- 1Laboratoire de Recherche sur le Paludisme, Institut de Recherche de Yaoundé (IRY), Organisation de Coordination pour la lutte contre les Endémies en Afrique Centrale (OCEAC), Yaoundé, Cameroon
- 2Faculty of Sciences, University of Yaoundé I, Yaoundé, Cameroon
- 3Laboratory of Animal Biology and Physiology, University of Douala, Douala, Cameroon
- 4National Advanced School of Engineering, University of Yaoundé I, Yaoundé, Cameroon
- 5Faculty of Medicine and Pharmaceutical Sciences, University of Douala, Yaoundé, Cameroon
- 6Ecole des Sciences de la Santé, Université Catholique d’Afrique Centrale, Yaoundé, Cameroon
- 7Institute for Insect Biotechnology, Justus-Liebig-University Giessen, Giessen, Germany
Ecological upheavals resulting from uncontrolled urbanization can lead to significant changes in vector borne diseases’ profiles, thus requiring a thorough revision of their prevention and control strategies. The current study aimed at characterizing malaria vector populations in the Simbock neighborhood of Yaoundé-city (Cameroon), in relation to its urbanization scheme. Adult mosquitoes were captured by human landing catches (HLC) in- and outdoors prior to (2000–2006) and during infrastructural development (2014–2016). Anophelines were morphologically identified and analyzed for Plasmodium (P.) falciparum circumsporozoite protein detection using the ELISA technique. Species of the Anopheles (An.) gambiae complex were identified using SINE-PCR. Adult An. gambiae s.l. from larvae collected between 2014 and 2017 were tested for susceptibility to insecticides (0.1% bendiocarb, 4% DDT, 0.75% permethrin and 0.05% deltamethrin) with or without piperonyl butoxide (PBO) synergist, using WHO standard bioassays. The Hot Oligonucleotide Ligation Assay was used to detect the knockdown resistance (kdr) L995F/S mutations. Overall, nine malaria vector species were identified in 2000-2006, mostly An. moucheti (49%), An. nili (13.5%) and An. gambiae s.l. (12%); the six remaining species were represented at less than 3% each. However, only three species were found in 2014-2016, with increasing proportions of An. gambiae s.l. (67%) and An. funestus (32%) (P<0.0001). An. gambiae s.l. consisted An. coluzzii (> 85%) and An. gambiae (<15%) species during the two study periods. Plasmodium falciparum infection rates were 2.1% and 1.0% in 2000-2006 and 2014-2016 respectively (P=0.4), with decreasing entomological inoculation rates (EIR) from 0.34 infective bites per man per night (ib/m/n) to 0.02 ib/m/n (P<0.0001). Anopheles gambiae s.l. was resistant to DDT and permethrin [<40% mortality rates (MR)], and deltamethrin (65-89% MR), but fully susceptible to bendiocarb (100% MR). Pre-exposure of mosquitoes to PBO resulted in 90-100% MR to deltamethrin but not to permethrin. Furthermore, the two kdr L995F/S resistance alleles were recorded at 0.64 and 0.006 frequencies respectively. This study highlights a shift from rural to urban malaria transmission in Simbock, coupled with DDT and pyrethroid resistance in An. gambiae s.l. Combination vector control interventions, e.g., PBO nets and bendiocarb indoor residual spraying are needed in such areas.
Introduction
African cities experience a rapid demographic expansion as a result of various factors among which rural to urban migration, improved life expectancy, high birth rates and developmental projects (1). In order to accommodate the fast-growing human populations, rural areas surrounding the cities have turned to urban neighborhoods. These new neighborhoods are characterized by uncontrolled habitat constructions and landscaping activities, leading to important environmental changes.
In general, environmental modifications occurring naturally or through human activities, disrupt the ecological balance thus creating new habitats for living organisms (2). These upheavals have deep consequences on vector biology whereby some competent species may emerge to the detriment of the others, thus influencing the transmission profile of vector-borne diseases (3). Previous studies have revealed deep changes in the epidemiology of vector-borne diseases (VBDs) such as dengue, onchocerciasis, and particularly malaria (4). Malaria is a major public health concern in Africa. From 2019 to 2020, the incidence of malaria cases increased from 213 million to 228 million, highlighting the need to intensify prevention and control efforts (5). Cameroon is among the eleven countries mostly affected in the world (5) with about eighteen anopheline species involved in the transmission of Plasmodium parasites. Major vector species are An. gambiae, An. coluzzii, An. arabiensis, An. funestus, An. nili, An. moucheti known for their high anthropophilic behaviour (6–9). Other species considered as secondary vectors include An. carnevalei, An. coustani, An. hancocki, An. leesoni, An. marshallii, An. melas, An. paludis, An. pharoensis, An. ovengensis, An. wellcomei, An. rufipes, and An. ziemanni (10–15). Some of these vectors exhibit the ability to adapt to changing environmental conditions (16–18), even though each of them shows ecological preferences. For example, An. coluzzii which has a strong preference for unpolluted waters (19) has been found breeding in highly polluted water sources after urbanization in several countries in Africa (20–22), moreover, Mbakop et al. (23) showed the emergence of An. paludis, responsible for the majority of malaria transmission in Nyabessan, Cameroun during the construction of the hydroelectric dam.
Malaria has generally been considered as a disease of rural areas, but many factors linked to a rapid and uncontrolled urbanization are increasing malaria transmission in cities across Africa (24, 25). The expansion of malaria transmission to urban areas is of particular concern to malaria control programs since populations in these areas are likely to be at higher risk of the development of severe malaria due to the lack of protective immunity and the spread of drug resistant Plasmodium strains.
In Yaoundé, malaria transmission is considered holo-endemic and seasonal, with An. gambiae as the main vector (22, 26). Average annual prevalence of P. falciparum in the general population is estimated to increase from 34% in the city centre to 50% in the periphery (27). Malaria prevention is essentially based on vector control. Chemical vector control in the Yaoundé city began in 1950s with DDT indoor spraying campaigns; then, in 1990 there was the distribution of impregnated mosquito nets with deltamethrin, in a few households (28). Later on, three other mass LLIN distribution campaigns were conducted in 2004, 2011 and 2015 (29, 30). In addition, to remedy the increase in culicidian nuisance induced by uncontrolled urbanization (31), the populations of Yaoundé also report the use of spirals, aerosol bombs, and mosquito repellent ointments (32). Following these interventions, bed net ownership in Yaoundé was estimated at 73.8% (33), with subsequent reduction of malaria incidence. However, since 2017, the country is witnessing the resurgence of malaria with a rise of cases and deaths up to 6612000 and 14448 respectively in 2021 (34), thus requiring a « High Burden to High Impact » approach. Within this approach, the arsenal for malaria prevention includes the promotion of ITN use, intermittent preventive treatment for pregnant women and seasonal malaria chemoprevention for children aged 3–59 months in the humid tropical regions.
Indeed, during the last two decades, Cameroon has been facing anarchic urbanization, with the extension of cities to their outskirts. This extension leads to a demographic explosion couple with insalubrity and increased nutritional needs. This situation results in permanent stagnant waste water and crop cultivation in urban lands (35, 36). The Simbock neighborhood in the city of Yaoundé is a perfect illustration of this phenomenon. Previous studies conducted between 1999 and 2009 has revealed deep changes in the epidemiology of malaria following the emergence of highly anthropophilic vectors such as An. gambiae s.l. and An. funestus (37–41). The most recent malaria entomological study in Simbock was from 2000 to 2001 (41), while the habitat and the ecology of the environment have deeply changed over time. Moreover, the susceptibility of emerging vector species to insecticides was not assessed. As such, a better understanding of the malaria entomological profile in such area under modification could improve and contextualize the implementation of new vector control interventions.
The current study aimed at assessing the entomological parameters of malaria transmission and the susceptibility of malaria vectors to insecticides in Simbock, a former rural area turned to a neighborhood of the Yaoundé, the capital city of Cameroon.
Materials and Methods
Study Design
This study included retrospective and prospective surveys of malaria transmission indicators and four annual cross-sectional surveys of An. gambiae s.l. susceptibility to insecticides from 2014 to 2017. Retrospective data (2000–2006) were retrieved from previous entomological surveys stored in a mosquito data base in the malaria research laboratory at Organisation de Coordination pour la lutte contre les Endémies en Afrique Centrale (LRP/OCEAC), while prospective data (2014–2016) were collected through surveys according to thesame data collection scheme of retrospective data.
Study Area
The study was carried out in Simbock (3° 50’N, 11° 30’E, 750 m) located about 10 km from the divisional head quarter of Yaoundé, the capital city of Cameroon (Figure 1A). The climate in this area is of equatorial type characterized by two dry seasons (December-February and July-August) and two rainy seasons (March-June and September-November). The average annual temperature is 23°C and the mean annual rainfall 1,727 mm (42). Simbock is watered by the Mefou river, exhibiting in its course swamps which constitute permanent breeding sites of mosquitoes. Furthermore, there are some artificial fish ponds.
Before the 2000s, this locality had a rural landscape with the rate of built-up land (RBL) < 40%. Houses were surrounded by the forest, built with mud, roofed with straw, without ceiling. The main track through the village was unpaved (Figure 1B). At that time, the mean entomological inoculation rate was 0.69 infected bites per man per night (ib/m/n), and the plasmodial species present were P. falciparum and P. ovale (41). In order to facilitate the traffic between Douala the economic capital and Yaoundé the political capital of the country, a road construction project via Simbock was launched in 2008. Following this project, this locality has been experiencing overcrowding from 300 inhabitants in 2002 (39) to around 10,000 inhabitants in 2021 (43) and anarchic housing multiplication with conditions commonly better than those of the retrospective period (Figure 1C). Most houses are built with concrete floors and walls, ceiling under the roof and screened windows. Socio-economic development has been accompanied by the poor water drainage, responsible for the creation of permanent breeding sites characteristic for the proliferation of mosquitoes of the genus Culex. Vegetable crops, often responsible for the creation of anopheline breeding sites, are not practiced there; but there are ponds that already existed and are in the process of eutrophisation because they are poorly maintained. Most anophelines breeding sites are temporary and are numerous during the rainy season. Most of the inhabitants work in the city center or in the shops built along the main axis.
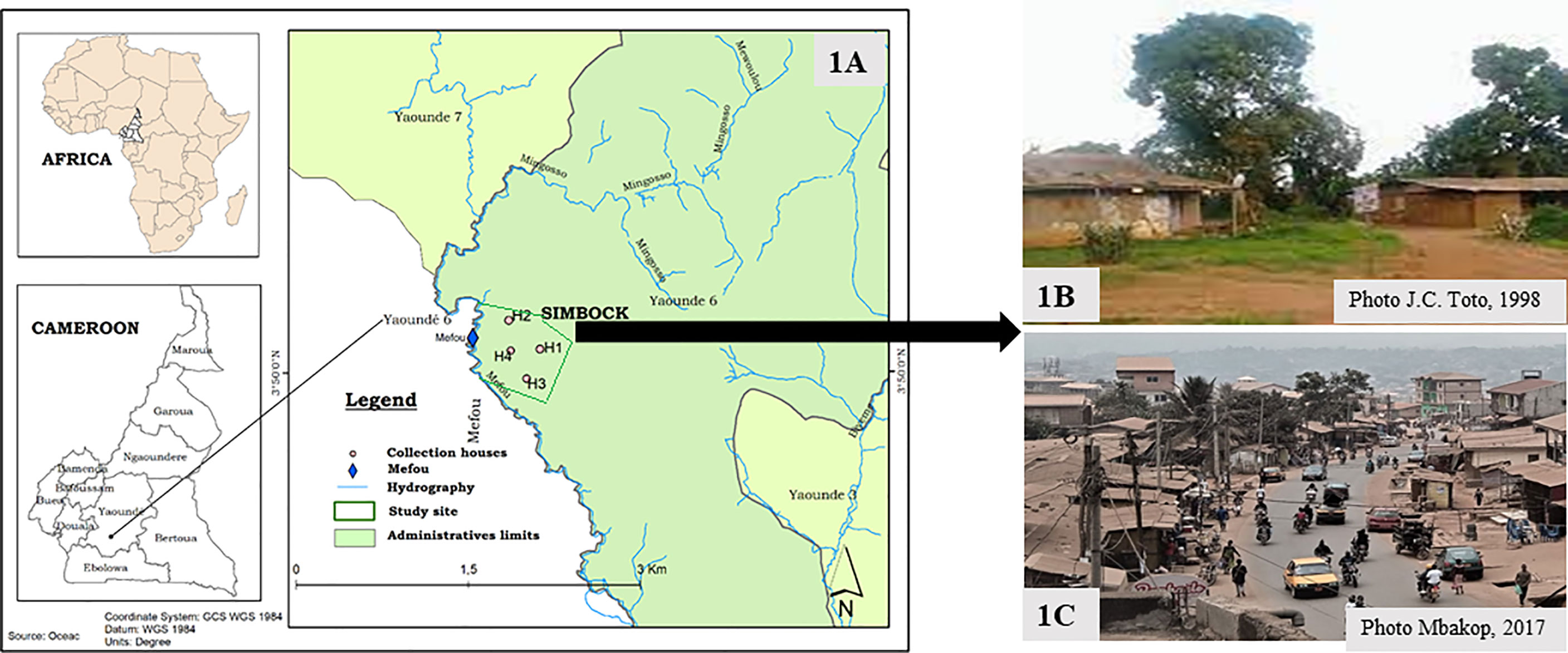
Figure 1 Map of Yaoundé subdivisions showing Simbock and his configuration before the 2000s and following the urbanization process. (A): Map of Yaoundé subdivisions showing Simbock, (B): Before urbanization, (C): Following the urbanization process, H1-H4: Mosquito collection houses.
Study Periods
This study included retrospective and prospective surveys of malaria transmission indicators and four annual cross-sectional surveys of An. gambiae s.l. susceptibility to insecticides from 2014 to 2017. Details on the periodicity of the adults and larval collections are summarized in Table 1. Retrospective data were drawn from the malaria entomological data base resulting from a study conducted by the “Laboratoire de Recherche sur le Paludisme” (LRP) of Organisation de Coordination pour la lutte Contre les Endémies en Afrique Centrale (OCEAC) between January 2000 and November 2006. Prospective data on malaria transmission were collected between 2014 and 2016.
Mosquito Collection and Morphological Identification
Adult mosquitoes were collected via human landing catches (HLC) both during the retrospective (2000–2006) and the prospective period (2014-2016). Mosquito collections were performed as described in (14). In 2000-2006, mosquito collections were performed in two to four households (collection points) randomly selected, for two consecutive nights per survey period, including 14 surveys in total (7 surveys in 2000, 4 in 2001, 2 in 2004 and 1 in 2006) (Table 1). In 2014-2016, mosquitoes were collected in 3 households selected among those of the retrospective period, also for two consecutive nights per period of survey, including 3 surveys in all (1 survey per year). The surveys were cross-sectional whether during the retrospective or the prospective period, but covered the 2 dry seasons and the 2 rainy seasons. Mosquito collections were carried out per night by 12 to 34 volunteers during the retrospective period and 12 to 18 volunteers during the prospective period, working in two teams, the first team captured adult mosquitoes from 19:00 to 01:00 and the second team collected from 01:00 to 05:00. At each selected house, one volunteer captured indoors while another captured outdoors. To avoid bias, the volunteers rotated between indoors and outdoors every two hours. Mosquitoes collected were placed into separate bags, labelled according household, position and time of collection; a supervisor collected the bags at the end of each hour of catches.After collections, mosquitoes were morphologically identified using the standard identification keys (44, 45) and individually stored in labeled 1.5 mL Eppendorf tubes containing silica gel desiccant for further analysis.
For insecticide resistance assessment, anopheline larvae and pupae samples were collected from water bodies, including puddles disseminated throughout the quarter, gutters and pools of water strewing the Mefou river. Samples were pooled and transferred at the OCEAC Malaria Research insectary for rearing. Mosquitoes were reared in the laboratory at optimal temperature (28–30°C) and relative humidity (70–80%).
Laboratory Analyses and Testing
Each collected adult mosquito was dissected into two parts, the head-thorax region and carcasses.
Plasmodium falciparum Detection
Anopheline head and thorax were used for the detection of P. falciparum circumsporozoite protein (CSP) using the ELISA CSP technique (46, 47).
Molecular Identification of Species
Carcasses (legs/wings/abdomen) were used for DNA extraction as described by Collins et al. (48). PCR was then performed on the DNA template using An. gambiae s.l. species diagnostic primers in a total reaction mix of 25 μl, according to the protocol of Santolamazza et al. (49). The PCR product was visualized on 1.5% agarose gel to determine the sibling species.
Insecticide Susceptibility Tests
Female adult mosquito resulting from larval collections and aged 2-5 days were morphologically identified as An. gambiae s.l (44, 45). and used for susceptibility tests. Other susceptibility tests were carried out with female mosquitoes of the Kisumu susceptible reference strain of An. gambiae, reared in the OCEAC medical entomology laboratory since more than 20 years.
Susceptibility tests were carried out according to the WHO standard protocol for adult mosquitoes using insecticide discriminating dosage on filter papers (50). Insecticide impregnated filter paper sheets (Whatman N°1, 12 cm x15 cm) were purchased from the WHO reference center at the Vector Control Research Unit, University Sains Malaysia. These papers were impregnated with discriminating dosages of insecticides: 0.05% deltamethrin, 0.75% permethrin, 4% DDT and 0.1% bendiocarb. The preparation of stock solution for PBO synergist and impregnation on filter papers (12 cm × 15 cm) were performed by our research unit, in the LRP/OCEAC.
Two types of susceptibility test were performed; test with synergist (PBO) and test without synergist. Tests with synergist were carried out to assess possible implication of monoxygenase-based metabolic resistance (P450); for this purpose, mosquitoes were exposed for 1 h to 4% piperonyl butoxide (PBO) synergist, prior to exposure to insecticides. All the tests were conducted under ambient room temperature (25 ± 3°C) and relative humidity (70 ± 10%). For each test, four batches of 20–25 mosquitoes were exposed to insecticide impregnated paper; and one-two batches of 20–25 mosquitoes used as control were exposed to papers impregnated with silicon oil, i.e., without insecticide. During exposure to insecticides, the number of mosquitoes knocked down was recorded at 5-minute intervals. After 1 h exposure, mosquitoes were transferred to holding tubes and then fed with a 10% sugar solution. The mortality rates were determined 24h post exposure.
Dead, survivor and control mosquitoes were individually kept in Eppendorf tubes with silica gel and stored at −20°C for molecular analyses.
The resistance status was evaluated according to the WHO criteria (50), which classify mortality rates less than 90% as indicative of resistance and those greater than 98% as indicative of susceptibility. Mortality rates between 90–98% suggest the possibility of resistance that needs to be verified.
Kdr L995F/S Genotyping
Two alleles at the kdr 995 locus were genotyped in mosquitoes that have survived exposure to insecticides and identified down to species as described by Santolamazza et al. (49). Their total DNA was analyzed to detect the kdr L995F/S mutations using hot oligonucleotide ligation assay (HOLA) as described by Lynd et al. (51). Extracted DNA was used for kdr status characterization using PCR method (52) then, the genotypes were determined by a hot ligation reaction with detector and reporter oligonucleotides. The kdr L995F/S genotypes were determined using a colorimetric test on microplates previously treated with streptavidin (51).
Statistical Analysis
The Human Biting Rates, the Infection Rates (IR) and the Entomological Inoculation Rates (EIR) were calculated for both retrospective and prospective data. The proportions of each species before and following the urbanization process were compared using theChi square test for equality of proportions. The average HBR, IR, and EIR at 95% confidence interval were compared using the ANOVA test. These analyses were done on R 3.5.0 software (R Development Core Team, Vienna, Austria, 2018).
The knockdown times for 50% and 95% of tested mosquitoes (kdt50 and kdt95) were estimated using a log-time probit model (53), performed with WIN DL (version 2.0, 1999) software. The kdt50 recorded from field-collected mosquitoes were compared with that of the Kisumu reference susceptible strain of An. gambiae by estimates of kdt50 Ratios (kdt50R). kdt50 Ratio >2 fold compared with the Kisumu reference susceptible mosquito strain indicates a significant increase of knock-down times (54). The reversion of knockdown times by PBO was estimated as described by Thomas et al. (55) using the formula Reversion of Knockdown time = (1– (kdt50 PBO + insecticide/kdt50 insecticide)) x 100.
The mortality rate in the control sub sample (i.e., exposed to silicon oil impregnated papers) was 0-3%, therefore, Abbot’s correction was not necessary during data analysis. The mortality rates of mosquitoes tested with insecticides alone were compared to that of specimens pre-exposed to PBO by means of a Chi square Mantel Haenszel test.Allelic and genotypic frequencies at the kdr 995 locus were calculated using Genepop online Version 4.5.1 (56).
Ethical Approval and Consent to Participate
The study was conducted under the ethical clearance no. 2016/01/685/CE/CNERSH/SP delivered by the Cameroon National Ethics (CNE) Committee for Research on Human Health. All volunteers participating in human landing catches signed a written informed consent form indicating their willingness to take part in the study. They also received free malaria prophylaxis.
Results
Composition and Abundance of Anopheline Fauna
A total of 5,341 adult female anophelines were captured in Simbock during the two study periods, including 5,240 by 316 volunteers during the retrospective period (2000–2006) (12-34.5 person–night/month), and 101 by 42 volunteers during the prospective period (2014-2016) (12-18 person–night/month). The distribution of anopheline species in both study periods is shown in Table 2.
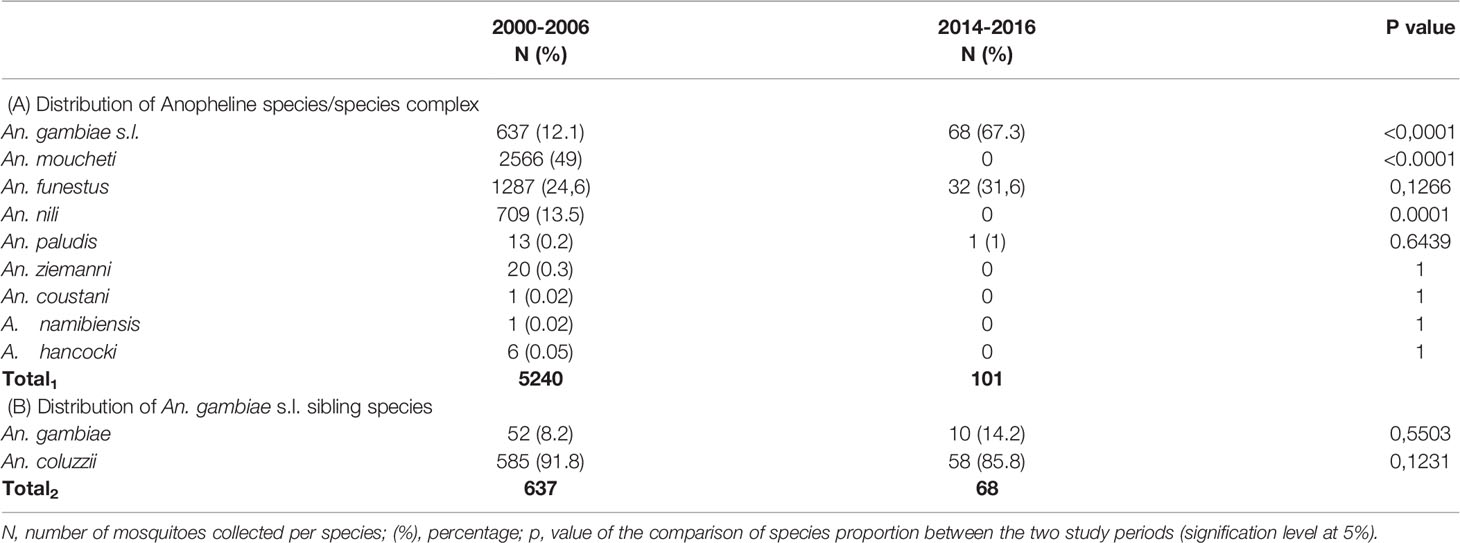
Table 2 Distribution of the anopheline species/species complex (A) and the An. gambiae s.l. sibling species in Simbock (B).
During the retrospective period, nine mosquito species/complex species were morphologically identified among the collected anopheline samples, namely An. gambiae s.l., An. moucheti, An. funestus, An. nili, An. paludis, An. ziemanni, An. coustani, An. namibiensis and An. hancocki. However, during the prospective period, only three anopheline species/complex species were identified; these include, An. gambiae s.l., An. funestus and An. paludis.
Between years 2000 and 2006 (retrospective period), An. moucheti was the major species (49%), followed by An. funestus (24.6%), An. nili (13.5%) and An. gambiae s.l. (12%); the six remaining species were represented at less than 3% each. By contrast from 2014 to 2016 (prospective period), An. moucheti and An. nili were not found in the collected mosquito samples; An. funestus remained the second most abundant species, with a non-significant increase in proportion from 24.6 in 2000-2006 to 31.6 in 2014-2016 (p= 0.12).The proportions of An. gambiae s.l. rather increased from 12% in 2000-2006 to 67% in 2014-2016 periods (p <0.0001), setting up this species complex as the major malaria vectors in Simbock.
Molecular identification carried out on 705 anophelines morphologically identified as belonging to the An. gambiae s.l. complex revealed the presence of two sibling species, An. gambiae and An. coluzzii. In general, the proportion of An. coluzzii was higher (> 85%) than that of An. gambiae (<15%), and no significant difference of the distribution of these two species was observed between the two study periods (p > 0.05).
Plasmodium falciparum Infections and Entomological Inoculation Rates
Overall, 3,619 female anophelines were analyzed by ELISA CSP for P. falciparum infection detection, including 3,518 collected in 2000-2006 and 101 in 2014-2016. Data on P. falciparum infection and entomological inoculation rates of anopheline species in the both study periods are shown in Table 3. The mean infection rates decreased from 2.1% in 2000-2006 to 1% in 2014-2016, though the difference was not significant (P=0.4). Likewise, the entomological inoculation rate decreased from 0.34 ib/m/n to 0.02 ib/m/n (P<0.0001) between the two study periods. Between years 2000 and 2006, four species were responsible for P. falciparum transmission, these include An. moucheti (IR=1.5, EIR=0.12 ib/m/n), An. funestus (IR=2.9, EIR=0.11 ib/m/n), An. gambiae s.l. (IR=3.9, EIR=0.07 ib/m/n) and An. nili (IR=1.8, EIR=0.03 ib/m/n). These species were involved in plasmodial transmission both indoors and outdoors, with EIRs ranging from 0.01 pi/h/n to 0.1 pi/h/n. Between years 2014 and 2016, no infective bite was recorded in An. funestus (in- and outdoors) as well as An. gambiae s.l. collected indoors. Only An. gambiae s.l. collected outdoors was found infected by P. falciparum (IR=1.5%, EIR=0.02 ib/m/n). There was no significant difference of outdoor EIRs of An. gambiae s.l. between the two study periods (p=0.6).
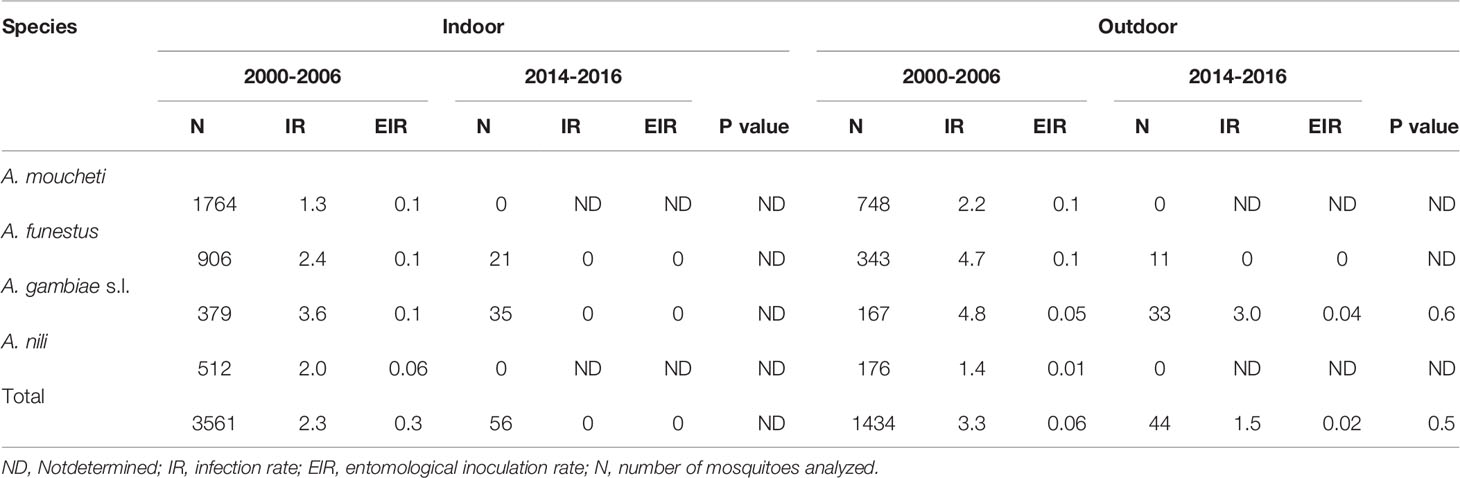
Table 3 Variations of malaria infection rates and entomological inoculation rates indoor and outdoor.
Susceptibility of An. gambiae s.l. to Insecticides
A total of 21 susceptibility tests were performed between 2014 and 2017, including 6 tests with mosquitoes of the reference susceptible strain Kisumu and 15 tests with wild mosquito samples. Among these, sixteen tests were conducted with insecticides only and five tests with insecticide-synergist combination whereby mosquitoes were exposed to 4% PBO synergist prior to pyrethroid insecticide susceptibility tests.
Knockdown and Mortality Rates of Anopheles gambiae s.l.
The Kisumu An. gambiae reference strain was found fully susceptible to deltamethrin, permethrin, DDT and bendiocarb, with 100% mortality rates. The kdt50 were less than 10 minutes to deltamethrin and permethrin with or without PBO and 19.1 minutes to DDT. The knockdown times for 95% mosquitoes (kdt95) varied from 14 to 31 minutes (Table 4).
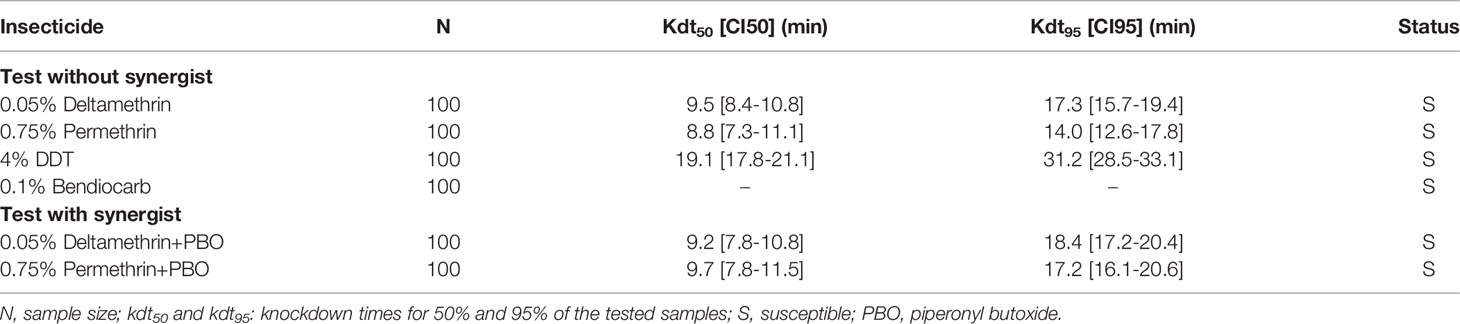
Table 4 Knockdown times of the Kisumu Anopheles gambiae s.s. reference strain to insecticides with or without PBO synergist.
The knockdown times for 50% (kdt50) and 95% (kdt95) mosquitoes tested with insecticides alone or in combination with PBO are given in Table 5, and mortality rates in Figure 2.
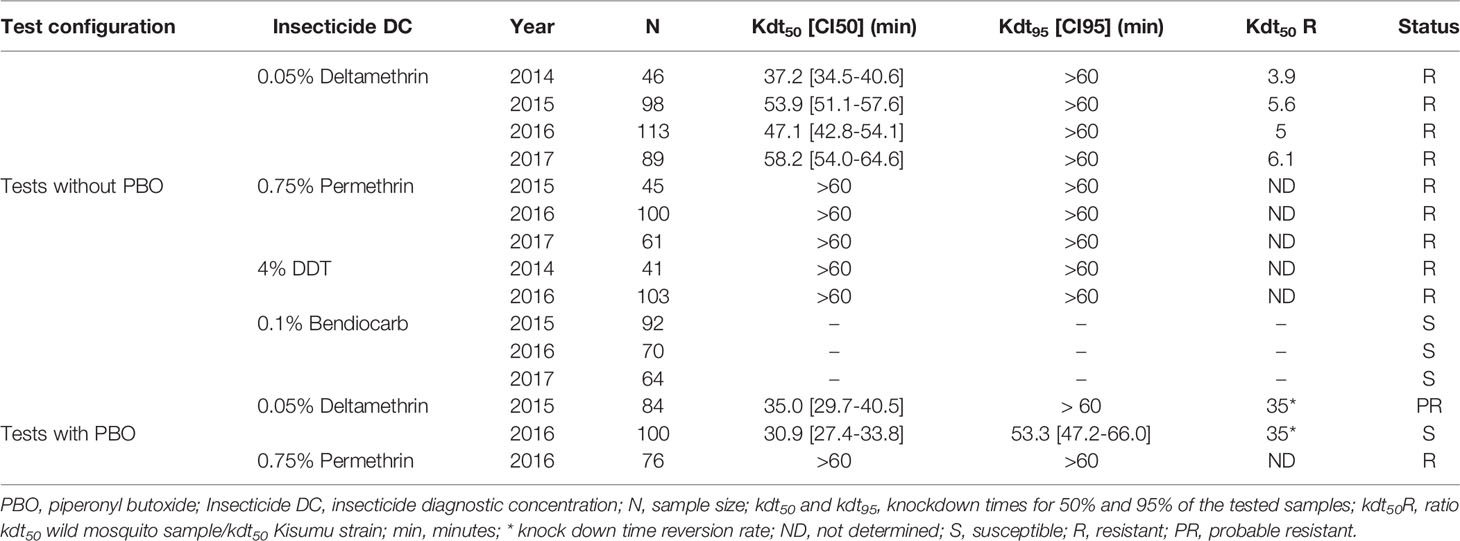
Table 5 Knockdown times and resistant status of wild Anopheles gambiae s.l. samples to insecticides with or without PBO synergist.
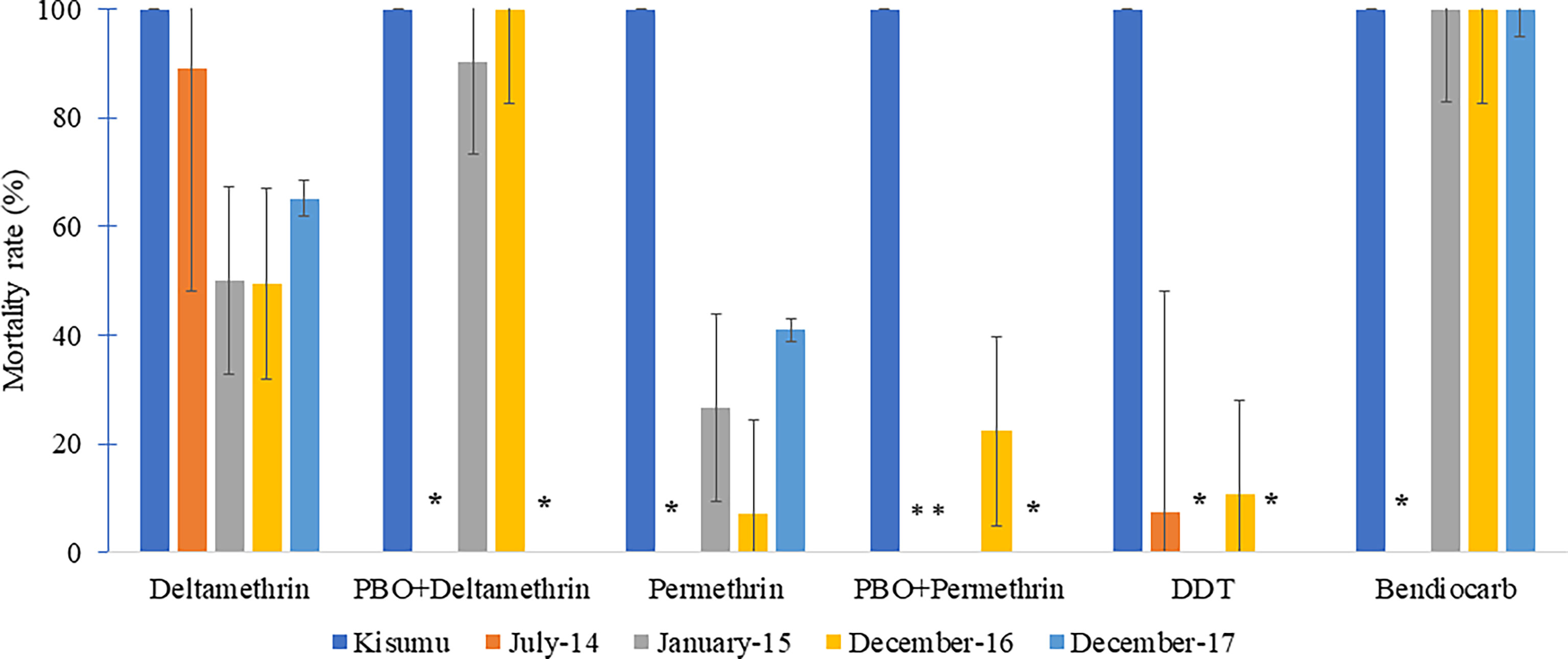
Figure 2 Mortality rates of An. gambiae s.l. of the Kisumu strain and field samples from Simbock to insecticides between 2014 and 2017. * Mortality rates not determined because the assay was not performed.
The wild An. gambiae s.l. samples regularly exhibited resistance to pyrethroids and DDT. Between 2014 and 2017, there was a significant increase of kdt50 to deltamethrin from 37 minutes to 58 minutes, with an upsurge of kdt50 ratios compared to the Kisumu reference strain from 3.9 in 2014 to 6.1 in 2017. The kdt50 to permethrin and DDT were always above 60 minutes (Table 5).
The mortality rates were 50-90% to deltamethrin, less than 40% to permethrin and less than 10% to DDT, with some variations from one year to another. However, this tested mosquito samples were fully susceptibility to bendiocarb, with mortality rate of 100% at each of the test period.
PBO Effect on An. gambiae s.l. Knockdown Times and Mortality Rates
Pre-exposure of wild mosquitoes to PBO resulted in 35% reversion of knockdown times with deltamethrin. However, the recorded kdt50 with permethrin remained greater than 60 minutes.
Mortality rates due to deltamethrin in combination with PBO synergist increased from 90% in 2015 to 100% in 2016, while the mortality rate recorded with permethrin remained lower than 30%. Tests with deltamethrin and permethrin combined with PBO were not performed in 2017 because of low sample size.
Kdr L995F/S Genotypic and Allelic Frequencies
A total of 80 mosquitoes randomly selected among those which survived exposure to pyrethroids, were genotyped for kdr 995F/S mutations. These mosquito samples were composed of An. coluzzii (N=72; 90%) and An. gambiae (N=8; 10%). The two kdr L995F and L995S alleles were found in An. coluzzii, while only the L995F allele was observed in An. gambiae (Table 6). Furthermore, there was a high genotypic diversity, with all possible genotypes being represented both in An. gambiae and An. coluzzii.

Table 6 Kdr L995F/S genotypic and allelic frequencies in wild An. gambiae and An. coluzzii samples which survived exposure to pyrethroid insecticides.
In the An. gambiae subsample, the kdr L995F allele was recorded at 70% frequency. The predominant genotype was L995F/L995F homozygote resistant (50%), followed by L995L/L995F heterozygote (37.5%) and L995L/L995L homozygote susceptible (12.5%).
In the An. coluzzii subsample, in addition to the kdr L995F allele found at a 60% frequency, the kdr L995S allele was also found, although at a heterozygote state with L995F in only one mosquito specimen (1% allelic frequency). The heterozygote L995L/L995F was the most represented (46%) genotype, followed by the homozygote resistant L995F/L995F (39%) and the homozygote susceptible L995L/L995L (14%).
The kdr L995F allelic frequencies were similar in both An. gambiae and An. coluzzii surviving subsamples (p= 0.5).
Discussion
The main objective of this work was to assess malaria transmission and vector susceptibility to insecticides in the Simbock neighborhood, following the environmental changes resulting from recent urbanization. Previous studies conducted in this locality between 1999 and 2009 have revealed the presence of six anopheline species, namely An. moucheti, An. funestus, An.gambiae s.l., An. nili, An. namibiensis and An. obscurus; with Plasmodium parasite transmission mainly carried out by An. moucheti, An. funestus (0.28-0.22 ib/m/n EIR), followed by An. gambiae s.l. and An. nili (0.13-0.06 ib/m/n EIR) (38, 39, 41). Data from the current study revealed that after a decade in which the urbanization process was accelerated in Simbock, the species distribution of anophelines has changed. Furthermore, An. gambiae s.l. resistance to pyrethroid insecticides is reported in this area for the first time. Indeed, we observed a decrease in the number of anopheline species from nine species recorded between years 2000 and 2006 to three species between 2014 and 2016. Furthermore, we have highlighted the persistence of Plasmodium sp. transmission in Simbock (0.02 ib/m/n) by An. gambiae s.l., which is now responsible for urban malaria in Simbock, as previously reported by Antonio et al. (39).
One limitation of this study is that, the number of entomological surveys conducted during the retrospective period (14 surveys during the four seasons of the year) was greater than that conducted during the prospective period (3 surveys during 3 seasons). Furthermore, during the prospective period, no survey was conducted during the short dry season. The small number of field surveys, the seasonal variations and bias in sampling sites could influence the outcomes of this study. It has been demonstrated that malaria transmission has a significant association with meteorological variables such as temperature, rainfall and humidity which play a major role in the life cycle of the malaria vectors and have been associated with their dynamics (57). More specifically, a recent study conducted in Cameroon in 2021 has revealed high anopheline densities and Plasmodium sp. parasite transmission during the short rainy season compared to the long rainy season (58). Therefore, the mosquito collections made during the two rainy seasons as well as the long dry season could allow to highlight the variations of the mosquito diversity associated with the urbanization process in Simbock. Indeed, the species composition and the infectivity of the anopheline fauna obtained during this prospective period are in agreement with previous findings in other neighborhoods of the Yaoundé city (22, 58). During the prospective period of the current study, the profile of malaria transmission in Simbock became that of urban malaria with increased densities of anthropophilic vectors such as An. gambiae and An. funestus and high feeding likelihood due to increased availability of human hosts following the demographic explosion.
Despite the small sample sizes used for EIR estimates, the presence of An. gambiae the major malaria vector in urban settings, is strongly suggestive of local Plasmodium sp. transmission (59). Furthermore, mosquito dispersal is much more limited in urban areas due to the higher housing density (60), causing urban malaria transmission to be highly focal (61). The destruction of vegetation due to land clearing along the Mefou River that crosses Simbock and deforestation may have led the disappearance of forest-dependent species such as An. moucheti, An. nili, An. namibiensis and An. hancocki which thrive in roosts with erect vegetation. The current predominance of An. gambiae s.l. in the Simbock neighborhood is in agreement with Ndoumbe et al. (62), who highlighted the role of species of the An. gambiae complex in malaria transmission across the most urban settings in Cameroon. The emergence of An. gambiae in Simbock could be explained by the migration of this species from the central neighborhoods to the periphery, attracted by the strong demographic pressure. Furthermore, An. gambiae is demonstrating a worrying trend of adaptation to polluted waters in urban environments (19). In recent years, this species has been found breeding in highly polluted water sources in Cote d’Ivoire (21) and Cameroon (22). In addition to An. gambiae s.l., An. funestus also appeared as an emerging species in the newly anthropized environment of Simbock, in agreement with Antonio et al. (40) and Munga et al. (63), despite the low abundance of these two species during the prospective period compared to the retrospective period. Ponds that were already present at Simbock during the retrospective period and that became poorly maintained during the prospective period, may have served as permanent or semi-permanent breeding sites for An. funestus. The low abundance of anopheline species during the prospective period could be partly due to many factors such as house construction with concrete floors and walls, ceiling under the roof and screened windows during the prospective period, insecticide-treated net (ITN) use, and other factors that influence human–vector contact such as environmental sanitation (64). Moreover, higher socioeconomic status through better education, higher exposure to TV and radio prevention campaigns, and increased ability to afford prevention methods, all contribute to increased vigilance of populations with regard to malaria vectors (29, 30, 65). Therefore, the intensification of vector control interventions could partly explain the lower anopheline abundance in Simbock during the prospective period. Indeed, the National Malaria control Programme has conducted two large-scale distributions of LLINs in 2011 and 2015, increasing the net ownership from 65,6% to 76,6% (66, 67). Moreover, Bamou et al. (68) showed that changes in mosquito bionomics and malaria transmission patterns were consistent with the influence of treated net use and the lack of bed net usage increased the risk of malaria infection 2.2-fold.
The distribution of sibling species of the An. gambiae s.l. complex remained unchanged between the two study periods, with An. coluzzii being the most represented species. The predominance of An. coluzzii in Simbock could be due to the presence of permanent breeding sites both before and after urbanization; which is consistent with previous studies (69, 70). It should be recalled that An. coluzzii is a species which colonizes urban environments (22, 26, 69); furthermore, this species is very likely to carry insecticide resistance genes, in particular the kdr L995F gene (34). Furthermore, the fact that this major malaria vector usually exhibits similar biting behavior indoors and outdoors, underlines the risk of contracting malaria both inside and outside dwellings. These data highlight the need for complementary vector control interventions on top of LLINs to control outdoors malaria transmission. Based on the fact that An. coluzzii develops in permanent roosts, larviciding operations which are under experimentation in the Yaoundé city (71) could be considered a potential complementary malaria vector control intervention in Simbock.
The insecticide susceptibility tests of An. gambiae s.l. carried out between 2014 and 2017 showed that An. gambiae s.l. from Simbock has developed resistance to DDT and pyrethroid insecticides, while remaining susceptible to bendiocarb. The frequencies of pyrethroid resistance recorded in Simbock are similar to those recently reported in other neighborhoods of Yaoundé, i.e., Nkolondom, Ekié and Nkolbisson, where market gardening is practiced throughout the year with uncontrolled use of insecticides (72). In the specific case of Simbock, theobserved resistance profile could be explained by the migration of An. gambiae species carrying the resistance alleles, from the central neighborhoods where market gardening is practiced to the periphery. Similar observations have already been made in other African countries (73, 74). To explore the role of target site mutations in the observed pyrethroid resistance, we conducted a preliminary screening of kdr 995F/L allele in mosquitoes which survived exposure to insecticides. Indeed, Donnelly et al. (75), asserted that the kdr L995F/F resistant homozygote genotype is correlated with DDT- and pyrethroid-resistance phenotype. Also, a strong correlation between high frequencies of kdr L995F allele and pyrethroid and DDT resistance was reported in several studies (76–78). Since the first report of resistance to insecticide in A. gambiae s.l. population in Cameroon (79, 80), the level of pyrethroid and DDT resistance has been increasing, alongside with the frequency of the L995F kdr allele (34, 81, 82).The kdr L995F allele was reported in this study at 70% frequency in An. gambiae and 62% in An. coluzzii. These high allelic frequencies of the kdr L995F were associated with high frequencies of homozygotes resistant and heterozygotes genotypes in both An. gambiae and An. coluzzii. These results are consistent with those reported in pyrethroid resistant populations of An. gambiae s.l. from others cities of Cameroon (54–56) and in West Africa (18, 52, 54, 83, 84). The resistant L995S allele was also found in An. coluzzii from Simbock, although at a very low frequency (1%). The scarcity of this allele in Cameroon has been reported in previous studies (80, 81). Santolamazza et al. (49) pointed out that the L995S mutation has a much more restricted geographic distribution in East Africa unlike the L995F mutation which is widespread in West and Central Africa. In the same vein, Nwane et al. (82) suggest that the L995S allele disperses from East to West and the L995F allele in the opposite direction. Although found at a low frequency, the kdr L995S mutation is already installed and continues to spread throughout Cameroon (85). Therefore, in order to assess the real frequencies of kdr L995F/S alleles in natural populations of An. gambiae and An. coluzzii from Simbock, further studies with representative sample sizes of randomly selected mosquitoes not exposed to insecticides are needed.
In the other hand, the presence of the susceptible homozygous L995L/L995L genotype in pyrethroid resistant mosquitoes suggest the involvement of alternative resistance mechanisms, or of some cofactors not yet identified (86). As such, we found an increase of mortality rates and 35% knockdown times reversion in mosquitoes pre-exposed to PBO synergist, suggesting the involvement of P450s’ detoxifying enzymes in tested An. gambiae s.l. resistant to deltamethrin. These results are consistent with previous studies conducted in the equatorial areas of Cameroon (32, 80, 82, 87). The lack of inhibitory effect of PBO on permethrin resistance suggests less involvement of P450s’ in the resistance to this insecticide. Such resistance could then be essentially linked to the “kdr” type mutations.
Given the fact that only survivor mosquitoes were genotyped for the kdr mutations and that very few susceptibility tests were carried out with PBO, a comprehensive study on the evolution of both kdr and metabolic resistance mechanisms is needed to assess the intensity and evolution of An. gambiae s.l. resistance to pyrethroid insecticides in Simbock. Nevertheless, tested mosquito samples remained full susceptible to bendiocarb; therefore, IRS with this insecticide may be advocated to complement LLINs in this area, in addition to bio larvicides.
Conclusion
This study highlights the occurrence of urban malaria transmission in Simbock, and describes for the first time, the profile of multiple pyrethroid resistance mechanisms in the local An. gambiae s.l. populations. It is therefore necessary to set up resistance management strategies based on an alternative vector control intervention, e.g., PBO LLINs, larviciding, housing IRS with bendiocarb.
Data Availability Statement
The original contributions presented in the study are included in the article/supplementary material. Further inquiries can be directed to the corresponding author.
Author Contributions
Conceptualization, JE and LM. Data curation, LM, JT and PA-A. Investigation and Methodology, LM, SM,WE and JT. Software, BF. Data analysis, LM, BF. Project administration, PA-A. Resources, PA-A. Supervision, AF and JE. Validation, PA-A, AF and JE. Writing—original draft, LM. Writing—review and editing, LM, WE, SM, MP, LA, HO, PA-A, PN, AF and JE. Supervision, JE. All authors contributed to the article and approved the submitted version.
Funding
This research was funded by the European Commission in the framework of INFRAVEC project, grant number 228421.
Conflict of Interest
The authors declare that the research was conducted in the absence of any commercial or financial relationships that could be construed as a potential conflict of interest.
Publisher’s Note
All claims expressed in this article are solely those of the authors and do not necessarily represent those of their affiliated organizations, or those of the publisher, the editors and the reviewers. Any product that may be evaluated in this article, or claim that may be made by its manufacturer, is not guaranteed or endorsed by the publisher.
References
1. Neiderud C-J. How Urbanization Affects the Epidemiology of Emerging Infectious Diseases. Inf Ecol Epid (2015) 5:127060. doi: 10.3402/iee.v5.27060
2. Patz JA, Graczyk TK, Geller N, Vittor AY. Effects of Environmental Change on Emerging Parasitic Diseases. Inter J Parasit (2000) 30(12-13):1395–405. doi: 10.1016/S0020-7519(00)00141-7
3. Hay SI, Guerra CA, Tatem AJ, Atkinson PM, Snow RW. Opinion Tropical Infectious Diseases: Urbanization, Malaria Transmission and Disease Burden in Africa. Nat Rev Microbiol (2005) 3(1):81–90. doi: 10.1038/nrmicro1069
4. Hunter JM, Rey L, Chu KY, Adekolu-John L, Mon KL. Parasitic Diseases in Water Resources Development. The Need for Inter Sectorial Negotiation. Genève, Switzerland: WHO (1993). 152p.
6. Ayala D, Costantini C, Ose K, Kamdem GC, Antonio-Nkondjio C, Agbor J-P, et al. Habitat Suitability and Ecological Niche Profile of Major Malaria Vectors in Cameroon. Malar J (2009) 8:307. doi: 10.1186/1475-2875-8-307
7. Atangana J, Bigoga JD, Patchoké S, Ndjemaï MN, Tabue RN, Nem TE, et al. Anopheline Fauna and Malaria Transmission in Four Ecologically Distinct Zones in Cameroon. Acta Tropica (2010) 115:131–6. doi: 10.1016/j.actatropica.2010.02.014
8. Tchuinkam T, Simard F, Lele-Defo E, Tene-Fossog B, Tateng-Ngouateu A, Antonio-Nkondjio C, et al. Bionomics of Anopheline Species and Malaria Transmission Dynamics Along an Altitudinal Transect in Western Cameroon. BMC Infect Dis (2010) 10:119. doi: 10.1186/1471-2334-10-119
9. Ekoko WE, Awono-Ambene P, Bigoga J, Mandeng S, Piameu M, Nvondo N, et al. Patterns of Anopheline Feeding/Resting Behaviour and Plasmodium Infections in North Cameroon, 2011–2014: Implications for Malaria Control. Parasit Vectors (2019) 12(1):1–12. doi: 10.1186/s13071-019-3552-2
10. Awono-Ambene HP, Kengne P, Simard F, Antonio-Nkondjio C, Fontenille D. Description and Bionomics of Anopheles (Cellia) Ovengensis (Diptera: Culicidae), A New Malaria Vector Species of the Anopheles Nili Group From South Cameroon. J Med entomol (2004) 41(4):561–8. doi: 10.1603/0022-2585-41.4.561
11. Tabue RN, Nem T, Atangana J, Bigoga JD, Patchoke S, Tchouine F, et al. Anopheles Ziemanni a Locally Important Malaria Vector in Ndop Health District, North West Region of Cameroon. Parasit Vectors (2014) 7(1):1–11. doi: 10.1186/1756-3305-7-262
12. Tabue RN, Awono-Ambene P, Etang J, Atangana J, Antonio-Nkondjio C, Toto JC, et al. Role of Anopheles (Cellia) Rufipes (Gough, 1910) and Other Local Anophelines in Human Malaria Transmission in the Northern Savannah of Cameroon: A Cross-Sectional Survey. Parasit Vectors (2017) 10(1):22. doi: 10.1186/s13071-016-1933-3
13. Awono-Ambene PH, Etang J, Antonio-Nkondjio C, Ndo C, Eyisap WE, Piameu MC, et al. The Bionomics of the Malaria Vector Anopheles Rufipes Gough, 1910 and its Susceptibility to Deltamethrin Insecticide in North Cameroon. Parasit Vectors (2018) 11:253. doi: 10.1186/s13071-018-2809-5
14. Amvongo-Adjia N, Wirsiy EL, Riveron JM, Chounna Ndongmo WP, Enyong PA, Njiokou F, et al. Bionomics and Vectorial Role of Anophelines in Wetlands Along the Volcanic Chain of Cameroon. Parasit Vectors (2018) 11:471.
15. The President’s Malaria Initiative (PMI)/VectorLink Project. In: The PMI VectorLink Cameroon Annual Entomology Report: October 2018– September 2019. Rockville, MD: The PMI VectorLink Project, Abt Associates. p. 1–74.
16. Keating J, Macintyre K, Mbogo CM, Githure JI, Beier JC. Characterization of Potential Larval Habitats for Anopheles Mosquitoes in Relation to Urban Land-Use in Malindi, Kenya. Int J H Geogr (2004) 3:9. doi: 10.1186/1476-072X-3-9
17. Sattler M, Mtasiwa D, Kiama M, Premji Z, Tanner M, Killeen GF, et al. Habitat Characterization and Spatial Distribution of Anopheles Sp. Mosquito Larvae in Dar Es Salaam (Tanzania) During an Extended Dry Period. Malar J (2005) 4:4. doi: 10.1186/1475-2875-4-4
18. Awolola TS, Oduola AO, Obansa JB, Chukwurar NJ, Unyimadu JP. Anopheles gambiae s.s. Breeding in Polluted Water Bodies in Urban Lagos, Southwestern Nigeria. J VBD (2007) 44(4):241.
19. Klinkenberg E, McCall PJ, Wilson MD, Amerasinghe FP, Donnelly MJ. Impact of Urban Agriculture on Malaria Vectors in Accra, Ghana. Malar J (2008) 7:151. doi: 10.1186/1475-2875-7-151
20. Dongus S, Nyik D, Kannady K, Mtasiwa D, Mshinda H, Gosoniu, et al. Urban Agriculture and Anopheles Habitats in Dar Es Salaam, Tanzania. Geospatial Health (2009) 3(2):189–210. doi: 10.4081/gh.2009.220
21. Matthys EK, N’Goran M, Kon´e, Koudou BG, Vounatsou P, Cissé G, et al. Urban Agricultural Land Use and Characterization of Mosquito Larval Habitats in a Medium-Sized Town of Côte D’ivoire. J Vector Ecol (2006) 31(2):319–33. doi: 10.3376/1081-1710(2006)31[319:UALUAC]2.0.CO;2
22. Antonio-Nkondjio C, Fossog B, Ndo C, Djantio B, Togouet S, Awono-Ambene P. Anopheles gambiae Distribution and Insecticide Resistance in the Cities of Douala and Yaounde (Cameroon): Influence of Urban Agriculture and Pollution. Malar J (2011) 10:154. doi: 10.1186/1475-2875-10-154
23. Mbakop LR, Awono-Ambene PH, Mandeng SE, Ekoko WE, Fesuh BN, Antonio-Nkondjio C, et al. Malaria Transmission Around the Memve’ele Hydroelectric Dam in South Cameroon: A Combined Retrospective and Prospective Study, 2000–2016. Int J Environ Res Public Health (2019) 16:1618. doi: 10.3390/ijerph16091618
24. Keiser J, Utzinger J, Caldas de Castro M, Smith T, Tanner M, Singer B. Urbanization in Sub-Saharan Africa and Implication for Malaria Control. Am J Trop Med Hyg (2004) 71:118–27. doi: 10.4269/ajtmh.2004.71.118
25. Wang S, Lengeler C, Smith T, Vounatsou P, Cisse G, Diallo D, et al. Rapid Urban Malaria Appraisal (RUMA) in Sub-Saharan Africa. Malar J (2005) 4:40–10. doi: 10.1186/1475-2875-4-40
26. Kamdem C, Fossog B, Simard F, Etouna J, Ndo C, Kengne P. Anthropogenic Habitat Disturbance and Ecological Divergence Between Incipient Species of the Malaria Mosquito Anopheles gambiae. PloS One (2012) 7:e39453. doi: 10.1371/journal.pone.0039453
27. Quakyi I, Leke R, Befidi-Mengue R, Tsafack M, Bomba-Nkolo D, Manga L, et al. The Epidemiology of Plasmodium Falciparum Malaria in Two Cameroonian Villages: Simbok and Etoa. Am J Trop Med Hyg (2000) 63:222–30. doi: 10.4269/ajtmh.2000.63.222
28. Carnevale P, Mouchet J. Malaria Vector Control in Cameroon: Past, Present, Future. Reflect Bull Soc Pathol Exot (2001) 94(2):202–9.
29. Ademowo OG, Falusi AG, Mewoyeka OO. Prevalence of Asymptomatic Parasitaemia in an Urban and Rural Community in South Western Nigeria. Cent Afr J Med (1995) 41(1):18–21.
30. Mireji PO, Keating J, Hassanali A, Mbogo CM, Nyambaka H, Kahindi S, et al. Heavy Metals in Mosquito Larval Habitats in Urban Kisumu and Malindi, Kenya, and Their Impact. Ecotoxicol Environ Saf (2008) 70(1):147–53. doi: 10.1016/j.ecoenv.2007.03.012
31. Antonio-Nkondjio C, Defo-Talom B, Tagne-Fotso R, Tene-Fossog B, Ndo C, Lehman LG, et al. High Mosquito Burden and Malaria Transmission in a District of the City of Douala, Cameroon. BMC Infect Dis (2012) 12:275. doi: 10.1186/1471-2334-12-275
32. Nwane P, Etang J, Chouaibou M, Toto JC, Kerah-Hinzoumbé C, Mimpfoundi R. Trends in DDT and Pyrethroid Resistance in Anopheles gambiae s.s. Populations From Urban and Agro-Industrial Settings in Southern Cameroon. BMC Infect Dis (2009) 9(1):1–10. doi: 10.1186/1471-2334-9-163
33. Ndo C, Menze-Djantio B, Antonio-Nkondjio C. Awareness, Attitudes and Prevention of Malaria in the Cities of Douala and Yaoundé (Cameroon). Parasit Vectors (2011) 4(1):1–6. doi: 10.1186/1756-3305-4-181
34. Etang J, Mbida Mbida A, Ntonga Akono P, Binyang J, Eboumbou Moukoko CE, Lehman LG, et al. Anopheles Coluzzii Larval Habitat and Insecticide Resistance in the Island Area of Manoka, Cameroon. BMC Infect Dis (2016) 16(1):1–11. doi: 10.1186/s12879-016-1542-y
35. Mkwambisi DD, Fraser ED, Dougill AJ. Urban Agriculture and Poverty Reduction: Evaluating How Food Production in Cities Contributes to Food Security, Employment and Income in Malawi. J Inter Dev (2011) 23(2):181–203. doi: 10.1002/jid.1657
36. Binns T, Lynch K. Feeding Africa's Growing Cities Into the 21st Century: The Potential of Urban Agriculture. J inter Dev (1998) 10(6):777–93. doi: 10.1002/(SICI)1099-1328(1998090)10:6<777::AID-JID532>3.0.CO;2-Z
37. Atangana S, Foumbi J, Charlois M, Ambroise-Thomas P, Ripert C. Étude Epidémiologique De L’onchocercose Et Du Paludisme Dans La Région Du Lac De Retenue De Bamendjin- Cameroun. Faune Malacologique Locale Et Possibilités D’implantation Des Bilharzioses. Med Trop (1979) 39:537–43.
38. Manga L, Toto J-C, Le Goff G, Brunhes J. The Bionomics of Anopheles Funestus and its Role in Malaria Transmission in a Forested Area of Southern Cameroon. Trans R Soc Trop Med Hyg (1997) 91(4):387–8. doi: 10.1016/S0035-9203(97)90249-2
39. Antonio-Nkondjio C, Simard F, Cohuet A, Fontenille D. Morphological Variability in An. Moucheti is Not Indicative of Speciation: Evidences From Sympatric South Cameroon Populations. Inf Gen Evol (2002) 2(1):69– 71. doi: 10.1016/s1567-1348(02)00084-9
40. Antonio-Nkondjio C, Simard F, Awono-Ambene P, Ngassam P, Toto JC, Tchuinkam T, et al. Malaria Vectors and Urbanization in the Equatorial Forest Region of South Cameroon. Trans R Soc Trop Med Hyg (2005) 99(5):347–54. doi: 10.1016/j.trstmh.2004.07.003
41. Awono-Ambene HP, Antonio-Nkondjio C, Toto JC, Ndo C, Etang J, Fontenille D, et al. Epidemological Importance of the Anopheles Nili Group of Malaria Vectors in Equatorial Villages of Cameroon, Central Africa. Sci Med Afr (2009) 1:13–20.
43. Available at: https://www.osidimbea.cm/collectivites/centre/yaounde-6-arr/.
44. Gillies MT, Coetzee M. A Supplement to the Anophelinae of Africa South of the Sahara (Afrotropical Region). Johannesburg: The South African Institute of Medical Research (1987).
45. Gillies MT, De Meillon B. The Anophelinae of Africa South of the Sahara (Ethiopian Zoogeographical Region). 2nd ed. Johannesburg: The South African Institute of Medical Research (1968).
46. Burkot TR, Williams JL, Schneider J. Identification of Plasmodium Falciparum Infected Mosquitoes by a Double Antibody Enzyme-Linked Immunosorbent Assay. Am J Trop Med Hyg (1984) 33:783–8. doi: 10.4269/ajtmh.1984.33.783
47. Wirtz RA, Burkot TR, Graves PM, Andre RG. Field Evaluation of Enzyme-Linked Immunosorbent Assays for Plasmodium Falciparum and Plasmodium Vivax Sporozoites in Mosquitoes (Diptera: Culicidae) From Papua New Guinea. J Med Entomol (1987) 24:433–7. doi: 10.1093/jmedent/24.4.433
48. Collins FH, Mendez MA, Razmussen MO, Mehaey PC, Besansky NJ, Finnerty V. Ribosomal RNA Gene Probe Differentiates Member Species of Anopheles gambiae Complex. Am J Trop Med Hyg (1987) 37:37–41. doi: 10.4269/ajtmh.1987.37.37
49. Santolamazza F, Calzetta M, Etang J. Distribution of Knock-Down Resistance Mutations in Anopheles Gambiae Molecular Forms in West and West-Central Africa. Malar J (2008) 7:74. doi: 10.1186/1475-2875-7-74
50. WHO. Test Procedures for Insecticide Resistance Monitoring in Malaria Vector Mosquitoes. Geneva: World Health Organization Press (2016). p. 43.
51. Lynd A, Ranson H, McCall PJ, Randle NP, Black WC IV, Walker ED, et al. A Simplified High-Throughput Method for Pyrethroid Knockdown Resistance (Kdr) Detection in Anopheles gambiae. Malar J (2005) 4:16. doi: 10.1186/1475-2875-4-16
52. Weill M, Chandre F, Brengues C, Manguin S, Akogbeto M, Pasteur N, et al. The Kdr Mutation Occurs in the Mopti Form of Anopheles gambiae s.s. Through Introgression. Insect Mol Biol (2000) 9(5):451–5. doi: 10.1046/j.1365-2583.2000.00206.x
54. Chandre F, Darrier F, Manga L, Akogbeto M, Faye O, Mouchet J, et al. Status of Pyrethroid Resistance in Anopheles gambiae Sensu Lato. Bull World Health Organ (1999) 77(3):230.
55. Thomas A, Kumar S, Pillai MKK. Piperonyl Butoxide as a Counter Measure for Deltamethrin Resistance in Culex quinquefasciatus Say. Entomol (1991) 18:1–10.
56. Rousset F. Genepop’007: A Complete Re-Implementation of the Genepop Software for Windows and Linux. Mol Ecol Res (2008) 8:103–6. doi: 10.1111/j.1471-8286.2007.01931.x
57. Alemu A, Abebe G, Tsegaye W, Golassa L. Climatic Variables and Malaria Transmission Dynamics in Jimma Town, South West Ethiopia. Parasit Vectors (2011) 4(1):1–11. doi: 10.1186/1756-3305-4-30
58. Doumbe-Belisse P, Ngadjeu CS, Sonhafouo-Chiana N, Talipouo A, Djamouko-Djonkam L, Kopya E, et al. High Malaria Transmission Sustained by Anopheles Gambiae s.L. Occurring Both Indoors and Outdoors in the City of Yaoundé, Cameroon. Wellcome Open Res (2018) 3:164. doi: 10.12688/wellcomeopenres.14963.1
59. De Silva PM, Marshall JM. Factors Contributing to Urban Malaria Transmission in Sub-Saharan Africa: A Systematic Review. J Trop Med (2012). doi: 10.1155/2012/819563
60. MacHault V, Gadiaga L, Vignolles C, Jarjaval F, Bouzid S, Sokhna C, et al. Highly Focused Anopheline Breeding Sites and Malaria Transmission in Dakar. Malar J (2009) 8(1):138. doi: 10.1186/1475-2875-8-138
61. Byrne N. Urban Malaria Risk in Sub-Saharan Africa: Where is the Evidence? Travel Med Infect Dis (2007) 5(2):135–7. doi: 10.1016/j.tmaid.2006.04.003
62. Doumbe-Belisse P, Kopya E, Ngadjeu CS, Sonhafouo-Chiana N, Talipouo A, Djamouko-Djonkam LH, et al. Urban Malaria in Sub-Saharan Africa: Dynamic of the Vectorial System and the Entomological Inoculation Rate. Malar J (2021) 20(1):1–18. doi: 10.1186/s12936-021-03891-z
63. Munga S, Minakawa N, Zhou G, Mushinzimana E, Barrack OOJ, Githeko AK, et al. Association Between Land Cover and Habitat Productivity of Malaria Vectors in Western Kenyan Highlands. Am J Trop Med Hyg (2006) 74(1):69–7. doi: 10.4269/ajtmh.2006.74.69
64. Cator LJ, Thomas S, Paaijmans KP, Ravishankaran S, Justin JA, Mathai MT, et al. Characterizing Microclimate in Urban Malaria Transmission Settings: A Case Study From Chennai, India. Malar J (2013) 12:1–10. doi: 10.1186/1475-2875-12-84
65. Imbahale SS, Fillinger U, Githeko A, Mukabana WR, Takken W. An Exploratory Survey of Malaria Prevalence and People’s Knowledge, Attitudes and Practices of Mosquito Larval Source Management for Malaria Control in Western Kenya. Acta Tropica (2010) 115(3):248–56. doi: 10.1016/j.actatropica.2010.04.005
66. PNLP. Plan Stratégique National De Lutte Contre Le Paludisme 2011–2015. Minsante Cameroun Yaoundé (2012).
67. PNLP. Rapport D’activités Du Programme National De Lutte Contre Le Paludisme. Minsante Cameroun Yaoundé (2015).
68. Bamou R, Mbakop LR, Kopya E, Ndo C, Awono-Ambene P, Tchuinkam T, et al. Changes in Malaria Vector Bionomics and Transmission Patterns in the Equatorial Forest Region of Cameroon Between 2000 and 2017. Parasit Vectors (2018) 11(1):1–13. doi: 10.1186/s13071-018-3049-4
69. Tene Fossog B, Ayala D, Acevedo P, Kengne P, Ngomo Abeso Mebuy I, et al. Habitat Segregation and Ecological Character Displacement in Cryptic African Malaria Mosquitoes. Evol Appl (2015) 8(4):326–45. doi: 10.1111/eva.12242
70. Kudom, AAn. Larval Ecology of Anopheles Coluzzii in Cape Coast, Ghana: Water Quality, Nature of Habitat and Implication for Larval Control. Malar J (2015) 14:447. doi: 10.1186/s12936-015-0989-4
71. Antonio-Nkondjio C, Doumbe-Belisse P, Djamouko-Djonkam L, Ngadjeu CS, Talipouo A, Kopya E, et al. High Efficacy of Microbial Larvicides for Malaria Vectors Control in the City of Yaounde Cameroon Following a Cluster Randomized Trial. Sci Rep (2021) 11(1):1–15. doi: 10.1038/s41598-021-96362-z
72. Piameu M, Nwane P, Toussile W, Mavridis K, Wipf NC, Kouadio PF, et al. Pyrethroid and Etofenprox Resistance in Anopheles gambiae and Anopheles Coluzzii From Vegetable Farms in Yaoundé, Cameroon: Dynamics, Intensity and Molecular Basis. Molecules (2021) 26(18):5543. doi: 10.3390/molecules26185543
73. Yadouleton AWM, Asidi A, Djouaka RF, Braïma J, Agossou CD, Akogbeto MC. Development of Vegetable Farming: A Cause of the Emergence of Insecticide Resistance in Populations of Anopheles gambiae in Urban Areas of Benin. Malar J (2009) 8(1):1–8. doi: 10.1186/1475-2875-8-103
74. Sternberg ED, Thomas MB. Insights From Agriculture for the Management of Insecticide Resistance in Disease Vectors. Evol Appl (20182009) 11(4):404–14. doi: 10.1111/eva.12501
75. Donnelly MJ, Corbel V, Weetman D, Wilding CS, Williamson MS, Black WC IV. Does Kdr Genotype Predict Insecticide-Resistance Phenotype in Mosquitoes? Trends Parasitol (2009) 25(5):213–9. doi: 10.1016/j.pt.2009.02.007
76. Kwiatkowska RM, Platt N, Poupardin R, Irving H, Dabire RK, Mitchell S, et al. Dissecting the Mechanisms Responsible for the Multiple Insecticide Resistance Phenotype in Anopheles Gambiae s.s., M Form, From Vallee Du Kou, Burkina Faso. Gene (2013) 519(1):98–106. doi: 10.1016/j.gene.2013.01.036
77. Reimer L, Fondjo E, Patchoké S, Diallo B, Lee Y, Ng A, et al. Relationship Between Kdr Mutation and Resistance to Pyrethroid and DDT Insecticides in Natural Populations of Anopheles gambiae. J Med Entomol (2014) 45(2):260–6. doi: 10.1093/jmedent/45.2.260
78. Moreno M, Vicente JL, Cano J, Berzosa PJ, De Lucio A, Nzambo S, et al. Knockdown Resistance Mutations (Kdr) and Insecticide Susceptibility to DDT and Pyrethroids in Anopheles Gambiae From Equatorial Guinea. Trop Med Int Health (2008) 13(3):430–3. doi: 10.1111/j.1365-3156.2008.02010.x
79. Etang J, Fondjo E, Chandre F, Morlais I, Brengues C, Nwane P, et al. First Report of Knockdown Mutations in the Malaria Vector Anopheles gambiae From Cameroon. Am J Trop Med Hyg (2006) 74(5):795–7. doi: 10.4269/ajtmh.2006.74.795
80. Nwane P, Etang J, Chouaїbou M, Toto JC, Mimpfoundi R, Simard F. Kdr-Based Insecticide Resistance in Anopheles gambiae s.s. Populations in Cameroon: Spread of the L995F and L995S Mutations. BMC Res Notes (2011) 4(1):1–9. doi: 10.1186/1756-0500-4-463
81. Bamou R, Sonhafouo-Chiana N, Mavridis K, Tchuinkam T, Wondji CS, Vontas J, et al. Status of Insecticide Resistance and Its Mechanisms in Anopheles gambiae and Anopheles Coluzzii Populations From Forest Settings in South Cameroon. Genes (2019) 10(10):741. doi: 10.3390/genes10100741
82. Nwane P, Etang J, Chouaїbou M, Toto JC, Koffi A, Mimpfoundi R, et al. Multiple Insecticide Resistance Mechanisms in Anopheles gambiae s.L. Populations From Cameroon, Central Africa. Parasit Vectors (2013) 6(1):1–14. doi: 10.1186/1756-3305-6-41
83. Diabate A, Baldet T, Chandre F, Akoobeto M, Guiguemde TR, Guillet P, et al. The Role of Agricultural Use of Insecticides in Resistance to Pyrethroids in Anopheles gambiae s.L. In Burkina Faso. Am J Trop Med Hyg (2002) 67(6):617–22. doi: 10.4269/ajtmh.2002.67.617
84. Yawson AE, McCall PJ, Wilson MD, Donnelly MJ. Species Abundance and Insecticide Resistance of Anopheles gambiae in Selected Areas of Ghana and Burkina Faso. Med Vet entomol (2004) 18(4):372–7. doi: 10.1111/j.0269-283X.2004.00519.x
85. Mandeng S, Awono-Ambene HP, Bigoga JD, Ekoko WE, Binyang J, Piameu M, et al. Spatial and Temporal Development of Deltamethrin Resistance in Malaria Vectors of the Anopheles gambiae Complex From North Cameroon. PloS One (2019) 14(2):e0212024. doi: 10.1371/journal.pone.0212024
86. Brooke BD. Kdr: Can a Single Mutation Produce an Entire Insecticide Resistance Phenotype? Trans R Soc Trop Med Hyg (2008) 102(6):524–5. doi: 10.1016/j.trstmh.2008.01.001
Keywords: insecticide resistance, urbanization, malaria transmission, malaria vectors, Anopheline mosquitoes, environmental change
Citation: Mbakop LR, Awono-Ambene PH, Ekoko WE, Mandeng SE, Nwane P, Fesuh BN, Toto J-C, Alenou LD, Onguina HG, Piameu M, Fomena A and Etang J (2022) Malaria Transmission and Vector Resistance to Insecticides in a Changing Environment: Case of Simbock in Yaoundé-City, Cameroon. Front. Trop. Dis 3:902211. doi: 10.3389/fitd.2022.902211
Received: 22 March 2022; Accepted: 27 April 2022;
Published: 08 July 2022.
Edited by:
Nathan Daniel Burkett-Cadena, University of Florida, United StatesReviewed by:
Anne Poinsignon, Institut de Recherche Pour le Développement (IRD), FranceRobert Tyrrell Jones, University of London, United Kingdom
Copyright © 2022 Mbakop, Awono-Ambene, Ekoko, Mandeng, Nwane, Fesuh, Toto, Alenou, Onguina, Piameu, Fomena and Etang. This is an open-access article distributed under the terms of the Creative Commons Attribution License (CC BY). The use, distribution or reproduction in other forums is permitted, provided the original author(s) and the copyright owner(s) are credited and that the original publication in this journal is cited, in accordance with accepted academic practice. No use, distribution or reproduction is permitted which does not comply with these terms.
*Correspondence: Josiane Etang, josyet2@gmail.com