- 1Department of Clinical Research, Faculty of Infectious and Tropical Diseases, London School of Hygiene and Tropical Medicine, London, United Kingdom
- 2Department of Infection Biology, Faculty of Infectious and Tropical Diseases, London School of Hygiene and Tropical Medicine, London, United Kingdom
- 3Scottish Oceans Institute, School of Biology, University of St Andrews, Scotland, United Kingdom
- 4Wolfson Wellcome Biomedical Laboratories, Department of Life Sciences, Natural History Museum, London, United Kingdom
- 5Department of Tropical Disease Biology, Liverpool School of Tropical Medicine, Liverpool, United Kingdom
- 6Medical Research Council/ Uganda Virus Research Institute (MRC/UVRI) and London School of Hygiene and Tropical Medicine (LSHTM) Uganda Research Unit, Entebbe, Uganda
Schistosomiasis is a neglected tropical disease (NTD) caused by parasitic trematodes belonging to the Schistosoma genus. The mainstay of schistosomiasis control is the delivery of a single dose of praziquantel (PZQ) through mass drug administration (MDA) programs. These programs have been successful in reducing the prevalence and intensity of infections. Due to the success of MDA programs, the disease has recently been targeted for elimination as a public health problem in some endemic settings. The new World Health Organization (WHO) treatment guidelines aim to provide equitable access to PZQ for individuals above two years old in targeted areas. The scale up of MDA programs may heighten the drug selection pressures on Schistosoma parasites, which could lead to the emergence of PZQ resistant schistosomes. The reliance on a single drug to treat a disease of this magnitude is worrying should drug resistance develop. Therefore, there is a need to detect and track resistant schistosomes to counteract the threat of drug resistance to the WHO 2030 NTD roadmap targets. Until recently, drug resistance studies have been hindered by the lack of molecular markers associated with PZQ resistance. This review discusses recent significant advances in understanding the molecular basis of PZQ action in S. mansoni and proposes additional genetic determinants associated with PZQ resistance. PZQ resistance will also be analyzed in the context of alternative factors that may decrease efficacy within endemic field settings, and the most recent treatment guidelines recommended by the WHO.
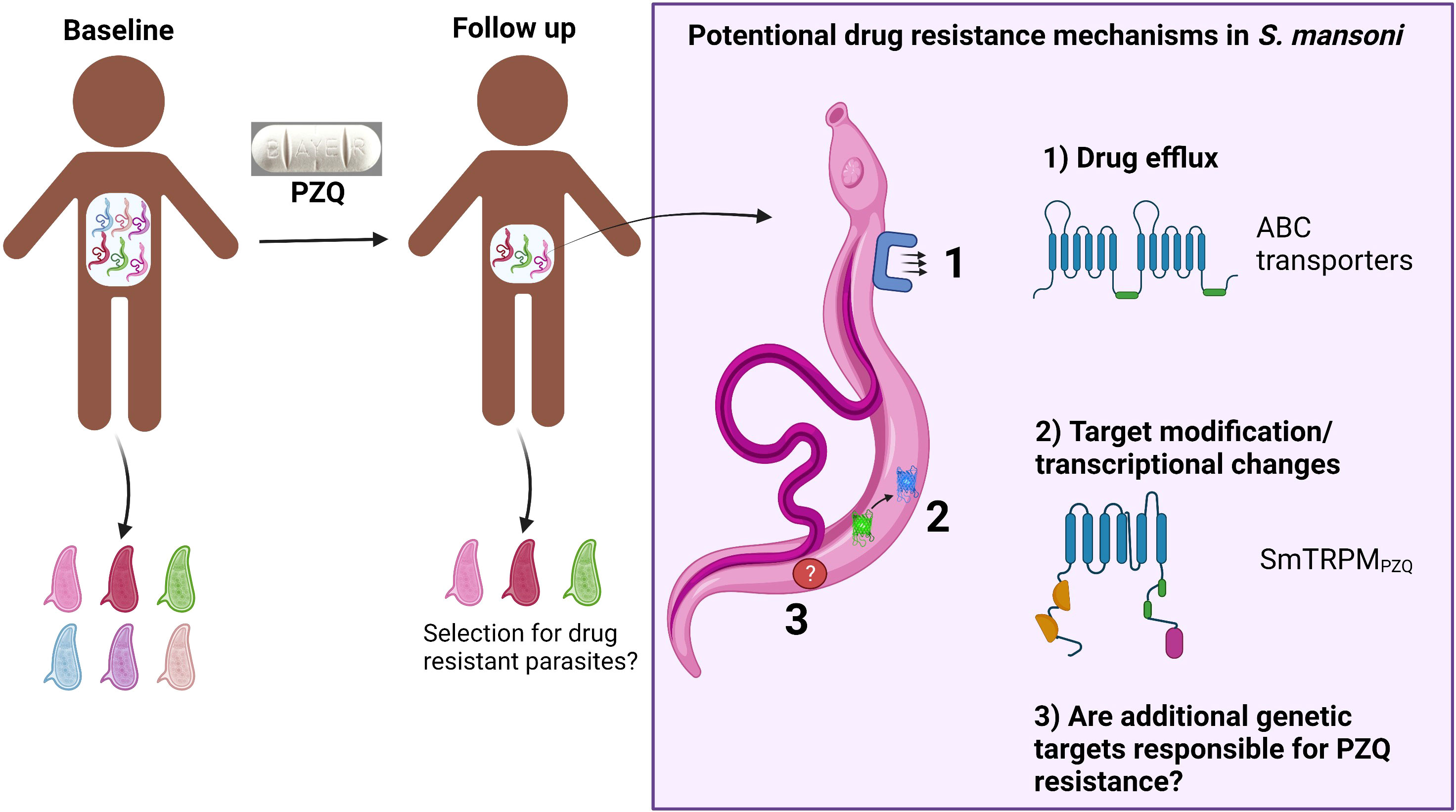
Graphical Abstract Potential drug resistance mechanisms in S. mansoni involve either drug efflux via ABC transporters or target modifications via SNPs in the PZQ binding site in SmTRPMPZQ or via transcriptional changes. Created with BioRender.com.
1 Introduction
Schistosomiasis is a disabling NTD caused by an infection with dioecious Schistosoma flukes, affecting 240 million people; circa 95% of infections occur in sub-Saharan Africa; S. mansoni and S. haematobium are responsible for intestinal and urogenital schistosomiasis, respectively (1, 2). Schistosoma has a two-host life cycle: miracidia infect the intermediate freshwater snail host to produce infective cercariae, which penetrate the skin of the definitive mammalian host. Following infection of the mammalian host, mature female worms produce eggs that become trapped in host tissue and induce inflammation, granuloma and subsequent fibrosis due to the immunopathological host response resulting in debilitating downstream morbidities (3). Non-trapped eggs are passed in the faeces or urine (Figure 1).
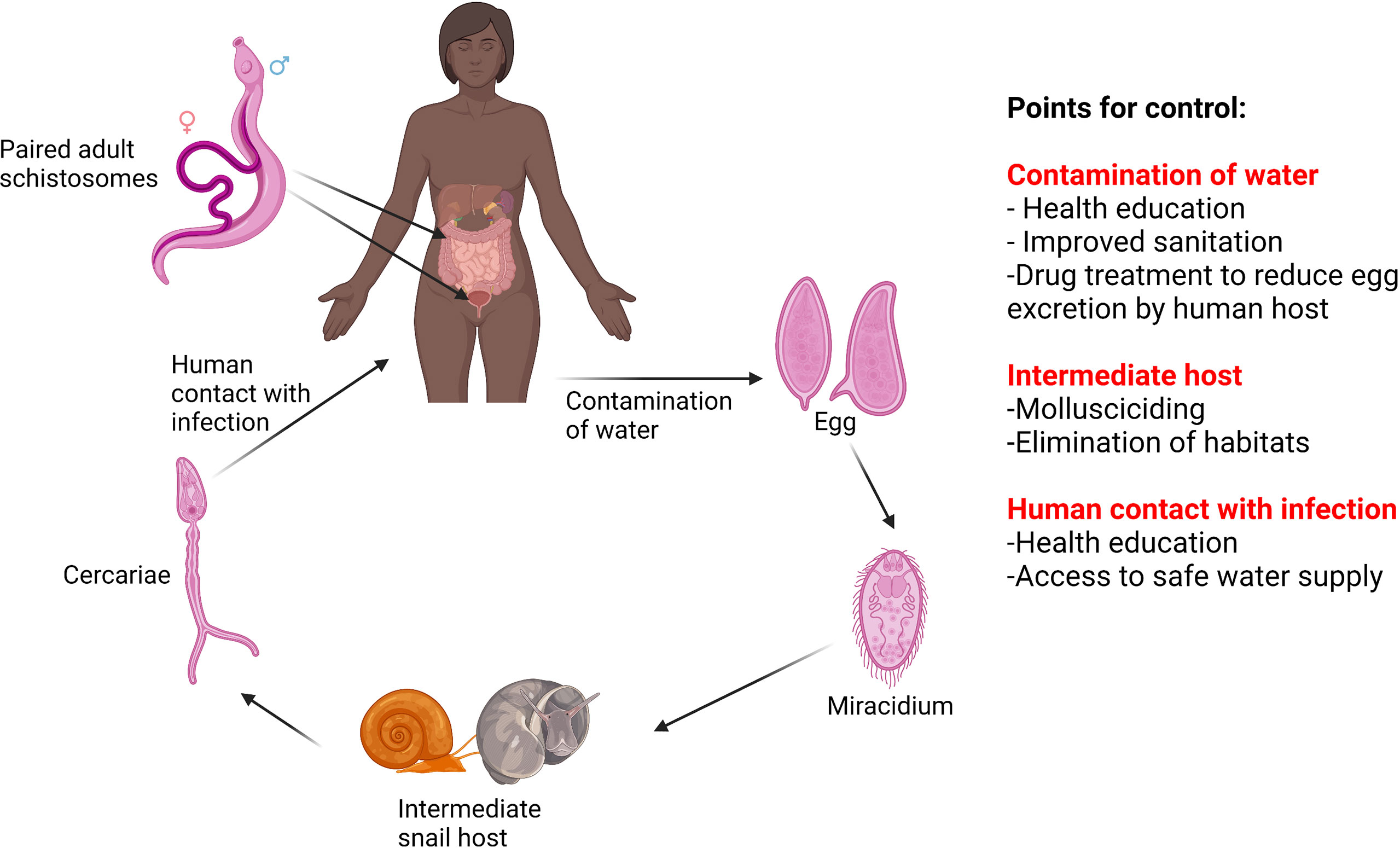
Figure 1 The lifecycle of S. mansoni and S. haematobium and points for control. Created with BioRender.com.
Schistosomiasis has an age-dependent intensity of infection and focal epidemiology, influenced by human water-contact patterns (4). Infants (<1 year) and preschool aged children (PSAC) (<5 years) can commonly harbour a schistosomiasis infection (5, 6).The prevalence of infection peaks during adolescence (10 to 15 years of age) and declines during adulthood (7, 8), although high prevalence of infection can remain in environmentally exposed subpopulations of adults (3, 4, 8).
The control and elimination of schistosomiasis requires interruption of a complex transmission pathway (4). There are several points of the lifecycle that can be targeted to reduce transmission; control measures to interrupt transmission include chemotherapy, snail control, health education, improved access to clean water, sanitation and hygiene (WASH) (4) (Figure 1).
The cornerstone of control programs is the administration of the drug PZQ, a broad-spectrum antihelmintic developed in the 1970s (9). The World Health Assembly (WHA) in 2001 endorsed preventive chemotherapy (PC) by PZQ as the main strategy of schistosomiasis control (10). PZQ is efficacious against all species of Schistosoma infecting humans and is given as a single oral dose (40 mg/kg recommended by the WHO) with minimal side effects, related to intensity of infection (11). MDA of PZQ began in sub-Saharan Africa from 2003; has been effective in reducing prevalence and intensity of infections (12, 13). The focus has now shifted to the elimination of schistosomiasis as a public health problem by 2030 (14). PZQ should be distributed for the first time to all age groups from two years of age. In recent years, the access to PZQ has been improved primarily through the pledge from Merck-KGaA to donate 250 million PZQ tablets annually to school-age children (SAC) indefinitely (14) aiding in reducing morbidity through the reduction of heavy infections. Despite annual MDA, hotspots of unabated transmission and associated morbidity persist (15–19).
The scale-up of MDA heightens drug selection pressures on the Schistosoma populations and could lead to the selection of PZQ resistant phenotypes, as has emerged in other parasitic infections including malaria (20) and in veterinary helminths (21–23). Despite the lack of consensus as to the molecular and genetic basis of PZQ resistance in S. mansoni, several resistance markers have been proposed (24–27). Additionally, PZQ resistance has been shown to be able to be induced in laboratory strains of S. mansoni, maintained in laboratory rodents (28–30), and several field reports suggest reduced efficacy of PZQ in specific areas of endemicity (31–35) due to inadequate cure rates (CR), although there are many factors that may influence the PZQ efficacy in specific individuals i.e. inadequate dosing, high level of juvenile worms and high worm burdens. Suggestions of widespread PZQ resistance in the field remain controversial as these reports are likely explained by factors other than drug resistant schistosomes, for example rapid re-infection (36–39). In the absence of novel anti-schistosomal drugs in the development pipeline, there is a reliance on a single drug to treat a disease of this importance and magnitude, which is worrying should drug resistance develop (40). Thus, there is an unmet need to detect and track resistant Schistosoma parasites to elucidate the threat of drug resistance to control programs.
This review draws together the recent literature on the molecular basis of PZQ action in S. mansoni and highlights advances in understanding the molecular basis and the proposed genetic determinants associated with PZQ resistance. PZQ resistance is also analyzed in the context of confounding factors within endemic field settings, and the most recent treatment guidelines recommended by the WHO.
2 Control of schistosomiasis: the new WHO guideline on control and elimination of human schistosomiasis
Schistosomiasis is a multifaceted disease with a complicated lifecycle, which requires a comprehensive control strategy. Over the past two decades, control efforts have led to a 58.3% decrease in the global burden of schistosomiasis (41). The new NTD roadmap (14) and guidelines on the control and elimination of schistosomiasis (42) set out to accelerate the progress made and are important milestones in the control of this disease. The new guidelines detail an integrated control strategy involving six evidence-based recommendations for endemic countries to facilitate the elimination of morbidity and interruption of transmission (42). The recommendations detail combining large scale PC programs, WASH, education, snail control and environmental modification to eliminate schistosomiasis as a public health problem (defined as <1% proportion of heavy intensity schistosomiasis infections) and to achieve interruption of transmission in selected countries (14, 42). Large-scale PC programs remain at the forefront of the control strategy. The main focus has been treatment of SAC, which neglected at risk populations, PSAC and adult populations including pregnant and lactating women. These programs will aim to provide equitable access to annual PZQ treatment to all age groups over the age of two years. The prevalence threshold for different interventions has been simplified and reduced to reflect the decline in the global burden of schistosomiasis (42). In endemic communities with a schistosomiasis prevalence > 10%, annual PC with PZQ is recommended. Whereas in communities with an infection prevalence < 10% a test and treat approach is recommended. In high prevalence settings and ‘persistent hotspots’ biannual PZQ PC is recommended to reduce the prevalence and intensity of infection, and specifically to prevent high intensity infections and the associated morbidity. The expanded eligibility for PZQ PC programs requires a larger global supply of PZQ than that currently donated (around 300 million tablets are donated annually). Providing a targeted treatment approach to communities through precision mapping, and more accurate diagnostics, could allow for more effective use of donated PZQ (42). The expanded PC programs aim to accelerate the path to schistosomiasis elimination, however; they also pose an increased risk of the selection of PZQ drug resistance within populations. Increased surveillance to monitor changes in drug efficacy via treatment monitoring and evaluation surveys (post treatment follow up surveys) have been recommended to ensure early detection of the emergence of PZQ resistance (42).
3 Mode of action of PZQ
Since the introduction of PZQ, the molecular mechanisms underpinning its antihelmintic activity have remained elusive despite research efforts [summarized in (43)]. PZQ sensitivity in schistosomes depends on several factors including the schistosome lifecycle stage, the state of adult pairing and the sex of schistosomes (43). Commercial preparations of PZQ consist of a racemic mixture of two isomers in a 1:1 ratio: (R)-PZQ and (S)-PZQ (Figure 2) (44). The (R)-PZQ isomer is responsible for the in vitro and in vivo anti-schistosomal action whereas the (S)-PZQ isomer is significantly less effective (45). The initial effects caused by PZQ have been known for decades and can be summarized into three events: 1) calcium influx into the schistosome, 2) muscle contraction and 3) surface modifications (46). The damage to the tegument leads to the exposure of Schistosoma antigens and subsequent immune recognition and clearance. This may partly explain differences in PZQ sensitivity between adult and juvenile stages (47). The latter processes are calcium dependent, laying the foundation for the calcium hypothesis for PZQ action and provided insights into the potential drug target.
3.1 Voltage gated calcium channels
Studies initially suggested that voltage gated calcium channels (VGCCs) are involved in the mode of action of PZQ (48–54). It was found that S. mansoni possess a ‘variant’ beta subunit of VGCCs which lacks two serine residues in the beta interaction domain (BID) constituting putative phosphorylation sites in the ‘conventional’ subunit (26, 49). Intriguingly, co-expression of the variant S. mansoni beta subunit with the conventional mammalian alpha 1 subunit in Xenopus oocytes and mutagenesis of the two-phosphorylation sites in a conventional mammalian beta subunit resulted in novel sensitivity to PZQ (49). Furthermore, other PZQ susceptible helminths (Taenia solium and Clonorchis sinensis) also possess the variant beta subunit of VGCCs, supporting the notion that the variant beta subunit of VGCCs is the drug target (46, 54). However, the PZQ binding site in VGCCs is yet to be identified.
In contrast other findings have suggested that VGCCs do not play a major role in PZQ sensitivity in schistosomes (51, 52). PZQ action is only partially inhibited by classical calcium channel inhibitors (nicardipine and nifedipine) and is completely abolished if schistosomes are pre-incubated with actin depolymerizing agent cytochalasin D (50). It has been demonstrated that although PZQ-mediated calcium influx in schistosomes is increased by cytochalasin D, they fail to display the expected sequence of events resulting in death (51). Additionally, juvenile schistosomes express the variant beta subunit of the VGCCs, but are not susceptible to PZQ. In the same report, the authors incubated adult and juvenile stages in medium containing radioactive Ca2+ and undertook in vitro PZQ exposure. Interestingly, the authors demonstrated that in the presence of the drug, PZQ-refractory juveniles experience a large influx of Ca2+ followed by muscular contraction and paralysis, but the worms are able to recover (51). PZQ induced Ca2+ influx was not correlated with worm death in two instances; in adult schistosomes incubated in cytochalasin D and in juvenile schistosomes. Whilst these in vitro findings are of interest, they only provide a partial insight into the mode of action of PZQ, a drug known to work in combination with the host’s immune system in killing schistosomes in vivo. These findings do not rule out that PZQ could be acting on VGCCs as part of its mode of action.
3.2 Schistosoma mansoni transient receptor potential melastatin ion channel PZQ
More recent significant advances have been made in genomic methods and work by Park et al. (55) and Le Clec’h et al. (27) s the S. mansoni transient receptor potential melastatin PZQ channel (SmTRPMPZQ) as being a key genomic target of PZQ response in S. mansoni. The discovery that the human transient receptor potential melastatin ion channel 8 (hTRPM8) is activated by (S)-PZQ, provided a structural blueprint to search for homologous targets in S. mansoni and led to the identification of SmTRPMPZQ candidate (Smp_246790.5) (55, 56). TRP channels are a diverse superfamily of cation channels, which can be activated by an extensive range of stimuli (57). Park et al. (55) used a pharmacological approach to elucidate that SmTRPMPZQ is the major target of PZQ and identified residues essential for the activation of PZQ (45, 55). Using Ca2+ imaging assays in human embryonic kidney 293 cells (HEK293) with heterologous expression of SmTRPMPZQ demonstrated that SmTRPMPZQ was stereoselectively activated by (R)-PZQ in the nanomolar range (EC50 ~ 150 nM), which led to a sustained cellular Ca2+ signal. The characteristics of PZQ action at SmTRPMPZQ nanomolar potency of PZQ, stereoselectivity and Ca2+ entry, mirror the key events of PZQ action in S. mansoni (45, 57). Currently, it is believed that SmTRPMPZQ provides the link between PZQ and Ca2+ (57). To further support these findings, it was found that the TRP channel antagonist La3+ blocked PZQ induced muscular paralysis in 5/15 experiments (58). Modelling and mutagenesis data from Park et al. (55) suggest that (R)-PZQ interacts with SmTRPMPZQ through a transmembrane binding pocket to induce channel activity and subsequent calcium influx and muscle contraction. Intriguingly, sequences of the SmTRPMPZQ homologues from PZQ sensitive flukes Clonorchis and Opisthorchis display complete conservation of the PZQ binding site (45, 55). Further studies are required to elucidate the properties of SmTRPMPZQ homologues in other PZQ sensitive flukes.
SmTRPMPZQ is a large gene (120 kb), and seven alternatively spliced isoforms exist but are yet to be functionally characterized. This is of importance as different isoforms will likely display unique properties, including isoforms that act as dominant negatives (59, 60). Characterization of the different isoforms will be important for understanding how SmTRPMPZQ activity is regulated throughout the different Schistosoma lifecycle stages.
3.3 Other PZQ drug targets
It has been suggested that the anti-schistosomal action of PZQ is mediated through binding to myosin light chain (61), glutathione transferase (62), inhibition of sphingomyelinase activity (43), inhibition of phosphoinositide turnover (63), inhibition of nucleoside uptake (63), reduction of schistosomal glutathione concentration (63), alteration of membrane fluidity (63) or antagonism with S. mansoni tegumental allergen like protein 1 (SmTAL1) (64). However, these suggested targets require rigorous investigation beyond the initial report, and it is not clear if these targets facilitate the dual pillars of PZQ action in schistosomes (paralysis and tegumental damage) (57, 63).
4 Proposed drug resistance targets
4.1 SmTRPMPZQ
Mutations of the drug target can alter the ability for the drug to bind and can interfere with the activity of the target. Potential PZQ resistance may result from genetic mutations in SmTRPMPZQ reducing PZQ activity in S. mansoni. Mutagenesis data (55) generated from in vitro functional assays in cells heterologously expressing SmTRPMPZQ found that mutations within 20 out of 23 residues lining the PZQ binding pocket in SmTRPMPZQ decreased sensitivity with many resulting in a complete loss of PZQ activity (55). Sequence analysis revealed a single amino acid difference in the predicted PZQ binding pocket of Fasciola hepatica FhTRPMPZQ that reflects a single nucleotide polymorphism (SNP) (ACT F. hepatica and AAT S. mansoni). This SNP, encoding an amino acid change from asparagine to threonine, rendered cells expressing FhTRPMPZQ resistant to PZQ and reciprocal point mutations confirmed this finding (55). These findings demonstrate that SNPs in the PZQ binding site in SmTRPMPZQ may underlie PZQ resistance. However, in vivo studies involving mutagenesis of SmTRPMPZQ in adult schistosomes are required to determine if SNPs in the drug-binding site causes PZQ resistance in S. mansoni.
Recently, Le Clec’h et al. (27) investigated the genetic basis underpinning the variation in PZQ sensitivity in a PZQ-selected S. mansoni laboratory population where 35% of worms survive high-dose PZQ treatment. The authors noted an unusual dose-response relationship in the laboratory derived ‘PZQ resistant’ S. mansoni strain, where worms demonstrated significant heterogeneity in response to PZQ. Some adult worms displayed a PZQ sensitive phenotype whilst others could withstand high drug doses, demonstrating that the ‘PZQ resistant’ S. mansoni strain was a mixed population with significant genetic variation for PZQ susceptibility/resistance. Therefore, a lactate assay to measure adult worm recovery following in vitro PZQ exposure was used to create pools of adult worms at either extreme (resistant or susceptible).
Genome-wide association analysis was used to compare the pools of worms at the differing extremes of PZQ response and map loci underlying PZQ sensitivity, two major peaks in the quantitative trait locus (QTL) on chromosome 2 and 3 were identified (27). Interestingly three partial ABC transporters, a VGCC subunit and SmTRPMPZQ were identified under the QTLs on these chromosomes, indicating the involvement of multiple loci in the PZQ resistance phenotype. The causative gene underlying the extreme PZQ resistance phenotype, SmTRPMPZQ, showed recessive inheritance, meaning only homozygotes carrying two copies of the SmTRPMPZQ- 741987C allele survived PZQ treatment. Two additional key findings were: firstly, the SNP (at position 1,029,621 T>C) marking the highest association peak was found in the SOX13 transcription factor which may be a role in the regulation of splicing variants (65); secondly, two large (150 kb) deletions were found, 6.5 kb adjacent to SmTRPMPZQ and 170 kb from the SOX13 transcription factor, respectively.
The authors then used marker-assisted selection of S. mansoni at a single SNP in SmTRPMPZQ to select for two populations: PZQ-enriched resistant and sensitive parasites. Comparison between the two populations showed a >377-fold difference in PZQ treatment response, and the resistant population exhibited reduced expression by 2.25-fold of SmTRPMPZQ. The 150 kb deletions were found to be close to fixation in the resistant population. Interestingly, the PZQ resistant S. mansoni population showed long-term stability with no reported fitness cost. Known activators and blockers of SmTRPMPZQ were shown to increase or decrease PZQ sensitivity respectively. Sex and stage differences in expression levels of SmTRPMPZQ were found between the selected S. mansoni populations (27). Both adult male and female worms from the selected resistant population showed a reduction in SmTRPMPZQ expression compared to the sensitive population. Of note, expression in female adult worms was >11 fold lower than in male worms, consistent with females being naturally PZQ tolerant (27). However, an unexpected finding was that both female and male juvenile schistosomes demonstrated higher levels of expression compared to adult worms (27). This indicates that downstream events are responsible for the inaction of PZQ against juvenile stages. Together, these findings led authors to conclude that PZQ response in S. mansoni may be due to expression patterns controlled by regulatory variants associated with the SOX13 transcription factor or the adjacent 150kb deletion. Furthermore, more evidence is required to determine whether altering the expression of different isoforms of SmTRPMPZQ can alter schistosome sensitivity to PZQ.
Further screening, by Le Clec’h et al. (27) of 259 field-collected/originated (Niger, Tanzania, Senegal, Oman, and Brazil) S. mansoni samples (miracidia, cercariae and adult worms) did not identify PZQ resistance conferring SNPs in natural S. mansoni populations. A single stop codon in a heterozygous state was identified from a S. mansoni sample collected from a rodent in Oman, thus unlikely to result in PZQ resistance. The study was not targeted towards samples from foci where PZQ efficacy maybe an issue, such as “hotspots” and so these would be good targets for future investigations. Interestingly whole genome sequencing (WGS) of miracidia from a Ugandan hotspot did not find evidence of genomic selection acting on SmTRPMPZQ (66). A possible reason for the lack of detection of PZQ resistant alleles in field derived S. mansoni populations is that PZQ resistance may be focal and PZQ resistant alleles maybe rare (67). Sample sizes from current studies (27, 66) are underpowered to detect PZQ resistance at a low allele frequency. Another important consideration is that SmTRPMPZQ was found to underlie resistance in a single laboratory-generated resistant line where PZQ resistance was induced in larval stages at the intramolluscan stage not typically exposed to drug pressure in the wild (27, 67). The selected drug resistance mechanism at the intramolluscan stage may differ from that in mammalian hosts. Additionally, the S. mansoni strain used is of Brazilian origin, which does not represent the extensive diversity observed in East African S. mansoni populations. It remains unclear if the PZQ resistance mechanism involving SmTRPMPZQ is conserved in African S. mansoni populations.
4.2 ABC transporters
ATP-binding cassette (ABC) transporters are proteins involved in the transmembrane flow of toxins and xenobiotics (68, 69). P-glycoprotein (P-gp) and multi-drug resistance (MDR)-associated proteins (MRPs) represent two classes of these transporters (68, 69). ABC transporters have been associated with drug resistance in parasites such Plasmodium falciparum (70, 71) and parasitic helminths (70–73). The S. mansoni genome has 24 genes predicted to encode ABC transporters (74) where three ABC transporters have been characterized: SMDR1, SMDR2 and SmMRP1 (25, 69). Increased activity of P-gp has been demonstrated in laboratory generated resistant isolates (25, 75, 76). PZQ is hypothesized to interact with MDRs or MRPs either as an efflux-substrate or as a competitor of transport mediated by the ABC-transport proteins (77). Other authors have also shown an increased expression of SmMDR2 RNA in PZQ resistant clinical isolates of S. mansoni (24, 25, 73, 78, 79). Intriguingly, a recent study involving WGS of miracidia collected from an area of endemicity in Uganda, found evidence of positive selection on several genes including SmMDR1 (66).
It has been hypothesized that the factors underpinning the PZQ refractory nature of juvenile schistosomes are two-fold: higher basal levels of SmMRP1 and SmMDR2 mRNA compared to mature adult schistosomes, and the ability to mobilize an effective transcriptomic response to PZQ (80). The transcriptomic flexibility of juvenile S. mansoni worms was demonstrated by Hines-Kay et al. (80); juveniles exposed to PZQ had significantly elevated expression of SMDR1, SMDR3, SmMRP1 and SmMRP2 transcripts (80).
Drug efflux through ABC transporters has also been proposed as a mechanism of PZQ resistance in adult schistosomes. Work involving the use of fluorescent substrates of Pgp and MRP as probes for the presence of these transporters discovered that ABC transporters are localized in the S. mansoni excretory system (81, 82). Following PZQ exposure, the pattern of fluorescence was dramatically disrupted (83, 84), but this did not occur in an experimentally induced PZQ resistant S. mansoni strain (85). Furthermore, a significant induction of SmMRP1 RNA in adult mixed sex infection and in mature males following six-hour exposure to PZQ was demonstrated (73). Pinto-Almeida et al. (75) demonstrated the potential involvement of SMDR2 in PZQ drug resistance in S. mansoni. An increase in P-gp-like efflux pump activity in male worms from resistant strains was shown as well as an increase in SmMDR2 expression following incubation in a sub-lethal concentration of PZQ. However, PZQ resistant adult female worms showed no significant change in SmMDR2 expression following exposure to PZQ and expression was approximately 10 times lower than in PZQ-susceptible females. Interestingly, PZQ has been shown to be an inhibitor and substrate for SMDR2. SMDR2 and SmMRP1 mRNA levels increase in females and males from single sex infections following exposure to PZQ (25).
4.3 Voltage gated calcium channels
Kohn et al. (86) observed that cells expressing the structurally unusual beta subunit of VGCCs exhibit novel PZQ sensitivity. This led to the hypothesis that mutations in the gene encoding the beta subunit are responsible in PZQ resistance in schistosomes. This hypothesis was further investigated; no mutations in the sequence nor changes in expression levels of the beta subunits between S. mansoni isolates varying in PZQ sensitivity were found (87). Moreover, studies have failed to find a significant difference in the expression of the beta subunit between sensitive adults and PZQ refractory juveniles (88, 89). These findings suggest changes in expression or mutations in the beta subunit in VGCCs are unlikely to underlie the PZQ resistance phenotype in S. mansoni.
5 Performance of PZQ in the field
Resistance to PZQ treatment is a complex issue given that until recently no molecular targets of PZQ in schistosomes were known (45, 90). There is a lack of robust methods for detection of true resistance in the field and clear universal criteria to classify a schistosome strain ‘PZQ resistant’ (43, 91, 92). Furthermore, drug resistance studies are restricted due to inaccessibility of adult schistosomes, thus larval stages (eggs and miracidia) can be collected and preserved for genetic analyses, although this is constrained by small quantities of DNA available from larval stages (93). A phenotypic assay to test for reduced PZQ efficacy in miracidia has been proposed (94) however; it is unclear if the miracidial phenotypic change upon PZQ exposure is underlined by a resistance genotype. Most published discussions on this topic conclude that there is insufficient evidence for the emergence of PZQ resistance in field schistosome populations of clinical relevance (36–38, 95, 96). Yet there are concerns surrounding changes in susceptibility status of schistosome populations in the field due to low CRs reported in endemic areas, treatment failures in infected travelers, and laboratory selection for resistant schistosomes (97–99).
5.1 Senegal
Following mass scale use of PZQ in Senegal, low CRs (18-39%) were reported in 1991 (31). Despite increasing the dose to 60 mg/kg, the CRs remained significantly low (35). Early studies have hypothesized and suggested that the low CRs are due to drug resistant S. mansoni populations. However, the lack of PZQ resistance markers and poor knowledge of the S. mansoni genome at the time did not allow authors to test this hypothesis genetically (37). Reported egg reduction rates (ERR) were satisfactory at 86%. It is likely that the low CRs observed are due to focal epidemiological factors; very intense transmission, high worm burdens, expanded S. mansoni distribution, the presence of PZQ tolerant juveniles at the time of treatment, differences in drug metabolism and rapid re-infection, and possibly not drug resistance (37).
Initial studies involving the use of an S. mansoni laboratory passaged strain obtained from Senegal demonstrated decreased susceptibility to PZQ (100–102). This finding may be an artefact of early treatment as mice were treated with PZQ at day 35-day post infection. The isolate was established from infected snails thus it is unclear as to the clinical relevance of these findings. A further study involved PZQ treatment of infected mice 60 days post infection, which showed, improved efficacy of treatment (100). Schistosome strains can vary in maturation times and thus the time it takes to become susceptible to PZQ (100). In addition, laboratory passage can impose genetic bottlenecks, sampling error and selection biases, therefore the resulting parasite line may not be representative of the true extent of genetic diversity seen in the original field population (103). Another study involved the comparision of oxamniquine (OXA) and PZQ treatment in this area revealed CRs of 79% and 36% for each drug respectively (104). But the ERRs were found to be within the normal range for both groups depsite the low CRs observed. Furthermore, the two drugs differ in their mode of action where OXA does not appear to require a host immune response for parasite clearance whereas PZQ does (104). Currently, there is no convincing evidence of PZQ resistance in S. mansoni in Senegal, however further investigations of larger sample sizes are warranted.
5.2 Egypt
Another important foci for PZQ resistance in S. mansoni is Egypt where PZQ treatment for schistosomiasis was widely adopted and involved the use of 60 million PZQ tablets between 1997 and 1999 (105). A study involving the treatment of S. mansoni infected patients with three doses of PZQ, with the last dose increased to 60 mg/kg, found that 2.4% of patients were still egg positive post treatment. Following the first dose of 40 mg/kg PZQ, satisfactory CRs were observed (34). It should be emphasised that patients were fasted prior to recieiving the second and third PZQ treatment. This can impact key PK parameters as the bioavailibility of PZQ increases with food administration, thus can play a role in the failure of patients to successfully clear the S. mansoni infection (106). Laboratory lifecycles were set up using eggs collected from stool before treatment from cured or uncured patients, respectively. S. mansoni isolates cultured in vivo from uncured patients gave rise to S. mansoni worms demonstrating lower sensitivity to PZQ when compared with isolates from cured patients (34, 107). Interestingly, following multiple passages through laboratory rodents and snails, in the absense of PZQ drug pressure, approximately half the isolates retained reduced efficacy to PZQ (108). This demonstrates the heritibility of this trait and does not require PZQ drug pressure for maintainence. However, the dose of PZQ required to reduce schistosome worm counts in mice by 50% (ED50) differs between the isolates from uncured and cured patients were modest (2-3 fold) and is not at the level indicative of resistance to PZQ. Further studies carried out 10 years later, within the same area, were unable to show any establishment of PZQ resistance despite a decade of annual drug pressure (96).
5.3 Kenya
More recent reports of suspected PZQ resistance have come from Kenya (32). Using an in vitro drug susceptibility assay it was found that miracidia varied in their susceptibility to PZQ; of interest, miracidia from patients who were previously treated with PZQ displayed significantly lower susceptibilty to the drug. Eggs excreted by a patient (KCW- whose infection was never completely cured despite receiving over 18 PZQ treatments) were used for in vivo and in vitro drug susceptibilty assays. Reduced susceptibility of a sub-isolate from KCW remained through at least six lifecycle passages which demonsrates the trait’s heritability, despite the absence of drug pressure. Of interest, an estimated odds ratio of 2.42 was obtained when comparing survival of miracida in a previously treated and an untreated group. This is a modest difference in PZQ susceptibility and is unlikely to reflect full PZQ resistance. It is important to highlight that the study cohort of car washers in Lake Victoria are exposed to S. mansoni contaminated water everyday for extended periods of time. Thus it is likely that the inability to achieve cure was due the elevated exposure to re-infection.
5.4 Uganda
An alarming finding from the Schistosomiasis in Mothers and Infants (SIMI) longitudinal study was that despite two PZQ administrations within a six-month period, there was little impact on reducing the local prevalence of intestinal schistosomiasis (109). Initially, this was thought to be due to rapid re-infection (109) or under dosing of PSAC (110). However, Sousa-Figueiredo et al. (111) compared PZQ efficacy from a random sample of children from the SIMI cohort ‘previously treated’ and a random sample of children who had not been previously treated with PZQ ‘treatment naïve’. Results revealed lower CRs in previously treated children (41.7% in those receiving any prior treatment and 29.2% in those receiving three treatments) compared to CRs of 78% in treatment naïve children (111). Age and intensity of infection were not found to explain these differences (111).
These early reports of suspected reduced PZQ efficacy of S. mansoni within endemic field settings do not provide sufficient evidence to show that PZQ resistant schistosomes are repsonsible for apparent treatment failures. In support of this conclusion a recent meta-analysis of 146 articles reporting PZQ efficacy as CR and ERR, has concluded that PZQ has retained its efficacy despite its extensive use for over four decades (39). The meta-analysis indicated that ERR is a more consistent measurement of PZQ efficacy compared to CR (39). The use of CRs to assess PZQ efficacy may lead to an underestimation of drug efficacy. The results from the analysis indicated that there was no evidence of schistosomes developing resistance or tolerance against PZQ. This raises the important question of why PZQ resistance in schistosomes has not been found widespread in field isolates
6 Factors preventing the establishment of PZQ resistance in the field
An array of factors could prevent the emergence and establishment of PZQ resistance in the field, and are further considered in this section (Figure 3).
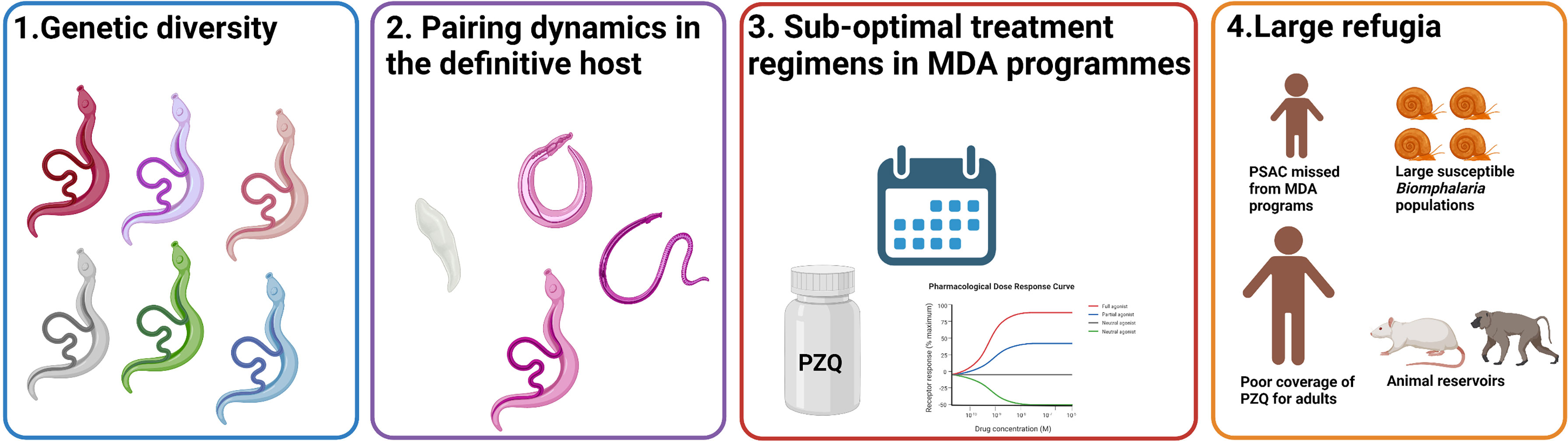
Figure 3 Contributing factors preventing the development of PZQ resistance in field S. mansoni populations. The extensive genetic diversity (1), complicated pairing dynamics of schistosomes (2), sub-optimal treatment regimens (3), and large refugia (4) are four key factors which may reduce the likelihood of PZQ resistance alleles fixing in field S. mansoni populations. Created with BioRender.com.
6.1 Genetic diversity of S. mansoni in response to PZQ treatment
Schistosoma mansoni exhibits high genetic diversity with a conspicuous amount of genetic diversity in East Africa (112) and displays a strong population structure at a country level (113–115). The high levels of genetic diversity seen in S. mansoni can reduce the likelihood of resistance alleles fixing within a population and help populations recover from bottlenecking selection following PZQ treatment (116).
Many factors can influence the genetic diversity of S. mansoni populations; the clonal nature of schistosomes within the intermediate snail host, and the fact that the snail host is typically infected with only few genotypes, may mitigate parasite genetic diversity (117, 123). In contrast, several factors can favor increase of the genetic diversity of schistosomes: mobility and long lifespan of the definitive host, genotype specific re-infection, snail-parasite compatibility and the rapid turnover of infected snail hosts (117). Within this context, the definitive host acts as a ‘genetic mixing bowl’ for the parasite (124). Population genetic studies have reported panmictic populations (all individuals within the population are potential partners) with most genetic variation and diversity occurring within individual human hosts rather than between different hosts (66, 125, 126). Interestingly, S. mansoni does not appear to display a strong intra- or interpopulation structure between hosts (117). This may be due to hosts in the same village being exposed to different microenvironments, which can facilitate individuals being exposed to different parasite populations (127).
It is essential to monitor any changes in schistosome genetic diversity and population structure to understand the impact of PZQ MDA (97). A reduction in genetic diversity following PZQ treatment may indicate that the schistosome population will be less likely to adapt to drug pressure, whereas an increase in genetic diversity may indicate high gene flow between schistosome populations (97). Regular mass treatment with PZQ for the broad-scale elimination of schistosomiasis could exert a significant selective pressure on Schistosoma parasite infrapopulations (population within a human host), which may increase the risk for the development of drug resistance (38, 117). Population genetic studies have investigated how schistosome populations have changed following PZQ MDA (Supplementary Table 1). These studies have been restricted to a small number of molecular markers including cytochrome oxidase subunit 1 (cox1) and microsatellite markers. More recently, studies analyzing the whole genome or exome sequences have emerged for S. mansoni (66, 128, 129). Parameters such as population structure and genetic diversity can be used to deduce demographic changes and efficacy of schistosome control interventions (66). A study compared S. mansoni populations from two Tanzanian schools pre- and post-treatment, using microsatellite markers (97). A significant decrease in genetic diversity was found at six months following a single round of PZQ treatment and the infrapopulations were significantly differentiated between the two time points. This is indicative of rapid re-infection. A reduction in genetic diversity was also observed in the control group of untreated PSAC, thus demonstrating the strong and long-term effect of PZQ on the population structure of S. mansoni. A follow up study (130) visited the same schools five years later and found a significant increase of genetic diversity in infrapopulations compared to the baseline estimates (97). A significant reduction in worm burdens within individual hosts was observed, indicating that PZQ had reduced infrapopulation sizes but not to the extent to result in a genetic bottleneck of the whole parasite population. These findings suggest that factors other than PZQ treatment may have caused the initial decline in genetic diversity (97) and the subsequent increase (130). This could reflect changes in temporal parasite transmission dynamics and high levels of re-infection as indicated by higher FST (the proportion of total genetic variance contained in a subpopulation) between years compared to between schools.
A long-term reduction in genetic diversity and the infrapopulation size following PZQ treatment could not be found by other studies. Studies had found either no change in genetic diversity or an increase in genetic diversity in S. mansoni populations following PZQ treatment (Supplementary Table 1). A study (116), which extensively sampled SAC from three primary schools at 11 time points, found a short-term reduction in allelic richness at 4 weeks after treatment, indicative of a PZQ induced genetic bottleneck, which rapidly recovered at six months post treatment. This indicates that high levels of gene flow ensure rapid recovery of the genetic diversity of S. mansoni populations, which may prevent the fixation of PZQ resistant/less tolerant genotypes (117–122). An interesting observation from Huyse et al. (131), was that despite six PZQ treatments within a two-year period, there was no difference in the genetic diversity of, and genetic differentiation between, S. mansoni infrapopulations before and after repeated PZQ treatment. This suggests that S. mansoni infrapopulations remained relatively stable over the course of treatment and is indicative of a surviving parasite population, which is tolerant to the administered PZQ dose (117).
Currently, it is difficult to apply comparative analyses to studies (Supplementary Table 1) due to the heterogeneity of methodologies used for example, differences in the molecular markers, follow up times, sampling design and genetic analysis used (117). Therefore, there is a need to adopt standardized procedures to investigate how S. mansoni populations respond to PZQ treatment. The lack of genetic markers for PZQ resistance has hindered the ability of these studies to understand schistosome genetic response to drug treatment. There is a need for the development of more powerful non-neutral molecular markers at the genome level to assess the extent of the genetic bottleneck post treatment and determine re-infection from persistent infections (13).
6.2 Schistosome pairing dynamics within the definitive host
Schistosomes are dioecious which enables the interaction between male and female parasites within the definitive mammalian host vasculature (130, 132). The male embraces the female schistosome within its gynaecophoric canal, forming a worm pair. Sexual development and egg production of female schistosomes is governed by this physical contact and expression of GLI1 (133). Interestingly, schistosomes do not form a worm pair ‘for life’ but are promiscuous and change partners (134).
The complex nature of schistosome pairing dynamics within the definitive host may pose a barrier to the establishment of the PZQ resistant trait in schistosome populations. For example, these interactions can lead to heterospecific worm pairings and subsequent hybridization, which may affect a range of phenotypic traits including PZQ sensitivity (135). Furthermore, studies have shown that PZQ sensitivity in schistosomes depends on several factors including the parasite stage, the state of adult pairing and the sex of schistosomes (43). Notably female schistosomes have demonstrated lower sensitivities to PZQ (136). Assuming PZQ randomly kills adult worms within the definitive host, a subset of the initial infrapopulation can remain (117). Studies have shown cessation of egg production following treatment despite infected individuals remaining antigen positive (137). This may be due to a single sex infection or disrupted sex ratio post-treatment. If female worms remain post-treatment, the lack of male worms lead females to revert to an immature stage and stop egg production (128). Notably, in vivo studies have found a decrease in the male: female ratio following PZQ treatment (136, 138).
Hosts in endemic areas are repeatedly exposed to contaminated water and become re-infected (139). Incoming schistosomes can pair with the remaining infrapopulation, which may coincide with the resumption of egg production. This can result in the PZQ resistance trait becoming rapidly outbred. Studies have demonstrated that PZQ resistance in schistosomes is recessively inherited, thus only homozygotes express this trait (27). There is a significant obstacle to the establishment of recessively inherited traits because they are present in heterozygotes at a low frequency and are consequently not exposed to selection (140). The bias against the establishment of recessives is referred to as ‘Haldane’s sieve’ (141) and is of particular relevance in the evolution of drug resistance in diploid organisms as mutations in drug targets often result in a resistance phenotype when two copies are present (140). The rate that drug resistance alleles spread within a population in response to drug exposure is dependent on their initial frequency when the drug is introduced (142, 143). If no drug resistant alleles are present when a new drug is introduced, then there is a waiting time for them to arise and a low probability of fixation. However, it is predicted (140) that pre-standing variation for PZQ resistance is likely because this trait has been rapidly selected for by several groups (144, 145) in independent laboratory populations. Importantly, the laboratory populations used were isolated prior to the widespread availability of PZQ (144, 145), thus were not subject to PZQ selection, which strongly suggests PZQ resistant alleles were already present in these populations. This is concerning as this scenario was the case for OXA resistance (Figure 4), a trait which is also recessively inherited (146), but consequently rendered the drug futile (140).
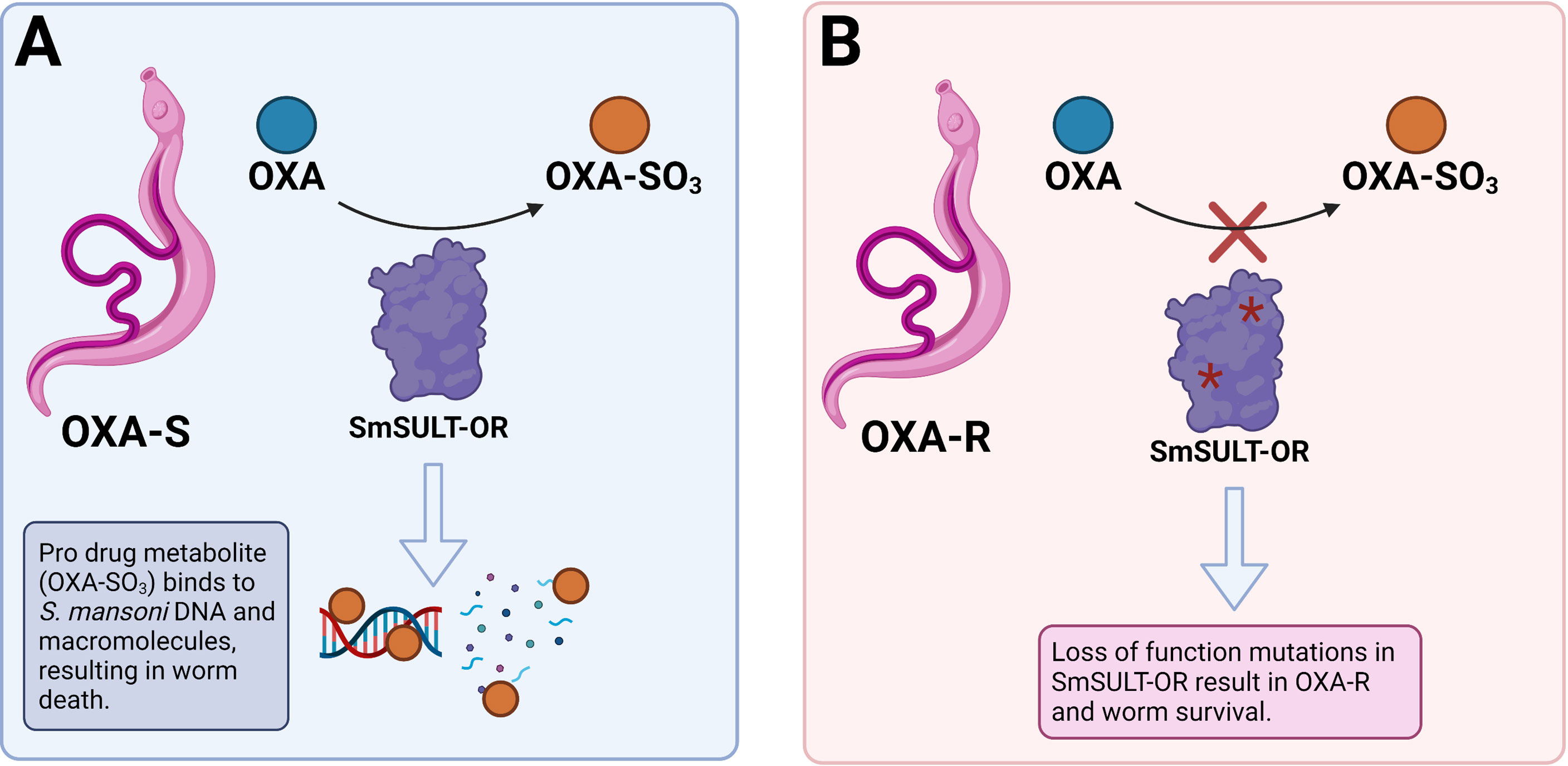
Figure 4 Mechanism of oxamniquine (OXA) resistance in S. mansoni. (A) OXA is a prodrug enzymatically activated by a sulfotransferase (SmSULT-OR) in susceptible S. mansoni (OXA-S). The activated OXA metabolite (OXA-SO3) binds to DNA and macromolecules which results in worm death. (B) Loss of function mutations in SmSULT-OR gene result in OXA-R and worm survival as the drug does not become activated by SmSULT-OR. Created with BioRender.com.
6.3 Sub-optimal treatment regimens in MDA programs
Sub-optimal treatment regimens for schistosomiasis control may pose a significant barrier to the widespread development of PZQ resistance in schistosomiasis. PZQ is administered in PC programs to infected children at a dose (40 mg/kg) extrapolated from adult tolerance studies (147). Since 2006, PC programs have delivered PZQ as a single oral dose to SAC and/or at-risk adult populations, depending on prevalence, in endemic communities. The current treatment regime has been challenged by recent pharmacokinetic (PK; the dynamics of administered drug concentration) and pharmacodynamic (PD; the impact of drug concentrations on parasite density) data which support a higher dose (110). Bustinduy et al. (110) conducted the first PK/PD study in children to compare two doses of PZQ (40 mg/kg and 60 mg/kg) for schistosomiasis treatment (110). Vast inter-individual PK variability was seen. A higher proportion of children receiving 60 mg/kg PZQ dose achieved a better reduction in infection intensity, however no child achieved antigenic cure during the study, suggesting that 40 mg/kg is insufficient, with modelling suggesting up to 80 mg/kg to achieve WHO recommended CRs. Only 75% individuals receiving 80 mg/kg PZQ had an estimated CR of >85%. Efficacy of higher doses (80 mg/kg in a split dose) are currently being explored in a randomized placebo-controlled trial in preschool children in Uganda (148).
Interestingly, the study revealed differences in enantiomeric activity on worm burden and found that the probability of achieving cure was strongly associated with high levels of (S)-PZQ. It is typically assumed that (R)-PZQ is the anti-parasitic enantiomer, it is worth noting that the (S)-PZQ enantiomer is efficacious against S. haematobium (149). The high levels of (S)-PZQ may cause the hepatic shift of paralyzed adult schistosomes into the liver to be eliminated. Both enantiomers have been shown to have opposing effects on vascular tone [R-PZQ causes contraction (150) whereas S-PZQ causes relaxation (151)] and may play a role in optimizing blood flow through splanchnic beds where schistosomes reside, thus contributing to their displacement (57). Given the much reduced efficacy of (S)-PZQ for SmTRPMPZQ (several logs), perhaps this enantiomer acts via a different schistosomal target that is still relevant for its mode of action. A recent PK study in S. mansoni or S. haematobium infected children also demonstrated higher area under the curves (AUC) for (S)-PZQ than (R)-PZQ (152). A relationship between (R)-PZQ AUC and Cmax (peak plasma concentration) and the probability of cure was indicated using logistic regression (153). However, this interpretation was based on a small sample size and more studies are required to determine which enantiomer is the eutomer of PZQ. To achieve a greater treatment success of individuals with schistosomiasis, the treatment regimens should place more emphasis on the PK and PD characteristics of PZQ as well as schistosome biology rather than solely logistics.
When administered as a single oral dose, PZQ acts only on adult schistosomes because the drug is rapidly cleared from the body in (80% cleared within four days of which 90% occurs in 24 hours) (9). Consequently, PZQ tolerant juvenile schistosome stages are not affected by drug treatment and mature into adults, resulting in a treatment inefficiency. The intermittent drug exposure (typically annual) and insufficient dosing of PZQ means that schistosomes have not been subject to sufficient drug selective pressure to result in the development of PZQ resistance of clinical relevance. Ironically, the aim of control rather than elimination of schistosomiasis has decreased the likelihood of widespread PZQ resistance occurring.
6.4 Large refugia
Within the context of drug resistance in parasitic helminths, a refugium refers to the proportion of untreated hosts or environments, which allow for the maintenance of drug sensitive parasites (Figure 3) (154). To maintain drug efficacy and combat selection pressure in veterinary helminths, refugia are used and it appears that the limited MDA coverage in endemic communities may have exerted a similar effect (66, 154). Until recently, not all infected individuals within an endemic community have equitable access to PZQ treatment. Notably adult and PSAC groups did not receive PZQ treatment during control programs. These groups act as refugia of diverse parasites, which support the panmictic S. mansoni population structure (66). The high rates of gene flow and diversity of S. mansoni populations could prevent selection and fixation of PZQ resistance by providing pools of susceptible alleles to dilute those conferring PZQ resistance selected in treated populations (SAC) (127). Despite multiple rounds of MDA, post-treatment schistosome populations are small in comparison to the size of refugia populations in snails, untreated humans and animal reservoirs of infection (primates (e.g., baboons and rodents) (127, 155). Mixing and high rates of gene flow between treated and untreated parasite populations allow for rapid recovery of parasite genetic diversity following treatment (66). Additionally, the long-life span of schistosomes is believed to increase the effectiveness of these reservoirs of parasite genetic diversity (66). Furthermore, the recent impact of the COVID-19 pandemic on PC delivery in endemic communities is expected to influence the refugia of schistosome populations. In 2020, 76.9 million people received PC compared to 105.5 million in 2019, due to pandemic control measures, which caused a suspension of PC programs in many endemic areas (156).
7 PZQ treatment failures within the context of confounding factors
Whilst drug resistant schistosomes have been suggested to play a role in the heterogeneity of CRs observed, it is important to investigate PZQ treatment failures in the context of confounding factors, which can affect drug efficacy. Quantifying PZQ treatment failures within the context of confounding factors are discussed in the following section (Figure 5).
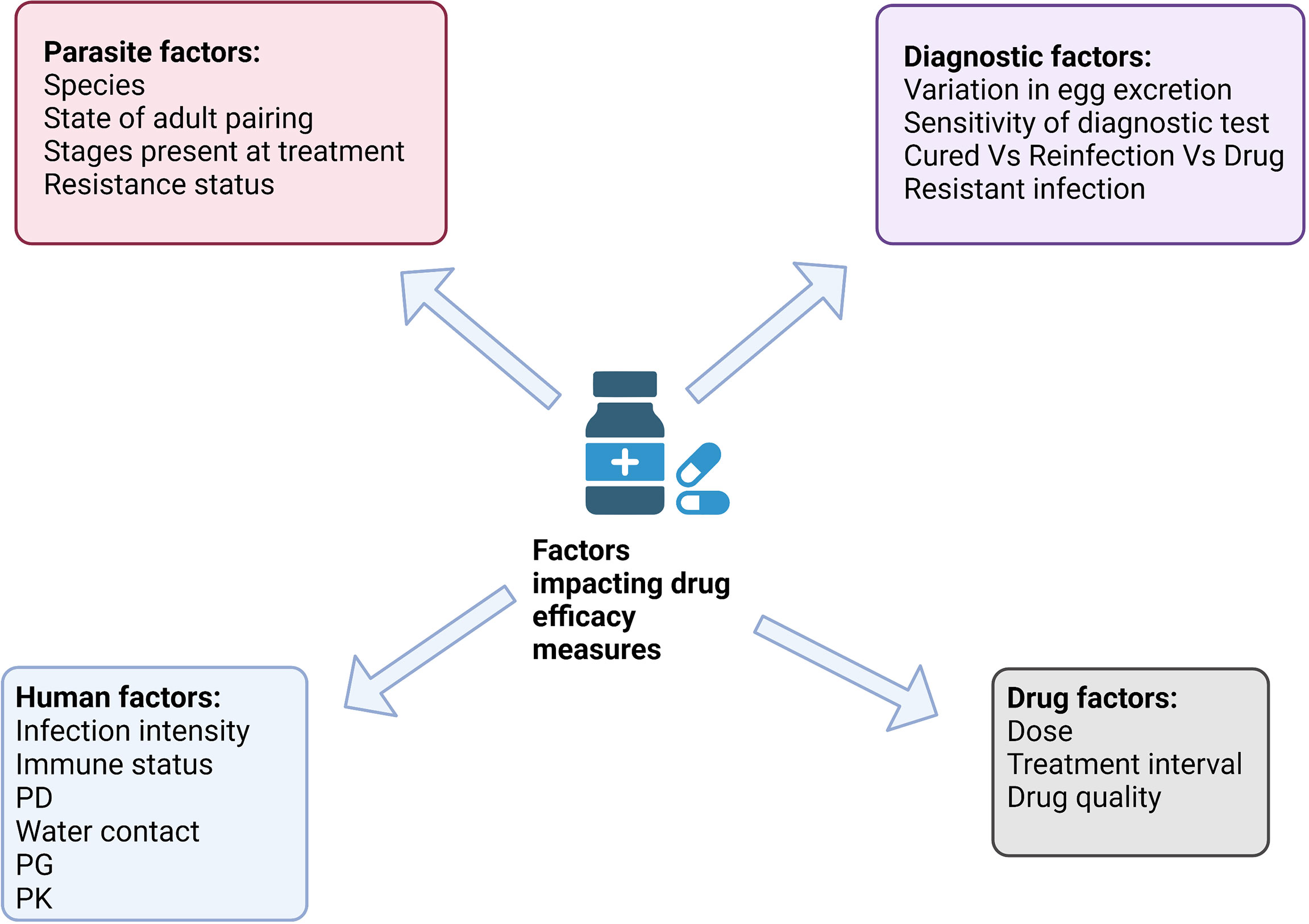
Figure 5 Factors impacting PZQ drug efficacy in schistosomes. Broadly parasite, diagnostic, human and drug factors are confounding factors when measuring the efficacy of PZQ in the field. Confounding factors can positively or negatively affect drug efficacy. Created with BioRender.com.
7.1 Diagnostic factors
The WHO recommends quantifying changes in egg excretion before and after PZQ treatment, as ERR and CR, to assess drug efficacy at an individual and community level (157). The tentative ERR threshold of 90% is an indicator of optimal efficacy for PZQ (11). By estimating the distribution of ERRs, changes in the efficacy of PZQ can be identified (158). Thus, the sensitivity of the diagnostic test used to assess PZQ efficacy is a key issue.
7.1.1 Parasitological diagnostics
Most drug efficacy studies use microscopy to quantify egg excretion, despite its lack of sensitivity to detect low intensity infections and treatment outcome. It has been demonstrated that individuals with low intensity infections are associated with decreased diagnostic accuracy before and after treatment (155, 159–161). This can lead to false negative results and the over estimation of drug efficacy. To improve diagnostic sensitivity, the number of parasitological samples should be increased (162). The use of at least six Kato-Katz (KK) tests (two smears per stool from three stools) has been suggested for more accurate prevalence assessment post-treatment (162). A recent meta-analysis (39) has demonstrated that an increase in the number of samples used had a significant impact for CR but not ERR, which demonstrates that ERR is less influenced by the diagnostic test sensitivity. Variation in egg excretion, can lead to incorrect diagnosis, underestimation of infection prevalence, and thus an inaccurate evaluation of drug efficacy (163, 164). Consequently, the ability to detect the emergence of drug resistant parasites is masked by the variable sensitivity of diagnostic methods based on egg detection following drug treatment. Diagnostic methods with superior sensitivity compared to microscopy are needed for the accurate mapping and identification of drug resistance hotspots.
7.1.2 Antigen detection
Antigen detection methods are a promising alternative to diagnostic microscopy. Specifically, the detection of schistosome gut-associated polysaccharides, circulating cathodic antigen (CCA) and circulating anodic antigen (CAA), have the potential to revolutionize field-based diagnostics (165). Urinary CCA-based diagnostic is used for detecting S. mansoni infections (166). CAA based diagnostic is able to detect all Schistosoma spp. infection and it is rapidly cleared from the bloodstream following PZQ treatment, thus its presence is indicative of an active infection (167). Hoekstra et al. (168) determined the efficacy of a single dose of PZQ (40 mg/kg) versus four repeated doses in SAC using either KK or point-of-care (POC)-CCA (168). The authors reported that POC-CCA gave considerably lower CRs compared to KK, which is suggestive of adult worms surviving treatment. Thus, depending on the diagnostic method used, the measures of drug efficacy (ERR and CR) can differ significantly. However, there are concerns surrounds false positive results from the POC-CCA (169, 170). Further explanations for a negative KK result and positive POC-CCA result include the presence of single sex infections either acquired or the result of drug treatment could explain the disruption of egg production despite an active infection (171–174). Mature adult worms surviving drug treatment may cease or reduce egg production whilst still excreting CCA.
7.2 Parasite factors
Parasite factors are important to consider when quantifying drug efficacy, for example human hosts can harbor different Schistosoma lifecycle stages at the time of treatment, from recently acquired juvenile stages to adult schistosomes. Different lifecycle stages and parasite sex are differentially sensitive to PZQ. This can lead to a disruption in the female: male sex ratio within the host and cause cessation of egg production despite active infection. Furthermore, juvenile schistosomes are not susceptible to PZQ induced killing and survive treatment. Thus, post-treatment, juvenile schistosomes go on to mature into adult schistosomes and produce eggs. This can explain the resumption of egg production at follow up parasitological surveys, which can appear as treatment failure (175, 176). It is difficult to determine at follow up if the resumption of egg production in treated individuals is the result of reinfection, juvenile schistosomes present at treatment maturing into egg producing adults or drug resistant schistosomes. The inaccessibility of adult schistosomes from human hosts further complicates genetic analysis to determine the source of egg resumption at post-treatment (reinfection versus drug resistant schistosomes).
7.3 Drug factors
Variation in the concentration of PZQ in the systemic circulation may contribute towards treatment failure in field settings, as the drug concentration may not be present at a lethal concentration to kill schistosomes. Bioavailability is one of the most important factors, which is used to indicate the fraction of an orally administered dose that reaches the systemic circulation as intact drug and has an anti-schistosomal effect (177). Apparent drug failures may be the result of issues with the metabolism of PZQ rather than innate parasite resistance. The effect of drug metabolism on treatment outcome has been evaluated by PK and PD studies (178, 179).
Furthermore, it has been proposed that drug quality is an important confounding factor for affecting PZQ efficacy. A study using healthy mice to determine the PK profile of different PZQ brands revealed vast differences in bioavailability between different PZQ brands (180). Furthermore, the efficacy of different PZQ brands (% worm reduction) was compared in mice infected with a PZQ susceptible or insusceptible S. mansoni strain. Diminished efficacy was reported in PZQ brands (Bilharzid, Epiquantel and T3A), which showed lower bioavailability (32-46% reduction), compared to pure PZQ, this difference was more prominent in the PZQ insusceptible S. mansoni (180). This study shows the importance of the quality of PZQ as an underlying factor for treatment failures in endemic areas.
Schistosomiasis is co-endemic with many other pathogens such as malaria, therefore, endemic populations are often subject to co-administration of different drugs that can give rise to drug-drug interactions (DDIs). CYPs are important enzymes in the first pass metabolism of many drugs. Proposed DDIs are discussed and reviewed in (181). Of interest, in humans it was found that the co-administration of PZQ and albendazole (ABZ) demonstrated a synergistic relationship where the AUC of (R)-PZQ increased by 76% (182). This is due to ABZ inhibiting CYP1A and CYP3A metabolic pathways involved in the metabolism of (R)-PZQ (182).
7.4 Human factors
It has been demonstrated that host factors, such as immune response and genetic variation in drug metabolizing enzymes, can influence the therapeutic response. PZQ is extensively metabolized by multiple Cytochrome P450 (CYP) enzymes, specifically CYP1A2, CYP2C19, CYP2C9, CYP2D6, CYP3A4 and CYP3A5 are involved (183). Host related factors can affect the metabolism and PK parameters of PZQ. Age-maturation of hepatic CYPs may affect the AUC of PZQ as the CYP pathways are not completely matured in children (110). For example, age-related metabolism has been shown where AUC values for PZQ were higher in PSAC compared to SAC (153). Higher concentrations of PZQ were found in PSAC whereas higher concentrations of (R)-4-OH-PZQ metabolite were found in SAC, suggesting that PSAC may metabolize PZQ more slowly. The comparatively higher exposure to PZQ may account for differences in CRs between the two age groups (153). Furthermore, liver morbidity has been shown to affect the metabolism of PZQ. Patients with liver cirrhosis were treated with PZQ and PK parameters were compared to healthy patients (147). Liver cirrhosis patients had a higher AUC and Cmax, which increased with increasing severity of cirrhosis (147). However, the extent of the impact of S. mansoni related liver morbidity on PZQ PK parameters is largely unknown and warrants further attention.
A less studied area of PZQ efficacy is host pharmacogenetics (PG; how genes can affect an individual’s response to a drug). Mutations in CYPs, primarily SNPs, have been associated with inter-individual variation of drug metabolism in efficacy and toxicity studies (152). It has been hypothesized that mutations in CYPs results in different PK of PZQ (13). This can lead to a variable PK effect. Different populations vary significantly in the distribution of CYP alleles hence it is of importance to optimize drugs for the targeted population (181). Currently, there have been no PG studies carried out to determine the genetic influences on PK of PZQ and to identify clinically relevant CYP variants in the target population (181). Such studies are required alongside parasite population genetic studies.
8 Other schistosome species
The current landscape of literature for drug resistance in schistosomes is saturated with S. mansoni studies, due to several factors; S. mansoni laboratory strains are established and characterized, the high-quality S. mansoni genome, PZQ resistance can be rapidly selected for and reports of reduced susceptibility to PZQ in S. mansoni. There is no evidence of S. haematobium developing resistance to PZQ where reported CRs in endemic areas have remained favorable. Furthermore, drug resistance in S. japonicum has received more attention in comparison, where PZQ resistance has been selected for laboratory rodents (184). Despite the extensive use of PZQ in control programs in China, PZQ has retained its efficacy against S. japonicum (185–187). There is a need for future studies to focus on other Schistosoma spp. response to PZQ treatment in the field and to characterize the molecular basis for drug resistance.
9 Discussion
The mechanism of action of PZQ has been a significant enigma in the schistosomiasis field for decades. Great advances have been made recently with two independent research groups (27, 45) identifying SmTRPMPZQ as a target of PZQ. Park et al. (55) used molecular modelling and targeted mutagenesis to identify the PZQ binding site. Le Clec’h et al. (27) used genome wide association followed by marker-assisted selection to identify SmTRPMPZQ as being the key determinant of PZQ response. Collectively, we speculate that the mode of action of PZQ involves multiple protein targets including SmTRPMPZQ and VGCCs, as part of a complex pathway resulting in disruption of S. mansoni calcium homeostasis and clearance. These recent advances provide a framework for future genetic surveillance for PZQ resistance. SmTRPMPZQ genotyping of natural S. mansoni populations could provide valuable insights into the variation within this gene and how this may affect treatment efficacy. Furthermore, genome-wide approaches should be undertaken to identify additional genomic regions under selective pressure and candidate genes for monitoring PZQ resistance. Although candidate gene approaches to monitor anthelminthic drug resistance have had mixed success to date, screening for non-synonymous mutations in the β- tubulin isotype 1, which confer resistance against the benzimidazole drug class used to treat the veterinary helminth Haemonchus contortus has been an useful example (188, 189).
These studies raised questions regarding the molecular basis for the natural tolerance of PZQ in juvenile schistosomes. It was shown that juveniles express higher levels of SmTRPMPZQ compared to adult schistosomes (27). This should be considered with another line of evidence; juvenile S. mansoni worms experience a Ca2+ influx and muscle contraction, but they recover from PZQ exposure. Perhaps, the molecular target of PZQ is the same for juveniles and adults but the research focus should be on juvenile recovery from drug exposure. Juvenile schistosomes are younger compared to adult stages, thus may be more successful to adapt and recover from drug pressure. The recovery of juveniles from PZQ warrants further research attention and will provide insights into the downstream effects following drug exposure.
PZQ resistance is a complicated issue, which should be considered within the context of confounding factors. The review highlighted significant gaps in knowledge particularly the scarcity of studies focusing on the genetic basis underlying the variability in the metabolism of PZQ, which can impact the efficacy of treatment. Furthermore, this review found no strong evidence for the emergence of drug resistant S. mansoni populations within field settings. The review proposes four key factors, which may explain the lack of PZQ resistance observed in field S. mansoni populations; the extensive genetic diversity, complicated pairing dynamics of schistosomes, sub-optimal treatment regimens and large refugia. Studies focusing on the genetic diversity and population structure of S. mansoni populations in response to MDA, and have suggested that rapid re-infection occurs. High levels of gene flow and large refugia rapidly restore the genetic diversity and provide pools of PZQ susceptible alleles to dilute drug resistance alleles. Whilst these studies have provided insights into how S. mansoni populations respond to PZQ treatment, more extensive genome-wide analysis is required. Particularly to provide greater resolution in determining re-infection from an infection which has failed to clear. MDA alone is insufficient to eliminate schistosomiasis and the drug pressure exerted by current drug treatment regimens appears to be inadequate to select for PZQ resistance.
10 Conclusion
The newly introduced WHO NTD roadmap and guidelines set an ambitious control strategy to achieve the elimination of schistosomiasis as a public health problem by 2030. PZQ remains at the forefront of schistosomiasis control and PC programs play a vital role in the integrated control strategy. However, the proposed scale up of PZQ use poses an increased risk to the development of PZQ resistance in schistosome populations. Thus, it is essential to monitor for changes in drug efficacy and for the emergence of PZQ resistant schistosomes to reduce the threat to the 2030 WHO control strategy. At present, there are no anti-schistosomal drugs undergoing clinical trials in humans, thus we must strive to retain the usefulness of PZQ in the schistosomiasis control toolbox.
Author contributions
SS conceptualized and wrote the first draft. TB, FA, JRS, AE, BLW, MAM, and ALB critically reviewed, edited, and approved the final version of the manuscript.
Funding
SS receives studentship funding from the UK Medical Research Council London Interdisciplinary Doctoral program (MR/N013638/1).
Conflict of interest
The authors declare that the research was conducted in the absence of any commercial or financial relationships that could be construed as a potential conflict of interest.
Publisher’s note
All claims expressed in this article are solely those of the authors and do not necessarily represent those of their affiliated organizations, or those of the publisher, the editors and the reviewers. Any product that may be evaluated in this article, or claim that may be made by its manufacturer, is not guaranteed or endorsed by the publisher.
Supplementary material
The Supplementary Material for this article can be found online at: https://www.frontiersin.org/articles/10.3389/fitd.2022.933097/full#supplementary-material
References
1. Schistosomiasis (2021). Available at: https://www.who.int/news-room/fact-sheets/detail/schistosomiasis.
2. Standley CJ, Dobson A, Russell J. Out of animals and back again: Schistosomiasis as a zoonosis in Africa. In: Schistosomiasis (2012), 209–30. doi: 10.5772/25567
3. Colley DG, Bustinduy AL, Secor WE, King CH. Human schistosomiasis. Lancet (2014) 382:2253–64. doi: 10.1016/S0140-6736(13)61949-2
4. McManus DP, Dunne DW, Sacko M, Utzinger J, Vennervald BJ, Zhou XN. Schistosomiasis. Nat Rev Dis Primers (2018) 1:1–19. doi: 10.1038/s41572-018-0013-8
5. Bosompem KM, Bentum IA, Otchere J, Anyan WK, Brown CA, Osada Y, et al. Infant schistosomiasis in Ghana: a survey in an irrigation community. Trop Med Int Health (2004) 8:917–22. doi: 10.1111/j.1365-3156.2004.01282.x
6. Odogwu SE, Ramamurthy NK, Kabatereine NB, Kazibwe F, Tukahebwa E, Webster JP, et al. Schistosoma mansoni in infants (aged <3 years) along the Ugandan shoreline of lake Victoria. Ann Trop Med Parasitol (2006) 4:315–26. doi: 10.1179/136485906X105552
7. Meurs L, Mbow M, Vereecken K, Menten J, Mboup S, Polman K. Epidemiology of mixed schistosoma mansoni and schistosoma haematobium infections in northern Senegal. Int J Parasitol (2012) 3:305–11. doi: 10.1016/j.ijpara.2012.02.002
8. Squire B, Stothard JR. Schistosomiasis. In: Beeching N, Gill G, editors. Tropical medicine lecture notes, 7th ed. John Wiley & Sons, Ltd., The Atrium, Southern Gate, Chichester, West Sussex PO198SQ, UK (2014). p. 151–62.
9. Andrews P, Thomas H, Pohlke R, Seubert. Praziquantel. Medicinal Res Rev (1983) 3:147–200. doi: 10.1002/med.2610030204
10. World Health Organization. Prevention and control of schistosomiasis and soil-transmitted helminthiasis: Report of a WHO expert committee. WHO Technical report series (2002) 912:24–46.
11. Crompton DWT, World Health Organization. Preventive chemotherapy in human helminthiasis – coordinated use of anthelminthic drugs in control interventions: A manual for health professionals and programme managers. World Health Organization (2006). Available at: https://apps.who.int/iris/handle/10665/43545
12. World Health Organization. Schistosomiasis and soil-transmitted helminthiases: numbers of people treated in 2019. Wkly Epidemiol rec (2020) 95:629–40.
13. Mawa PA, Kincaid-Smith J, Tukahebwa EM, Webster JP, Wilson S. Schistosomiasis morbidity hotspots: Roles of the human host, the parasite and their interface in the development of severe morbidity. Front Immunol (2021) 0:751. doi: 10.3389/fimmu.2021.635869
14. World Health Organization. Ending the neglect to attain the sustainable development goals – a road map for neglected tropical diseases 2021–2030. Geneva: World Health Organization (2021).
15. Deol AK, Fleming FM, Calvo-Urbano B, Walker M, Bucumi V, Gnandou I, et al. Schistosomiasis — assessing progress toward the 2020 and 2025 global goals. N Engl J Med(2019) 26:2519–28. doi: 10.1056/NEJMoa1812165
16. Mutuku M, Laidemitt M, Beechler B, Mwangi I, Otiato F, Agola E, et al. A search for snail-related answers to explain differences in response of schistosoma mansoni to praziquantel treatment among responding and persistent hotspot villages along the Kenyan shore of lake Victoria. Am J Trop Med Hyg (2019) 1:65–77. doi: 10.4269/ajtmh.19-0089
17. Assaré RK, N’Tamon RN, Bellai LG, Koffi JA, Mathieu TBI, Ouattara M, et al. Characteristics of persistent hotspots of schistosoma mansoni in western côte d’Ivoire. Parasites Vectors (2020) 13:1. doi: 10.1186/s13071-020-04188-x
18. Kittur N, King C, Campbell C, Kinung’hi S, Mwinzi P, Karanja D, et al. Persistent hotspots in schistosomiasis consortium for operational research and evaluation studies for gaining and sustaining control of schistosomiasis after four years of mass drug administration of praziquantel. Am J Trop Med Hyg (2019) 3:617–27. doi: 10.4269/ajtmh.19-0193
19. Wiegand RE, Mwinzi PNM, Montgomery SP, Chan YL, Andiego K, Omedo M, et al. A persistent hotspot of schistosoma mansoni infection in a five-year randomized trial of praziquantel preventative chemotherapy strategies. J Infect Dis (2017) 11:1425–33. doi: 10.1093/infdis/jix496
20. Haldar K, Bhattacharjee S, Safeukui I. Drug resistance in plasmodium. Nat Rev Microbiol (2018) 3:156–70. doi: 10.1038/nrmicro.2017.161
21. Kaplan R, Vidyashankar A. An inconvenient truth: global worming and anthelmintic resistance. Vet Parasitol (2012) 1–2:70–8. doi: 10.1016/j.vetpar.2011.11.048
22. Rose H, Rinaldi L, Bosco A, Mavrot F, de Waal T, Skuce P, et al. Widespread anthelmintic resistance in European farmed ruminants: a systematic review. Vet Rec (2015) 21:546. doi: 10.1136/vr.102982
23. Schwab AE, Boakye DA, Kyelem D, Prichard RK. Detection of benzimidazole resistance-associated mutations in the filarial nematode wuchereria bancrofti and evidence for selection by albendazole and ivermectin combination treatment. Am J Trop Med Hygiene (2005) 2:234–8. doi: 10.4269/ajtmh.2005.73.234
24. Messerli SM, Kasinathan RS, Morgan W, Spranger S, Greenberg RM. Schistosoma mansoni p-glycoprotein levels increase in response to praziquantel exposure and correlate with reduced praziquantel susceptibility. Mol Biochem Parasitol (2009) 1:54–9. doi: 10.1016/j.molbiopara.2009.04.007
25. Kasinathan RS, Morgan WM, Greenberg RM. Schistosoma mansoni express higher levels of multidrug resistance-associated protein 1 (SmMRP1) in juvenile worms and in response to praziquantel. Mol Biochem Parasitol (2010) 1:25–31. doi: 10.1016/j.molbiopara.2010.05.003
26. Kohn AB, Roberts-Misterly JM, Anderson PAV, Khan N, Greenberg RM. Specific sites in the beta interaction domain of a schistosome Ca 2+ channel β subunit are key to its role in sensitivity to the anti-schistosomal drug praziquantel. Parasitology (2003) 127:349–56. doi: 10.1017/S003118200300386X
27. Le Clec’h W, Chevalier FD, Mattos ACA, Strickland A, Diaz R, McDew-White M, et al. Genetic analysis of praziquantel response in schistosome parasites implicates a transient receptor potential channel. Sci Trans Med (2021) 13(625):1–3. doi: 10.1126/scitranslmed.abj9114
28. Fallon PG, Doenhoff MJ. Drug-resistant schistosomiasis: Resistance to praziquantel and oxamniquine induced in schistosoma mansoni in mice is drug specific. Am J Trop Med Hygiene (1994) 51:83–8. doi: 10.4269/ajtmh.1994.51.83
29. Couto FFB, Coelho PMZ, Araújo N, Kusel JR, Katz N, Jannotti-Passos LK, et al. Schistosoma mansoni: A method for inducing resistance to praziquantel using infected biomphalaria glabrata snails. Memorias do Instituto Oswaldo Cruz (2011) 106:153–7. doi: 10.1590/S0074-02762011000200006
30. Mwangi I, Sanchez M, Mkoji G, Agola L, Runo S, Cupit P, et al. Praziquantel sensitivity of Kenyan schistosoma mansoni isolates and the generation of a laboratory strain with reduced susceptibility to the drug. Int J Parasitol Drugs Drug Resist (2014) 3:296–300. doi: 10.1016/j.ijpddr.2014.09.006
31. Stelma FF, Talla I, Sow S, Kongs A, Niang M, Polman K, et al. Efficacy and side effects of praziquantel in an epidemic focus of schistosoma mansoni. Am J Trop Med Hygiene (1995) 2:167–70. doi: 10.4269/ajtmh.1995.53.167
32. Melman SD, Steinauer ML, Cunningham C, Kubatko LS, Mwangi IN, Wynn NB, et al. Reduced susceptibility to praziquantel among naturally occurring Kenyan isolates of schistosoma mansoni. PloS Negl Trop Dis (2009) 8:e504. doi: 10.1371/journal.pntd.0000504
33. Crellen T, Walker M, Lamberton PHL, Kabatereine NB, Tukahebwa EM, Cotton JA, et al. Reduced efficacy of praziquantel against schistosoma mansoni is associated with multiple rounds of mass drug administration. Clin Infect Dis (2016) 63:1151–9. doi: 10.1093/cid/ciw506
34. Ismail M, Metwally A, Farghaly A, Bruce J, Tao LF, Bennett JL. Characterization of isolates of schistosoma mansoni from Egyptian villagers that tolerate high doses of praziquantel. Am J Trop Med Hygiene (1996) 552:214–8. doi: 10.4269/ajtmh.1996.55.214
35. Guisse F, Polman K, Stelma FF, Mbaye A, Talla I, Niang M, et al. Therapeutic evaluation of two different dose regimens of praziquantel in a recent schistosoma mansoni focus in northern Senegal. Am J Trop Med Hygiene (1997) 5:511–4. doi: 10.4269/ajtmh.1997.56.511
36. King CH, Muchiri EM, Ouma JH. Evidence against rapid emergence of praziquantel resistance in schistosoma haematobium, Kenya. Emerging Infect Dis (2000) 6:585–94. doi: 10.3201/eid0606.000606
37. Gryseels B, Mbaye A, De Vlas SJ, Stelma FF, Guissé F, Van Lieshout L, et al. Are poor responses to praziquantel for the treatment of schistosoma mansoni infections in Senegal due to resistance? an overview of the evidence. Trop Med Int Health (2001) 6:864–73. doi: 10.1046/j.1365-3156.2001.00811.x
38. Fenwick A, Webster JP. Schistosomiasis: Challenges for control, treatment and drug resistance. vol. 19, current opinion in infectious diseases. Curr Opin Infect Dis (2006) 19:577–82. doi: 10.1097/01.qco.0000247591.13671.6a
39. Fukushige M, Chase-Topping M, Woolhouse MEJ, Mutapi F. Efficacy of praziquantel has been maintained over four decades (From 1977 to 2018): A systematic review and meta-analysis of factors influence its efficacy. PloS Negl Trop Dis (2021) 15:15(3). doi: 10.1371/journal.pntd.0009189
40. Greenberg R. New approaches for understanding mechanisms of drug resistance in schistosomes. Parasitology (2013) 12:1534–46. doi: 10.1017/S0031182013000231
41. Kokaliaris C, Garba A, Matuska M, Bronzan RN, Colley DG, Dorkenoo AM, et al. Effect of preventive chemotherapy with praziquantel on schistosomiasis among school-aged children in sub-Saharan Africa: a spatiotemporal modelling study. Lancet Infect Dis (2022) 1:136–49. doi: 10.1016/S1473-3099(21)00090-6
42. WHO guideline on control and elimination of human schistosomiasis. Geneva: World Health Organization (2022). Licence: CC BY-NC-SA 3.0 IGO.
43. Vale N, Gouveia MJ, Rinaldi G, Brindley PJ, Gärtner F, Da Costa JMC. Praziquantel for schistosomiasis: Single-drug metabolism revisited, mode of action, and resistance. Antimicrobial Agents Chemother (2017) 5:e02582–16. doi: 10.1128/AAC.02582-16
44. Olliaro P, Delgado-Romero P, Keiser J. The little we know about the pharmacokinetics and pharmacodynamics of praziquantel (racemate and r-enantiomer). J Antimicrobial Chemother (2014) 4:863–70. doi: 10.1093/jac/dkt491
45. Park SK, McCusker P, Dosa PI, Chan JD, Marchant JS. The anthelmintic drug praziquantel activates a schistosome transient receptor potential channel. J Biol Chem (2019) 294:18873–80. doi: 10.1074/jbc.AC119.011093
46. Cioli D, Pica-Mattoccia L, Basso A, Guidi A. Schistosomiasis control: Praziquantel forever? Mol Biochem Parasitol Elsevier (2014) 195:23–9. doi: 10.1016/j.molbiopara.2014.06.002
47. Harnett W, Kusel JR. Increased exposure of parasite antigens at the surface of adult male schistosoma mansoni exposed to praziquantel in vitro. Parasitology (1986) 2:401–5. doi: 10.1017/S0031182000051568
48. Pax R, Bennett JL, Fetterer R. A benzodiazepine derivative and praziquantel: Effects on musculature of schistosoma mansoni and schistosoma japonicum. Naunyn-Schmiedeberg’s Arch Pharmacol (1978) 304:309–15. doi: 10.1007/BF00507974
49. Kohn AB, Anderson PAV, Roberts-Misterly JM, Greenberg RM. Schistosome calcium channel β subunits: Unusual modulatory effects and potential role in the action of the antischistosomal drug praziquantel. J Biol Chem (2001) 276:36873–6. doi: 10.1074/jbc.C100273200
50. Pica-Mattoccia L, Valle C, Basso A, Troiani AR, Vigorosi F, Liberti P, et al. Cytochalasin d abolishes the schistosomicidal activity of praziquantel. Exp Parasitol (2007) 115:344 –351. doi: 10.1016/j.exppara.2006.09.017
51. Pica-Mattoccia L, Orsini T, Basso A, Festucci A, Liberti P, Guidi A, et al. Schistosoma mansoni: Lack of correlation between praziquantel-induced intra-worm calcium influx and parasite death. Exp Parasitol (2008) 119:332–5. doi: 10.1016/j.exppara.2008.03.012
52. Nogi T, Zhang D, Chan JD, Marchant JS. A novel biological activity of praziquantel requiring voltage-operated Ca2+ channel β subunits: Subversion of flatworm regenerative polarity. PloS Negl Trop Dis (2009) 3:e464. doi: 10.1371/journal.pntd.0000464
53. Chan JD, Agbedanu PN, Zamanian M, Gruba SM, Haynes CL, Day TA, et al. “Death and axes”: Unexpected Ca2+ entry phenologs predict new anti-schistosomal agents. PloS Pathog (2014) 10:e1003942. doi: 10.1371/journal.ppat.1003942
54. Jeziorski MC, Greenberg RM. Voltage-gated calcium channel subunits from platyhelminths: Potential role in praziquantel action. Int J Parasitol (2006) 36:625–32. doi: 10.1016/j.ijpara.2006.02.002
55. Park SK, Friedrich L, Yahya NA, Rohr CM, Chulkov EG, Maillard D, et al. Mechanism of praziquantel action at a parasitic flatworm ion channel. Sci Trans Med (2021) 13:625. doi: 10.1126/scitranslmed.abj5832
56. Babes RM, Selescu T, Domocos D, Babes A. The anthelminthic drug praziquantel is a selective agonist of the sensory transient receptor potential melastatin type 8 channel. Toxicol Appl Pharmacol (2017) 336:55–65. doi: 10.1016/j.taap.2017.10.012
57. Park SK, Marchant JS. The journey to discovering a flatworm target of praziquantel: A long TRP. Trends Parasitol (2020) 36:182–94. doi: 10.1016/j.pt.2019.11.002
58. Fetterer RH, Pax RA, Bennett JL. Praziquantel, potassium and 2,4-dinitrophenol: Analysis of their action on the musculature of schistosoma mansoni. Eur J Pharmacol (1980) 64(1):31–8. doi: 10.1016/0014-2999(80)90366-0
59. Zhang W, Chu X, Tong Q, Cheung JY, Conrad K, Masker K, et al. A novel TRPM2 isoform inhibits calcium influx and susceptibility to cell death. J Biol Chem (2003) 278:16222–9. doi: 10.1074/jbc.M300298200
60. Thomas H, Gönnert R. The efficacy of praziquantel against cestodes in animals. Z für Parasitenkunde (1977) 52:117–27. doi: 10.1007/BF00389898
61. Gnanasekar M, Salunkhe AM, Mallia AK, He YX, Kalyanasundaram R. Praziquantel affects the regulatory myosin light chain of schistosoma mansoni. Antimicrobial Agents Chemother (2009) 3:1054. doi: 10.1128/AAC.01222-08
62. McTigue MA, Williams DWR, Tainer JA. Crystal structures of a schistosomal drug and vaccine target: Glutathione s-transferase from schistosoma japonica and its complex with the leading antischistosomal drug praziquantel. J Mol Biol (1995) 246:21–7. doi: 10.1006/jmbi.1994.0061
63. Cupit P, Cunningham C. What is the mechanism of action of praziquantel and how might resistance strike? Future Med Chem (2015) 6:701–5. doi: 10.4155/fmc.15.11
64. Thomas CM, Fitzsimmons CM, Dunne DW, Timson DJ. Comparative biochemical analysis of three members of the schistosoma mansoni TAL family: Differences in ion and drug binding properties. Biochimie (2014) 108:40–7. doi: 10.1016/j.biochi.2014.10.015
65. Girardot M, Bayet E, Maurin J, Fort P, Roux P, Raynaud P. SOX9 has distinct regulatory roles in alternative splicing and transcription. Nucleic Acids Res (2018) 17:9106–18. doi: 10.1093/nar/gky553
66. Berger DJ, Crellen T, Lamberton PHL, Allan F, Tracey A, Noonan JD, et al. Whole-genome sequencing of schistosoma mansoni reveals extensive diversity with limited selection despite mass drug administration. Nat Commun (2021) 12:1–14. doi: 10.1038/s41467-021-24958-0
67. Cotton JA, Doyle SR. A genetic TRP down the channel to praziquantel resistance. Trends Parasitol (2022) 38:351–52. doi: 10.1016/j.pt.2022.02.006
68. Lespine A, Alvinerie M, Vercruysse J, Prichard RK, Geldhof P. ABC Transporter modulation: a strategy to enhance the activity of macrocyclic lactone anthelmintics. Trends Parasitol (2008) 24:293–8. doi: 10.1016/j.pt.2008.03.011
69. Bosch IB, Wang ZX, Tao LF, Shoemaker CB. Two schistosoma mansoni cDNAs encoding ATP-binding cassette (ABC) family proteins. Mol Biochem Parasitol (1994) 65:351–56. doi: 10.1016/0166-6851(94)90085-X
70. Lespine A, Ménez C, Bourguinat C, Prichard R. P-glycoproteins and other multidrug resistance transporters in the pharmacology of anthelmintics: Prospects for reversing transport-dependent anthelmintic resistance. Int J Parasitol Drugs Drug Resist (2011) 2:58–75. doi: 10.1016/j.ijpddr.2011.10.001
71. James C, Hudson A, Davey M. Drug resistance mechanisms in helminths: is it survival of the fittest? Trends Parasitol (2009) 7:328–35. doi: 10.1016/j.pt.2009.04.004
72. Kerboeuf D, Blackhall W, Kaminsky R, von Samson-Himmelstjerna G. P-glycoprotein in helminths: function and perspectives for anthelmintic treatment and reversal of resistance. Int J Antimicrob Agents (2003) 3:332–46. doi: 10.1016/S0924-8579(03)00221-8
73. Kasinathan RS, Greenberg RM. Pharmacology and potential physiological significance of schistosome multidrug resistance transporters. Exp Parasitol (2012) 1:2–6. doi: 10.1016/j.exppara.2011.03.004
74. Greenberg RM. ABC Multidrug transporters in schistosomes and other parasitic flatworms. Parasitol Int (2013) 6:647. doi: 10.1016/j.parint.2013.02.006
75. Pinto-Almeida A, Mendes T, Armada A, Belo S, Carrilho E, Viveiros M, et al. The role of efflux pumps in schistosoma mansoni praziquantel resistant phenotype. PloS One (2015) 10:e0140147. doi: 10.1371/journal.pone.0140147
76. Kasinathan RS, Sharma LK, Cunningham C, Webb TR, Greenberg RM. Inhibition or knockdown of ABC transporters enhances susceptibility of adult and juvenile schistosomes to praziquantel. PloS Negl Trop Dis (2014) 8:e3265. doi: 10.1371/journal.pntd.0003265
77. James CE, Hudson AL, Davey MW. An update on p-glycoprotein and drug resistance in schistosoma mansoni. Trends Parasitol (2009) 25:538–9. doi: 10.1016/j.pt.2009.09.007
78. Kasinathan RS, Goronga T, Messerli SM, Webb TR, Greenberg RM. Modulation of a schistosoma mansoni multidrug transporter by the antischistosomal drug praziquantel. FASEB J (2010) 24:128–35. doi: 10.1096/fj.09-137091
79. Liang YS, Wang W, Dai JR, Li HJ, Tao YH, Zhang JF, et al. Susceptibility to praziquantel of male and female cercariae of praziquantel-resistant and susceptible isolates of schistosoma mansoni. J Helminthol (2010) 84:202–7. doi: 10.1017/S0022149X0999054X
80. Hines-Kay J, Cupit PM, Sanchez MC, Rosenberg GH, Hanelt B, Cunningham C. Transcriptional analysis of schistosoma mansoni treated with praziquantel in vitro. Mol Biochem Parasitol (2012) 186:87–94. doi: 10.1016/j.molbiopara.2012.09.006
81. Sato H, Kusel J, Thornhill J. Excretion of fluorescent substrates of mammalian multidrug resistance-associated protein (MRP) in the schistosoma mansoni excretory system. Parasitology (2004) 128:43–52. doi: 10.1017/S0031182003004177
82. Sato H, Kusel JR, Thornhill J. Functional visualization of the excretory system of adult schistosoma mansoni by the fluorescent marker resorufin. Parasitology (2002) 6:527–35. doi: 10.1017/S0031182002002536
83. Kusel J, Oliveira F, Todd M, Ronketti F, Lima S, Mattos A, et al. The effects of drugs, ions, and poly-l-lysine on the excretory system of schistosoma mansoni. Memorias do Instituto Oswaldo Cruz (2006) 101:293–8. doi: 10.1590/S0074-02762006000900046
84. Oliveira F, Kusel J, Ribeiro F, Coelho P. Responses of the surface membrane and excretory system of schistosoma mansoni to damage and to treatment with praziquantel and other biomolecules. Parasitology (2006) 132:321–30. doi: 10.1017/S0031182005009169
85. Couto F, Coelho P, Araújo N, Kusel J, Katz N, Mattos A. Use of fluorescent probes as a useful tool to identify resistant schistosoma mansoni isolates to praziquantel. Parasitology (2010) 12:1791–7. doi: 10.1017/S003118201000065X
86. Kohn AB, Anderson PAV, Roberts-Misterly JM, Greenberg RM. Schistosome calcium channel beta subunits. unusual modulatory effects and potential role in the action of the antischistosomal drug praziquantel. J Biol Chem (2001) 40:36873–6. doi: 10.1074/jbc.C100273200
87. Valle C, Troiani AR, Festucci A, Pica-Mattoccia L, Liberti P, Wolstenholme A, et al. Sequence and level of endogenous expression of calcium channel β subunits in schistosoma mansoni displaying different susceptibilities to praziquantel. Mol Biochem Parasitol (2003) 130:111–5. doi: 10.1016/S0166-6851(03)00171-3
88. Chen YH, Li MH, Zhang Y, He LL, Yamada Y, Fitzmaurice A, et al. Structural basis of the α1–β subunit interaction of voltage-gated Ca2+ channels. Nature (2004) 6992:675–80. doi: 10.1038/nature02641
89. Salvador-Recatalà V, Greenberg RM. Calcium channels of schistosomes: Unresolved questions and unexpected answers. Wiley Interdiscip Rev: Membrane Transport Signalling (2012) 1:85–93. doi: 10.1002/wmts.19
90. Harder A. Activation of transient receptor potential channel Sm.(Schistosoma mansoni)TRPM PZQ by PZQ, enhanced Ca ++ influx, spastic paralysis, and tegumental disrupture-the deadly cascade in parasitic schistosomes, other trematodes, and cestodes. Parasitol Res (2020) 119:2371–82. doi: 10.1007/s00436-020-06763-8
91. Fallon PG. Schistosome resistance to praziquantel. Drug Resistance Updates (1998) 1:236–41. doi: 10.1016/S1368-7646(98)80004-6
92. Van Wyk JA. Refugia - overlooked as perhaps the most potent factor concerning the development of anthelmintic resistance. Onderstepoort J Veterinary Res (2001) 68:55–67.
93. Le Clec’h W, Chevalier FD, McDew-White M, Allan F, Webster BL, Gouvras AN, et al. Whole genome amplification and exome sequencing of archived schistosome miracidia. Parasitology (2018) 13:1739. doi: 10.1017/S0031182018000811
94. Lamberton PHL, Hogan SC, Kabatereine NB, Fenwick A, Webster JP. In vitro praziquantel test capable of detecting reduced in vivo efficacy in schistosoma mansoni human infections. Am J Trop Med Hygiene (2010) 6:1340–7. doi: 10.4269/ajtmh.2010.10-0413
95. Hagan P, Appleton CC, Coles GC, Kusel JR, Tchuem-Tchuenté LA. Schistosomiasis control: Keep taking the tablets. Trends Parasitol Elsevier Ltd (2004) 20:92–7. doi: 10.1016/j.pt.2003.11.010
96. Botros S, Sayed H, Amer N, El-Ghannam M, Bennett JL, Day TA. Current status of sensitivity to praziquantel in a focus of potential drug resistance in Egypt. Int J Parasitol (2005) 35:787–91. doi: 10.1016/j.ijpara.2005.02.005
97. Norton AJ, Gower CM, Lamberton PHL, Webster BL, Lwambo NJS, Blair L, et al. Genetic consequences of mass human chemotherapy for schistosoma mansoni: Population structure pre-and post-praziquantel treatment in Tanzania. Am J Trop Med Hygiene (2010) 4:951–7. doi: 10.4269/ajtmh.2010.10-0283
98. Doenhoff MJ, Pica-Mattoccia L. Praziquantel for the treatment of schistosomiasis: Its use for control in areas with endemic disease and prospects for drug resistance. Expert Rev Anti-Infective Ther (2006) 4:199–210. doi: 10.1586/14787210.4.2.199
99. Danso-Appiah A, De Vlas SJ. Interpreting low praziquantel cure rates of schistosoma mansoni infections in Senegal. Trends Parasitol (2002) 18:125–9. doi: 10.1016/S1471-4922(01)02209-7
100. Fallon PG, Mubarak JS, Fookes RE, Niang M, Butterworth AE, Sturrock RF, et al. Schistosoma mansoni: Maturation rate and drug susceptibility of different geographic isolates. Exp Parasitol (1997) 1:29–36. doi: 10.1006/expr.1997.4149
101. Fallon PG, Sturrock RF, Capron A, Niang M, Doenhoff MJ. Short report: Diminished susceptibility to praziquantel in a Senegal isolate of schistosoma mansoni. Am J Trop Med Hygiene (1995) 1:61–2. doi: 10.4269/ajtmh.1995.53.61
102. Liang YS, Coles GC, Doenhoff MJ, Southgate VR. In vitro responses of praziquantel-resistant and -susceptible schistosoma mansoni to praziquantel. Int J Parasitol (2001) 11:1227–35. doi: 10.1016/S0020-7519(01)00246-6
103. Gower C, Shrivastava J, Lamberton P, Rollinson D, Webster B, Emery A, et al. Development and application of an ethically and epidemiologically advantageous assay for the multi-locus microsatellite analysis of schistosoma mansoni. Parasitology (2007) 134:523–36. doi: 10.1017/S0031182006001685
104. Stelma FF, Sall S, Daff B, Sow S, Niang M, Gryseels B. Oxamniquine cures schistosoma mansoni infection in a focus in which cure rates with praziquantel are unusually low. J Infect Dis (1997) 1:304–7. doi: 10.1086/517273
105. Doenhoff M, Cioli D, Kimani G. Praziquantel and the control of schistosomiasis. Parasitol Today (2000) 16:364–6. doi: 10.1016/S0169-4758(00)01749-X
106. Castro N, Medina R, Sotelo J, Jung H. Bioavailability of praziquantel increases with concomitant administration of food. Antimicrobial Agents Chemother (2000) 10:2903–4. doi: 10.1128/AAC.44.10.2903-2904.2000
107. Ismail M, Botros S, Metwally A, William S, Farghally A, Tao LF, et al. Resistance to praziquantel: Direct evidence from schistosoma mansoni isolated from egyptian villagers. Am J Trop Med Hygiene (1999) 6:932–5. doi: 10.4269/ajtmh.1999.60.932
108. Sabra ANA, Botros SS. Response of schistosoma mansoni isolates having different drug sensitivity to praziquantel over several life cycle passages with and without therapeutic pressure. J Parasitol (2008) 2:537–41. doi: 10.1645/GE-1297.1
109. Stothard JR, Sousa-Figueiredo JC, Betson M, Bustinduy A, Reinhard-Rupp J. Schistosomiasis in African infants and preschool children: let them now be treated! Trends Parasitol (2013) 4:197. doi: 10.1016/j.pt.2013.02.001
110. Bustinduy AL, Waterhouse D, de Sousa-Figueiredo JC, Roberts SA, Atuhaire A, van Dam GJ, et al. Population pharmacokinetics and pharmacodynamics of praziquantel in Ugandan children with intestinal schistosomiasis: Higher dosages are required for maximal efficacy. mBio (2016) 4:1–9. doi: 10.1128/mBio.00227-16
111. Sousa-Figueiredo JC, Betson M, Atuhaire A, Arinaitwe M, Navaratnam AMD, Kabatereine NB, et al. Performance and safety of praziquantel for treatment of intestinal schistosomiasis in infants and preschool children. PloS Negl Trop Dis (2012) 10:e1864. doi: 10.1371/journal.pntd.0001864
112. Morgan JAT, Dejong RJ, Adeoye GO, Ansa EDO, Barbosa CÇS, Brémond P, et al. Origin and diversification of the human parasite schistosoma mansoni. Mol Ecol (2005) 14:3889–902. doi: 10.1111/j.1365-294X.2005.02709.x
113. Thiele EA, Sorensen RE, Gazzinelli A, Minchella DJ. Genetic diversity and population structuring of schistosoma mansoni in a Brazilian village. Int J Parasitol (2008) 3–4:389–99. doi: 10.1016/j.ijpara.2007.07.011
114. Stothard JR, Webster BL, Weber T, Nyakaana S, Webster JP, Kazibwe F, et al. Molecular epidemiology of schistosoma mansoni in Uganda: DNA barcoding reveals substantial genetic diversity within lake Albert and lake Victoria populations. Parasitology (2009) 13:1813–24. doi: 10.1017/S003118200999031X
115. van den Broeck F, Maes GE, Larmuseau MHD, Rollinson D, Sy I, Faye D, et al. Reconstructing colonization dynamics of the human parasite schistosoma mansoni following anthropogenic environmental changes in Northwest Senegal. PloS Negl Trop Dis (2015) 8:e0003998. doi: 10.1371/journal.pntd.0003998
116. Faust CL, Crotti M, Moses A, Oguttu D, Wamboko A, Adriko M, et al. Two-year longitudinal survey reveals high genetic diversity of schistosoma mansoni with adult worms surviving praziquantel treatment at the start of mass drug administration in Uganda. Parasites Vectors (2019) 1:1–12. doi: 10.1186/s13071-019-3860-6
117. Rey O, Webster BL, Huyse T, Rollinson D, Van den Broeck F, Kincaid-Smith J, et al. Population genetics of African schistosoma species. Infect Genet Evol (2021) 89:104727. doi: 10.1016/j.meegid.2021.104727
118. Lelo AE, Mburu DN, Magoma GN, Mungai BN, Kihara JH, Mwangi IN, et al. No apparent reduction in schistosome burden or genetic diversity following four years of school-based mass drug administration in mwea, central kenya, a heavy transmission area. PloS Negl Trop Dis (2014) 10:e3221–1. doi: 10.1371/journal.pntd.0003221
119. Vianney TJ, Berger DJ, Doyle SR, Sankaranarayanan G, Serubanja J, Nakawungu PK, et al. Genome-wide analysis of schistosoma mansoni reveals population structure and praziquantel drug selection pressure within Ugandan hot-spot communities. bioRxiv (2022). doi: 10.1101/2022.01.25.477652
120. Trienekens SCM, Faust CL, Meginnis K, Pickering L, Ericssonid O, Nankasi A, et al. Impacts of host gender on schistosoma mansoni risk in rural Uganda-a mixed-methods approach. PloS Negl Trop Dis (2020) 5:1–19. doi: 10.1371/journal.pntd.0008266
121. Barbosa LM, Reis EA, dos Santos CRA, Costa JM, Carmo TM, Aminu PT, et al. Repeated praziquantel treatments remodel the genetic and spatial landscape of schistosomiasis risk and transmission. Int J Parasitol (2016) 5–6:343–50. doi: 10.1016/j.ijpara.2016.01.007
122. Blanton RE, Blank WA, Costa JM, Carmo TM, Reis EA, Silva LK, et al. Schistosoma mansoni population structure and persistence after praziquantel treatment in two villages of bahia, Brazil. Int J Parasitol (2011) 10:1093. doi: 10.1016/j.ijpara.2011.06.002
123. Theron A, Sire C, Rognon A, Prugnolle F, Durand P. Molecular ecology of schistosoma mansoni transmission inferred from the genetic composition of larval and adult infrapopulations within intermediate and definitive hosts. Parasitology (2004) 129:571–85. doi: 10.1017/S0031182004005943
124. Curtis J, Minchella DJ. Schistosome population genetic structure: When clumping worms is not just splitting hairs. Parasitol Today (2000) 2:68–71. doi: 10.1016/S0169-4758(99)01553-7
125. Gower CM, Gouvras AN, Lamberton PH, Deol A, Shrivastava J, Mutombo PN, et al. Population genetic structure of schistosoma mansoni and schistosoma haematobium from across six sub-Saharan African countries: Implications for epidemiology, evolution and control. Acta Tropica (2013) 2:261–74. doi: 10.1016/j.actatropica.2012.09.014
126. Webster B, Webster J, Gouvras A, Garba A, Lamine M, Diaw O, et al. DNA “barcoding” of schistosoma mansoni across sub-Saharan Africa supports substantial within locality diversity and geographical separation of genotypes. Acta Trop (2013) 2:250–60. doi: 10.1016/j.actatropica.2012.08.009
127. Betson M, Sousa-Figueiredo JC, Kabatereine NB, Stothard JR. New insights into the molecular epidemiology and population genetics of schistosoma mansoni in Ugandan pre-school children and mothers. PloS Negl Trop Dis (2013) 12:e2561. doi: 10.1371/journal.pntd.0002561
128. Guzman MA, Rugel AR, Tarpley RS, Alwan SN, Chevalier FD, Kovalskyy DP, et al. An iterative process produces oxamniquine derivatives that kill the major species of schistosomes infecting humans. PloS Negl Trop Dis (2020) 8:e0008517. doi: 10.1371/journal.pntd.0008517
129. Crellen T, Allan F, David S, Durrant C, Huckvale T, Holroyd N, et al. Whole genome resequencing of the human parasite schistosoma mansoni reveals population history and effects of selection. Sci Rep (2016) 1:1–13. doi: 10.1038/srep20954
130. Gower CM, Gehre F, Marques SR, Lamberton PHL, Lwambo NJ, Webster JP. Phenotypic and genotypic monitoring of schistosoma mansoni in Tanzanian schoolchildren five years into a preventative chemotherapy national control programme. Parasites Vectors (2017) 1:593. doi: 10.1186/s13071-017-2533-6
131. Huyse T, Van den Broeck F, Jombart T, Webster BL, Diaw O, Volckaert FAM, et al. Regular treatments of praziquantel do not impact on the genetic make-up of schistosoma mansoni in northern Senegal. Infect Genet Evol (2013) 18:100–5. doi: 10.1016/j.meegid.2013.05.007
132. Huyse T, Webster BL, Geldof S, Stothard JR, Diaw OT, Polman K, et al. Bidirectional introgressive hybridization between a cattle and human schistosome species. PloS Pathog (2009) 9:e1000571. doi: 10.1371/journal.ppat.1000571
133. Chen R, Wang J, Gradinaru I, Deberardinis RJ, Ross EM, Collins JJ, et al. A male-derived nonribosomal peptide pheromone controls female schistosome development. Cell (2022) 185:1506–1520.e17. doi: 10.1016/j.cell.2022.03.017
134. Loker ES, Brant SV. Diversification, dioecy and dimorphism in schistosomes. Trends Parasitol (2006) 11:521–8. doi: 10.1016/j.pt.2006.09.001
135. Leger E, Webster JP. Hybridizations within the genus schistosoma: Implications for evolution, epidemiology and control. Parasitology (2017) 1:65–80. doi: 10.1017/S0031182016001190
136. Sanchez MC, Cupit PM, Bu L, Cunningham C. Transcriptomic analysis of reduced sensitivity to praziquantel in schistosoma mansoni. Mol Biochem Parasitol (2019) 228:6. doi: 10.1016/j.molbiopara.2018.12.005
137. Lu DB, Deng Y, Ding H, Liang YS, Webster JP. Single-sex schistosome infections of definitive hosts: Implications for epidemiology and disease control in a changing world. PloS Pathog (2018) 3:e1006817. doi: 10.1371/journal.ppat.1006817
138. Lamberton PHL, Faust CL, Webster JP. Praziquantel decreases fecundity in schistosoma mansoni adult worms that survive treatment: evidence from a laboratory life-history trade-offs selection study. Infect Dis Poverty (2017) 6:1–11. doi: 10.1186/s40249-017-0324-0
139. Steinauer ML. The sex lives of parasites: investigating the mating system and mechanisms of sexual selection of the human pathogen schistosoma mansoni. Int J Parasitol (2009) 10:1157–63. doi: 10.1016/j.ijpara.2009.02.019
140. Chevalier F, le Clec’h W, McDew-White M, Menon V, Guzman M, Holloway S, et al. Oxamniquine resistance alleles are widespread in old world schistosoma mansoni and predate drug deployment. PloS Pathog (2019) 10:1–25. doi: 10.1371/journal.ppat.1007881
141. Orr HA, Betancourt AJ. Haldane’s sieve and adaptation from the standing genetic variation. Genetics (2001) 2:875–84. doi: 10.1093/genetics/157.2.875
142. Barrett RDH, Schluter D. Adaptation from standing genetic variation. Trends Ecol Evol (2008) 1:38–44. doi: 10.1016/j.tree.2007.09.008
143. Hermisson J, Pennings PS. Soft sweeps: molecular population genetics of adaptation from standing genetic variation. Genetics (2005) 4:2335–52. doi: 10.1534/genetics.104.036947
144. Coles GC, Mutahi WT, Kinoti GK, Bruce JI, Katz N. Tolerance of Kenyan schistosoma mansoni to oxamniquine. Trans R Soc Trop Med Hyg (1987) 5:782–5. doi: 10.1016/0035-9203(87)90032-0
145. Lewis FA, Stirewalt MA, Souza CP, Gazzinelli G. Large-Scale laboratory maintenance of schistosoma mansoni, with observations on three schistosome/snail host combinations. J Parasitol (1986) 6:813–29. doi: 10.2307/3281829
146. Cioli D, Pica-Mattoccia L, Moroni R. Schistosoma mansoni: hycanthone/oxamniquine resistance is controlled by a single autosomal recessive gene. Exp Parasitol (1992) 4:425–32. doi: 10.1016/0014-4894(92)90255-9
147. el Guiniady MA, el Touny MA, Abdel-Bary MA, Abdel-Fatah SA, Metwally A. Clinical and pharmacokinetic study of praziquantel in Egyptian schistosomiasis patients with and without liver cell failure. Am J Trop Med Hygiene (1994) 6:809–18. doi: 10.4269/ajtmh.1994.51.809
148. Webb E, Edielu A, Wu H, Kabatereine N, Tukahebwa E, Mubangizi A, et al. The praziquantel in preschoolers (PIP) trial: study protocol for a phase II PK/PD-driven randomised controlled trial of praziquantel in children under 4 years of age. Trials (2021) 1:601. doi: 10.1186/s13063-021-05558-1
149. Kovač J, Vargas M, Keiser J. In vitro and in vivo activity of r- and s- praziquantel enantiomers and the main human metabolite trans-4-hydroxy-praziquantel against schistosoma haematobium. Parasite Vectors (2017) 10:365. doi: 10.1186/s13071-017-2293-3
150. Chan JD, Cupit PM, Gunaratne GS, McCorvy JD, Yang Y, Stoltz K, et al. The anthelmintic praziquantel is a human serotoninergic G-protein-coupled receptor ligand. Nat Commun (2017) 8:1–7. doi: 10.1038/s41467-017-02084-0
151. Gunaratne GS, Yahya NA, Dosa PI, Marchant JS. Activation of host transient receptor potential (TRP) channels by praziquantel stereoisomers. PloS Negl Trop Dis (2018) 12(4):1–18. doi: 10.1371/journal.pntd.0006420
152. Evans WE, Relling Mv. Pharmacogenomics: Translating functional genomics into rational therapeutics. Science (1999) 5439:487–91. doi: 10.1126/science.286.5439.487
153. Kovač J, Meister I, Neodo A, Panic G, Coulibaly JT, Falcoz C, et al. Pharmacokinetics of praziquantel in schistosoma mansoni- and schistosoma haematobium-infected school- and preschool-aged children. Antimicrobial Agents Chemother (2018) 8:1–11. doi: 10.1128/AAC.02253-17
154. Hodgkinson JE, Kaplan RM, Kenyon F, Morgan ER, Park AW, Paterson S, et al. Refugia and anthelmintic resistance: Concepts and challenges. Int J Parasitol: Drugs Drug Resistance (2019) 10:51–7. doi: 10.1016/j.ijpddr.2019.05.001
155. Enk MJ, Oliveira e Silva G, Rodrigues NB. Diagnostic accuracy and applicability of a PCR system for the detection of schistosoma mansoni DNA in human urine samples from an endemic area. PloS One (2012) 6:38947. doi: 10.1371/journal.pone.0038947
156. WHO. Schistosomiasis and soil-transmitted helminthiases: progress report. Weekly epidemiological record (2020) 48:1–12.
157. Cavalcanti MG, Peralta JM. Investment on new technologies for diagnosis of schistosomiasis is justified in the era of schistosoma elimination? Contagion (2018) 3:1.
158. Walker M, Mabud TS, Olliaro PL, Coulibaly JT, King CH, Raso G, et al. New approaches to measuring anthelminthic drug efficacy: parasitological responses of childhood schistosome infections to treatment with praziquantel. Parasites Vectors (2016) 1:1–15. doi: 10.1186/s13071-016-1312-0
159. Ajibola O, Gulumbe B, Eze A, Obishakin E. Tools for detection of schistosomiasis in resource limited settings. Med Sci (2018) 6(2):39. doi: 10.3390/medsci6020039
160. Cavalcanti MG, Silva LF, Peralta RHS, Barreto MGM, Peralta JM. Schistosomiasis in areas of low endemicity: A new era in diagnosis. Trends Parasitol (2013) 29:75–82. doi: 10.1016/j.pt.2012.11.003
161. Ibironke OA, Phillips AE, Garba A, Lamine SM, Shiff C. Diagnosis of schistosoma haematobium by detection of specific DNA fragments from filtered urine samples. Am J Trop Med Hygiene (2011) 84(6):998–1001. doi: 10.4269/ajtmh.2011.10-0691
162. Lamberton PHL, Kabatereine NB, Oguttu DW, Fenwick A, Webster JP. Sensitivity and specificity of multiple kato-Katz thick smears and a circulating cathodic antigen test for schistosoma mansoni diagnosis pre- and post-repeated-Praziquantel treatment. PloS Negl Trop Dis (2014) 8(9):1–15. doi: 10.1371/journal.pntd.0003139
163. Kongs A, Marks G, Verle P, van der Stuyft P. The unreliability of the kato-Katz technique limits its usefulness for evaluating s. mansoni infections. Trop Med Int Health (2001) 6(3):163–9. doi: 10.1046/j.1365-3156.2001.00687.x
164. Vinkeles Melchers NVS, van Dam GJ, Shaproski D, Kahama AI, Brienen EAT, Vennervald BJ, et al. Diagnostic performance of schistosoma real-time PCR in urine samples from Kenyan children infected with schistosoma haematobium: Day-to-day variation and follow-up after praziquantel treatment. PloS Negl Trop Dis (2014) 8(4):1–9. doi: 10.1371/journal.pntd.0002807
165. Cavalcanti MG, Cunha AFA, Peralta JM. The advances in molecular and new point-of-Care (POC) diagnosis of schistosomiasis pre- and post-praziquantel use: In the pursuit of more reliable approaches for low endemic and non-endemic areas. Front Immunol (2019) 1:858. doi: 10.3389/fimmu.2019.00858
166. Kittur N, Castleman JD, Campbell CH Jr., King CH, Colley DG. Comparison of schistosoma mansoni prevalence and intensity of infection, as determined by the circulating cathodic antigen urine assay or by the kato-Katz fecal assay: A systematic review. Am J Trop Med Hygiene (2016) 94(3):605. doi: 10.4269/ajtmh.15-0725
167. Corstjens PLAM, de Dood CJ, Knopp S, Clements MN, Ortu G, Umulisa I, et al. Circulating anodic antigen (CAA): A highly sensitive diagnostic biomarker to detect active schistosoma infections–improvement and use during SCORE. Am J Trop Med Hygiene (2020) 103:50. doi: 10.4269/ajtmh.19-0819
168. Hoekstra Id PT, Casacuberta-Partal M, Van Lieshout L, Corstjens PLAM, Tsonaka R, Assaré RK, et al. Efficacy of single versus four repeated doses of praziquantel against schistosoma mansoni infection in school-aged children from côte d’Ivoire based on kato-Katz and POC-CCA: An open-label, randomised controlled trial (RePST). PloS Neglected Tropical Diseases (2020) 14:e0008189. doi: 10.1371/journal.pntd.0008189
169. Casacuberta-Partal M, Beenakker M, de Dood CJ, Hoekstra PT, Kroon L, Kornelis D, et al. Specificity of the point-of-Care urine strip test for schistosoma circulating cathodic antigen (POC-CCA) tested in non-endemic pregnant women and young children. Am J Trop Med Hygiene (2021) 104(4):1412–7. doi: 10.4269/ajtmh.20-1168
170. RMD. Technical brochure–rapid test for qualitative detection of: Bilharzia (Schistosomiasis). (2018).
171. Utzinger J, Booth M, N’Goran EK, Müller I, Tanner M, Lengeler C. Relative contribution of day-to-day and intra-specimen variation in faecal egg counts of schistosoma mansoni before and after treatment with praziquantel. Parasitology (2001) 122(5):537–44. doi: 10.1017/S0031182001007752
172. Engels D, Sinzinkayo E, Gryseels B. Day-to-day egg count fluctuation in schistosoma mansoni infection and its operational implications. Am J Trop Med Hygiene (1996) 54(4):319–24. doi: 10.4269/ajtmh.1996.54.319
173. Colley DG, Andros TS, Campbell CH. Schistosomiasis is more prevalent than previously thought: What does it mean for public health goals, policies, strategies, guidelines and intervention programs? Infect Dis Poverty (2017) 6:1–8. doi: 10.1186/s40249-017-0275-5
174. Haggag AA, Rabiee A, Abd Elaziz KM, Campbell CH, Colley DG, Ramzy RMR. Thirty-day daily comparisons of kato–Katz and CCA assays of 45 Egyptian children in areas with very low prevalence of schistosoma mansoni. Am J Trop Med Hygiene (2019) 100(3):578–83. doi: 10.4269/ajtmh.18-0829
175. Xiao SH, Catto BA, Webster LT. Effects of praziquantel on different developmental stages of schistosoma mansoni in vitro and in vivo. J Infect Dis (1985) 151):1130 –1137. doi: 10.1093/infdis/151.6.1130
176. Pica-Mattoccia L, Cioli D. Sex- and stage-related sensitivity of schistosoma mansoni to in vivo and in vitro praziquantel treatment. Int J Parasitol (2004) 34):527–33. doi: 10.1016/j.ijpara.2003.12.003
178. Nsanzabana C, Djalle D, Guérin P, Ménard D, González I. Tools for surveillance of anti-malarial drug resistance: an assessment of the current landscape. Malar (2018) 1:1–16. doi: 10.1186/s12936-018-2185-9
179. White NJ. Assessment of the pharmacodynamic properties of antimalarial drugs in vivo. Antimicrobial Agents Chemother (1997) 7:1413. doi: 10.1128/AAC.41.7.1413
180. Botros S, El-Lakkany N, Seif el-Din SH, Sabra AN, Ibrahim M. Comparative efficacy and bioavailability of different praziquantel brands. Exp Parasitol (2011) 2:515–21. doi: 10.1016/j.exppara.2010.10.019
181. Zdesenko G, Mutapi F. Drug metabolism and pharmacokinetics of praziquantel: A review of variable drug exposure during schistosomiasis treatment in human hosts and experimental models. PloS Negl Trop Dis (2020) 9:1–26. doi: 10.1371/journal.pntd.0008649
182. Lima RM, Ferreira MAD, de Jesus Ponte Carvalho TM, Dumêt Fernandes BJ, Takayanagui OM, Garcia HH, et al. Albendazole-praziquantel interaction in healthy volunteers: kinetic disposition, metabolism and enantioselectivity. Br J Clin Pharmacol (2011) 4:528. doi: 10.1111/j.1365-2125.2010.03874.x
183. Wang H, Fang ZZ, Zheng Y, Zhou K, Hu C, Krausz KW, et al. Metabolic profiling of praziquantel enantiomers. Biochem Pharmacol (2014) 2:166–78. doi: 10.1016/j.bcp.2014.05.001
184. Li HJ, Liang YS, Dai JR, Wang W, Qu GL, Li YZ, et al. Studies on resistance of schistosoma to praziquantel XIV experimental comparison of susceptibility to praziquantel between PZQ-resistant isolates and PZQ-susceptible isolates of schistosoma japonicum in stages of adult worms, miracidia and cercariae. Chin J Schistosomiasis Control (2011) 23:611–9. doi: 10.16250/j.32.1374.2017101
185. Seto EYW, Wong BK, Lu D, Zhong B. Human schistosomiasis resistance to praziquantel in China: Should we be worried? Am J Trop Med Hygiene (2011) 1:74–82. doi: 10.4269/ajtmh.2011.10-0542
186. Wang W, Dai JR, Li HJ, Shen XH, Liang YS. Is there reduced susceptibility to praziquantel in schistosoma japonicum? evidence from China. Parasitology (2010) 137:1906–12. doi: 10.1017/S0031182010001204
187. Wang W, Dai JR, Li HJ, Shen XH, Liang YS. Short report: The sensitivity of schistosoma japonicum to praziquantel: A field evaluation in areas with low endemicity of China. Am J Trop Med Hygiene (2012) 5:834–6. doi: 10.4269/ajtmh.2012.11-0701
188. Kwa MSG, Veenstra JG, Roos MH. Benzimidazole resistance in haemonchus contortus is correlated with a conserved mutation at amino acid 200 in β-tubulin isotype 1. Mol Biochem Parasitol (1994) 63:299–303. doi: 10.1016/0166-6851(94)90066-3
Keywords: preventive chemotherapy, praziquantel, drug resistance, drug tolerance, blood flukes, schistosomes, Schistosoma mansoni
Citation: Summers S, Bhattacharyya T, Allan F, Stothard JR, Edielu A, Webster BL, Miles MA and Bustinduy AL (2022) A review of the genetic determinants of praziquantel resistance in Schistosoma mansoni: Is praziquantel and intestinal schistosomiasis a perfect match? Front. Trop. Dis. 3:933097. doi: 10.3389/fitd.2022.933097
Received: 30 April 2022; Accepted: 01 July 2022;
Published: 22 August 2022.
Edited by:
Stephen R. Doyle, Wellcome Sanger Institute (WT), United KingdomReviewed by:
John D. Chan, University of Wisconsin–Oshkosh, United KingdomJose Ma. Moncada Angeles, University of the Philippines Manila, Philippines
Copyright © 2022 Summers, Bhattacharyya, Allan, Stothard, Edielu, Webster, Miles and Bustinduy. This is an open-access article distributed under the terms of the Creative Commons Attribution License (CC BY). The use, distribution or reproduction in other forums is permitted, provided the original author(s) and the copyright owner(s) are credited and that the original publication in this journal is cited, in accordance with accepted academic practice. No use, distribution or reproduction is permitted which does not comply with these terms.
*Correspondence: Shannan Summers, U2hhbm5hbi5zdW1tZXJzMUBsc2h0bS5hYy51aw==