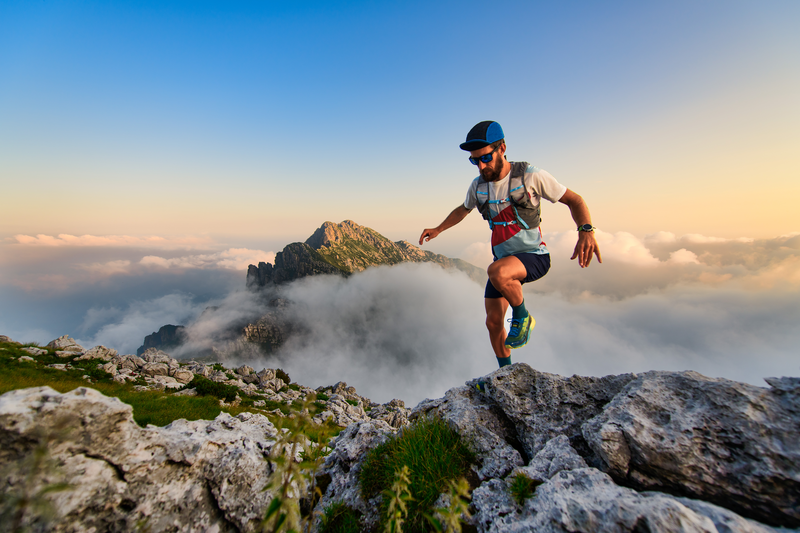
95% of researchers rate our articles as excellent or good
Learn more about the work of our research integrity team to safeguard the quality of each article we publish.
Find out more
ORIGINAL RESEARCH article
Front. Trop. Dis. , 14 September 2022
Sec. Vector Biology
Volume 3 - 2022 | https://doi.org/10.3389/fitd.2022.920224
This article is part of the Research Topic Mosquitoes and Their Role in Disease Transmission: Recent Advances and Innovations View all 9 articles
In 2016, an entomological study was carried out in a railway transect between Banfora and Ouagadougou, Burkina Faso. The objective was to assess the risk factors of arbovirus outbreaks, including vector-borne infection status within representative regions of the country. Aedes aegypti mosquitoes were collected at the larval stage from their natural rearing habitats in four study sites when estimating the main larval index, then reared until adult stage and kept in RNAlater for the detection of arbovirus RNA. In the laboratory, mosquito samples were tested for dengue virus (DENV) and Chikungunya virus (CHIKV) using a real-time qRT-PCR stage. A DENV-2 positive pool was detected in Ouagadougou with a minimum infection rate (MIR) of 16.67 and other six CHIKV-positive pools with a MIR of 66.67 in Ouagadougou, Banfora, and Boromo. This qRT-PCR approach, if validated with various samples also comprising wild blood-fed adults, is a useful tool for arbovirus circulation and disease monitoring in Burkina Faso.
Arboviruses are a major public health concern worldwide and have been a source of many outbreaks and epidemics. Over the past few years, some arboviruses like yellow fever virus (YFV), dengue virus (DENV), Chikungunya virus (CHIKV), and Zika virus (ZIKV) have rapidly expanded globally (1, 2). CHIKV is now disseminated all over the world due to the prevalence of vectors and their effectiveness in the transmission of the virus. Another potential reason for the spread of CHIKV is displacement (3). The epidemiological pattern of CHIKV includes sporadic and epidemic cases in West Africa, from Cameroon to Senegal, as well as in several other African countries (Central African Republic, South Africa, Angola, Nigeria, Democratic Republic of the Congo, South Africa, Malawi, Guinea, and Uganda) (3). Dengue fever is endemic in over 128 countries according to the World Health Organization (4). In sub-Saharan Africa, the situation of the arbovirus is mainly characterized by dengue fever epidemics located in certain West African countries (4). The number of dengue cases reported to WHO increased over 8-fold over the last two decades, from 505,430 cases in 2000, to over 2.4 million in 2010, and 5.2 million in 2019 (4). Travel habits have increased the importation of the virus to new geographic areas by individuals with viremia (5). In sub-Saharan Africa, the arboviral diseases are mainly dominated by dengue fever epidemics located mostly in the Western African countries (6–10).
From 1983 to 1986, a study conducted in the western region of Burkina Faso reported a number of arboviruses, primarily flaviviruses, associated with sylvatic Aedes species including Aedes furcifer, Aedes luteocephalus, Aedes cumminsi, Aedes opok, and Aedes aegypti (11). Since then, in 2016, an outbreak of DENV has been reported in the human population within Ouagadougou without any clearly incriminated vector (12). Arboviral infection can be effectively controlled using safe and effective vaccines. Unfortunately, except for the yellow fever vaccine and Rift Valley fever vaccine (available for livestock) no effective vaccine is available for the other forms of arboviruses such as dengue (13). Preventing or minimizing vector–human contact and/or early detection of virus in the vectors or humans (for Ae. aegypti-borne arboviruses) can be key parameters to preventing or minimizing outbreaks. Detecting the presence of arboviruses in natural vector populations has been attempted several times with inconclusive results in some cases, suggesting that this approach to arbovirus detection in natural mosquito populations can be challenging (14). However, to predict the emergence of arboviral epidemics, the vector monitoring and control programs should be established in order to monitor and assess the prevalence of virus infection in natural and wild local vector populations. During the dengue outbreak of 2016, the monitoring and control strategy in Burkina Faso had consisted of passive detection of symptomatic human cases and spraying insecticides targeting adult Ae. aegypti, the presumed vector for DENV in this outbreak. Targeting the aquatic stages of Ae. aegypti was included in the national dengue vector control strategy, and this consisted primarily of the systematic removal of artificial breeding sites. As a result, that ultimately leads to the reduction of the adult vectors’ population and the possibility of vertical transmission, as revealed by several studies of Ae. aegypti populations (15–18). Indeed, adult stages resulting from the development of aquatic stages being infected by their previous mothers through transovarian transmission (TOT) are potential reservoirs for arboviruses and may initiate transmission and outbreaks. The aquatic stages that are not reached by spray campaigns can continue residual and autochthonous arbovirus transmission especially around the end of the rainy season, when the adult vector abundance is low (19). Therefore, the need to incriminate the vector species responsible for the transmission of a particular arbovirus, and to enhance disease monitoring in the vector population, are both important to develop successful outbreak responses.
Within the genus Aedes, Ae. aegypti is probably the most important species; it has been incriminated in a large number of arboviral outbreaks (1). Recent studies conducted in Burkina Faso indicated that of one in four cities along a railway transect, Ae. aegypti was the most common vector species collected in the container in domestic and peri domestic areas (20, 21). The abundance of Ae. aegypti is associated with a high risk of transmission of DENV and other arboviruses in Ouagadougou, Bobo-Dioulasso, Boromo, and Banfora cities. All stegomyian indices such as the house index (HI), the container index (CI), and the Breteau index (BI) exceeded the risk level associated with arboviral outbreak (20). However, the mosquitoes have not been processed for the presence of arboviruses.
Reverse-transcriptase quantitative polymerase chain reaction (qRT-PCR) in a molecular approach has been used in the recent years to detect the presence of arboviruses in Aedes mosquitoes and has proved to be a sensitive tool (22, 23); we have used this technique to screen the mosquito samples collected during previous entomological monitoring (20) for their infection profile. The ultimate objective is first to validate this approach with our previous samples from 2016 collections and then to extend this approach with wild adults including blood-fed females. For entomological monitoring, determining the infection rate in the wild population is crucial and the outbreak risk within the study area could be better understood.
Samples of Ae. aegypti collected in August 2016 during dengue outbreaks in Ouagadougou (12°21′56″N, 1°32′01″W), Bobo-Dioulasso (11° 10’ 41.16”N, 4° 17’ 30.38”W), Boromo (11° 45’ 0.00”N, 2° 55’ 60.00”W), and Banfora (10° 37’ 60.00”N, 4° 45’ 0.00”W), as previously reported in (22) and (23), were analyzed (Figure 1). Ouagadougou and Bobo-Dioulasso are urban whereas Banfora and Boromo are peri-urban. In short, at all four study sites, mosquito larvae were sampled from June to November 2016 to determine the indices of stegomyian (23). In each study area and in each house sampled, all containers found inside and outside the houses were inspected for the presence of water larvae and mosquitoes. Each container was recorded based on type, presence of water, and presence or absence of larvae. From positive containers, the mosquito larvae were pipetted and placed into plastic cups containing water and bearing the house identification number. The larvae have been enumerated and categorized as Culicinae genus. The mosquito reproductive habitats have been grouped into five types: plastic containers, metal containers, terra cotta pots, discarded tyres, and other containers (23). Subsequently, the extirpated adults were identified at the level of the species genus under binocular magnification (10×). Despite the fact that other species of Aedes have been identified, such as Ae. vittatus (found in very small number), only Ae. aegypti adults have been analyzed for this study. Hence, the female Ae. aegypti mosquitoes were segregated into pools depending on the site and placed in a cryo-tube containing RNA later (Qiagen RNAlater RNA Stabilization Reagent, 250 ml, lot No. 151026237, Qiagen Straße 1, 40724 Hilden Germany) and stored at −20°C for further laboratory analysis. A total of 580 females were grouped in 33 pools of 10 and 20 mosquitoes ready to be screened for the detection of dengue viruses 2 and CHIKV in this preliminary data research article. The analysis focused on DENV2 detection due to the reported results of serological surveys from positive cases detected during the 2016 outbreak in Burkina Faso (23). The other arboviruses (WNV, DENV1, etc.) will be subjected to subsequent analyses. Samples were prepared for shipment to the Insect Pest Control Laboratory (IPCL), Joint FAO/IAEA Programme of Nuclear Techniques in Food and Agriculture, Seibersdorf, Austria for the RNA extraction and qRT-PCR analysis.
Figure 1 Study sites located on railway transect in Burkina Faso (Banfora, Bobo-Dioulasso, Boromo, and Ouagadougou).
Total RNA was extracted from mosquitoes using the TRIzol ™Reagent (Invitrogen, Waltham, MA, USA, Catalog Numbers 15596026 and 15596018) as directed by the supplier. Depending on the size of the pool, adult mosquitoes were homogenized into 1 ml of TRIzol using a sterile pestle. All pools were individually homogenized by vertexing for 15 s and incubated for 5 min at ambient temperature to allow complete dissociation of the nucleoprotein complex. Chloroform (200 μl per 1 ml of TRIzol™ Reagent) was added to the mixture, and homogenization was performed by shaking the tubes vigorously for 15 s by hand. The mixture was then incubated at ambient temperature for 2–3 min. The samples were centrifuged at 12,000 g for 15 min at 4°C. The mixture splits into a weaker red phenol-chloroform interphase and a colorless superior aqueous phase. The aqueous phase of each sample was removed and transferred to a new tube containing 500 μl of 100% isopropanol by angling the tube at 45° and pipetting the solution out. This mixture was incubated at ambient temperature for 10 min and then centrifuged at 12,000 g for 10 min at 4°C. Total RNA precipitate forms a white gel-like pellet at the bottom of the tube. The supernatant was removed, and the RNA pellet was washed with 1 ml of 75% ethanol mixing occasionally by gentle inversion. The samples were homogenized briefly and then centrifuged at 7,500 g for 5 min at 4°C. The supernatant was discarded, and the RNA was then air-dried for 15 min. The RNA pellet was resuspended in 50 µl of RNase-free water by pipetting up and down, then incubated in a water bath or heat block set at 55°C for 10–15 min. The quantity and quality of RNA samples were determined using a Synergy™ H1 microplate reader (BioTek, Winooski, Vermont, USA). The RNA samples were serially diluted in 10-fold steps from 10 to 0.0001 ng/μl for a concentration that would consistently give the same amount per well in the qRT-PCR and were stored at −80°C.
The standard curve for the DENV-2 virus was conducted according the method described by Tang et al. (24). In brief, the RNA sequences containing and flanking the sequence regions of the virus-specific primers and probes of CHIKV and DENV2 were amplified using the primers listed in Table 1 and cloned into pGEM-T vector (Promega, Madison, Wisconsin, USA). The primers in Table 1 have been selected using the primer3 software with the default setting (http://bioinfo.ut.ee/primer3-0.4.0/) and the sequence of CHIKV (GenBank: NC004162) and DENV2 (GenBank: MN294937). These plasmids were linearized through digestion with EcoRI, and the target sequences were amplified using the in vitro RNA transcription kit (Roche Diagnostics, IN, USA). The plasmids containing the cDNA of two arboviruses collected from blood samples from positive patients were treated with the Fast-Media® LB Amp. They were extracted and purified using the QIAprep® Miniprep purification kit (Qiagen). The SuperScript® III First-Strand Synthesis System for RT-qPCR (Invitrogen) was used to synthesize first-strand cDNA from purified poly(A)+ or total RNA. The targeted sequences were amplified by Taq PCR Master Mix (Qiagen) with the following PCR conditions: 5 min at 94°C; 35 cycles of 30 s at 94°C, 30 s at 58°C, and 1 min at 72°C; and 10 min at 72°C. The PCR product was purified using the High Pure PCR Clean-up Micro Kit (Roche, Basel, Switzerland). The cDNA was quantified using a Synergy™ H1 microplate reader (BioTek, Winooski, Vermont, USA). The copy number of the cDNA was calculated based on the concentration and its molecular weight using NEBioCalculator (https://nebiocalculator.neb.com/#!/dsdnaamt), then seven concentrations with known DNA copy numbers/µl were prepared by serial dilutions and used as standard in all qRT-PCRs. A series of dilutions of seven known concentrations were used as a positive control for each qRT-PCR reaction. Sterile, nuclease-free water was used as a no template control (NTC).
ARBOV detection was performed by quantitative RT-PCR using a C1000 TouchR Thermal Cycler machine (CFX96R Real-Time System from BIO-RAD). One-step real-time qRT-PCR was conducted using ARBO virus-specific primers, and TaqMan probes previously reported to detect each specific virus were synthesized by the Eurofins Genomics with 5-FAM, HEX as the reporter dye for the probe. The details of the primer and probe sequences and their characteristics are summarized in Table 2 (25, 26). Using the Quantitect Probe qRT-PCR kit (Qiagen), qRT-PCR reactions were performed in a 20-µl volume mixture containing 2 µl of RNA template, 10 µl of 2× QuantiTect Probe qRT-PCR Master Mix, 8 µM of forward primer, 8 µM of reverse primer, 2.5 µM of probe, 0.25 µl of QuantiTect RT Mix, and 4.95 µl of RNase-free water. The primers and probes have been double tested. qRT-PCR cycling included a single cycle of reverse transcription of 30 min at 50°C, followed by 15 min at 95°C for reverse transcriptase inactivation and DNA polymerase activation, and then 45 cycles of 15 s at 94°C and 60 s at 60°C (annealing-extension step). The quantity of viral RNA in each sample was estimated by comparing the threshold values for cycle quantification (Cq) to the standard curve for each qRT-PCR test. The number of viral copies in each positive sample was estimated by qRT-PCR using absolute quantification by the standard curve method and reported as viral RNA copies/μl (of the eluted RNA). For each qRT-PCR test, both negative (RNA-free water) and positive samples (RNA sample tested positive for each virus) were added to ensure that no contamination occurred.
qRT-PCR data were analyzed using the CFX managed software version 3.1 supplied by Bio-Rad. Negative and positive controls were included in all PCR reactions conducted. A sample was determined empirically to be positive if the cycle quantification (Cq) value was lower than 36, based on background cross-reactivity of the primers and probes in non-template control reactions. Positive results were determined according to the amplification cycle at which the relative fluorescence unit (RFU) was detected below the cycle quantification.
The Excel add-in PooledInfRate v.4 statistical software package was used to calculate the minimal infection rate (MIR) of arboviruses in mosquitoes with a 95% confidence interval (27). Only the positive pools of female Aedes mosquitoes have been calculated for their MIR. The MIR was estimated based on the number of female mosquitoes in a positive pool divided by the number of female mosquitoes tested multiplied by 1,000. The MIR uses the assumption that a positive pool contains only one infected mosquito. The statistical analysis was carried out by means of Fisher’s exact test. Data were compared by sites and also following two types of area such as urban representing the two big cities (Ouagadougou and Bobo-Dioulasso) and suburbs, which are also cities but considerably smaller than the first ones mentioned above (Banfora and Boromo).
The RNA concentrations were 11.53 ng/µl and 490.98 ng/µl in pools of 10 and 20 mosquitoes, respectively. The ratio of A260:A280 was approximately two values with RNA purity extracted from the samples.
Above the cycle threshold (Cq) of 36, the amplified RNA samples showing curves were considered to have very low concentrations, and as a result, the samples were not considered to be infected by the targeted virus. Following the protocol described by Tang et al. (24), the purified plasmid was used for each virus and a DNA concentration with a known copy number of 4.7 × 109 was prepared. Subsequently, 10-fold serial dilutions in water were used to prepare seven DNA concentrations with copy numbers ranging from 4.7 × 10-2 to 4.7 × 10-8 per ml, which were used to prepare the calibration curves for primer and probe for DENV2. Viral DNA detection has been a success for both viruses, and standard curves have shown a linearity of more than seven orders of magnitude (Figure 2). The correlation coefficient (R2 value) was 0.998 for DENV2. The calibration curves for CHIKV were previously described per Tang et al. (24), where the correlation coefficient (R2 value) was 1.000. The regression coefficients (R2) of 0.998 and 1.000 for DENV2 and CHIKV, respectively, indicated the high reproducibility of the test.
Figure 2 Amplification and standard curves of serial dilution of plasmid containing the sequence targeted by the primers and probes for the qRT-PCR for DENV2 detection: (A) the correlation between the relative fluorescent unit (RFU) and the quantification cycle (Cq) and (B) the virus log 10 copy number and the Cq. The regression equations and correlation coefficients (R) are given for the DENV2 plot.
In addition, the quantification cycle (Cq) values for the standard DENV2 concentration of seven series dilutions ranged from 11 ± 0.02 to 33.5 ± 0.065 Cq (Figure 2). Samples taken in Ouagadougou for DENV-2 showed a Cq amplifying curve of 32, indicating that the samples were infected with DENV2 (Figure 3). Otherwise, the quantitative cycle values of the standard CHIKV concentration of seven dilutions ranged from 10 ± 0.05 to 30 ± 0.21 Cq as previously described by Tang et al. (24). The amplification curves of RNA samples tested for detecting CHIKV started at 28 Cq and reached 35 Cq (Figure 4), indicating the presence of RNA virus at different densities varied based on samples tested. All samples analyzed for CHIKV detection were less than 36 Cq (Figure 4) and therefore were all considered positive.
Figure 3 Positive RNA samples from Ouagadougou for the detection of dengue virus 2 (DENV2) RNA by one-step real-time qRT-PCR assay.
Figure 4 Positive RNA samples from Ouagadougou, Boromo, and Banfora for the detection of chikungunya virus (CHIKV) by real-time qRT-PCR assay.
A total of 27 pools of Aedes mosquitoes were tested for arboviruses. The mosquitoes were collected in August 2016, corresponding to the time of a dengue outbreak in Burkina Faso. Overall, 480 Ae. aegypti were tested (Table 3). The qRT-PCR analysis showed the presence of arboviruses with one pool positive for DENV-2 (3.7%) and six pools positive for CHIKV (22.22%), but density varied depending on locality (Table 3). Indeed, the minimum infection rates (MIRs) of CHIKV in Aedes mosquitoes were estimated at 66.67 (95% CI: 41.2–72.6) (4 infected pools/60 samples tested), 0 (0 infected pool/140 samples tested), 7.14 (95% CI:4.3–10.2) (1 infected pool/140 samples tested), and 7.14 (95% CI: 4.3–10.2) (1 infected pool/140 samples tested) in Ouagadougou, Bobo-Dioulasso, Banfora, and Boromo, respectively (Table 4). The MIR of CHIKV was significantly higher in Ouagadougou compared to the other locations (p = 0.0219). The MIR of DENV-2 was estimated at 16.67 (95% CI: 14.7–19.4) (1 infected pools/60samples tested) in Ouagadougou while no detection has been recorded in the other sites (Tables 3, 4). When the study sites were categorized based on their predominant land use type (urban or peri-urban), MIR differed. In urban areas, the MIR of DENV-2 was 5 (95% CI: 3.7–6.9) and that for CHIKV was 20 (95% CI: 17.5–26.1), both higher than that in peri-urban sites (p < 0.01) (Table 4). The data also showed a co-infection of arboviruses (DENV/CHIKV) in one pool from Ouagadougou (Tables 3, 4).
Table 3 Summary of arbovirus examination of pools (10–20 size) of Aedes mosquitoes collected in Burkina Faso.
Table 4 Minimum infection rates (with 95% confidence interval) of targeted ARBOV screened in Ae. aegypti mosquitoes.
This study is the result of entomological investigations conducted in 2016 in urban and peri-urban areas along a rail transect from Banfora to Ouagadougou during a dengue epidemic in Burkina Faso. In the first published article on this study, we found that all the larval indices that have been examined had largely exceeded the critical level outbreaks in the four cities where the study has been conducted. This implies an increased transmission risk for at least DENV including the peri-urban areas such as Boromo and Banfora (20). Current evidence builds on the previous findings by identifying and quantifying the presence of arbovirus in wild Ae. aegypti populations. In fact, DENV-2 and CHIKV have been detected in certain pools with pronounced rates of infection recorded especially in Ouagadougou for DENV-2. Although CHIKV infection was also detected in Boromo and Banfora, its prevalence was higher in Ouagadougou, indicating probably that these viruses were more concomitantly circulating in the capital city than in the other study areas.
Globally, the infection features described in Ae. aegypti populations confirmed the autochthonous urban transmission of dengue in urban cities as Ouagadougou. They were more or less consistent with human infection patterns where serotypes DENV-2 and DENV-3 were most detected in humans (28–32). However, in exceptional cases, DENV-4 was reported by Ridde et al. (23) at the same time as DENV-2 and DENV-3. The presence of DENV-2 was also found in the blood of febrile travelers returning from Burkina Faso to France during this period (28).
The findings also showed the occurrence of CHIKV in the vector populations from three of the four sites sampled. This was more prevalent in Ouagadougou than the other areas. No data have previously confirmed their presence in human patients, probably due to the lack of specific serological tests targeting these viruses during the diagnosis process.
This is the first study confirming the circulation of arboviruses in vector populations from both urban and peri-urban areas in Burkina Faso. In 1993, the sylvatic circulation of arbovirus was confirmed by the isolation of both DENV-2 and CHIKV in the surrounding forest gallery of the Bobo-Dioulasso region from wild Aedes mosquitoes such as Ae. furcifer, Ae. luteocephalus, Ae. cumminsi, Ae. opok, and in Bobo-Dioulasso city for DENV-2 from Ae. aegypti (13). The epidemiology of arboviruses in Africa in the last 5 years has shown an expansion of certain arboviruses such as West Nile, Chikungunya, and Zika in West Africa (29–32).
Our findings confirm a vertical transmission of arboviruses (16, 17) as well as DENV-2 and CHIKV in Ae. aegypti populations of urban and peri-urban settlements, which has been reported from earlier studies (18, 19). Vertical transmission (infected female mosquito–infected offspring) is assumed to be a mechanism ensuring the maintenance of the virus during conditions that would be adverse for horizontal transmission (harsh winters, inter-epidemic stages) (33) and can also potentially accelerate the epidemiology of ARBOV if the monitoring system is not sufficiently reliable. Although it is mainly the horizontal transmission (human–mosquito–human) of arboviruses that determines the epidemiology of the disease, health authorities should therefore be vigilant for future outbreaks. Furthermore, a co-infection (DENV/CHIKV) was detected in a pool of Aedes from Ouagadougou highlighting the co-circulation of both arboviruses. Even if, our control system focused on the larval stages providing vertical transmission, it can support autochthone transmission if the larval indices and the domestic index reach the threshold. In addition, it should be noted that using field-collected larvae and keeping it in the laboratory until adult emergence for the detection of ARBO was successful, but it may not be the best approach for disease surveillance because it may lose infection in dead larvae or pupae before adult emergence. The greatest limitation of this study is that no sample of mosquitoes directly collected in the field at the adult stage that can include blood-fed females was considered in the analyzed samples due to the collection method. As such, the data presented here are more observational and preliminary and the conclusion needs to be observational and not yet predictive until we expand the testing for adult field mosquitoes. Furthermore, the annual recurrence of dengue outbreaks in Burkina over the last five years increases the interest in developing such a molecular diagnostic approach for vector surveillance. Indeed, the growth of international travel to and from Burkina Faso, especially from passengers arriving from other countries where arboviruses are endemic, should be seen as a potential reservoir of virus. These conditions support the circulation of arboviruses in Burkina Faso. During the rainy season, this vertical transmission could be the source of a resurgence of dengue.
Our study found that DENV-2 and CHIKV, in both urban and peri-urban areas, were detected from wild Ae. aegypti larvae bred to adulthood. This highlighted the occurrence of a vertical transmission and leads us to recommend the urgent need to implement a vector control program including larval source reduction in Burkina Faso, instead of only fogging adult Ae. aegypti populations with insecticides, whose effectiveness in the field has not been assessed after intervention. This molecular approach is the first successful investigation using molecular diagnostics in Burkina Faso to detect and quantify arboviruses within wild mosquito populations during outbreak periods even from adults bred from wild larva collection. This tool is powerful and suitable and should be adopted by the national monitoring program of arboviruses; if extended to adult mosquitoes directly collected in the field including blood-fed females, it remains specific and suitable. Nevertheless, the results are preliminary and should be seen as indicative until they become validated by wide-scale and large spectrum of samples.
The original contributions presented in the study are included in the article/Supplementary Material. Further inquiries can be directed to the corresponding authors.
DKR, VR, EB, and AA-A conceived and designed the experiments. ELPO, IS, SPS, and DDS participated in the field collection. ASH, AA-A and CK performed the RT-qPCR to detect arboviruses. AH, CK, ELPO, AA-A, and RKD analyzed the data. ASH, ELPO, IS, and RD wrote the paper. ASH, JB, FH, AD, AA-A, and RKD contributed to the paper writing and editing. All authors read and approved the final version of the manuscript.
This research was funded by BKF5020 Technical Project from the International Atomic Energy Agency (IAEA) in Vienna (Austria). The funders had no role in the design of the study; in the collection, analyses, or interpretation of data; in the writing of the manuscript; or in the decision to publish the results.
We thank the technicians of the IRSS team who conducted mosquito collections and insectary works. We also thank the IAEA authorities for the training of ASH, which allowed us to reach these preliminary results. We are grateful to households in the cities where the surveys were performed.
The authors declare that the research was conducted in the absence of any commercial or financial relationships that could be construed as a potential conflict of interest.
All claims expressed in this article are solely those of the authors and do not necessarily represent those of their affiliated organizations, or those of the publisher, the editors and the reviewers. Any product that may be evaluated in this article, or claim that may be made by its manufacturer, is not guaranteed or endorsed by the publisher.
1. Hertz JT, Lyaruu LJ, Ooi EE, Mosha FW, Crump JA. Distribution of aedes mosquitoes in the Kilimanjaro region of northern Tanzania. Pathog Glob Health (2016) 110(3):108–12. doi: 10.1080/20477724.2016.1182719
2. Patterson J, Sammon M, Garg M. Dengue, zika and chikungunya: Emerging arboviruses in the new world. West J Emerg Med (2016) 17(6):671–9. doi: 10.5811/westjem.2016.9.30904
3. Johansson MA, Powers AM, Pesik N, Cohen NJ, Staples JE. Nowcasting the spread of chikungunya virus in the americas. PloS One (2014) 9(8):e104915. doi: 10.1371/journal.pone.0104915
4. World Health Organization (WHO). Dengue and severe dengue. Available at: https://www.who.int/news-room/fact-sheets/detail/dengue-and-severe-dengue.
5. Wahid B, Ali A, Rafique S, Idrees M. Global expansion of chikungunya virus: mapping the 64-year history. Int J Inf Dis (2017) 58:69–76. doi: 10.1016/j.ijid.2017.03.006
6. Wahid B. Current status of dengue virus, poliovirus, and chikungunya virus in Pakistan. J Med Virol (2019) 91(10):1725–8. doi: 10.1002/jmv.25513
7. Sow A, Loucoubar C, Diallo D, Faye O, Ndiaye Y, Senghor SC, et al. Concurrent malaria and arbovirus infections in kedougou, southeastern Senegal. Malar J (2016) 15(1):47. doi: 10.1186/s12936-016-1100-5
8. Oyero OG, Ayukekbong JA. High dengue NS1 antigenemia in febrile patients in ibadan, Nigeria. Virus Res (2014) 191(1):59–61. doi: 10.1016/j.virusres.2014.07.023
9. Phoutrides EK, Coulibaly MB, George CM, Sacko A, Traore S, Bessoff K, et al. Dengue virus seroprevalence among febrile patients in Bamako, Mali: results of a 2006 surveillance study. Vector Borne Zoonotic Dis (2011) 11(11):1479–85. doi: 10.1089/vbz.2011.0622
10. L’Azou M, Succo T, Kamagate M, Ouattara A, Gilbernair E, Adjogoua E, et al. Dengue: etiology of acute febrile illness in Abidjan, cote d’Ivoire, in 2011-2012. Trans R Soc Trop Med Hyg (2015) 109(11):717–22. doi: 10.1093/trstmh/trv076
11. Mamoudou S, Boushab BM. Formes hémorragiques de dengue observées dans le service des maladies infectieuses du CHU yalgado ouédraogo, Burkina Faso. Pan Afr Med J (2016) 23:1–4. doi: 10.11604/pamj.2016.23.168.9234
12. Robert V, Lhuillier M, Meunier D, Sarthou JL, Monteny N, Digoutte JP, et al. Virus amaril, dengue 2 et autres arbovirus isolés de moustiques au Burkina Faso, de 1983 à 1986 : Considérations entomologiques et épidémiologiques. Bull Soc Pathol Exot (1993) 86:90–100.
13. Fournet F, Rican S, Vaillant Z, Roudot A, Meunier-Nikiema A, Kassié D, et al. The influence of urbanization modes on the spatial circulation of flaviviruses within Ouagadougou (Burkina fao). Int J Environ Res Public Health (2016) 13:1226. doi: 10.3390/ijerph13121226
14. Ramírez AL, Van Den Hurk AF, Meyer D, Ritchie SA. Searching for the proverbial needle in a haystack: Advances in mosquito-borne arbovirus surveillance. Parasites Vectors (2018) 11(1):320. doi: 10.1186/s13071-018-2901-x
15. Gu W, Novak RJ. Short report: Detection probability of arbovirus infection in mosquito populations. Am J Trop Med Hyg (2004) 71(5):636–8. doi: 10.4269/ajtmh.2004.71.636
16. da Costa CF, da Silva AV, do Nascimento VA, de Souza VC, da Silva Monteiro DC, Terrazas WCM, et al. Evidence of vertical transmission of zika virus in field-collected eggs of Aedes aegypti in the Brazilian Amazon. PLoS negl trop dis (2018) 12(7). doi: 10.1371/journal.pntd.0006594
17. Unlu I, MacKay AJ, Roy A, Yates MM, Foil LD. Evidence of vertical transmission of West Nile virus in field-collected mosquitoes. J Vector Ecol (2010) 35(1):95–9. doi: 10.1111/j.1948-7134.2010.00064.x
18. Günther J, Martínez-Muñoz JP, Pérez-Ishiwara DG, Salas-Benito J. Evidence of vertical transmission of dengue virus in two endemic localities in the state of oaxaca, Mexico. Intervirology (2007) 50(5):347–3. doi: 10.1159/000107272
19. Jain J, Kushwah RBS, Singh SS, Sharma A, Adak T, Singh OP, et al. Evidence for natural vertical transmission of chikungunya viruses in field populations of Aedes aegypti in Delhi and haryana states in India-a preliminary report. Acta Trop (2016), 162:46–55. doi: 10.1016/j.actatropica.2016.06.004
20. Ouattara LPE, Sangaré I, Namountougou M, Hien A, Ouari A, Soma DD, et al. Surveys of arboviruses vectors in four cities stretching along a railway transect of Burkina Faso: Risk transmission and insecticide susceptibility status of potential vectors. Front Vet Sci (2019) 6(140). doi: 10.3389/fvets.2019.00140
21. Ouédraogo S, Benmarhnia T, Bonnet E, Somé PA, Barro AS, Kafando Y, et al. Evaluation of effectiveness of a community-based intervention for control of dengue virus vector, Ouagadougou, Burkina Faso. Emerg Infect Dis (2018) 24(10):1859. doi: 10.3201/eid2410.180069
22. Chieng C, Lutomiah J, Makio A, Koka H, Chepkorir E, Yalwala S, et al. Mosquito-borne arbovirus surveillance at selected sites in diverse ecological zones of kenya; 2007 - 2012. Virol J (2013) 10(1):140. doi: 10.1186/1743-422X-10-140
23. Ridde V, Agier I, Bonnet E, Carabali M, Dabiré KR, Fournet F, et al. Presence of three dengue serotypes in Ouagadougou (Burkina faso): research and public health implications. Infect Dis Poverty (2016) 5(1):1–13. doi: 10.1186/s40249-016-0120-2
24. Tang Z, Yamada H, Kraupa C, Canic S, Busquets N, Talavera S, et al. High sensitivity of one-step real-time reverse transcription quantitative PCR to detect low virus titers in large mosquito pools. Parasites Vectors (2020) 13(1):1–13. doi: 10.1186/s13071-020-04327-4
25. Johnson BW, Chambers TV, Crabtree MB, Guirakhoo F, Monath TP, Miller BR. Analysis of the replication kinetics of the ChimeriVax-DEN 1, 2, 3, 4 tetravalent virus mixtures in aedes aegypti by real-time reverse transcriptase-polymerase chain reaction. Am J Trop Med Hyg (2004) 70:89–97. doi: 10.4269/ajtmh.2004.70.89
26. Lanciotti RS, Kosoy OL, Laven JJ, Panella AJ, Velez JO, Lambert AJ, et al. Chikungunya virus in US travelers returning from India. Emerg Infect Dis (2006) 13:764–7. doi: 10.3201/eid1305.070015
27. Biggerstaff BJ. PooledInfRate, version 3.0: a Microsoft excel add-in to compute prevalence estimates from pooled samples. Fort Collins CO: Centers Dis Control Prev (2006) 970:221–6473.
28. Eldin C, Gautret P, Nougairede A, Sentis M, Ninove L, Saidani N, et al. Identification of dengue type 2 virus in febrile travellers returning from Burkina Faso to France, related to an ongoing outbreak, October to November 2016. Eurosurveillance (2016) 21(50):30425. doi: 10.2807/1560-7917.ES.2016.21.50.30425
29. Tarnagda Z, Congo M, Sagna T, Ouédraogo C, Nikiéma V, Cissé A, et al. Outbreak of dengue fever in Ouagadougou, Burkina Faso, 2013. Int J Microbiol Immunol Res (2014) 2(7):101–8.
30. Safronetz D, Sacko M, Sogoba N, Rosenke K, Martellaro C, Traoré S, et al. Vector-borne infections, Mali. Emerg Infect Dis (2016) 22(2):340. doi: 10.3201/eid2202.150688
31. Manu SK, Bonney JHK, Pratt D, Abdulai FN, Agbosu EE, Frimpong PO, et al. Arbovirus circulation among febrile patients at the greater Accra regional hospital, Ghana. BMC Res Notes (2019) 12(1):332. doi: 10.1186/s13104-019-4378-x
32. Bacci A, Marchi S, Fievet N, Massougbodji A, Perrin RX, Chippaux JP, et al. High seroprevalence of chikungunya virus antibodies among pregnant women living in an urban area in Benin, West Africa. Am J Trop Med Hyg (2015) 92(6):1133–6. doi: 10.4269/ajtmh.14-0092
Keywords: arboviruses, dengue, Chikungunya, molecular biology, qRT-PCR, Aedes aegypti, Burkina Faso
Citation: Hien AS, Sangaré I, Ouattara ELP, Sawadogo SP, Soma DD, Maiga H, Diabaté A, Bonnet E, Ridde V, Fournet F, Hawkes FM, Kaupra C, Bouyer J, Abd-Alla AMM and Dabiré RK (2022) Chikungunya (Togaviridae) and dengue 2 (Flaviviridae) viruses detected from Aedes aegypti mosquitoes in Burkina Faso by qRT-PCR technique: Preliminary results and perspective for molecular characterization of arbovirus circulation in vector populations. Front. Trop. Dis 3:920224. doi: 10.3389/fitd.2022.920224
Received: 14 April 2022; Accepted: 09 August 2022;
Published: 14 September 2022.
Edited by:
Henri Edouard Zefack Tonnang, International Centre of Insect Physiology and Ecology (ICIPE), KenyaReviewed by:
Natarajan Gopalan, Central University of Tamil Nadu, IndiaCopyright © 2022 Hien, Sangaré, Ouattara, Sawadogo, Soma, Maiga, Diabaté, Bonnet, Ridde, Fournet, Hawkes, Kaupra, Bouyer, Abd-Alla and Dabiré. This is an open-access article distributed under the terms of the Creative Commons Attribution License (CC BY). The use, distribution or reproduction in other forums is permitted, provided the original author(s) and the copyright owner(s) are credited and that the original publication in this journal is cited, in accordance with accepted academic practice. No use, distribution or reproduction is permitted which does not comply with these terms.
*Correspondence: Roch K. Dabiré, ZGFiaXJlcm9jaEBnbWFpbC5jb20=; Adly M. M. Abd-Alla, YS5tLm0uYWJkLWFsbGFAaWFlYS5vcmc=
†These authors have contributed equally to this work and share last authorship
Disclaimer: All claims expressed in this article are solely those of the authors and do not necessarily represent those of their affiliated organizations, or those of the publisher, the editors and the reviewers. Any product that may be evaluated in this article or claim that may be made by its manufacturer is not guaranteed or endorsed by the publisher.
Research integrity at Frontiers
Learn more about the work of our research integrity team to safeguard the quality of each article we publish.