range of individuals and institutions come together to enhance capacity and unveil additional knowledge on molecular, biological, environmental, and socioeconomic factors the common good of humanity. This alliance will help achieve the surveillance and control of rickettsiosis across the continent and further help to achieve sustainable control of rickettsiosis. Finally, there is a need for more decent animal or laboratory facilities in many parts of Africa, as only a few countries can boast a reasonable number of facilities to carry out good research.
Concluding remarks and future directions
The genus Rickettsia encompasses a diverse assemblage (> 30 species) of obligate intracellular coccobacilli with a unique and fascinating biology. Since the discovery of rickettsiae over a century ago, the study of their biology has been challenging, largely in part because of their intracellular nature. With several neglected but globally emerging human pathogens within this genus, it is critical to gain a better understanding of the distribution and epidemiology of these pathogens across Africa. In this review, we have highlighted broadly and in detail, the epidemiological relationship between ticks and rickettsial pathogens in Africa looking at the prevalence, distribution, and major types of rickettsial diseases in humans and animals, and reports on the detection of SFG Rickettsia in different tick vectors across Africa. We discussed the role of domestic animals, birds, small mammals, and wildlife in the epidemiology of SFG rickettsiae across Africa and provide insight into the problems of rickettsial diagnosis and reports in Africa. Several knowledge gaps have been identified that need to be filled, and we propose that research efforts should be directed to help solve these contributing issues.
Given the diverse ecological conditions across the African continent, it is evident that a wide range of ecosystems facilitate the development of numerous classes of arthropods, especially ticks and mammalian hosts. Therefore, we can assume that many undescribed rickettsial species still exist in the continent, demanding improvements in surveillance for SFG Rickettsia across wildlife, domestic animals, avian hosts, livestock workers, agricultural workers, forest guards, veterinarians, and people living in poor rural and urban settlements in Africa. Furthermore, we advocate for the “One Health” approach to the study of rickettsial diseases in the continent.
Despite the economic importance of rickettsial disease and its potential as an emerging and re-emerging disease, little attention or recognition has been paid to it. This suggests the need to increase awareness among patients, clinicians, and scientists. In addition, there is a need to map and identify the geographical foci of various Rickettsia species and/or diseases, allowing the characterization of vectors and reservoirs of the disease within that particular area. To improve the epidemiological surveillance of SFG rickettsioses, among patients with febrile illness, rickettsial infections should be included as a differential diagnosis in patients with non-malarial febrile illnesses. Unlike in most parts of the world, where reasonable studies on vector competence for SFG rickettsiae have been undertaken, there is a dearth of experimental studies using field-collected tick samples in Africa to evaluate their vectorial capacity to transmit Rickettsia species. Therefore, we encourage more transmission experiments to be undertaken, and studies evaluating the potential of some vertebrate hosts to serve as amplifying hosts need to be conducted.
The development and use of molecular diagnostics, including PCR and sequencing, and the use of ticks as specimens, have resulted in a better understanding of the repertoire of tick-associated rickettsia. The true prevalence of SFG rickettsiae is underreported, with a resulting impact on funding and public health decisions (195). With global warming largely driven by climate changes, it is becoming apparent that the geographical range of rickettsial diseases is expanding significantly. Training physicians and/or clinicians in various parts of the world must be harmonized so that teaching on VBD includes rickettsial diseases, ensuring early emphasis on these diseases, and their inclusion as a differential diagnostic together with the current practice focused mainly on Plasmodium, Leishmania, and Trypanosoma parasites. In Africa, the majority of DNA-based detection and characterization of Rickettsia have solely been based on the use of short DNA sequences generated from Sanger sequencing following PCR amplification. Whole-genome sequence data from Africa derived from tick vectors, wildlife, and humans are scarce in public genomic repositories and/or GenBank. We advocate for more whole-genome sequence data from African isolates, particularly from tick vectors and humans. These genomic data will be used for a more in-depth core genome phylogenetic analysis, classification, and taxonomy of Rickettsia species within the continent.
We propose that future studies should be designed and conducted in such a way that there is comprehensive sampling and increased genome sequencing of positive Rickettsia species from diverse vertebrate hosts and vectors from different ecosystems, which will help provide great insight into the evolution and diversification within this genus. Furthermore, future studies in Africa should attempt to unravel the molecular determinants responsible for the gene expression patterns observed within different vector tissues as well as the epidemiological implications of vector microbiota and rickettsial coinfections on the emergence of TBR within Africa.
Author contributions
TO perform the majority of the review of literature and initial manuscript design. TO, TS and ML were responsible for manuscript revisions, preparation and decision to publish. All authors contributed to the article and approved the submitted version.
Funding
This work was supported with funds from the NIAID Division of Intramural Research.
Acknowledgments
We gratefully acknowledge support from the University of Maiduguri, Nigeria. The technical assistance of Mpho Tawana (NWU- Potchefstroom campus, South Africa) in creating the heat map is hereby acknowledged. We thank Rose Perry-Gottschalk from the Research Technologies Branch, NIAID, NIH for creating the figures.
Conflict of interest
The authors declare that the research was conducted in the absence of any commercial or financial relationships that could be construed as a potential conflict of interest.
Publisher’s note
All claims expressed in this article are solely those of the authors and do not necessarily represent those of their affiliated organizations, or those of the publisher, the editors and the reviewers. Any product that may be evaluated in this article, or claim that may be made by its manufacturer, is not guaranteed or endorsed by the publisher.
References
1. Dantas-Torres F, Otranto D. Best practices for preventing vector-borne diseases in dogs and humans. Trends Parasitol (2016) 32(1):43–55. doi: 10.1016/j.pt.2015.09.004
2. Salje J, Weitzel T, Newton PN, Varghese GM, Day N. Rickettsial infections: A blind spot in our view of neglected tropical diseases. PLoS Negl Trop Dis (2021) 15(5):e0009353. doi: 10.1371/journal.pntd.0009353
3. Diop A, Raoult D, Fournier PE. Paradoxical evolution of rickettsial genomes. Ticks Tick Borne Dis (2019) 10(2):462–9. doi: 10.1016/j.ttbdis.2018.11.007
4. Kernif T, Socolovschi C, Bitam I, Raoult D, Parola P. Vector-borne rickettsioses in north Africa. Infect Dis Clin North Am (2012) 26(2):455–78. doi: 10.1016/j.idc.2012.03.007
5. Minahan NT, Chao CC, Tsai KH. The re-emergence and emergence of vector-borne rickettsioses in Taiwan. Trop Med Infect Dis (2017) 3(1):1–19. doi: 10.3390/tropicalmed3010001
6. Kernif T, Leulmi H, Raoult D, Parola P. Emerging tick-borne bacterial pathogens. Microbiol Spectr (2016) 4(3):1–11. doi: 10.1128/microbiolspec.EI10-0012-2016
7. Ammerman NC, Swanson KI, Anderson JM, Schwartz TR, Seaberg EC, Glass GE, et al. Spotted-fever group rickettsia in dermacentor variabilis, Maryland. Emerg Infect Dis (2004) 10(8):1478–81. doi: 10.3201/eid1008.030882
8. Parola P, Paddock CD, Raoult D. Tick-borne rickettsioses around the world: Emerging diseases challenging old concepts. Clin Microbiol Rev (2005) 18(4):719–56. doi: 10.1128/CMR.18.4.719-756.2005
9. Fournier PE, Raoult D. Tick-borne spotted fever rickettsioses. In: Ryan ETHD, Solomon T, Endy TP, Aronson N, editors. Hunter's tropical medicine and emerging infectious diseases, 10th ed, vol. p. Philadelphia: Elseviers (2020). p. 587–93.
10. Estrada-Pena A, Binder LC, Nava S, Szabo MPJ, Labruna MB. Exploring the ecological and evolutionary relationships between rickettsia and hard ticks in the Neotropical region. Ticks Tick Borne Dis (2021) 12(5):101754. doi: 10.1016/j.ttbdis.2021.101754
11. Parola P, Paddock CD, Socolovschi C, Labruna MB, Mediannikov O, Kernif T, et al. Update on tick-borne rickettsioses around the world: A geographic approach. Clin Microbiol Rev (2013) 26(4):657–702. doi: 10.1128/CMR.00032-13
12. Bitam I. Vectors of rickettsiae in Africa. Ticks Tick Borne Dis (2012) 3(5-6):382–6. doi: 10.1016/j.ttbdis.2012.10.011
13. Abdel-Shafy S, Abdullah H, El-Molla A, Salib A, Ghazy A. Epidemiology and diagnosis of rickettsiosis in animal hosts and tick vectors. Bulg J Vet Med (2018) 22:371–98. doi: 10.15547/bjvm.2137
14. Burgdorfer W, Brinton LP. Mechanisms of transovarial infection of spotted fever rickettsiae in ticks. Ann NY Acad Sci (1975) 266:61–72. doi: 10.1111/j.1749-6632.1975.tb35088.x
15. Nieri-Bastos FA, Szabo MP, Pacheco RC, Soares JF, Soares HS, Moraes-Filho J, et al. Comparative evaluation of infected and noninfected amblyomma triste ticks with rickettsia parkeri, the agent of an emerging rickettsiosis in the new world. BioMed Res Int (2013) 2013:402737. doi: 10.1155/2013/402737
16. Krawczak FS, Agostinho WC, Polo G, Moraes-Filho J, Labruna MB. Comparative evaluation of amblyomma ovale ticks infected and noninfected by rickettsia sp. strain Atlantic rainforest, the agent of an emerging rickettsiosis in Brazil. Ticks Tick Borne Dis (2016) 7(3):502–7. doi: 10.1016/j.ttbdis.2016.02.007
17. Gerardi M, Ramirez-Hernandez A, Binder LC, Krawczak FS, Gregori F, Labruna MB. Comparative susceptibility of different populations of amblyomma sculptum to rickettsia rickettsii. Front Physiol (2019) 10:653. doi: 10.3389/fphys.2019.00653
18. Labruna MB. Ecology of rickettsia in south America. Ann NY Acad Sci (2009) 1166:156–66. doi: 10.1111/j.1749-6632.2009.04516.x
19. Polo G, Mera Acosta C, Labruna MB, Ferreira F. Transmission dynamics and control of rickettsia rickettsii in populations of hydrochoerus hydrochaeris and amblyomma sculptum. PLoS Negl Trop Dis (2017) 11(6):e0005613. doi: 10.1371/journal.pntd.0005613
20. Freedman DO, Weld LH, Kozarsky PE, Fisk T, Robins R, von Sonnenburg F, et al. Spectrum of disease and relation to place of exposure among ill returned travelers. N Engl J Med (2006) 354(2):119–30. doi: 10.1056/NEJMoa051331
21. Jensenius M, Davis X, von Sonnenburg F, Schwartz E, Keystone JS, Leder K, et al. Multicenter geosentinel analysis of rickettsial diseases in international travelers, 1996-2008. Emerg Infect Dis (2009) 15(11):1791–8. doi: 10.3201/eid1511.090677
22. Piotrowski M, Rymaszewska A. Expansion of tick-borne rickettsioses in the world. Microorganisms (2020) 8(12):1–28. doi: 10.3390/microorganisms8121906
23. Saito TB, Bechelli J, Smalley C, Karim S, Walker DH. Vector tick transmission model of spotted fever rickettsiosis. Am J Pathol (2019) 189(1):115–23. doi: 10.1016/j.ajpath.2018.09.005
24. Ledger KJ, Beati L, Wisely SM. Survey of ticks and tick-borne rickettsial and protozoan pathogens in eswatini. Pathogens (2021) 10(8):1–25. doi: 10.3390/pathogens10081043
25. Demoncheaux JP, Socolovschi C, Davoust B, Haddad S, Raoult D, Parola P. First detection of rickettsia aeschlimannii in hyalomma dromedarii ticks from Tunisia. Ticks Tick Borne Dis (2012) 3(5-6):398–402. doi: 10.1016/j.ttbdis.2012.10.003
26. Socolovschi C, Parola P, Raoult D. Tick-borne spotted fever rickettsioses. In: Magill AJRE, Hill DR, Solomon T, editors. Hunter's tropical medicine and emerging infectious disease, 9th ed, vol. . p . Saunders, London: Elsevier (2013). p. 546–52.
27. Merhej V, Angelakis E, Socolovschi C, Raoult D. Genotyping, evolution and epidemiological findings of rickettsia species. Infect Genet Evol (2014) 25:122–37. doi: 10.1016/j.meegid.2014.03.014
28. Fournier PE, Dumler JS, Greub G, Zhang J, Wu Y, Raoult D. Gene sequence-based criteria for identification of new rickettsia isolates and description of rickettsia heilongjiangensis sp. Nov. J Clin Microbiol (2003) 41(12):5456–65. doi: 10.1128/JCM.41.12.5456-5465.2003
29. Weisburg WG, Dobson ME, Samuel JE, Dasch GA, Mallavia LP, Baca O, et al. Phylogenetic diversity of the rickettsiae. J Bacteriol (1989) 171(8):4202–6. doi: 10.1128/jb.171.8.4202-4206.1989
30. Roux V, Rydkina E, Eremeeva M, Raoult D. Citrate synthase gene comparison, a new tool for phylogenetic analysis, and its application for the rickettsiae. Int J Syst Bacteriol (1997) 47(2):252–61. doi: 10.1099/00207713-47-2-252
31. Ngwamidiba M, Blanc G, Ogata H, Raoult D, Fournier PE. Phylogenetic study of rickettsia species using sequences of the autotransporter protein-encoding gene Sca2. Ann NY Acad Sci (2005) 1063:94–9. doi: 10.1196/annals.1355.015
32. Ngwamidiba M, Blanc G, Raoult D, Fournier PE. Sca1, a previously undescribed paralog from autotransporter protein-encoding genes in rickettsia species. BMC Microbiol (2006) 6:12. doi: 10.1186/1471-2180-6-12
33. Roux V, Raoult D. Phylogenetic analysis of members of the genus rickettsia using the gene encoding the outer-membrane protein rompb (Ompb). Int J Syst Evol Microbiol (2000) 50 Pt 4:1449–55. doi: 10.1099/00207713-50-4-1449
34. Sekeyova Z, Roux V, Raoult D. Phylogeny of rickettsia spp. inferred by comparing sequences of 'Gene d', which encodes an intracytoplasmic protein. Int J Syst Evol Microbiol (2001) 51(Pt 4):1353–60. doi: 10.1099/00207713-51-4-1353
35. Merhej V, El Karkouri K, Raoult D. Whole genome-based phylogenetic analysis of rickettsiae. Clin Microbiol Infect (2009) 15 Suppl 2:336–7. doi: 10.1111/j.1469-0691.2008.02265.x
36. El Karkouri K, Ghigo E, Raoult D, Fournier PE. Genomic evolution and adaptation of arthropod-associated rickettsia. Sci Rep (2022) 12(1):3807. doi: 10.1038/s41598-022-07725-z
37. Hsi TE, Hsiao SW, Minahan NT, Yen TY, de Assuncao Carvalho AV, Raoult D, et al. Seroepidemiological and molecular investigation of spotted fever group rickettsiae and coxiella burnetii in sao tome island: A one health approach. Transbound Emerg Dis (2020) 67 Suppl 2:36–43. doi: 10.1111/tbed.13191
38. Tufa TB, Wolfel S, Zubrikova D, Vichova B, Andersson M, Riess R, et al. Tick species from cattle in the adama region of Ethiopia and pathogens detected. Exp Appl Acarol (2021) 84(2):459–71. doi: 10.1007/s10493-021-00623-5
39. Mediannikov O, Davoust B, Socolovschi C, Tshilolo L, Raoult D, Parola P. Spotted fever group rickettsiae in ticks and fleas from the democratic republic of the Congo. Ticks Tick Borne Dis (2012) 3(5-6):371–3. doi: 10.1016/j.ttbdis.2012.10.015
40. Mediannikov O, Diatta G, Zolia Y, Balde MC, Kohar H, Trape JF, et al. Tick-borne rickettsiae in Guinea and Liberia. Ticks Tick Borne Dis (2012) 3(1):43–8. doi: 10.1016/j.ttbdis.2011.08.002
41. Tomassone L, De Meneghi D, Adakal H, Rodighiero P, Pressi G, Grego E. Detection of rickettsia aeschlimannii and rickettsia africae in ixodid ticks from Burkina Faso and Somali region of Ethiopia by new real-time pcr assays. Ticks Tick Borne Dis (2016) 7(6):1082–8. doi: 10.1016/j.ttbdis.2016.09.005
42. Omondi D, Masiga DK, Fielding BC, Kariuki E, Ajamma YU, Mwamuye MM, et al. Molecular detection of tick-borne pathogen diversities in ticks from livestock and reptiles along the shores and adjacent islands of lake Victoria and lake baringo, Kenya. Front Vet Sci (2017) 4:73. doi: 10.3389/fvets.2017.00073
43. Koka H, Sang R, Kutima HL, Musila L. The detection of spotted fever group rickettsia DNA in tick samples from pastoral communities in Kenya. J Med Entomol (2017) 54(3):774–80. doi: 10.1093/jme/tjw238
44. Byaruhanga C, Akure PC, Lubembe DM, Sibeko-Matjila K, Troskie M, Oosthuizen MC, et al. Molecular detection and characterisation of protozoan and rickettsial pathogens in ticks from cattle in the pastoral area of karamoja, Uganda. Ticks Tick Borne Dis (2021) 12(4):101709. doi: 10.1016/j.ttbdis.2021.101709
45. Kolo AO, Sibeko-Matjila KP, Maina AN, Richards AL, Knobel DL, Matjila PT. Molecular detection of zoonotic rickettsiae and anaplasma spp. in domestic dogs and their ectoparasites in bushbuckridge, south Africa. Vector Borne Zoonotic Dis (2016) 16(4):245–52. doi: 10.1089/vbz.2015.1849
46. Jongejan F, Berger L, Busser S, Deetman I, Jochems M, Leenders T, et al. Amblyomma hebraeum is the predominant tick species on goats in the mnisi community area of mpumalanga province south Africa and is Co-infected with ehrlichia ruminantium and rickettsia africae. Parasit Vectors (2020) 13(1):172. doi: 10.1186/s13071-020-04059-5
47. Pillay AD, Mukaratirwa S. Genetic diversity of rickettsia africae isolates from amblyomma hebraeum and blood from cattle in the Eastern cape province of south Africa. Exp Appl Acarol (2020) 82(4):529–41. doi: 10.1007/s10493-020-00555-6
48. Horton KC, Jiang J, Maina A, Dueger E, Zayed A, Ahmed AA, et al. Evidence of rickettsia and orientia infections among abattoir workers in Djibouti. Am J Trop Med Hyg (2016) 95(2):462–5. doi: 10.4269/ajtmh.15-0775
49. Lorusso V, Gruszka KA, Majekodunmi A, Igweh A, Welburn SC, Picozzi K. Rickettsia africae in amblyomma variegatum ticks, Uganda and Nigeria. Emerg Infect Dis (2013) 19(10):1705–7. doi: 10.3201/eid1910.130389
50. Vanegas A, Keller C, Kruger A, Manchang TK, Hagen RM, Frickmann H, et al. Molecular detection of spotted fever group rickettsiae in ticks from Cameroon. Ticks Tick Borne Dis (2018) 9(4):1049–56. doi: 10.1016/j.ttbdis.2018.03.022
51. Yssouf A, Socolovschi C, Kernif T, Temmam S, Lagadec E, Tortosa P, et al. First molecular detection of rickettsia africae in ticks from the union of the Comoros. Parasit Vectors (2014) 7:444. doi: 10.1186/1756-3305-7-444
52. Ehounoud CB, Yao KP, Dahmani M, Achi YL, Amanzougaghene N, Kacou N'Douba A, et al. Multiple pathogens including potential new species in tick vectors in cote d'ivoire. PLoS Negl Trop Dis (2016) 10(1):e0004367. doi: 10.1371/journal.pntd.0004367
53. Reye AL, Arinola OG, Hubschen JM, Muller CP. Pathogen prevalence in ticks collected from the vegetation and livestock in Nigeria. Appl Environ Microbiol (2012) 78(8):2562–8. doi: 10.1128/AEM.06686-11
54. DIOBO FN, YAO PK, DIAHA ACA, ADJOGOUA EV, FAYE HK. Detection of rickettsia africae in ticks and cattle in côte d'ivoire by real-time pcr. J Appl Biosci (2021) 166:17242–51. doi: 10.35759/JABs.166.8
55. Adjou Moumouni PF, Terkawi MA, Jirapattharasate C, Cao S, Liu M, Nakao R, et al. Molecular detection of spotted fever group rickettsiae in amblyomma variegatum ticks from Benin. Ticks Tick Borne Dis (2016) 7(5):828–33. doi: 10.1016/j.ttbdis.2016.03.016
56. Diarra AZ, Almeras L, Laroche M, Berenger JM, Kone AK, Bocoum Z, et al. Molecular and maldi-tof identification of ticks and tick-associated bacteria in Mali. PLoS Negl Trop Dis (2017) 11(7):e0005762. doi: 10.1371/journal.pntd.0005762
57. Sambou M, Faye N, Bassene H, Diatta G, Raoult D, Mediannikov O. Identification of rickettsial pathogens in ixodid ticks in northern Senegal. Ticks Tick Borne Dis (2014) 5(5):552–6. doi: 10.1016/j.ttbdis.2014.04.002
58. Nakayima J, Hayashida K, Nakao R, Ishii A, Ogawa H, Nakamura I, et al. Detection and characterization of zoonotic pathogens of free-ranging non-human primates from Zambia. Parasit Vectors (2014) 7:490. doi: 10.1186/s13071-014-0490-x
59. Barradas PF, Vilhena H, Oliveira AC, Granada S, Amorim I, Ferreira P, et al. Serological and molecular detection of spotted fever group rickettsia in a group of pet dogs from Luanda, Angola. Parasit Vectors (2017) 10(1):271. doi: 10.1186/s13071-017-2216-3
60. Guo H, Adjou Moumouni PF, Thekisoe O, Gao Y, Liu M, Li J, et al. Genetic characterization of tick-borne pathogens in ticks infesting cattle and sheep from three south African provinces. Ticks Tick Borne Dis (2019) 10(4):875–82. doi: 10.1016/j.ttbdis.2019.04.008
61. Halajian A, Palomar AM, Portillo A, Heyne H, Romero L, Oteo JA. Detection of zoonotic agents and a new rickettsia strain in ticks from donkeys from south Africa: Implications for travel medicine. Travel Med Infect Dis (2018) 26:43–50. doi: 10.1016/j.tmaid.2018.10.007
62. Matsimbe AM, Magaia V, Sanches GS, Neves L, Noormahomed E, Antunes S, et al. Molecular detection of pathogens in ticks infesting cattle in nampula province, Mozambique. Exp Appl Acarol (2017) 73(1):91–102. doi: 10.1007/s10493-017-0155-5
63. Nakao R, Qiu Y, Salim B, Hassan SM, Sugimoto C. Molecular detection of rickettsia africae in amblyomma variegatum collected from Sudan. Vector Borne Zoonotic Dis (2015) 15(5):323–5. doi: 10.1089/vbz.2014.1748
64. Leulmi H, Aouadi A, Bitam I, Bessas A, Benakhla A, Raoult D, et al. Detection of bartonella tamiae, coxiella burnetii and rickettsiae in arthropods and tissues from wild and domestic animals in northeastern Algeria. Parasit Vectors (2016) 9:27. doi: 10.1186/s13071-016-1316-9
65. Kamani J, Baneth G, Mumcuoglu KY, Waziri NE, Eyal O, Guthmann Y, et al. Molecular detection and characterization of tick-borne pathogens in dogs and ticks from Nigeria. PLoS Negl Trop Dis (2013) 7(3):e2108. doi: 10.1371/journal.pntd.0002108
66. Proboste T, Kalema-Zikusoka G, Altet L, Solano-Gallego L, Fernandez de Mera IG, Chirife AD, et al. Infection and exposure to vector-borne pathogens in rural dogs and their ticks, Uganda. Parasit Vectors (2015) 8:306. doi: 10.1186/s13071-015-0919-x
67. Bitam I, Parola P, Matsumoto K, Rolain JM, Baziz B, Boubidi SC, et al. First molecular detection of r. conorii, r. aeschlimannii, and r. massiliae in ticks from Algeria. Ann N Y Acad Sci (2006) 1078:368–72. doi: 10.1196/annals.1374.073
68. Onyiche TE, Raileanu C, Tauchmann O, Fischer S, Vasic A, Schafer M, et al. Prevalence and molecular characterization of ticks and tick-borne pathogens of one-humped camels (Camelus dromedarius) in Nigeria. Parasit Vectors (2020) 13(1):428. doi: 10.1186/s13071-020-04272-2
69. Morita C, El Hussein AR, Matsuda E, Abdel Gabbar KM, Muramatsu Y, Abdel Rahman MB, et al. Spotted fever group rickettsiae from ticks captured in Sudan. Jpn J Infect Dis (2004) 57(3):107–9.
70. Selmi R, Ben Said M, Ben Yahia H, Abdelaali H, Messadi L. Molecular epidemiology and phylogeny of spotted fever group rickettsia in camels (Camelus dromedarius) and their infesting ticks from Tunisia. Transbound Emerg Dis (2020) 67(2):733–44. doi: 10.1111/tbed.13392
71. Shuaib YA, Elhag AMW, Brima YA, Abdalla MA, Bakiet AO, Mohmed-Noor SE, et al. Ixodid tick species and two tick-borne pathogens in three areas in the Sudan. Parasitol Res (2020) 119(2):385–94. doi: 10.1007/s00436-019-06458-9
72. Kernif T, Djerbouh A, Mediannikov O, Ayach B, Rolain JM, Raoult D, et al. Rickettsia africae in hyalomma dromedarii ticks from Sub-Saharan Algeria. Ticks Tick Borne Dis (2012) 3(5-6):377–9. doi: 10.1016/j.ttbdis.2012.10.013
73. Loftis AD, Reeves WK, Szumlas DE, Abbassy MM, Helmy IM, Moriarity JR, et al. Rickettsial agents in Egyptian ticks collected from domestic animals. Exp Appl Acarol (2006) 40(1):67–81. doi: 10.1007/s10493-006-9025-2
74. Kamani J, Baneth G, Apanaskevich DA, Mumcuoglu KY, Harrus S. Molecular detection of rickettsia aeschlimannii in hyalomma spp. ticks from camels (Camelus dromedarius) in Nigeria, West Africa. Med Vet Entomol (2015) 29(2):205–9. doi: 10.1111/mve.12094
75. Beati L, Meskini M, Thiers B, Raoult D. Rickettsia aeschlimannii sp. nov., a new spotted fever group rickettsia associated with hyalomma marginatum ticks. Int J Syst Bacteriol (1997) 47(2):548–54. doi: 10.1099/00207713-47-2-548
76. Mediannikov O, Diatta G, Fenollar F, Sokhna C, Trape JF, Raoult D. Tick-borne rickettsioses, neglected emerging diseases in rural Senegal. PLoS Negl Trop Dis (2010) 4(9):1–13. doi: 10.1371/journal.pntd.0000821
77. Djerbouh A, Kernif T, Beneldjouzi A, Socolovschi C, Kechemir N, Parola P, et al. The first molecular detection of rickettsia aeschlimannii in the ticks of camels from southern Algeria. Ticks Tick Borne Dis (2012) 3(5-6):374–6. doi: 10.1016/j.ttbdis.2012.10.014
78. Parola P, Inokuma H, Camicas JL, Brouqui P, Raoult D. Detection and identification of spotted fever group rickettsiae and ehrlichiae in African ticks. Emerg Infect Dis (2001) 7(6):1014–7. doi: 10.3201/eid0706.010616
79. Mura A, Socolovschi C, Ginesta J, Lafrance B, Magnan S, Rolain JM, et al. Molecular detection of spotted fever group rickettsiae in ticks from Ethiopia and Chad. Trans R Soc Trop Med Hyg (2008) 102(9):945–9. doi: 10.1016/j.trstmh.2008.03.015
80. Beati L, Kelly PJ, Matthewman LA, Mason PR, Raoult D. Prevalence of rickettsia-like organisms and spotted fever group rickettsiae in ticks (Acari: Ixodidae) from Zimbabwe. J Med Entomol (1995) 32(6):787–92. doi: 10.1093/jmedent/32.6.787
81. Kernif T, Messaoudene D, Ouahioune S, Parola P, Raoult D, Bitam I. Spotted fever group rickettsiae identified in dermacentor marginatus and ixodes ricinus ticks in Algeria. Ticks Tick Borne Dis (2012) 3(5-6):380–1. doi: 10.1016/j.ttbdis.2012.10.012
82. Teshale S, Kumsa B, Menandro ML, Cassini R, Martini M. Anaplasma, ehrlichia and rickettsial pathogens in ixodid ticks infesting cattle and sheep in Western oromia, Ethiopia. Exp Appl Acarol (2016) 70(2):231–7. doi: 10.1007/s10493-016-0067-9
83. Iweriebor B. Molecular screening of ticks for the presence of rickettsia species: A public health concern. Asian Pacific J Trop Dis (2017) 7:199–204. doi: 10.12980/apjtd.7.2017D6-411
84. Belkahia H, Selmi R, Zamiti S, Daaloul-Jedidi M, Messadi L, Ben Said M. Zoonotic rickettsia species in small ruminant ticks from Tunisia. Front Vet Sci (2021) 8:676896. doi: 10.3389/fvets.2021.676896
85. Znazen A, Khrouf F, Elleuch N, Lahiani D, Marrekchi C, M'Ghirbi Y, et al. Multispacer typing of rickettsia isolates from humans and ticks in Tunisia revealing new genotypes. Parasit Vectors (2013) 6:367. doi: 10.1186/1756-3305-6-367
86. Berrelha J, Briolant S, Muller F, Rolain JM, Marie JL, Pages F, et al. Rickettsia felis and rickettsia massiliae in ivory coast, Africa. Clin Microbiol Infect (2009) 15 Suppl 2:251–2. doi: 10.1111/j.1469-0691.2008.02273.x
87. Kelly PJ, Beati L, Mason PR, Matthewman LA, Roux V, Raoult D. Rickettsia africae sp. nov., the etiological agent of African tick bite fever. Int J Syst Bacteriol (1996) 46(2):611–4. doi: 10.1099/00207713-46-2-611
88. Jensenius M, Fournier PE, Kelly P, Myrvang B, Raoult D. African Tick bite fever. Lancet Infect Dis (2003) 3(9):557–64. doi: 10.1016/s1473-3099(03)00739-4
89. Frean J, Grayson W. South African tick bite fever: An overview. Dermatopathology (Basel) (2019) 6(2):70–6. doi: 10.1159/000495475
91. Delord M, Socolovschi C, Parola P. Rickettsioses and q fever in travelers (2004-2013). Travel Med Infect Dis (2014) 12(5):443–58. doi: 10.1016/j.tmaid.2014.08.006
92. Eldin C, Parola P. Update on tick-borne bacterial diseases in travelers. Curr Infect Dis Rep (2018) 20(7):17. doi: 10.1007/s11908-018-0624-y
93. Zammarchi L, Farese A, Trotta M, Amantini A, Raoult D, Bartoloni A. Rickettsia africae infection complicated with painful sacral syndrome in an Italian traveller returning from Zimbabwe. Int J Infect Dis (2014) 29:194–6. doi: 10.1016/j.ijid.2014.10.017
94. Duval R, Merrill PT. Spotted fever group rickettsia retinitis in a traveler to Africa. Retin Cases Brief Rep (2016) 10(1):89–92. doi: 10.1097/ICB.0000000000000168
95. Althaus F, Greub G, Raoult D, Genton B. African Tick-bite fever: A new entity in the differential diagnosis of multiple eschars in travelers. description of five cases imported from south Africa to Switzerland. Int J Infect Dis (2010) 14 Suppl 3:e274–6. doi: 10.1016/j.ijid.2009.11.021
96. Ndip LM, Fokam EB, Bouyer DH, Ndip RN, Titanji VP, Walker DH, et al. Detection of rickettsia africae in patients and ticks along the coastal region of Cameroon. Am J Trop Med Hyg (2004) 71(3):363–6. doi: 10.4269/ajtmh.2004.71.363
97. Mouffok N, Parola P, Lepidi H, Raoult D. Mediterranean Spotted fever in Algeria–new trends. Int J Infect Dis (2009) 13(2):227–35. doi: 10.1016/j.ijid.2008.06.035
98. Conor A, Brush A. Une fievre boutonneuse observee en tunisie. Bull la société Pathologie Exotiques Filiales (1910) 8:492–6.
99. Durand P, Conseil E. Transmission expérimentale de la fièvre boutonneuse par rhipicephalus sanguineus. CR Acad Sci [D] (1930) 190:1244.
100. Rovery C, Raoult D. Mediterranean Spotted fever. Infect Dis Clin North Am (2008) 22(3):515–30. doi: 10.1016/j.idc.2008.03.003
101. Milhano N, Saito TB, Bechelli J, Fang R, Vilhena M, DES R, et al. The role of rhipicephalus sanguineus sensu lato saliva in the dissemination of rickettsia conorii in C3h/Hej mice. Med Vet Entomol (2015) 29(3):225–9. doi: 10.1111/mve.12118
102. Parola P, Socolovschi C, Jeanjean L, Bitam I, Fournier PE, Sotto A, et al. Warmer weather linked to tick attack and emergence of severe rickettsioses. PLoS Negl Trop Dis (2008) 2(11):e338. doi: 10.1371/journal.pntd.0000338
103. Boudebouch N, Sarih M, Socolovschi C, Amarouch H, Hassar M, Raoult D, et al. Molecular survey for spotted fever group rickettsiae in ticks from Morocco. Clin Microbiol Infect (2009) 15 Suppl 2:259–60. doi: 10.1111/j.1469-0691.2008.02226.x
104. Kaabia N, Bellazreg F, Hachfi W, Khalifa M, Ghanouchi N, Bahri F, et al. Rickettsial infection in hospitalised patients in central Tunisia: Report of 119 cases. Clin Microbiol Infect (2009) 15 Suppl 2:216–7. doi: 10.1111/j.1469-0691.2008.02139.x
105. Doube BM, Kemp DH. The influence of temperature, relative humidity and host factors on the attachment and survival of boophilus microplus (Canestrini) larvae to skin slices. Int J Parasitol (1979) 9(5):449–54. doi: 10.1016/0020-7519(79)90048-1
106. Amoo A, Dipeolu OO. The effects of temperature, relative humidity and host factors on the attachment and survival of boophilus decoloratus and boophilus geigyi larvae to skin slices. Folia Parasitol (Praha) (1985) 32(1):83–8.
107. Goldwasser RA, Steiman Y, Klingberg W, Swartz TA, Klingberg MA. The isolation of strains of rickettsiae of the spotted fever group in Israel and their differentiation from other members of the group by immunofluorescence methods. Scand J Infect Dis (1974) 6(1):53–62. doi: 10.3109/inf.1974.6.issue-1.10
108. Zhu Y, Fournier PE, Eremeeva M, Raoult D. Proposal to create subspecies of rickettsia conorii based on multi-locus sequence typing and an emended description of rickettsia conorii. BMC Microbiol (2005) 5:11. doi: 10.1186/1471-2180-5-11
109. Boillat N, Genton B, D'Acremont V, Raoult D, Greub G. Fatal case of Israeli spotted fever after Mediterranean cruise. Emerg Infect Dis (2008) 14(12):1944–6. doi: 10.3201/eid1412.070641
110. Gross EM, Yagupsky P. Israeli Rickettsial spotted fever in children. a review of 54 cases. Acta Trop (1987) 44(1):91–6.
111. Wolach B, Franco S, Bogger-Goren S, Drucker M, Goldwasser RA, Sadan N, et al. Clinical and laboratory findings of spotted fever in Israeli children. Pediatr Infect Dis J (1989) 8(3):152–5.
112. Giammanco GM, Vitale G, Mansueto S, Capra G, Caleca MP, Ammatuna P. Presence of rickettsia conorii subsp. israelensis, the causative agent of Israeli spotted fever, in Sicily, Italy, ascertained in a retrospective study. J Clin Microbiol (2005) 43(12):6027–31. doi: 10.1128/JCM.43.12.6027-6031.2005
113. Znazen A, Hammami B, Lahiani D, Ben Jemaa M, Hammami A. Israeli Spotted fever, Tunisia. Emerg Infect Dis (2011) 17(7):1328–30. doi: 10.3201/eid1707.101648
114. Philip RN, Casper EA, Burgdorfer W, Gerloff RK, Hughes LE, Bell EJ. Serologic typing of rickettsiae of the spotted fever group by microimmunofluorescence. J Immunol (1978) 121(5):1961–8.
115. Yu X, Jin Y, Fan M, Xu G, Liu Q, Raoult D. Genotypic and antigenic identification of two new strains of spotted fever group rickettsiae isolated from China. J Clin Microbiol (1993) 31(1):83–8. doi: 10.1128/jcm.31.1.83-88.1993
116. Fournier PE, Gouriet F, Brouqui P, Lucht F, Raoult D. Lymphangitis-associated rickettsiosis, a new rickettsiosis caused by rickettsia sibirica mongolotimonae: Seven new cases and review of the literature. Clin Infect Dis (2005) 40(10):1435–44. doi: 10.1086/429625
117. Fournier PE, Grunnenberger F, Jaulhac B, Gastinger G, Raoult D. Evidence of rickettsia helvetica infection in humans, Eastern France. Emerg Infect Dis (2000) 6(4):389–92. doi: 10.3201/eid0604.000412
118. Nouchi A, Monsel G, Jaspard M, Jannic A, Angelakis E, Caumes E. Rickettsia sibirica mongolitimonae infection in a woman travelling from Cameroon: A case report and review of the literature. J Travel Med (2018) 25(1):1–3. doi: 10.1093/jtm/tax074
119. Norte AC, Harris DJ, Silveira D, Nunes CS, Nuncio MS, Martinez EG, et al. Diversity of microorganisms in hyalomma aegyptium collected from spur-thighed tortoise (Testudo graeca) in north Africa and Anatolia. Transbound Emerg Dis (2021) 69(4):1951–62. doi: 10.1111/tbed.14188
120. Socolovschi C, Mediannikov O, Sokhna C, Tall A, Diatta G, Bassene H, et al. Rickettsia felis-associated uneruptive fever, Senegal. Emerg Infect Dis (2010) 16(7):1140–2. doi: 10.3201/eid1607.100070
121. Raoult D, Fournier PE, Abboud P, Caron F. First documented human rickettsia aeschlimannii infection. Emerg Infect Dis (2002) 8(7):748–9. doi: 10.3201/eid0807.010480
122. Znazen A, Rolain JM, Hammami A, Jemaa MB, Raoult D. Rickettsia felis infection, Tunisia. Emerg Infect Dis (2006) 12(1):138–40. doi: 10.3201/eid1201.050876
123. Mokrani N, Parola P, Tebbal S, Dalichaouche M, Aouati A, Raoult D. Rickettsia aeschlimannii infection, Algeria. Emerg Infect Dis (2008) 14(11):1814–5. doi: 10.3201/eid1411.071221
124. Pretorius AM, Birtles RJ. Rickettsia aeschlimannii: A new pathogenic spotted fever group rickettsia, south Africa. Emerg Infect Dis (2002) 8(8):874. doi: 10.3201/eid0808.020199
125. Bitam I, Kernif T, Harrat Z, Parola P, Raoult D. First detection of rickettsia aeschlimannii in hyalomma aegyptium from Algeria. Clin Microbiol Infect (2009) 15 Suppl 2:253–4. doi: 10.1111/j.1469-0691.2008.02274.x
126. Kumsa B, Socolovschi C, Raoult D, Parola P. Spotted fever group rickettsiae in ixodid ticks in oromia, Ethiopia. Ticks Tick Borne Dis (2015) 6(1):8–15. doi: 10.1016/j.ttbdis.2014.08.001
127. Matsumoto K, Parola P, Brouqui P, Raoult D. Rickettsia aeschlimannii in hyalomma ticks from Corsica. Eur J Clin Microbiol Infect Dis (2004) 23(9):732–4. doi: 10.1007/s10096-004-1190-9
128. Sarih M, Socolovschi C, Boudebouch N, Hassar M, Raoult D, Parola P. Spotted fever group rickettsiae in ticks, Morocco. Emerg Infect Dis (2008) 14(7):1067–73. doi: 10.3201/eid1407.070096
129. Chitimia-Dobler L, Dobler G, Schaper S, Kupper T, Kattner S, Wolfel S. First detection of rickettsia conorii ssp. caspia in rhipicephalus sanguineus in Zambia. Parasitol Res (2017) 116(11):3249–51. doi: 10.1007/s00436-017-5639-z
130. Garcia-Garcia JC, Portillo A, Nunez MJ, Santibanez S, Castro B, Oteo JA. A patient from Argentina infected with rickettsia massiliae. Am J Trop Med Hyg (2010) 82(4):691–2. doi: 10.4269/ajtmh.2010.09-0662
131. Portillo A, Santibanez S, Garcia-Alvarez L, Palomar AM, Oteo JA. Rickettsioses in Europe. Microbes Infect (2015) 17(11-12):834–8. doi: 10.1016/j.micinf.2015.09.009
132. Raoult D, Fournier PE, Eremeeva M, Graves S, Kelly PJ, Oteo JA, et al. Naming of rickettsiae and rickettsial diseases. Ann NY Acad Sci (2005) 1063:1–12. doi: 10.1196/annals.1355.002
133. Portillo A, de Sousa R, Santibanez S, Duarte A, Edouard S, Fonseca IP, et al. Guidelines for the detection of rickettsia spp. Vector Borne Zoonotic Dis (2017) 17(1):23–32. doi: 10.1089/vbz.2016.1966
134. Matsumoto K, Parola P, Rolain JM, Jeffery K, Raoult D. Detection of "Rickettsia sp. strain uilenbergi" and "Rickettsia sp. strain davousti" in amblyomma tholloni ticks from elephants in Africa. BMC Microbiol (2007) 7:74. doi: 10.1186/1471-2180-7-74
135. Lopez-Velez R, Palomar AM, Oteo JA, Norman FF, Perez-Molina JA, Portillo A. Novel candidatus rickettsia species detected in nostril tick from human, Gabon, 2014. Emerg Infect Dis (2015) 21(2):325–7. doi: 10.3201/eid2102.141048
136. Buysse M, Duron O. Two novel rickettsia species of soft ticks in north Africa: 'Candidatus rickettsia africaseptentrionalis' and 'Candidatus rickettsia mauretanica'. Ticks Tick Borne Dis (2020) 11(3):101376. doi: 10.1016/j.ttbdis.2020.101376
137. Milhano N, Palma M, Marcili A, Nuncio MS, de Carvalho IL, de Sousa R. Rickettsia lusitaniae sp. nov. isolated from the soft tick ornithodoros erraticus (Acarina: Argasidae). Comp Immunol Microbiol Infect Dis (2014) 37(3):189–93. doi: 10.1016/j.cimid.2014.01.006
138. Turebekov N, Abdiyeva K, Yegemberdiyeva R, Dmitrovsky A, Yeraliyeva L, Shapiyeva Z, et al. Prevalence of rickettsia species in ticks including identification of unknown species in two regions in Kazakhstan. Parasit Vectors (2019) 12(1):197. doi: 10.1186/s13071-019-3440-9
139. Cox JA, Visser LG. A family with African tick bite fever. Travel Med Infect Dis (2015) 13(3):274–5. doi: 10.1016/j.tmaid.2015.03.012
140. Reisen W. Epidemiology of vector-borne diseases., p 15–27. Med Veterinary Entomology Elsevier NY (2002) 15–27. doi: 10.1016/B978-012510451-7/50004-9
141. Laukaitis HJ, Macaluso KR. Unpacking the intricacies of rickettsia-vector interactions. Trends Parasitol (2021) 37(8):734–46. doi: 10.1016/j.pt.2021.05.008
142. Socolovschi C, Huynh TP, Davoust B, Gomez J, Raoult D, Parola P. Transovarial and trans-stadial transmission of rickettsiae africae in amblyomma variegatum ticks. Clin Microbiol Infect (2009) 15 Suppl 2:317–8. doi: 10.1111/j.1469-0691.2008.02278.x
143. Moraes-Filho J, Costa FB, Gerardi M, Soares HS, Labruna MB. Rickettsia rickettsii Co-feeding transmission among amblyomma aureolatum ticks. Emerg Infect Dis (2018) 24(11):2041–8. doi: 10.3201/eid2411.180451
144. Parola P, Raoult D. Tick-borne bacterial diseases emerging in Europe. Clin Microbiol Infect (2001) 7(2):80–3. doi: 10.1046/j.1469-0691.2001.00200.x
145. Raoult D, Roux V. Rickettsioses as paradigms of new or emerging infectious diseases. Clin Microbiol Rev (1997) 10(4):694–719. doi: 10.1128/CMR.10.4.694
146. Horak IG, Camicas JL, Keirans JE. The argasidae, ixodidae and nuttalliellidae (Acari: Ixodida): A world list of valid tick names. Exp Appl Acarol (2002) 28(1-4):27–54. doi: 10.1023/a:1025381712339
147. Weinert LA, Araujo-Jnr EV, Ahmed MZ, Welch JJ. The incidence of bacterial endosymbionts in terrestrial arthropods. Proc Biol Sci (2015) 282(1807):20150249. doi: 10.1098/rspb.2015.0249
148. Duron O, Morel O, Noel V, Buysse M, Binetruy F, Lancelot R, et al. Tick-bacteria mutualism depends on b vitamin synthesis pathways. Curr Biol (2018) 28(12):1896–902 e5. doi: 10.1016/j.cub.2018.04.038
149. Hornok S, Szoke K, Meli ML, Sandor AD, Gorfol T, Estok P, et al. Molecular detection of vector-borne bacteria in bat ticks (Acari: Ixodidae, argasidae) from eight countries of the old and new worlds. Parasit Vectors (2019) 12(1):50. doi: 10.1186/s13071-019-3303-4
150. Moreira-Soto RD, Moreira-Soto A, Corrales-Aguilar E, Calderon-Arguedas O, Troyo A. 'Candidatus rickettsia nicoyana': A novel rickettsia species isolated from ornithodoros knoxjonesi in Costa Rica. Ticks Tick Borne Dis (2017) 8(4):532–6. doi: 10.1016/j.ttbdis.2017.02.015
151. Yan P, Qiu Z, Zhang T, Li Y, Wang W, Li M, et al. Microbial diversity in the tick argas japonicus (Acari: Argasidae) with a focus on rickettsia pathogens. Med Vet Entomol (2019) 33(3):327–35. doi: 10.1111/mve.12373
152. Duron O, Binetruy F, Noel V, Cremaschi J, McCoy KD, Arnathau C, et al. Evolutionary changes in symbiont community structure in ticks. Mol Ecol (2017) 26(11):2905–21. doi: 10.1111/mec.14094
153. Norval RA. The limiting effect of host availability for the immature stages on population growth in economically important ixodid ticks. J Parasitol (1979) 65(2):285–7. doi: 10.2307/3280166
154. Hornok S, Abichu G, Meli ML, Tanczos B, Sulyok KM, Gyuranecz M, et al. Influence of the biotope on the tick infestation of cattle and on the tick-borne pathogen repertoire of cattle ticks in Ethiopia. PLoS One (2014) 9(9):e106452. doi: 10.1371/journal.pone.0106452
155. Mutai BK, Wainaina JM, Magiri CG, Nganga JK, Ithondeka PM, Njagi ON, et al. Zoonotic surveillance for rickettsiae in domestic animals in Kenya. Vector Borne Zoonotic Dis (2013) 13(6):360–6. doi: 10.1089/vbz.2012.0977
156. Socolovschi C, Matsumoto K, Marie JL, Davoust B, Raoult D, Parola P. Identification of rickettsiae, Uganda and Djibouti. Emerg Infect Dis (2007) 13(10):1508–10. doi: 10.3201/eid1310.070078
157. Abdullah H, El-Shanawany EE, Abdel-Shafy S, Abou-Zeina HAA, Abdel-Rahman EH. Molecular and immunological characterization of hyalomma dromedarii and hyalomma excavatum (Acari: Ixodidae) vectors of q fever in camels. Vet World (2018) 11(8):1109–19. doi: 10.14202/vetworld.2018.1109-1119
158. Al-Deeb MA, Muzaffar SB, Abu-Zeid YA, Enan MR, Karim S. First record of a spotted fever group rickettsia sp. and theileria annulata in hyalomma dromedarii (Acari: Ixodidae) ticks in the united Arab Emirates. Florida Entomologist (2015) 98(1):135–9. doi: 10.1653/024.098.0123
159. Ereqat S, Nasereddin A, Al-Jawabreh A, Azmi K, Harrus S, Mumcuoglu K, et al. Molecular detection and identification of spotted fever group rickettsiae in ticks collected from the West bank, Palestinian territories. PLoS Negl Trop Dis (2016) 10(1):e0004348. doi: 10.1371/journal.pntd.0004348
160. Kleinerman G, Baneth G, Mumcuoglu KY, van Straten M, Berlin D, Apanaskevich DA, et al. Molecular detection of rickettsia africae, rickettsia aeschlimannii, and rickettsia sibirica mongolitimonae in camels and hyalomma spp. ticks from Israel. Vector Borne Zoonotic Dis (2013) 13(12):851–6. doi: 10.1089/vbz.2013.1330
161. Dantas-Torres F. The brown dog tick, rhipicephalus sanguineus (Latreille, 1806) (Acari: Ixodidae): From taxonomy to control. Vet Parasitol (2008) 152(3-4):173–85. doi: 10.1016/j.vetpar.2007.12.030
162. Estrada-Peña A, Bouattour A, Camicas J, Walker A. Ticks of domestic animals in the Mediterranean region. Spain: University of Zaragoza (2004). p. 131.
164. Brumpt E. Longevité Du virus de la fièvre boutonneuse (Rickettsia conorii, n. sp.) chez la tique rhipicephalus sanguineus. CR Soc Biol (1932) 110:1197–9.
165. Ogo NI, de Mera IG, Galindo RC, Okubanjo OO, Inuwa HM, Agbede RI, et al. Molecular identification of tick-borne pathogens in Nigerian ticks. Vet Parasitol (2012) 187(3-4):572–7. doi: 10.1016/j.vetpar.2012.01.029
166. Gillespie JJ, Beier MS, Rahman MS, Ammerman NC, Shallom JM, Purkayastha A, et al. Plasmids and rickettsial evolution: Insight from rickettsia felis. PLoS One (2007) 2(3):e266. doi: 10.1371/journal.pone.0000266
167. Qiu Y, Simuunza M, Kajihara M, Chambaro H, Harima H, Eto Y, et al. Screening of tick-borne pathogens in argasid ticks in Zambia: Expansion of the geographic distribution of rickettsia lusitaniae and rickettsia hoogstraalii and detection of putative novel anaplasma species. Ticks Tick Borne Dis (2021) 12(4):101720. doi: 10.1016/j.ttbdis.2021.101720
168. Moonga LC, Hayashida K, Nakao R, Lisulo M, Kaneko C, Nakamura I, et al. Molecular detection of rickettsia felis in dogs, rodents and cat fleas in Zambia. Parasit Vectors (2019) 12(1):168. doi: 10.1186/s13071-019-3435-6
169. Duh D, Punda-Polic V, Avsic-Zupanc T, Bouyer D, Walker DH, Popov VL, et al. Rickettsia hoogstraalii sp. nov., isolated from hard- and soft-bodied ticks. Int J Syst Evol Microbiol (2010) 60(Pt 4):977–84. doi: 10.1099/ijs.0.011049-0
170. Duh D, Punda-Polic V, Trilar T, Petrovec M, Bradaric N, Avsic-Zupanc T. Molecular identification of rickettsia felis-like bacteria in haemaphysalis sulcata ticks collected from domestic animals in southern Croatia. Ann NY Acad Sci (2006) 1078:347–51. doi: 10.1196/annals.1374.068
171. Reeves WK, Mans BJ, Durden LA, Miller MM, Gratton EM, Laverty TM. Rickettsia hoogstraalii and a rickettsiella from the bat tick argas transgariepinus, in Namibia. J Parasitol (2020) 106(5):663–9. doi: 10.1645/20-46
172. Chitanga S, Chambaro HM, Moonga LC, Hayashida K, Yamagishi J, Muleya W, et al. Rickettsia lusitaniae in ornithodoros porcinus ticks, Zambia. Pathogens (2021) 10(10):1–10. doi: 10.3390/pathogens10101306
173. Pader V, Nikitorowicz Buniak J, Abdissa A, Adamu H, Tolosa T, Gashaw A, et al. Candidatus rickettsia hoogstraalii in Ethiopian argas persicus ticks. Ticks Tick Borne Dis (2012) 3(5-6):338–45. doi: 10.1016/j.ttbdis.2012.10.021
174. Trape JF, Diatta G, Arnathau C, Bitam I, Sarih M, Belghyti D, et al. The epidemiology and geographic distribution of relapsing fever borreliosis in West and north Africa, with a review of the ornithodoros erraticus complex (Acari: Ixodida). PLoS One (2013) 8(11):e78473. doi: 10.1371/journal.pone.0078473
175. Troyo A, Moreira-Soto RD, Calderon-Arguedas O, Mata-Somarribas C, Ortiz-Tello J, Barbieri AR, et al. Detection of rickettsiae in fleas and ticks from areas of Costa Rica with history of spotted fever group rickettsioses. Ticks Tick Borne Dis (2016) 7(6):1128–34. doi: 10.1016/j.ttbdis.2016.08.009
176. Cazorla C, Socolovschi C, Jensenius M, Parola P. Tick-borne diseases: Tick-borne spotted fever rickettsioses in Africa. Infect Dis Clin North Am (2008) 22(3):531–44, ix-x. doi: 10.1016/j.idc.2008.03.009
177. Abdelkadir K, Palomar AM, Portillo A, Oteo JA, Ait-Oudhia K, Khelef D. Presence of rickettsia aeschlimannii, 'Candidatus rickettsia barbariae' and coxiella burnetii in ticks from livestock in northwestern Algeria. Ticks Tick Borne Dis (2019) 10(4):924–8. doi: 10.1016/j.ttbdis.2019.04.018
178. Abdel-Shafy S, Allam NA, Mediannikov O, Parola P, Raoult D. Molecular detection of spotted fever group rickettsiae associated with ixodid ticks in Egypt. Vector Borne Zoonotic Dis (2012) 12(5):346–59. doi: 10.1089/vbz.2010.0241
179. Sadeddine R, Diarra AZ, Laroche M, Mediannikov O, Righi S, Benakhla A, et al. Molecular identification of protozoal and bacterial organisms in domestic animals and their infesting ticks from north-Eastern Algeria. Ticks Tick Borne Dis (2020) 11(2):101330. doi: 10.1016/j.ttbdis.2019.101330
180. Khaldi M, Socolovschi C, Benyettou M, Barech G, Biche M, Kernif T, et al. Rickettsiae in arthropods collected from the north African hedgehog (Atelerix algirus) and the desert hedgehog (Paraechinus aethiopicus) in Algeria. Comp Immunol Microbiol Infect Dis (2012) 35(2):117–22. doi: 10.1016/j.cimid.2011.11.007
181. Bouchaib H, Eldin C, Laroche M, Raoult D, Parola P. Tick- and flea-borne rickettsioses in tizi-ouzou, Algeria: Implications for travel medicine. Travel Med Infect Dis (2018) 26:51–7. doi: 10.1016/j.tmaid.2018.11.005
182. Nakao R, Qiu Y, Igarashi M, Magona JW, Zhou L, Ito K, et al. High prevalence of spotted fever group rickettsiae in amblyomma variegatum from Uganda and their identification using sizes of intergenic spacers. Ticks Tick Borne Dis (2013) 4(6):506–12. doi: 10.1016/j.ttbdis.2013.07.001
183. Keller C, Kruger A, Schwarz NG, Rakotozandrindrainy R, Rakotondrainiarivelo JP, Razafindrabe T, et al. High detection rate of rickettsia africae in amblyomma variegatum but low prevalence of anti-rickettsial antibodies in healthy pregnant women in Madagascar. Ticks Tick Borne Dis (2016) 7(1):60–5. doi: 10.1016/j.ttbdis.2015.08.005
184. Mtshali K, Khumalo Z, Nakao R, Grab DJ, Sugimoto C, Thekisoe O. Molecular detection of zoonotic tick-borne pathogens from ticks collected from ruminants in four south African provinces. J Vet Med Sci (2016) 77(12):1573–9. doi: 10.1292/jvms.15-0170
185. Chiuya T, Masiga DK, Falzon LC, Bastos ADS, Fevre EM, Villinger J. Tick-borne pathogens, including Crimean-Congo haemorrhagic fever virus, at livestock markets and slaughterhouses in Western Kenya. Transbound Emerg Dis (2021) 68(4):2429–45. doi: 10.1111/tbed.13911
186. Maina AN, Jiang J, Omulo SA, Cutler SJ, Ade F, Ogola E, et al. High prevalence of rickettsia africae variants in amblyomma variegatum ticks from domestic mammals in rural Western Kenya: Implications for human health. Vector Borne Zoonotic Dis (2014) 14(10):693–702. doi: 10.1089/vbz.2014.1578
187. Macaluso KR, Davis J, Alam U, Korman A, Rutherford JS, Rosenberg R, et al. Spotted fever group rickettsiae in ticks from the Masai Mara region of Kenya. Am J Trop Med Hyg (2003) 68(5):551–3. doi: 10.4269/ajtmh.2003.68.551
188. Dupont HT, Brouqui P, Faugere B, Raoult D. Prevalence of antibodies to coxiella burnetti, rickettsia conorii, and rickettsia typhi in seven African countries. Clin Infect Dis (1995) 21(5):1126–33. doi: 10.1093/clinids/21.5.1126
189. Levin ML, Zemtsova GE, Montgomery M, Killmaster LF. Effects of homologous and heterologous immunization on the reservoir competence of domestic dogs for rickettsia conorii (Israelensis). Ticks Tick Borne Dis (2014) 5(1):33–40. doi: 10.1016/j.ttbdis.2013.07.010
190. Boucheikhchoukh M, Laroche M, Aouadi A, Dib L, Benakhla A, Raoult D, et al. Maldi-tof Ms identification of ticks of domestic and wild animals in Algeria and molecular detection of associated microorganisms. Comp Immunol Microbiol Infect Dis (2018) 57:39–49. doi: 10.1016/j.cimid.2018.05.002
191. Ehlers J, Kruger A, Rakotondranary SJ, Ratovonamana RY, Poppert S, Ganzhorn JU, et al. Molecular detection of rickettsia spp., borrelia spp., bartonella spp. and yersinia pestis in ectoparasites of endemic and domestic animals in southwest Madagascar. Acta Trop (2020) 205:105339. doi: 10.1016/j.actatropica.2020.105339
192. Nnabuife HE, Matur B, Ogo NI, Goselle O, Dakul A, Egbuji A, et al. Seroprevalence of spotted fever group rickettsiae in extensively managed sheep and goats in Nigeria, West Africa. Trop Anim Health Prod (2021) 53(4):425. doi: 10.1007/s11250-021-02868-z
193. Bessas A, Leulmi H, Bitam I, Zaidi S, Ait-Oudhia K, Raoult D, et al. Molecular evidence of vector-borne pathogens in dogs and cats and their ectoparasites in Algiers, Algeria. Comp Immunol Microbiol Infect Dis (2016) 45:23–8. doi: 10.1016/j.cimid.2016.01.002
194. Sfar N, M'Ghirbi Y, Letaief A, Parola P, Bouattour A, Raoult D. First report of rickettsia monacensis and rickettsia helvetica from Tunisia. Ann Trop Med Parasitol (2008) 102(6):561–4. doi: 10.1179/136485908X311795
195. Newton PN, Guerin PJ. Febrile illness mapping-much of the world without data and without evidence-based treatments. BMC Med (2020) 18(1):287. doi: 10.1186/s12916-020-01747-y
196. Walker DH, Cain BG, Olmstead PM. Laboratory diagnosis of rocky mountain spotted fever by immunofluorescent demonstration of rickettsia in cutaneous lesions. Am J Clin Pathol (1978) 69(6):619–23. doi: 10.1093/ajcp/69.6.619
197. Khrouf F, M'Ghirbi Y, Znazen A, Ben Jemaa M, Hammami A, Bouattour A. Detection of rickettsia in rhipicephalus sanguineus ticks and ctenocephalides felis fleas from southeastern Tunisia by reverse line blot assay. J Clin Microbiol (2014) 52(1):268–74. doi: 10.1128/JCM.01925-13
198. Mwamuye MM, Kariuki E, Omondi D, Kabii J, Odongo D, Masiga D, et al. Novel rickettsia and emergent tick-borne pathogens: A molecular survey of ticks and tick-borne pathogens in shimba hills national reserve, Kenya. Ticks Tick Borne Dis (2017) 8(2):208–18. doi: 10.1016/j.ttbdis.2016.09.002
199. Mediannikov O, Parola P, Raoult D. Other tick-borne rickettsioses. Rickettsial Diseases. CRC Press (2007) . p:151–74. doi: 10.3109/9781420019971.011
200. Palomar AM, Molina I, Bocanegra C, Portillo A, Salvador F, Moreno M, et al. Old zoonotic agents and novel variants of tick-borne microorganisms from benguela (Angola), July 2017. Parasit Vectors (2022) 15(1):140. doi: 10.1186/s13071-022-05238-2
201. Qiu Y, Simuunza M, Kajihara M, Ndebe J, Saasa N, Kapila P, et al. Detection of tick-borne bacterial and protozoan pathogens in ticks from the Zambia-Angola border. Pathogens (2022) 11(5):1–12. doi: 10.3390/pathogens11050566
202. Chitanga S, Chibesa K, Sichibalo K, Mubemba B, Nalubamba KS, Muleya W, et al. Molecular detection and characterization of rickettsia species in ixodid ticks collected from cattle in southern Zambia. Front Vet Sci (2021) 8:684487. doi: 10.3389/fvets.2021.684487
203. Mahlobo-Shwabede SIC, Zishiri OT, Thekisoe OMM, Makalo MJR. Molecular detection of coxiella burnetii, rickettsia africae and anaplasma species in ticks from domestic animals in Lesotho. Pathogens (2021) 10(9):1–18. doi: 10.3390/pathogens10091186
204. Mazhetese E, Lukanji Z, Byaruhanga C, Neves L, Morar-Leather D. Rickettsia africae infection rates and transovarial transmission in amblyomma hebraeum ticks in mnisi, bushbuckridge, south Africa. Exp Appl Acarol (2022) 86(3):407–18. doi: 10.1007/s10493-022-00696-w
205. Magaia V, Taviani E, Cangi N, Neves L. Molecular detection of rickettsia africae in amblyomma ticks collected in cattle from southern and central Mozambique. J Infect Dev Ctries (2020) 14(6):614–22. doi: 10.3855/jidc.11625
206. Ehlers J, Ganzhorn JU, Silaghi C, Kruger A, Pothmann D, Ratovonamana RY, et al. Tick (Amblyomma chabaudi) infestation of endemic tortoises in southwest Madagascar and investigation of tick-borne pathogens. Ticks Tick Borne Dis (2016) 7(2):378–83. doi: 10.1016/j.ttbdis.2015.12.011
207. Fournier PE, El Karkouri K, Leroy Q, Robert C, Giumelli B, Renesto P, et al. Analysis of the rickettsia africae genome reveals that virulence acquisition in rickettsia species may be explained by genome reduction. BMC Genomics (2009) 10:166. doi: 10.1186/1471-2164-10-166
208. Lauzi S, Maia JP, Epis S, Marcos R, Pereira C, Luzzago C, et al. Molecular detection of anaplasma platys, ehrlichia canis, hepatozoon canis and rickettsia monacensis in dogs from maio island of cape Verde archipelago. Ticks Tick Borne Dis (2016) 7(5):964–9. doi: 10.1016/j.ttbdis.2016.05.001
209. Benredjem W, Leulmi H, Bitam I, Raoult D, Parola P. Borrelia garinii and rickettsia monacensis in ixodes ricinus ticks, Algeria. Emerg Infect Dis (2014) 20(10):1776–7. doi: 10.3201/eid2010.140265
210. Jado I, Oteo JA, Aldamiz M, Gil H, Escudero R, Ibarra V, et al. Rickettsia monacensis and human disease, Spain. Emerg Infect Dis (2007) 13(9):1405–7. doi: 10.3201/eid1309.060186
211. Madeddu G, Mancini F, Caddeo A, Ciervo A, Babudieri S, Maida I, et al. Rickettsia monacensis as cause of Mediterranean spotted fever-like illness, Italy. Emerg Infect Dis (2012) 18(4):702–4. doi: 10.3201/eid1804.111583
212. Plantard O, Bouju-Albert A, Malard MA, Hermouet A, Capron G, Verheyden H. Detection of wolbachia in the tick ixodes ricinus is due to the presence of the hymenoptera endoparasitoid ixodiphagus hookeri. PLoS One (2012) 7(1):e30692. doi: 10.1371/journal.pone.0030692
213. Bohacsova M, Mediannikov O, Kazimirova M, Raoult D, Sekeyova Z. Arsenophonus nasoniae and rickettsiae infection of ixodes ricinus due to parasitic wasp ixodiphagus hookeri. PLoS One (2016) 11(2):e0149950. doi: 10.1371/journal.pone.0149950
214. Williamson BN, Schwan TG. Conspecific hyperparasitism: An alternative route for borrelia hermsii transmission by the tick ornithodoros hermsi. Ticks Tick Borne Dis (2018) 9(2):334–9. doi: 10.1016/j.ttbdis.2017.11.009
215. Davis A. Bibliography of the ixodiphagini (Hymenoptera, chalcidoidea, encyrtidae), parasites of ticks (Acari, ixodidae), with notes on their biology. Tijdschrift voor entomologie (1986) 129(6):181–90.
216. Gaye M, Amanzougaghene N, Laidoudi Y, Niang EHA, Sekeyova Z, Laroche M, et al. Hymenopteran parasitoids of hard ticks in Western Africa and the Russian far East. Microorganisms (2020) 8(12):1–13. doi: 10.3390/microorganisms8121992
217. Franck P, Maalouly-Matar M, Olivares J. Molecular tools for the detection and the identification of hymenoptera parasitoids in tortricid fruit pests. Int J Mol Sci (2017) 18(10):1–16. doi: 10.3390/ijms18102031
218. Kelly PJ, Mason PR, Manning T, Slater S. Role of cattle in the epidemiology of tick-bite fever in Zimbabwe. J Clin Microbiol (1991) 29(2):256–9. doi: 10.1128/jcm.29.2.256-259.1991
219. Kelly P, Lucas H, Beati L, Yowell C, Mahan S, Dame J. Rickettsia africae in amblyomma variegatum and domestic ruminants on eight Caribbean islands. J Parasitol (2010) 96(6):1086–8. doi: 10.1645/GE-2552.1
220. Parola P, Vestris G, Martinez D, Brochier B, Roux V, Raoult D. Tick-borne rickettiosis in Guadeloupe, the French West indies: Isolation of rickettsia africae from amblyomma variegatum ticks and serosurvey in humans, cattle, and goats. Am J Trop Med Hyg (1999) 60(6):888–93. doi: 10.4269/ajtmh.1999.60.888
221. Harrus S, Lior Y, Ephros M, Grisaru-Soen G, Keysary A, Strenger C, et al. Rickettsia conorii in humans and dogs: A seroepidemiologic survey of two rural villages in Israel. Am J Trop Med Hyg (2007) 77(1):133–5. doi: 10.4269/ajtmh.2007.77.133
222. McDade JE, Newhouse VF. Natural history of rickettsia rickettsii. Annu Rev Microbiol (1986) 40:287–309. doi: 10.1146/annurev.mi.40.100186.001443
223. Scinachi CA, Takeda G, Mucci LF, Pinter A. Association of the occurrence of Brazilian spotted fever and Atlantic rain forest fragmentation in the sao paulo metropolitan region, Brazil. Acta Trop (2017) 166:225–33. doi: 10.1016/j.actatropica.2016.11.025
224. Savani E, Costa FB, Silva EA, Couto ACF, Gutjahr M, Alves J, et al. Fatal Brazilian spotted fever associated with dogs and amblyomma aureolatum ticks, Brazil, 2013. Emerg Infect Dis (2019) 25(12):2322–3. doi: 10.3201/eid2512.191146
225. Binder LC, Ramirez-Hernandez A, Serpa MCA, Moraes-Filho J, Pinter A, Scinachi CA, et al. Domestic dogs as amplifying hosts of rickettsia rickettsii for amblyomma aureolatum ticks. Ticks Tick Borne Dis (2021) 12(6):101824. doi: 10.1016/j.ttbdis.2021.101824
226. Kamani J, Baneth G, Gutierrez R, Nachum-Biala Y, Mumcuoglu KY, Harrus S. Coxiella burnetii and rickettsia conorii: Two zoonotic pathogens in peridomestic rodents and their ectoparasites in Nigeria. Ticks Tick Borne Dis (2018) 9(1):86–92. doi: 10.1016/j.ttbdis.2017.10.004
227. Diarra AZ, Kone AK, Doumbo Niare S, Laroche M, Diatta G, Atteynine SA, et al. Molecular detection of microorganisms associated with small mammals and their ectoparasites in Mali. Am J Trop Med Hyg (2020) 103(6):2542–51. doi: 10.4269/ajtmh.19-0727
228. Halajian A, Palomar AM, Portillo A, Heyne H, Luus-Powell WJ, Oteo JA. Investigation of rickettsia, coxiella burnetii and bartonella in ticks from animals in south Africa. Ticks Tick Borne Dis (2016) 7(2):361–6. doi: 10.1016/j.ttbdis.2015.12.008
229. Ioannou I, Chochlakis D, Kasinis N, Anayiotos P, Lyssandrou A, Papadopoulos B, et al. Carriage of rickettsia spp., coxiella burnetii and anaplasma spp. by endemic and migratory wild birds and their ectoparasites in Cyprus. Clin Microbiol Infect (2009) 15 Suppl 2:158–60. doi: 10.1111/j.1469-0691.2008.02207.x
230. Mukherjee N, Beati L, Sellers M, Burton L, Adamson S, Robbins RG, et al. Importation of exotic ticks and tick-borne spotted fever group rickettsiae into the united states by migrating songbirds. Ticks Tick Borne Dis (2014) 5(2):127–34. doi: 10.1016/j.ttbdis.2013.09.009
231. Ebani VV, Mancianti F. Potential role of avian populations in the epidemiology of rickettsia spp. and babesia spp. Vet Sci (2021) 8(12):1–14. doi: 10.3390/vetsci8120334
232. Gonzalez FA, Martins J, Coelho M, Amaro M, Milhano N, Santos AS, et al. African Tick bite fever in a Portuguese traveler returning from south Africa. Infect Dis Clin Pract (2012) 20(2):150–1. doi: 10.1097/IPC.0b013e31821ed104
233. Kelly PJ, Mason PR. Transmission of a spotted fever group rickettsia by amblyomma hebraeum (Acari: Ixodidae). J Med Entomol (1991) 28(5):598–600. doi: 10.1093/jmedent/28.5.598
234. Silva-Ramos CR, Faccini-Martinez AA. Clinical, epidemiological, and laboratory features of rickettsia africae infection, African tick-bite fever: A systematic review. Infez Med (2021) 29(3):366–77. doi: 10.53854/liim-2903-7
235. Albizuri Prado F, Sanchez A, Feito M, Mayor A, Rodriguez A, de Lucas R. Fever and multiple eschars after an African safari: Report of three cases. Pediatr Dermatol (2017) 34(4):e179–81. doi: 10.1111/pde.13163
236. Strand A, Paddock CD, Rinehart AR, Condit ME, Marus JR, Gillani S, et al. African Tick bite fever treated successfully with rifampin in a patient with doxycycline intolerance. Clin Infect Dis (2017) 65(9):1582–4. doi: 10.1093/cid/cix363
237. Nilsson K, Wallmenius K, Rundlof-Nygren P, Stromdahl S, Pahlson C. African Tick bite fever in returning Swedish travellers. report of two cases and aspects of diagnostics. Infect Ecol Epidemiol (2017) 7(1):1343081. doi: 10.1080/20008686.2017.1343081
238. Bogovic P, Lotric-Furlan S, Korva M, Avsic-Zupanc T. African Tick-bite fever in traveler returning to Slovenia from Uganda. Emerg Infect Dis (2016) 22(10):1848–9. doi: 10.3201/eid2210.160650
239. Hauser N, Arzomand Z, Fournier J, Breen C, Jamali L, Cossman J, et al. A case of African tick-bite fever in a returning traveler. IDCases (2016) 5:78–9. doi: 10.1016/j.idcr.2016.07.004
240. Harrison N, Burgmann H, Forstner C, Ramharter M, Szell M, Schotta AM, et al. Molecular diagnosis of African tick bite fever using eschar swabs in a traveller returning from Tanzania. Wien Klin Wochenschr (2016) 128(15-16):602–5. doi: 10.1007/s00508-016-1047-0
241. Binder WD, Gupta R. African Tick-bite fever in a returning traveler. J Emerg Med (2015) 48(5):562–5. doi: 10.1016/j.jemermed.2014.12.044
242. Bohaty BR, Hebert AA. Images in clinical medicine: African tick-bite fever after a game-hunting expedition. N Engl J Med (2015) 372(10):e14. doi: 10.1056/NEJMicm1312910
243. Angerami RN, Krawczak FS, Nieri-Bastos FA, Santos F, Medorima C, Resende MR, et al. First report of African tick-bite fever in a south American traveler. SAGE Open Med Case Rep (2018) 6:2050313X18775301. doi: 10.1177/2050313X18775301
244. Armitano RI, Borras PJ, Govedic F, Prieto MA, Guillemi E. African Tick bite fever: First imported cases diagnosed by pcr in Argentina. Travel Med Infect Dis (2021) 40:101959. doi: 10.1016/j.tmaid.2020.101959
245. Fujisawa T, Kadosaka T, Fujita H, Ando S, Takano A, Ogasawara Y, et al. Rickettsia africae infection in a Japanese traveller with many tick bites. Acta Derm Venereol (2012) 92(4):443–4. doi: 10.2340/00015555-1313
246. Lee W, Seong H, Kim JH, Choi H, Kim JH, Ahn JY, et al. A case of African tick-bite fever in a returning traveler from southern Africa. Infect Chemother (2022) 54(1):202–7. doi: 10.3947/ic.2019.0073
247. Schwartz RA, Kapila R, McElligott SC, Atkin SH, Lambert WC. Cutaneous leishmaniasis and rickettsial African tick-bite fever: A combination of exotic traveler's diseases in the same patient. Int J Dermatol (2012) 51(8):960–3. doi: 10.1111/j.1365-4632.2011.05362.x
248. Chmielewski T, Szymanek A, Maczka I, Fiecek B, Simon K, Tylewska-Wierzbanowska S. Case report of African tick-bite fever from Poland. Postepy Dermatol Alergol (2013) 30(6):396–8. doi: 10.5114/pdia.2013.39438
249. Antal AS, Flaig MJ, Schneck C, Thoma B, Herzinger T. Souvenir from south Africa. Infection (2013) 41(2):597–8. doi: 10.1007/s15010-013-0425-z
250. Yankura J, Ioffreda MD. Jaad grand rounds quiz. necrotic plaques in a traveler. J Am Acad Dermatol (2014) 70(5):959–61. doi: 10.1016/j.jaad.2012.10.028
251. Franon E, Manckoundia P. Memory of an exotic holiday. Eur J Intern Med (2015) 26(10):e57–8. doi: 10.1016/j.ejim.2015.07.007
252. Tomasiewicz K, Krzowska-Firych J, Bielec D, Socolovschi C, Raoult D. First case of imported African tick-bite fever in Poland - case report. Ann Agric Environ Med (2015) 22(3):412–3. doi: 10.5604/12321966.1167703
253. Liao E, Carr D. A tale of black eschar in a returning traveller. J Emerg Med (2017) 53(6):904–6. doi: 10.1016/j.jemermed.2017.08.002
254. Menzer C, Fink C, Enk A, Haenssle HA. African Tick-bite fever - a tantalizing souvenir from south Africa. J Dtsch Dermatol Ges (2017) 15(10):1027–8. doi: 10.1111/ddg.13322
255. van Geffen M, van Niekerk J, Posthouwer D. A patient with fever and skin lesions after vacation in south Africa. In: A skin lesion after a visit to south africa; what is your diagnosis? (2018). p. 134. The Netherlands Journal of Medicine, Van Zuiden Communications B.V. PO Box 2122 2400 CC Alphen aan den Rijn The Netherlands
256. Ando N, Kutsuna S, Takaya S, Katanami Y, Ohmagari N. Imported African tick bite fever in Japan: A literature review and report of three cases. Intern Med (2022) 61(7):1093–8. doi: 10.2169/internalmedicine.7109-21
257. Thorner AR, Walker DH, Petri Jr.WA. Rocky mountain spotted fever. Clin Infect Dis (1998) 27(6):1353–9. doi: 10.1086/515037
258. McClain MT, Sexton DJ. Surveillance for spotted fever group rickettsial infections: Problems, pitfalls, and potential solutions. J Infect Dis (2020) 221(8):1238–40. doi: 10.1093/infdis/jiz317
259. Lange JV, Walker DH, Wester TB. Documented rocky mountain spotted fever in wintertime. JAMA (1982) 247(17):2403–4. doi: 10.1001/jama.1982.03320420053034
260. Salgo MP, Telzak EE, Currie B, Perlman DC, Litman N, Levi M, et al. A focus of rocky mountain spotted fever within new York city. N Engl J Med (1988) 318(21):1345–8. doi: 10.1056/NEJM198805263182101
261. Binder AM, Nichols Heitman K, Drexler NA. Diagnostic methods used to classify confirmed and probable cases of spotted fever rickettsioses - united states, 2010-2015. MMWR Morb Mortal Wkly Rep (2019) 68(10):243–6. doi: 10.15585/mmwr.mm6810a3
262. Carpenter CF, Gandhi TK, Kong LK, Corey GR, Chen SM, Walker DH, et al. The incidence of ehrlichial and rickettsial infection in patients with unexplained fever and recent history of tick bite in central north Carolina. J Infect Dis (1999) 180(3):900–3. doi: 10.1086/314954
263. Paddock CD, Greer PW, Ferebee TL, Singleton J Jr., McKechnie DB, Treadwell TA, et al. Hidden mortality attributable to rocky mountain spotted fever: Immunohistochemical detection of fatal, serologically unconfirmed disease. J Infect Dis (1999) 179(6):1469–76. doi: 10.1086/314776
264. Sexton DJ, Corey GR. Rocky mountain "Spotless" and "Almost spotless" fever: A wolf in sheep's clothing. Clin Infect Dis (1992) 15(3):439–48. doi: 10.1093/clind/15.3.439
265. Eisawi NM, Hassan DA, Hussien MO, Musa AB, El Hussein ARM. Seroprevalence of spotted fever group (Sfg) rickettsiae infection in domestic ruminants in Khartoum state, Sudan. Vet Med Sci (2017) 3(2):91–8. doi: 10.1002/vms3.59
Keywords: spotted fever, rickettsia spp., ticks, Africa, epidemiology
Citation: Onyiche TE, Labruna MB and Saito TB (2022) Unraveling the epidemiological relationship between ticks and rickettsial infection in Africa. Front. Trop. Dis 3:952024. doi: 10.3389/fitd.2022.952024
Received: 24 May 2022; Accepted: 22 July 2022;
Published: 06 September 2022.
Edited by:
Mathilde Gendrin, Institut Pasteur de la Guyane, French GuianaReviewed by:
Jessica Rauch, Bernhard Nocht Institute for Tropical Medicine (BNITM), GermanyLaurence Vial, Institut National de la Recherche Agronomique (INRA), France
Copyright © 2022 Onyiche, Labruna and Saito. This is an open-access article distributed under the terms of the Creative Commons Attribution License (CC BY). The use, distribution or reproduction in other forums is permitted, provided the original author(s) and the copyright owner(s) are credited and that the original publication in this journal is cited, in accordance with accepted academic practice. No use, distribution or reproduction is permitted which does not comply with these terms.
*Correspondence: Tais B. Saito, dGFpcy5iZXJlbGxpc2FpdG9AbmloLmdvdg==