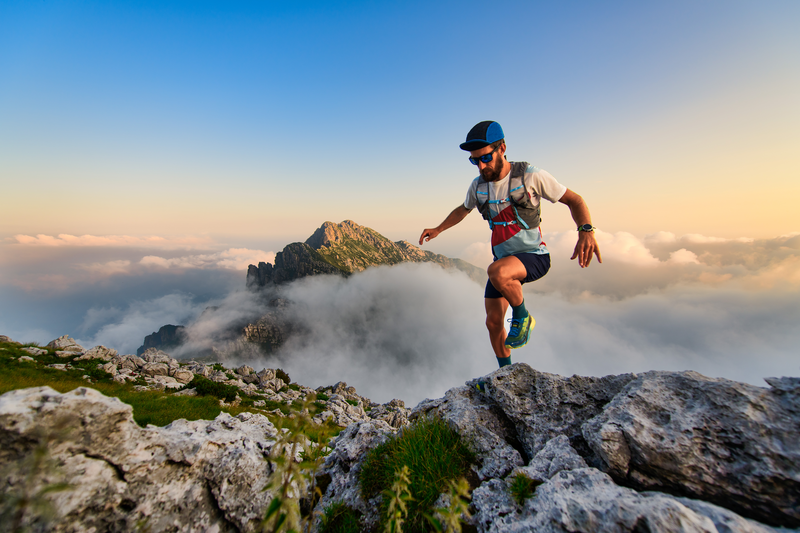
95% of researchers rate our articles as excellent or good
Learn more about the work of our research integrity team to safeguard the quality of each article we publish.
Find out more
ORIGINAL RESEARCH article
Front. Trop. Dis. , 05 July 2022
Sec. Vector Biology
Volume 3 - 2022 | https://doi.org/10.3389/fitd.2022.903654
This article is part of the Research Topic Highlights in Vector Biology View all 8 articles
Extensive use of insecticides has led to the selection of resistance alleles in malaria vectors threatening the control programs. Even if mosquitoes are not killed directly in the contact of insecticide-treated bed nets, their capacity to transmit malaria parasite could be decreased because of the consequences on their life-history traits after repeated exposure. The current work investigated the effects of organochlorine, carbamate, organophosphate, and pyrethroid insecticide exposure on the reproductive success in Anopheles gambiae s.s. Two Anopheles gambiae strains, AcerKis, KisKdr, were used. According to WHO recommendations, female mosquitoes of these resistant strains were exposed to discriminant doses of DDT, chlorpyriphos-methyl, bendiocarb, and permethrin insecticides. Surviving mosquitoes were then fed and allowed to lay eggs. Fecundity was assessed by examining the number of eggs per mosquito, the number of larvae per egg batch and larval hatching rates were used to evaluate the fertility. The data showed that AcerKis females surviving chlorpyriphos-methyl exposure significantly laid few eggs. No significant difference in the hatching rate was noticed in AcerKis females exposed to bendiocarb compared to their control. No significant effect on the fecundity and fertility was observed in KisKdr females exposed to permethrin. Our finding showed that organophosphate insecticides represented here by chlorpyriphos-methyl could hamper egg-laying in insensitive acetylcholinesterase An. gambiae female mosquitoes. This knowledge could help design alternative vector control strategies targeting fecundity and fertility in resistant malaria vectors.
Malaria is still among the infectious diseases ranked in the top causes of death worldwide, especially in low-income countries (1). This parasitic disease is transmitted to humans by female mosquitoes of the genus Anopheles. Three strategies are developed for malaria control, including prevention, diagnosis, and treatment. To date, insecticide-based vector control through the use of Long-Lasting Insecticidal Nets (LLINs) and the Indoor Residual Spraying (IRS) is, among the prevention programs, the most widely implemented strategy and remains the most efficient method for malaria control in most endemic countries (2). Indeed, the percentage of the population protected by the vector control interventions increased from 29% in 2010 to 50% in 2018, representing half of the population worldwide (3).
The World Health Organization (WHO) recommended five classes of insecticides for vector control interventions, including pyrethroids, organochlorines, carbamates, organophosphates, and neonicotinoids (3). Unfortunately, the long-term success of both LLINS and IRS strategies is nowadays hampered by the widespread emergence of insecticide resistance mechanisms in mosquito populations, especially in sub-Saharan African regions (4–7). Besides, WHO reported that if there is no change in the current trend in malaria incidence (57 cases per 1000 population at risk), the predicted malaria case incidence would be 48 in 2025 and 42 in 2030, rather than 14 and 6 necessary to achieve the global technical strategy (GTS) pillars for malaria elimination by 2030 (3). Therefore, National Malaria Control Programmes (NMCPs), international policymakers, and funding agencies should make efforts to increase resistance monitoring and surveillance in Anopheline vectors (8). Since most of NMCPs developed in the endemic countries are in line with the malaria elimination and eradication goals, it appears essential that the implications of the insecticide resistance phenomenon in malaria transmission are much more explored and strategies to alleviate these effects are performed (4).
In most African countries, the major malaria vector Anopheles gambiae already displays a multi-resistance to the existing insecticides (9–11). However, in the absence of an effective malaria vaccine for individuals above 5 years old, there is still the need to look for alternative vector control strategies to protect the global population at risk.
Since the same insecticides recommended are still being used, the expanding coverage of vector control strategies leads to frequent contact of the resistant vectors with the insecticides (12). Therefore, these vectors can ingest higher doses of insecticides since the resistant alleles can allow them to prolong contact with treated materials (13) without being killed. Thus, one should ask whether the long-term accumulation of insecticide residues affects mosquito life-history traits. It was suggested that even if insecticides have no direct impact on the resistant vectors, they could significantly influence malaria transmission if they negatively affect the life-history trait of the vectors (14). Available data suggest that fitness costs are associated with insecticide resistance following insecticide exposure. This might influence malaria transmission by impacting mosquito life-history traits, such as reducing their lifespan (14). The discovery of much more life-history traits affected by the insecticide exposure would be useful for controlling resistant malaria vectors. Knowledge of vector biology has also been revealed to be important when implementing current control strategies. For instance, the understanding of mosquito blood-feeding and host-seeking abilities has allowed the development of vector control strategies such as i) zooprophylaxis to divert mosquitoes from human to nonhuman hosts set as bait (15); ii) Attractive Toxic Sugar Baits (ATSB), where mosquitoes are killed following feeding on toxic sugar meals to which they are attracted to (15); iii) Push-pull strategies that aim to reduce outdoor biting (16), by coupling the use of repellents with a baited trap to direct host-seeking mosquitoes from sprayed areas to the trap (17, 18).
However, little is known about the direct consequences of insecticide exposure on vector life-history traits, especially on the reproductive success of Anopheles mosquitoes. To contribute to the understanding of insecticide resistance implications for malaria vector control based on the mosquito reproductive system, this study investigated the effect of exposure to organochlorine (Dichlorodiphenyltrichloroethane), organophosphate (chlorpyriphos-methyl), carbamate (bendiocarb) and pyrethroid (permethrin) on the fecundity and fertility in two Anopheles gambiae strains.
Two Anopheles gambiae sensu stricto (An. gambiae s.s.) laboratory strains already available at the insectarium of the Laboratory of Infectious Vector-Borne Diseases of the University of Abomey-Calavi in Ouidah (Benin) were used in this study. AcerKis strain is resistant to organophosphate and carbamate insecticides and is homozygous for the ace-1R (G119S) allele (19). KisKdr, which is homozygous for the kdrR (L1014F) allele, is resistant to pyrethroid and DDT insecticides (20). AcerKis and KisKdr were supposed to share the same genetic background but differ by the presence of resistance alleles (L1014F and G119S). All the colonies of An. gambiae were maintained at the insectarium under optimum conditions (27 ± 2°C temperature and 70 ± 8% relative humidity). Larvae were reared in the same conditions in dechlorinated water and were fed with Tetramin Baby fish food. After emergence, adult mosquitoes were fed ad libitum on a 10% honey solution and maintained in 30x30x30 cm cages until they were ready to be used for further assays.
Non-blood-fed mosquito females aged 3–5 days from all strains were exposed to discriminating dosages of several insecticides using the WHO standard vertical tube kits (21). Insecticide exposures were performed as follows: 1 hour exposure to bendiocarb 0.1% (BDC 0.1%); 30 minutes exposure to chlorpyrifos-methyl 0.4% (CM 0.4%); 3 hours exposure to permethrin 0.75% (PM 0.75%) and Dichlorodiphenyltrichloroethane 4% (DDT 4%). Control tests were also set up for each strain by exposing adult females to untreated papers. Test papers were used no more than five times before being replaced. After insecticide exposures, mosquitoes were gently transferred into holding tubes, provided with a 10% honey solution and mortality was recorded 24 hours later.
After the holding period, AcerKis and KisKdr females were blood-fed on a rabbit and were allowed to blood feed again the third day after the first blood-feeding. Each blood-feeding session lasted for 30 minutes. The gravid mosquitoes of each strain were individually transferred into plastic cups containing wet Whatman filter paper for oviposition. They were allowed to feed on 10% honey solution until egg-laying. The number of females laying eggs was recorded, and eggs were counted under a stereomicroscope (Leica Microsystems EZ4HD). Egg batches (from individual females) were transferred in separate plastic trays filled with dechlorinated water, and the number of hatched larvae was recorded. Two biological replicates were performed.
When control mortality is ≥ 5%, then the observed mortality in test groups was corrected using Abbott’s formula, as follows (21):
The WHO insecticide susceptibility test with discriminating concentration criteria was used to determine the phenotypic resistance status of the exposed mosquitoes to each class of insecticides (21). Insecticide resistance status was declared when the mortality rates were less than 90%. When the mortality rates were greater than 97%, mosquitoes were considered susceptible.
The fecundity in each mosquito strain was assessed as the total number of eggs over the total number of females that contributed to oviposition. Fertility in each mosquito strain expressed as a percentage of hatched larvae was evaluated by dividing the total number of first instar larvae over the total number of eggs. The median was used as the estimated parameters showed non-normal distribution (Shapiro-Wilk test: p < 0.05). Fecundity and fertility were compared with the Kruskal-Wallis test using R version 4.0.1 (www.cran.r-project.org). All the p-values were corrected for multiple comparisons by the Bonferroni method. The Chi-square test of independence of the package “Stats” was used to compare proportion where applicable and mortality rates using R software. All the analyses were set at a significance threshold of p < 0.05. Graphics were built using GraphPad Prism 8.0.2 software (San Diego, California USA).
A total of four hundred and eighty (480) Anopheles gambiae females of AcerKis (n= 240) and KisKdr (n= 240) were exposed to the insecticides. In control groups, female mortality rates were below 5% (2.5 ± 3.42% for AcerKis) (Figure 1A) except in the KisKdr strain (5 ± 4.77%) (Figure 1B).
Figure 1 Mortality and fecundity in An. gambiae strains following insecticide exposures. Upper panels show An. gambiae mortality rates in AcerKis (A) and KisKdr (B). Dichlorodiphenyltrichloroethane 4% (DDT 4%) and permethrin 0.75% (PMT 0.75%) exposure time was 3 hours; b endiocarb 0.1% (BDC 0.1%) and chlorpyriphos-methyl 0.4% (CM 0.4%) exposure times were 1 hour and 30 min respectively. All mortality rates were scored 24 hours later. Lower panels show egg production in the same strains: AcerKis (C) and KisKdr (D). Each dot represents the number of eggs laid by each female within each group of treatment in each mosquito strain. Only females that laid at least one egg were included for data analysis. Statistical differences were indicated: ns, no significant; ***p value < 0.01; *p value < 0.05. Median numbers of eggs are represented with interquartile ranges. The dots are offset horizontally where overlapping.
AcerKis resistance was confirmed to both bendiocarb (48.75 ± 10.95%) and chlorpyriphos-methyl (71.25 ± 9.92%) (Figure 1A). KisKdr resistance was also confirmed to DDT (2.63 ± 1.05%) and permethrin (42.11 ± 3.44%) (Figure 1B).
Females mosquitoes that survived the insecticide susceptibility tests were allowed to lay eggs individually after blood-feeding. AcerKis females laid significantly fewer eggs following exposure to chlorpyriphos-methyl with a median number of eggs per female of 49 eggs compared to that of bendiocarb exposed (82 eggs) and the control (51.5 eggs) mosquitoes (Kruskal-Wallis χ2 = 6.4374, df = 2, p = 0.040) (Figure 1C). The percentage of AcerKis females that contributed to the oviposition was higher in bendiocarb exposed (26.83%; CI95%= [14.22 – 42.99]) and in chlorpyriphos-methyl exposed females (21.74%; CI95%= [7.46% – 43.70%]). However, there was no significant difference compared to their control (15.38%; CI95%= [8.21 – 25.33]) (Chi-square χ2 = 2.2944, df = 2, p = 0.318) (Figure 2, Table 1).
Figure 2 Percentage of females that contributed to the oviposition in each mosquito strain. Dichlorodiphenyltrichloroethane 4% (DDT 4%); permethrin 0.75% (PMT 0.75%); bendiocarb 0.1% (BDC 0.1%) and chlorpyriphos-methyl 0.4% (CM 0.4%). ns, non significant.
In KisKdr females, were more eggs in individuals who survived DDT exposure (Figure 1D). However, no significant difference was observed in the median number of eggs within the different groups (50, 78, 63.5 eggs per female respectively in control, DDT and permethrin exposures; Kruskal-Wallis χ2 = 3.439, df = 2, p = 0.179) (Figure 1D). Besides, there was no difference in the percentage of the females that contributed to the oviposition (control: 9.21%, CI95%= [3.79 – 18.06]; DDT 4% exposure: 13.51%, CI95%= [6.68 – 23.45]; permethrin 0.75% exposure: CI95%= [11.47 – 37.84]; Chi-square χ2 = 4.265, df = 2, p = 0.118) (Figure 2, Table 1).
The egg batches collected from AcerKis and KisKdr females that contributed to the oviposition were allowed to hatch, and the number of larvae was counted. A significant difference in the median number of larvae was observed in AcerKis females following bendiocarb (65 larvae) and chlorpyriphos-methyl (22 larvae) exposure compared to the control females (26 larvae) (Kruskal-Wallis χ2 = 6.9321, df = 2, p = 0.031) (Figure 3A). However, there was no significant difference in the hatching rates in the exposed females (67.47 ± 20.42% for bendiocarb and 36.32 ± 16.1% for chlorpyriphos-methyl) compared to the control females (46.92 ± 35.47% (Figure 3C).
Figure 3 Fertility and hatching rate in An. gambiae strains following insecticide exposure. Upper panels show the number of larvae per egg batch in AcerKis (A), and KisKdr (B). Each dot denotes the number of larvae hatched from individual female egg batch within each group of treatment in each strain. Lower panels show the mean larval hatching rates in the same mosquito strains: AcerKis (C) and KisKdr (D) *p value <0.05; ns, non significant.
The results showed a slight increased fertility in KisKdr females exposed to DDT (median larvae per female of 81.91) compared to their control (median larvae per female of 57.89) (Kruskal-Wallis χ2 = 4.221, df = 1, p= 0.039) (Figure 3B). As illustrated in Figure 3D, the hatching rates were also slightly different within the groups: 60.54%, CI95% = [44.32 – 76.76] for the control; 77.81%, CI95% = [68.28 – 87.34] for DDT exposure; and 73.66%, CI95% = [63.73 – 83.60] for permethrin exposure (Kruskal-Wallis χ2 = 6.355, df = 2, p = 0.042).
To date, vector control through the use of insecticides to impregnate mosquito bed nets and dwelling spraying remains the strategy that has significantly contributed to the decline of the malaria burden (2). Nevertheless, due to the increasing insecticide resistance phenomenon, resistant mosquitoes are not instantly killed after their contact with the treated bed nets. However, vectorial capacity could be decreased because of the longer-term impacts of insecticide residues on the mosquito life-history traits that continue after exposure to the impregnated nets (14). The current work investigated the effects of organochlorine (DDT 4%), carbamate (bendiocarb 0.1%), organophosphate (chlorpyriphos-methyl 0.4%), and pyrethroid (permethrin 0.75%) insecticides exposure on Anopheles gambiae s.s. fecundity and fertility.
Regarding KisKdr female exposure to permethrin, no significant impact was observed on the fecundity and fertility in this strain. Additionally, only a slight difference in the hatching rate was noticed. These observations imply that permethrin and bendiocarb exposure does not directly affect the reproductive success of the An. gambiae mosquitoes carrying kdrR allele. This knowledge could guide the choice of insecticide compounds when implementing the management programmes of resistant An. gambiae mosquitoes to prevent the rapid loss of the effectiveness of the insecticides currently available for vector control. However, the insecticide doses used in our study are the discriminating doses recommended by the WHO (21). They are lower than those used for indoor residual spraying and mosquito nets impregnation. Then, it will be interesting to investigate the effect of direct and long-term exposure to the impregnated mosquito nets on reproductive success using wild-resistant An. gambiae mosquitoes. Indeed, Mulatier et al. (22) have already reported that exposure to the bed nets impregnated with permethrin at the WHO recommended dose did not affect fecundity, fertility, or survival of An. gambiae laboratory colony carrying the kdrR allele. It is suitable for further works to investigate the long-term effects of insecticides on resistant mosquitoes regarding other life-history traits critical for mosquito vectorial capacity.
Surprisingly, we observed that female mosquitoes carrying the insensitive acetylcholinesterase allele (ace-1R) that survived after being exposed to chlorpyriphos-methyl laid significantly fewer eggs. This result indicates that the organophosphate compound used might negatively impact the fecundity of homozygous AcerKis female mosquitoes. This would mean that the insecticide hinders either egg development or the ability to lay eggs normally in this mosquito strain. Thus, we assume that the chlorpyriphos-methyl exposure may affect the expression level of key genes involved in egg production and development, such as the Mating Induced Stimulator of Oogenesis (MISO) (23). Further investigations are needed to understand better which process has led to the egg-laying disruption when exposed to insecticide compounds.
Furthermore, although AcerKis females show resistance to chlorpyriphos-methyl, its impact on the mosquito’s fecundity indicates that this insecticide could reduce the vector density (even if the females were not immediately dead) in the long term, reducing malaria transmission. Indeed, natural populations of Anopheles gambiae s.l. were already susceptible to pirimiphos-methyl (24) and other organophosphate compounds such as malathion (25, 26) in some African countries. Moreover, wild populations of Anopheles coluzzii, a sibling species of An. gambiae, have also been shown to be fully susceptible to fenitrothion and pirimiphos-methyl (27).
The presence of alleles that confer insecticide resistance in mosquitoes is correlated to the changes in physiological processes, which drive vector fitness disadvantages (28). For instance, Alout et al. (12), have reported that exposure to pyrethroid insecticides reduced the prevalence and intensity of Plasmodium falciparum infection in Anopheles gambiae s.s. carrying kdrR and ace-1R alleles. It was also reported that P. falciparum infection decreases the resistant vector survivorship following insecticide exposure (29). Exposition to pyrethroid insecticide has also been shown to reduce parity in resistant Anopheles gambiae and Anopheles coluzzii mosquitoes (30). Putting all these observations together, it has become evident that the interaction between insecticide exposure and the mosquitoes’ life-history traits can represent a critical aspect of vectors biology that may impact the pattern of malaria parasites transmission and the outputs of vector control measures.
The raw data supporting the conclusions of this article will be made available by the authors, without undue reservation.
AM, and LSD conceived the study, designed the experiments and revised the manuscript. EBJS, EGS, EA and LD performed the experiments. OD acquired, analysed the data and drafted the manuscript. All authors read and approved the final manuscript.
The authors thank Wellcome Trust for the financial support to AM through the intermediate fellowship in public health and tropical medicine (grant n°109917/Z/15/Z) awarded to LSD.
The authors declare that the research was conducted without any commercial or financial relationships that could be construed as a potential conflict of interest.
All claims expressed in this article are solely those of the authors and do not necessarily represent those of their affiliated organizations, or those of the publisher, the editors and the reviewers. Any product that may be evaluated in this article, or claim that may be made by its manufacturer, is not guaranteed or endorsed by the publisher.
1. World Health Organization. The Top 10 Causes of Death (2018). Available at: https://www.who.int/news-room/fact-sheets/detail/the-top-10-causes-of-death (Accessed July 06, 2020).
2. Bhatt S, Weiss D, Cameron E, Bisanzio D, Mappin B, Dalrymple U. The Effect of Malaria Control on Plasmodium Falciparum in Africa Between 2000 and 2015. Nature (2015) 526(7572):207–11. doi: 10.1038/nature15535
3. World Health Organization. World Malaria Report 2019 (2019). Available at: https://www.who.int/publications/i/item/world-malaria-report-2019 (Accessed April 10, 2020).
4. Ranson H, N’guessan R, Lines J, Moiroux N, Nkuni Z, Corbel V. Pyrethroid Resistance in African Anopheline Mosquitoes: What are the Implications for Malaria Control? Trends Parasitol. (2011) 27(2):91–8. doi: 10.1016/j.pt.2010.08.004
5. Djogbénou L, Pasteur N, Akogbéto M, Weill M, Chandre F. Insecticide Resistance in the Anopheles Gambiae Complex in Benin: A Nationwide Survey. Med Vet Entomol. (2011) 25(3):256–67. doi: 10.1111/j.1365-2915.2010.00925.x
6. Strode C, Donegan S, Garner P, Enayati AA, Hemingway J. The Impact of Pyrethroid Resistance on the Efficacy of Insecticide-Treated Bed Nets Against African Anopheline Mosquitoes: Systematic Review and Meta-Analysis. PloS Med (2014) 11(3):e1001619. doi: 10.1371/journal.pmed.1001619
7. Corbel V, N’Guessan R. Distribution, Mechanisms, Impact and Management of Insecticide Resistance in Malaria Vectors: A Pragmatic Review. In: Manguin S, editor. Anopheles Mosquitoes - New Insights Into Malaria Vectors (France: Sylvie Manguin) (2013).
8. Hemingway J, Shretta R, Wells TNC, Bell D, Djimdé AA, Achee N, et al. Tools and Strategies for Malaria Control and Elimination: What Do We Need to Achieve a Grand Convergence in Malaria? PloS Biol (2016) 14(3):e1002380. doi: 10.1371/journal.pbio.1002380
9. Collins E, Vaselli NM, Sylla M, Beavogui AH, Orsborne J, Lawrence G, et al. The Relationship Between Insecticide Resistance, Mosquito Age and Malaria Prevalence in Anopheles Gambiae s.L. From Guinea. Sci Rep (2019) 9(1):1–12. doi: 10.1038/s41598-019-45261-5
10. Salako AS, Ahogni I, Aïkpon R, Sidick A, Dagnon F, Sovi A, et al. Insecticide Resistance Status, Frequency of L1014F Kdr and G119S Ace-1 Mutations, and Expression of Detoxification Enzymes in Anopheles Gambiae (s.L.) in Two Regions of Northern Benin in Preparation for Indoor Residual Spraying. Parasit. Vectors (2018) 11(1):618. doi: 10.1186/s13071-018-3180-2
11. Lynd A, Oruni A, van’t Hof AE, Morgan JC, Naego LB, Pipini D, et al. Insecticide Resistance in Anopheles Gambiae From the Northern Democratic Republic of Congo, With Extreme Knockdown Resistance (Kdr) Mutation Frequencies Revealed by a New Diagnostic Assay. Malar. J (2018) 17(1):412. doi: 10.1186/s12936-018-2561-5
12. Alout H, Djègbè I, Chandre F, Djogbénou LS, Dabiré RK, Corbel V, et al. Insecticide Exposure Impacts Vector–Parasite Interactions in Insecticide-Resistant Malaria Vectors. Proc Biol Sci (2014) 281(1786):0389–95. doi: 10.1098/rspb.2014.0389
13. Chandre F, Darriet F, Duchon S, Finot L, Manguin S, Carnevale P, et al. Modifications of Pyrethroid Effects Associated With Kdr Mutation in Anopheles Gambiae. Med Vet Entomol. (2000) 14(1):81–8. doi: 10.1046/j.1365-2915.2000.00212.x
14. Viana M, Hughes A, Matthiopoulos J, Ranson H, Ferguson HM. Delayed Mortality Effects Cut the Malaria Transmission Potential of Insecticide-Resistant Mosquitoes. Proc Natl Acad Sci USA (2016) 113(32):8975–80. doi: 10.1073/pnas.1603431113
15. Lobo NF, Achee NL, Greico J, Collins FH. Modern Vector Control. Cold Spring Harb Perspect Med (2018) 8(1):a025643. doi: 10.1101/cshperspect.a025643
16. Goodyer LI, Croft AM, Frances SP, Hill N, Moore SJ, Onyango SP, et al. Expert Review of the Evidence Base for Arthropod Bite Avoidance. J Travel. Med (2010) 17(3):182–92. doi: 10.1111/j.1708-8305.2010.00402.x
17. Cook SM, Khan ZR, Pickett JA. The Use of Push-Pull Strategies in Integrated Pest Management. Annu Rev Entomol. (2007) 52:375–400. doi: 10.1146/annurev.ento.52.110405.091407
18. Paz-Soldan VA, Plasai V, Morrison AC, Rios-Lopez EJ, Guedez-Gonzales S, Grieco JP, et al. Initial Assessment of the Acceptability of a Push-Pull Aedes Aegypti Control Strategy in Iquitos, Peru and Kanchanaburi, Thailand. Am J Trop Med Hyg (2011) 84(2):208–17. doi: 10.4269/ajtmh.2011.09-0615
19. Djogbénou L, Weill M, Hougard JM, Raymond M, Akogbéto M, Chandre F. Characterization of Insensitive Acetylcholinesterase (Ace-1R) in Anopheles Gambiae (Diptera: Culicidae): Resistance Levels and Dominance. J Med Entomol. (2007) 44(5):805–10. doi: 10.1093/jmedent/44.5.805
20. Alout H, Ndam NT, Sandeu MM, Djégbe I, Chandre F, Dabiré RK, et al. Insecticide Resistance Alleles Affect Vector Competence of Anopheles Gambiae s.s. For Plasmodium Falciparum Field Isolates. PloS One (2013) 8(5):e63849. doi: 10.1371/journal.pone.0063849
21. World Health Organization. Test Procedures for Insecticide Resistance Monitoring in Malaria Vector Mosquitoes (2018). Available at: https://apps.who.int/iris/bitstream/handle/10665/250677/9789241511575-eng.pdf.
22. Mulatier M, Pennetier C, Porciani A, Chandre F, Dormont L, Cohuet A. Prior Contact With Permethrin Decreases its Irritancy at the Following Exposure Among a Pyrethroid-Resistant Malaria Vector Anopheles Gambiae. Sci Rep (2019) 9(1):8177. doi: 10.1038/s41598-019-44633-1
23. Baldini F, Gabrieli P, South A, Valim C, Mancini F, Catteruccia F. The Interaction Between a Sexually Transferred Steroid Hormone and a Female Protein Regulates Oogenesis in the Malaria Mosquito Anopheles Gambiae. PloS Biol (2013) 11(10):e1001695. doi: 10.1371/journal.pbio.1001695
24. Medjigbodo AA, Djogbenou LS, Koumba AA, Djossou L, Badolo A, Adoha CJ, et al. Phenotypic Insecticide Resistance in Anopheles Gambiae (Diptera: Culicidae): Specific Characterization of Underlying Resistance Mechanisms Still Matters. J Med Entomol. (2020) 58(2):730–8. doi: 10.1093/jme/tjaa195
25. Wanjala CL, Mbugi JP, Ototo E, Gesuge M, Afrane YA, Atieli HE, et al. Pyrethroid and DDT Resistance and Organophosphate Susceptibility Among Anopheles Spp. Mosquitoes, Western Kenya. Emerg Infect Dis (2015) 21(12):2178–81. doi: 10.3201/eid2112.150814
26. Fodjo BK, Koudou BG, Tia E, Saric J, N’dri PB, Zoh MG, et al. Insecticides Resistance Status of An. Gambiae in Areas of Varying Agrochemical Use in Côte D’ivoire. BioMed Res Int (2018) 2018:1–9. doi: 10.1155/2018/2874160
27. Poda SB, Soma DD, Hien A, Namountougou M, Gnankiné O, Diabaté A, et al. Targeted Application of an Organophosphate-Based Paint Applied on Windows and Doors Against Anopheles Coluzzii Resistant to Pyrethroids Under Real Life Conditions in Vallée Du Kou, Burkina Faso (West Africa). Malar. J (2018) 17:136. doi: 10.1186/s12936-018-2273-x
28. Alout H, Roche B, Dabiré RK, Cohuet A. Consequences of Insecticide Resistance on Malaria Transmission. PloS Pathog (2017) 13(9):e1006499. doi: 10.1371/journal.ppat.1006499
29. Alout H, Yameogo B, Djogbénou LS, Chandre F, Dabiré RK, Corbel V, et al. Interplay Between Plasmodium Infection and Resistance to Insecticides in Vector Mosquitoes. J Infect Dis (2014) 210(9):1464–70. doi: 10.1093/infdis/jiu276
Keywords: insecticide exposure, resistant Anopheles gambiae, fecundity, fertility, malaria
Citation: Medjigbodo A, Djihinto OY, Salavi EBJ, Sonounameto EG, Abbey E, Djossou L and Djogbénou LS (2022) Organophosphate Insecticide Exposure Impacts Reproductive Success in Insensitive Acetylcholinesterase Anopheles gambiae Mosquitoes. Front. Trop. Dis 3:903654. doi: 10.3389/fitd.2022.903654
Received: 24 March 2022; Accepted: 03 May 2022;
Published: 05 July 2022.
Edited by:
Josiane Etang, University of Douala, CameroonReviewed by:
William Hawley, Centers for Disease Control and Prevention (CDC), United StatesCopyright © 2022 Medjigbodo, Djihinto, Salavi, Sonounameto, Abbey, Djossou and Djogbénou. This is an open-access article distributed under the terms of the Creative Commons Attribution License (CC BY). The use, distribution or reproduction in other forums is permitted, provided the original author(s) and the copyright owner(s) are credited and that the original publication in this journal is cited, in accordance with accepted academic practice. No use, distribution or reproduction is permitted which does not comply with these terms.
*Correspondence: Oswald Y. Djihinto, b3N3YWxkZGppaGludG9Ab3V0bG9vay5mcg==
Disclaimer: All claims expressed in this article are solely those of the authors and do not necessarily represent those of their affiliated organizations, or those of the publisher, the editors and the reviewers. Any product that may be evaluated in this article or claim that may be made by its manufacturer is not guaranteed or endorsed by the publisher.
Research integrity at Frontiers
Learn more about the work of our research integrity team to safeguard the quality of each article we publish.