- 1Department of Pathobiology and Population Sciences, Royal Veterinary College, University of London, Hatfield, United Kingdom
- 2Department of Infectious Disease Epidemiology, London Centre for Neglected Tropical Disease Research, School of Public Health, Imperial College London, London, United Kingdom
- 3Interactions Hôtes-Agents Pathogènes, Institut National de Recherche pour l’Agriculture, l’Alimentation et l’Environnement, École Nationale Vétérinaire de Toulouse, Université de Toulouse, Toulouse, France
- 4Big Data Institute, University of Oxford, Oxford, United Kingdom
- 5Unité de Formation et de Recherche des Sciences Agronomiques, d’Aquaculture et de Technologies Alimentaires, Université Gaston Berger, Saint-Louis, Senegal
Schistosomiasis, a neglected tropical disease, is a widespread chronic helminthiasis reported in 78 countries, predominantly those within sub-Saharan Africa, as well as Latin America, Asia, and most recently, even Europe. Species of the causative blood fluke infect not only humans but also animals, and hybrids between previously assumed human-specific and animal-specific schistosomes are being increasingly reported. Existing control programs across Africa focus on humans and rely heavily on mass drug administration of praziquantel, the sole drug available against schistosomiasis. Praziquantel is safe and highly efficacious but could become ineffective if resistance emerges. To reach the revised World Health Organization goal of elimination of schistosomiasis as a public health problem, and interruption of transmission within selected regions, by 2030, new consideration of the role of animal reservoirs in human transmission in general, and whether to also treat livestock with praziquantel in particular, has been raised. However, whilst there are no dedicated control programs targeting animals outside of Asia, there are emerging reports of the use and misuse of praziquantel in livestock across Africa. Therefore, to effectively treat livestock in Africa and to help mitigate against the potential evolution of praziquantel resistance, structured control strategies are required. Here, using a transmission modelling approach, we evaluate the potential effectiveness of a theoretical test-and-treat (TnT) strategy to control bovine schistosomiasis using a currently available point-of-care diagnostic test (developed for human use) to detect circulating cathodic antigen (POC-CCA). We show that implementing TnT at herd-level from 2022 to 2030 could be highly effective in suppressing infection in cattle and even, in lower prevalence settings, reaching nominal ‘elimination’ targets. We highlight the importance of enhancing the specificity of POC-CCA for use in livestock to avoid unnecessary treatments and discuss the outstanding challenges associated with implementing TnT as part of a holistic One Health approach to tackling human and animal schistosomiasis.
Introduction
A substantial proportion of the world’s disease burden is caused by infectious agents that lead to high mortality, morbidity, and reduced productivity among millions of people and their animals. Schistosomiasis is a major freshwater-snail-borne neglected tropical disease (NTD) caused by blood flukes of the genus Schistosoma. This parasitic disease remains a major public health problem in the majority of 78 tropical and subtropical countries, infecting >240 million people (1–3), 90% living in sub-Saharan Africa (SSA) (2, 4).
Current global efforts to control human schistosomiasis rely predominantly on mass drug administration (MDA), whereby populations (mainly school-aged children) are mass treated with the donated anthelminthic, praziquantel (PZQ). Praziquantel is safe, highly efficacious, and can be administered easily. Consequently, MDA programs have had, in general, major impacts on reducing infection prevalence, intensity and morbidity (2, 3, 5–7). The success of such activities has led the World Health Organization (WHO) to set revised targets for the ‘elimination as a public health problem’ (EPHP) (<1% heavy intensity infections) of schistosomiasis in all affected countries, together with interruption of transmission within selected regions, by 2030 (8). The potential role of schistosomiasis in animals does, however, raise significant challenges for achieving these goals, and stresses the critical need for a One Health approach globally.
Traditionally, only the Asian schistosome species S. japonicum and S. mekongi were widely acknowledged as zoonotic (9). Domestic animals, notably bovines, have been suggested as responsible for up to 97% of schistosomiasis japonica disease transmission in Marshland regions of China (10). Consequently, China has performed integrated mass PZQ treatment of both humans and bovines, as well as improvements to water, sanitation and hygiene (WASH), replacement of bovines with tractors, and has attempted to develop a bovine vaccine. These sustained efforts have yielded great progress: from being a country with the highest schistosomiasis burden in the 1950s to, since 2015, reaching their EPHP goal (11). Indeed, the overall prevalence of S. japonicum in China is now <1% in both humans and livestock populations (11, 12), and the majority of endemic provinces have either reached the Chinese authorities criteria of transmission control [i.e., under 1% infection prevalence in humans and livestock, in addition to negative local malacological surveys in two consecutive days plus absence of acute schistosomiasis cases (11)] or transmission interruption [i.e.: no infections in humans or livestock for five consecutive years, in addition to absence of infected intermediate snails for at least five years (11)].
In contrast, the role of animal schistosomiasis in SSA, in terms of either zoonotic risk or economic impact has, until very recently, been largely ignored, and there are no formal anti-schistosome control nor donation programs involving animals. Livestock schistosomiasis caused by S. bovis, S. curassoni and/or S. mattheei in cattle, sheep and goats is, however, prevalent across SSA (9, 13). Furthermore, while human urogenital schistosomiasis was previously thought to be solely caused by the human-specific parasite S. haematobium, an ever-growing number of molecular studies have identified viable hybrids between this species and co-endemic livestock-specific parasites (9, 14–21), shed in the urine of human patients of several SSA countries including Senegal (14, 16, 21), Niger (20), Benin (17), Malawi (15), Côte d’Ivoire (18) and Nigeria (19). Notably, S. bovis hybrids appear to be common among infected children in locations of Senegal (72% S. haematobium - S. bovis hybrid prevalence) (16) and Nigeria (89% S. bovis - S. haematobium hybrid prevalence) (19), with ‘pure’ S. bovis human infections recently confirmed in Côte d’Ivoire (18) and Nigeria (19). A case of hybridized livestock S. bovis with S. curassoni viably infecting a child in Niger has also been identified (20). Interestingly, a hybridized S. haematobium - S. bovis zoonotic strain likely introduced from either Senegal (22, 23) or Benin (17, 23) appears to be now established within Corsica, Europe (23, 24).
These findings demonstrate that there is an important zoonotic component of schistosomiasis transmission in SSA, and one which has been predicted to be of increasing importance as elimination programs targeting humans intensify (25). Additionally, there are highly important, if also often overlooked, animal welfare, productivity, and socioeconomic impacts of animal schistosomiasis, which further jeopardize livelihoods, food security and nutrition amongst neglected communities (26, 27). Schistosome infection in cattle, though often classed as sub-clinical, has been associated with diarrhea, anemias, weight loss, growth retardation, susceptibility to infection, and/or intestinal and hepatic lesions (13, 28, 29). Similar, or often more severe, morbidity (and mortality), combined with reduced productivity profiles have also recently been reported in infected sheep and goats (26, 27). Indeed, the financial impact projected over one year of withholding livestock schistosomiasis interventions was recently predicted to be substantial, where its costs surpassed those of testing and treating suspected cases in Northern Senegal (27).
The importance of veterinary public health interventions in achieving the WHO elimination 2030 targets has now been acknowledged in both the newly launched WHO NTD Roadmap 2021-2030 (8) and the Schistosomiasis Guideline for control and elimination of human schistosomiasis (30, 31). Amongst challenges identified to reach this goal are gaps in the scientific understanding of zoonotic transmission and implementation of effective interventions to address zoonotic reservoirs, as well as critical actions regarding diagnostics development, including standardized point-of-care (POC) tests (8). It is unlikely that an equivalent MDA strategy as used in humans would be deemed acceptable for livestock because of the potential risk of promoting the emergence of PZQ resistance. This is critical, given there is now resistance to all known veterinary anthelminthics globally (32), and any exacerbation of the risk of emerging resistance is particularly pertinent for schistosomiasis as PZQ is the only efficacious drug for both humans and livestock.
Nevertheless, recent work indicates already widespread use and misuse of PZQ in livestock–including, but not exclusive to, PZQ tablets donated via the WHO MDA programs for school-aged children, with little knowledge of application or dosage requirements (26). Indeed, more than a third of respondents in a recent survey within Senegal stated that they had given anti-schistosomiasis treatment to their livestock within the preceding four years (27). Such documented indiscriminate PZQ use against schistosomes of domestic ruminants highlights a previously unforeseen yet existing demand for animal disease control. A critical need to develop a structured approach for the treatment of livestock schistosomiasis in SSA is thus evident.
A test-and-treat (TnT, and/or the related T3: Test, Treat, Track) design for controlling livestock schistosomiasis would anchor the key recent WHO policy recommendations on diagnostic testing, treatment and surveillance in general (8, 30). Such a strategy would enable local veterinarians, veterinary technicians and/or community leaders within endemic countries to easily and inexpensively assess whether a herd is infected with schistosomiasis and only then distribute appropriately dosed PZQ. Such a policy could minimize the costs and suffering to the livestock and their owners, and at the same time mitigate the risk of promoting the emergence of PZQ resistance as compared to either PZQ misuse or an MDA approach of treating all herds irrespective of their infection status. Here, we evaluate the impact of a theoretical herd-level TnT intervention targeting bovine schistosomiasis running from 2022 through 2030 using a mathematical transmission model. We use our recent epidemiological data on the prevalence of bovine schistosomiasis collected in farming locations of Northern Senegal to inform model parameterization and we discuss our findings in terms of their theoretical and applied implications for integrated One Health schistosomiasis disease control and elimination policy and practice.
Materials and methods
Ethical statement
Ethical approval for the fieldwork was provided by: (i) Imperial College London (London, UK) application 03.36; (ii) the CRERB at the Royal Veterinary College (London, UK) application URN20151327; and (iii) the Comité National d’Ethique pour la Recherche en Santé (Dakar, Senegal) application SEN15/68. Written informed consent was obtained from all livestock owners. Livestock owners were offered standard anthelmintics for infected animals.
Parasitological data
Prevalence estimates presented here focus on data from cattle (live Bos taurus, Bos indicus and cross breeds) coprological surveys conducted in 2017 in the Senegal River Basin area of northern Senegal, West Africa, as part of the Zoonoses and Emerging Livestock System (ZELS) program (16, 25). In this human schistosomiasis endemic area, Richard Toll and Lac de Guiers (estimated urogenital schistosomiasis prevalence in children and adults was 87% and 79% in 2016, and 88% and 41% in 2017-18, respectively (16)), henceforth referred to as RT, schistosome transmission is perennial due to the year-round persistence of freshwater habitats for intermediate snail hosts (i.e., the Senegal River and the Lac de Guiers lake) (16).
Faecal samples from 200 sympatric live-sampled bovines in eight RT site locations (Didjiery; Mbane; Medina Cheikhou; Ndombo; Sanente; Temeye; Thiago and Yetti Yone) were collected following randomized protocols and processed using two schistosomiasis tests: Kato-Katz (KK) for presence of Schistosoma spp. eggs (examination of two slides per sample), and an adapted Miracidial Hatching Technique (MHT) to determine egg viability (and also used as an alternative diagnostic for the presence of eggs). Protocols for both techniques are described elsewhere (16).
Prevalence estimates derived from the results of both coprological exams informed the prevalence range explored in the modelled TnT strategy (Figure 1) using an imperfect semiquantitative POC diagnostic measuring urine levels of the adult worm gut-associated circulating cathodic antigen (CCA), hereby referred to as POC-CCA (33). This POC diagnostic is ordinarily employed for S. mansoni human-endemicity mapping given its superior sensitivity in comparison to the reference standard KK (34). Preliminary sensitivity and specificity estimates of POC-CCA using Bayesian latent class approaches based on bovine urine samples collected during the ZELS program (35)–where the overwhelming majority of infected cattle shed S. bovis (16)–were used for modelling TnT (Table 1). These POC-CCA performance estimates were based on the interpretation of a positive result as indicative of an infected animal, and of a negative result as indicative of an uninfected animal (positive cut-off value: ≥ trace, i.e., trace, +, ++ or +++) (35).
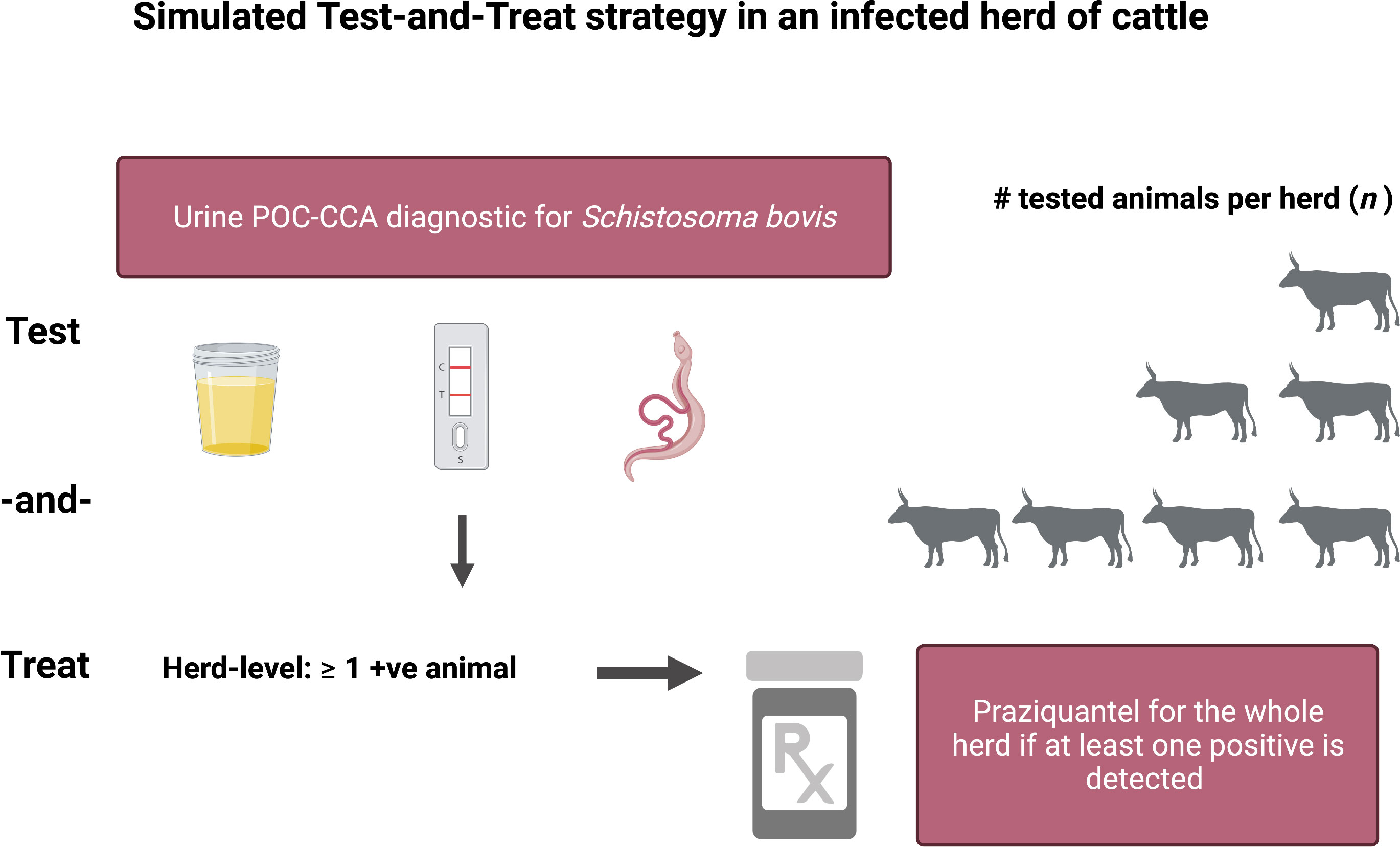
Figure 1 Schematic of the proposed Test-and-Treat (TnT) strategy simulated in the herd-level transmission model. Created with BioRender.com.
Prevalence estimation
The KK and MHT data were modelled as two independent Bernoulli-distributed variables conditional on the location-specific prevalence, πi, and the sensitivity, se, and specificity, sp, of the respective diagnostics. Therefore, the probability that an individual tests positive for each diagnostic is
The sensitivity of each test was assigned an informative beta prior parameterized using published performance values (16) such that the median and central 95% percentile was 0.31 (0.16 – 0.49) for KK and 0.79 (0.60- 0.91) for MHT. The specificity of both tests was assumed to be 1 (i.e., 100%). The prevalence in each location was estimated using a Bayesian Markov Chain Monte Carlo (MCMC) approach using the JAGS program (JAGS version 4.3.0) called with the rjags package version 4-12 (38) for R version 4.0.2 (39).
Herd-level transmission model
We developed a simple deterministic mathematical transmission model based on that originally published by Anderson and May (36, 40) to track the mean number of schistosomes per host (worm burden), W(t), during a TnT intervention. The model is defined by a single ordinary differential equation,
where μ0 and μ1 denote the cattle and schistosome mortality rates respectively and the environmental and intermediate host stages of the schistosome lifecycle are compressed into the basic reproduction number R0=RH→SRS→H. The component RH→S captures transmission from cattle to snails and RS→H transmission from snails to cattle. The snail mortality rate is denoted μ2 and N1/N2 is the definitive (cattle) to intermediate (snail) host population size. The finite nature (and rapid turnover) of the snail population size provides a natural negative density dependence to constrain the size of the parasite population and the mating probability, Φ(W(t), k(t)) (40) is a positive density-dependent process that leads to the existence of a transmission breakpoint (41). Parameter k(t) is the overdispersion parameter of the negative binomial distribution, which is assumed to capture adequately the distribution of schistosomes among hosts. The time dependency of k(t) results from the changing overdispersion following initiation of treatment (42),
where k0, k1, W0 and W1 are the overdispersion parameters and mean worm burden at baseline (pre-intervention, subscript 0) and immediately after treatment (subscript 1), respectively, and tT denotes a treatment time. The prevalence of infection, π(t), is derived from the negative binomial distribution of worms among hosts (40)
Baseline conditions, parametric uncertainty, and interventions
We ran the model to endemic equilibrium using 100,000 parameter sets drawn from a Latin hypercube, sampling R0, RH→S, N1/N2 and k0 from uniform prior distributions (see Table 1). We then selected randomly 250 parameter sets within ± 1% of 5% increments in baseline prevalence from 40% to 95%. Using these parameter sets we modelled the impact of a TnT intervention strategy (see Figure 1) using PZQ starting in 2022 and continuing annually with the last TnT round implemented in 2030. At each TnT round, the probability of a herd receiving treatment is given by the probability of at least one positive POC-CCA test result, P(+≥1), from n sampled animals
where sePOC and spPOC denote the sensitivity and specificity of the POC-CCA respectively. Note that we assume a parallel testing regimen whereby n animals are tested simultaneously and if at least one positive test is returned, treatment is triggered. Combining P(+≥1) with the efficacy of PZQ, ϵ, we thus modelled the (average) effect of treatment at each TnT round as
Test-and-treat effectiveness indicators
We evaluated the effectiveness of the TnT intervention by two metrics. First, we calculated the case-years averted to the end of 2030 as a percentage of the baseline prevalence in 2022 (endemic equilibrium before the first round of TnT),
Second, we calculated the percentage of simulations within each prevalence category that nominally reached elimination, here defined as having a prevalence of less than 1% at the end of 2030. Note that this is different from the WHO elimination as a public health problem threshold of 1% heavy infections which could not be applied readily to livestock because of the difficulty in quantifying infection intensity.
Diagnostic performance dynamics
In addition to calculating the probability that at least one POC-CCA would return a positive result (and thus trigger treatment with PZQ, Equation 5), we also calculated the positive predictive value (PPV, assuming a parallel testing strategy) as an indicator of the performance of TnT in being able to detect correctly infected bovines during the intervention,
Results
Prevalence of bovine schistosomiasis
The apparent prevalence (by either KK or MHT) and the estimated ‘true’ prevalence of Schistosoma spp. infection among cattle in the eight sampled locations in RT are shown in Table 2 and Figure 2. The high sensitivity of MHT compared to KK (~79% vs. ~31%) ensures greater concordance between the ‘true’ prevalence and that observed by MHT compared to KK (Figure 2). Bayesian posterior ‘true’ prevalence estimates ranged from 43% (95% credible interval, CrI: 13%, 82%) to 95% (95% CrI: 85%, 100%), with six of the eight locations having a ‘true’ prevalence greater than 50%. These estimates informed the prevalence range (40% to 95%) considered in the modelling of TnT.
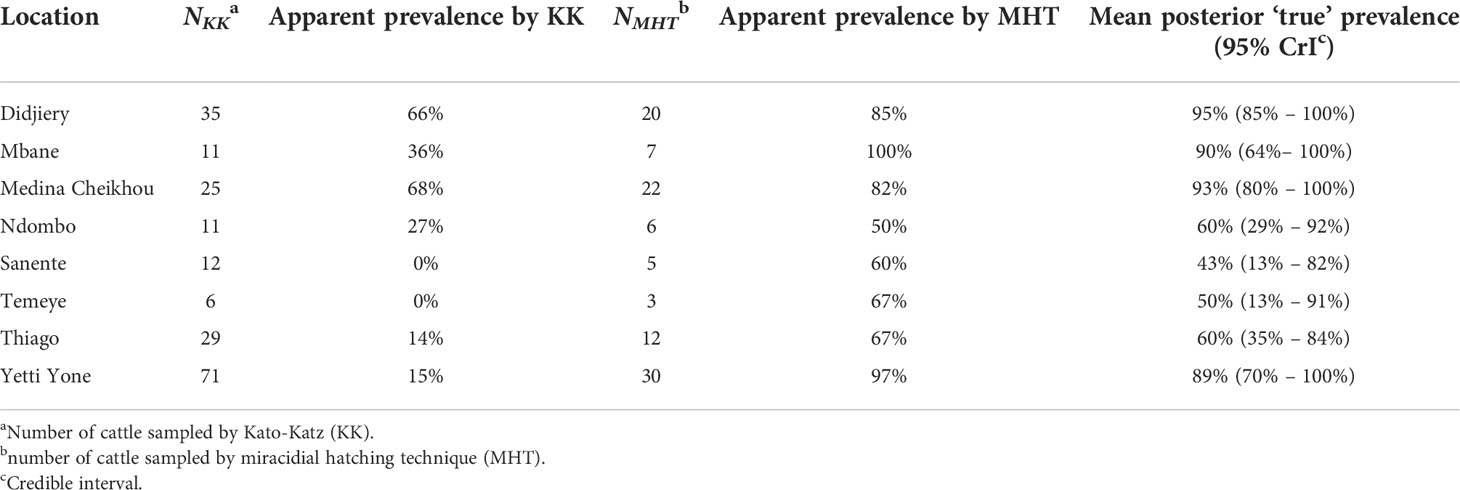
Table 2 Estimates of ‘apparent’ and ‘true’ prevalence of bovine schistosomiasis in eight locations in Richard Toll, Senegal sampled in 2017.
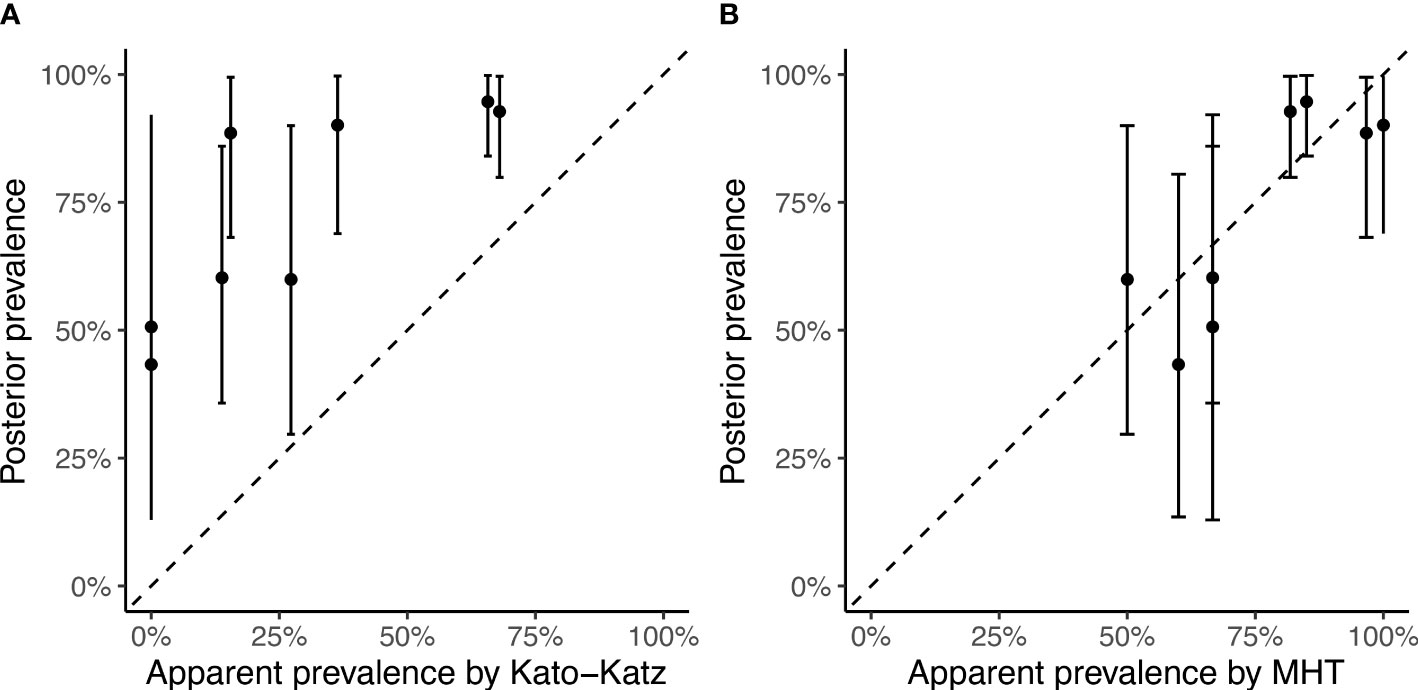
Figure 2 Posterior mean ‘true’ prevalence (points) and 95% credible intervals (vertical lines) versus apparent prevalence by (A) Kato-Katz and (B) Miracidial Hatching Technique (MHT) in eight locations in Richard Toll, Senegal sampled in 2017. The dotted line of y = x is a guide to assess visually the degree of concordance between the apparent and true prevalence by each diagnostic.
Infection dynamics
The modelled prevalence dynamics during a TnT intervention between 2022 and 2030 using either n=1, n=2 or n=4 POC-CCA tests per herd are illustrated in Figure 3 for a (pre-intervention) baseline prevalence of 50%, 70% and 90%. Each TnT round is assumed to be implemented at the start of each year, with the reduction in prevalence being a function of the efficacy of PZQ (90%, Table 1) and the probability of at least one positive POC-CCA test (i.e., conditional on at least one positive test, all animals are treated). Consequently, the more animals tested, the greater the impact of each TnT round. Depending on the level of endemicity (baseline prevalence) and the number of tests administered per TnT round, infection dynamics can either stabilize at a suppressed ‘pseudo equilibrium’ or can continue to decline towards transmission breakpoints.
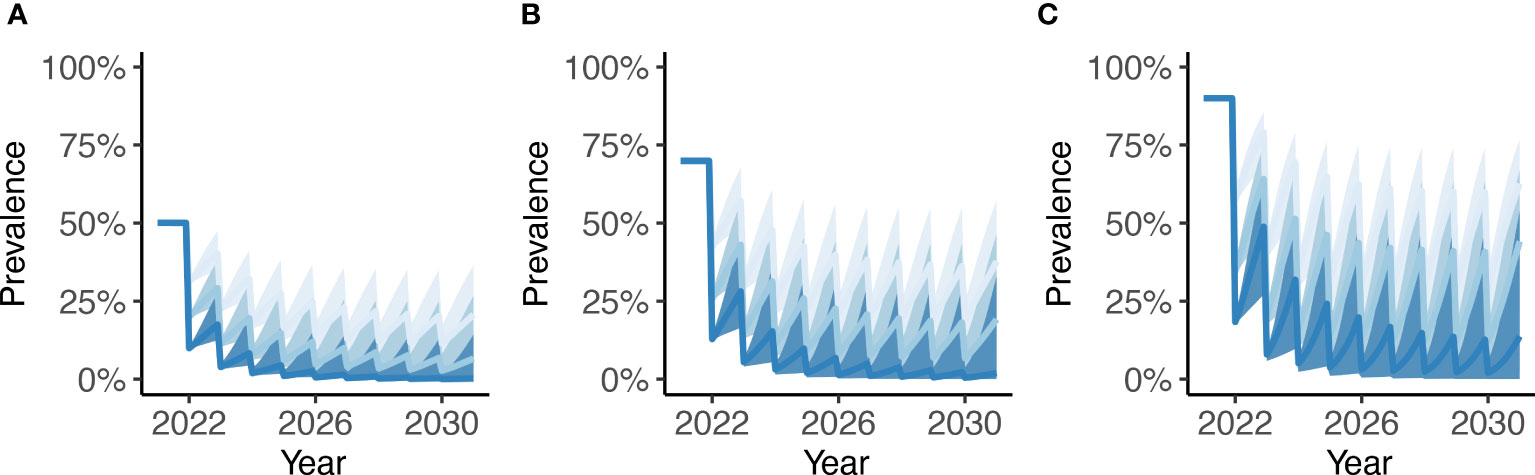
Figure 3 Modelled prevalence dynamics of bovine schistosomiasis during a test-and-treat (TnT) intervention between 2022 and 2030 using either n =1 (light blue), n = 2 (baby blue) or n = 4 (sapphire blue) POC-CCA tests administered per TnT round. Dynamics are shown for a baseline prevalence of (A) 50%, (B) 70% and (C) 90% with uncertainty generated by 250 parameter sets yielding a baseline prevalence ± 1% of the target. In each panel, the thick solid line depicts the mean and the shaded area the 95% credible interval.
Effectiveness of test-and-treat
The reduction in bovine schistosomiasis case-years as a percentage of the baseline prevalence achieved by the end of 2030 are shown in Figure 4. The relative impact of interventions decreases slightly with increasing baseline prevalence. For example, when four animals are tested per TnT round, the mean case-years averted range from a maximum of approximately 85% at a starting prevalence of 40% to approximately 75% at 95% baseline prevalence. When two animals are tested per round, the corresponding reductions range from approximately 75% at 40% baseline prevalence to approximately 60% at 95% baseline prevalence. Irrespective of baseline prevalence, the maximum achievable percentage reduction in case-years over the 9-year time horizon (2022 through 2030) is approximately 85%.
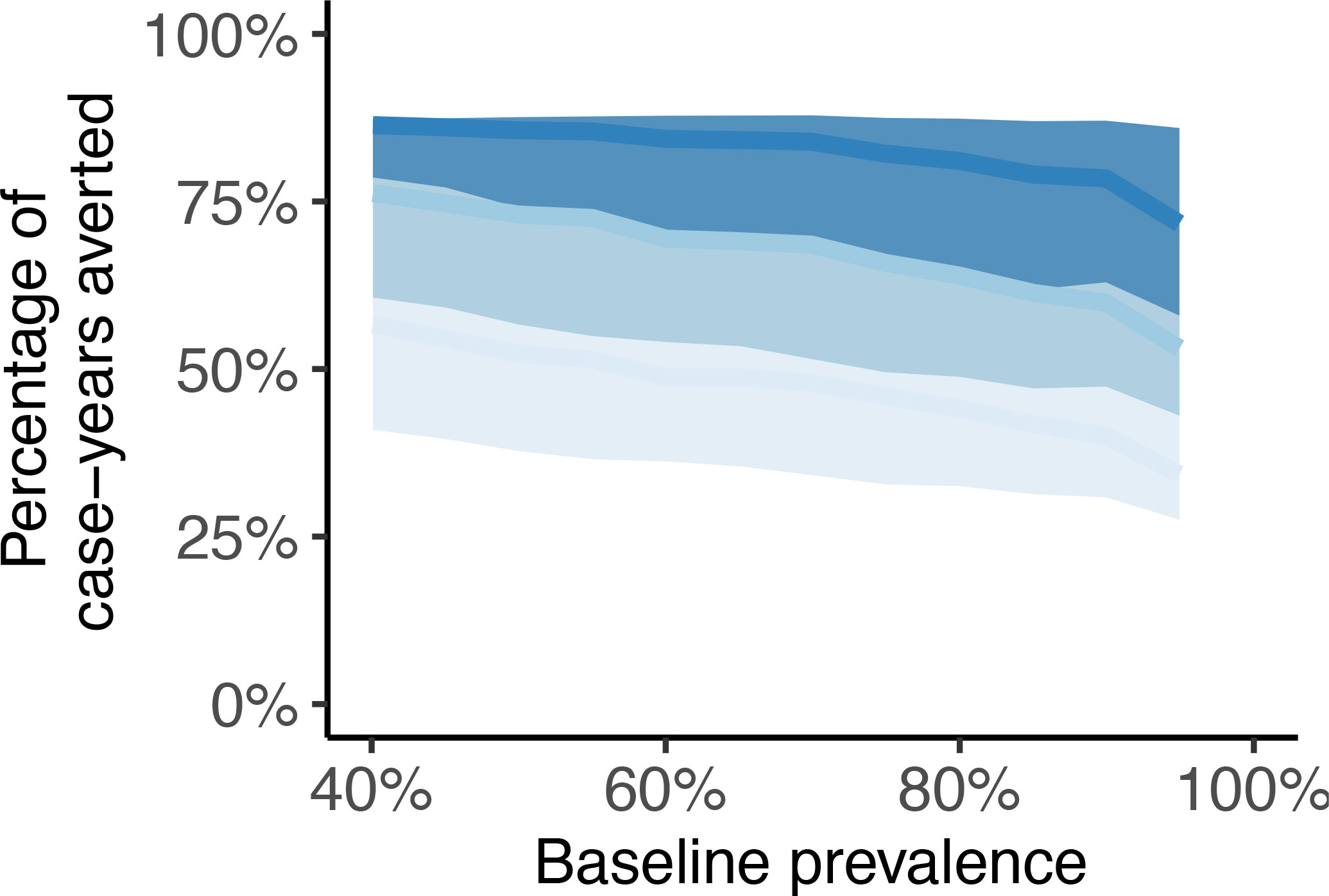
Figure 4 Case-years of bovine schistosomiasis averted by the end of 2030 as percentage of the (pre-intervention) baseline prevalence in 2022 using either n =1 (light blue), n = 2 (baby blue) or n = 4 (sapphire blue) POC-CCA tests administered per test-and-treat round. Solid lines depict the mean and colored bands 95% credible intervals.
The percentage of simulations reaching a prevalence of less than 1% by the end of 2030, defined nominally here as ‘elimination’ is shown in Figure 5. Elimination is an unlikely outcome when using n=1 or n=2 tests per TnT round, but for n=4 there is up to approximately 75% chance of achieving the 1% elimination target by the end of 2030 in low prevalence settings and, even in the highest prevalence settings, the chance is greater than 10%.
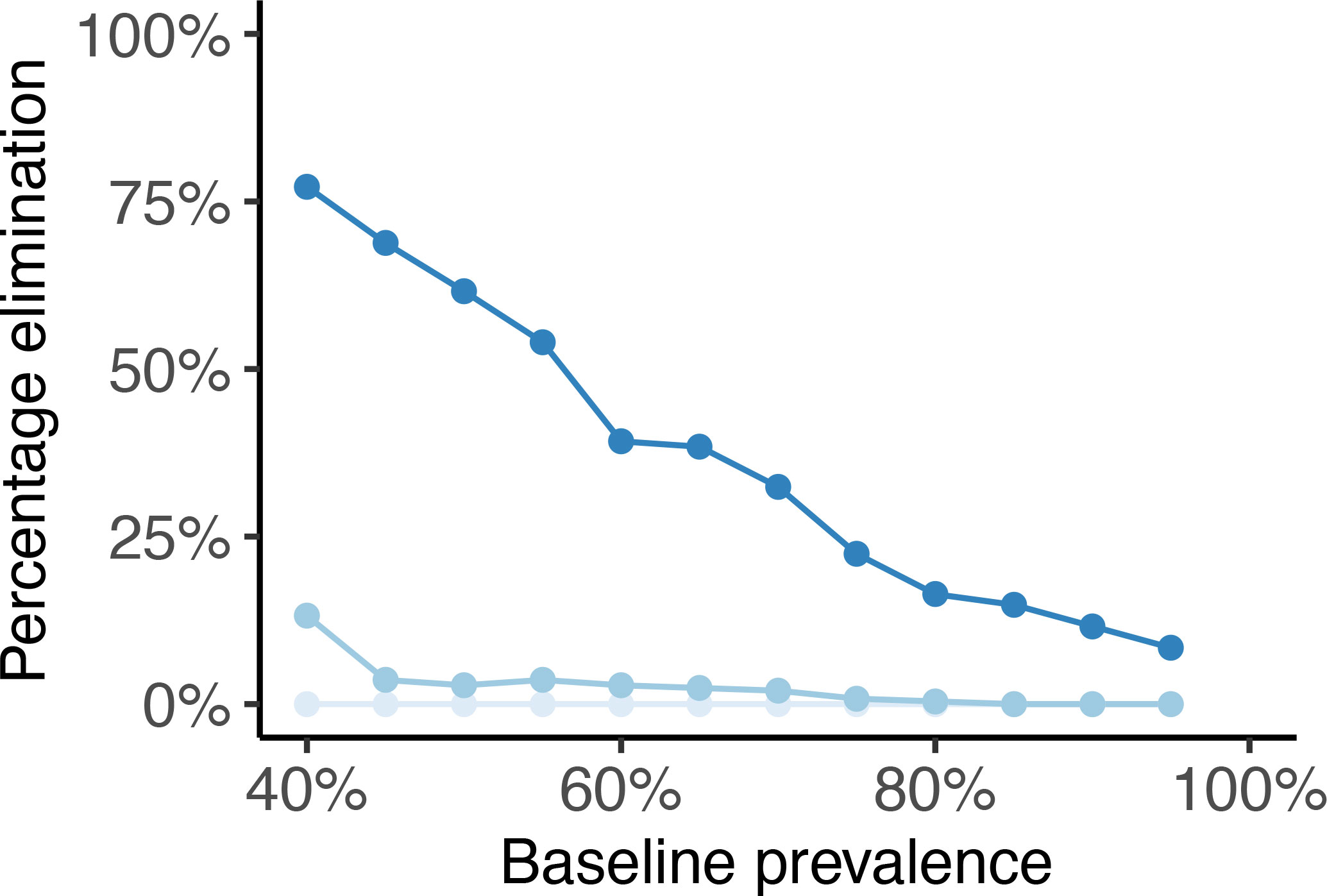
Figure 5 Percentage of bovine schistosomiasis simulations reaching less than 1% prevalence by the end of 2030 following nine annual rounds of test-and-treat (TnT) against (pre-intervention) baseline prevalence in 2022 using either n =1 (light blue), n = 2 (baby blue) or n = 4 (sapphire blue) POC-CCA tests administered per TnT round.
Diagnostic performance dynamics
Modelled changes in the PPV during the TnT intervention are shown in Figure 6. The dynamics illustrate how the PPV (or the probability of detecting at least one truly infected animal; ‘true positive’)—while being relatively high at pre-intervention baseline—declines during TnT with the declining prevalence of schistosomiasis (Figure 3). By 2030, for n=4 tests per TnT round, the PPV is less than 25% for 90% baseline prevalence and less than 5% for 50% and 70% baseline prevalence. The use of fewer tests retains a higher PPV because of the diminished likelihood of detecting a false positive—specificity declines in powers of n— and the reduced impact on schistosomiasis prevalence (Figures 3, 4).
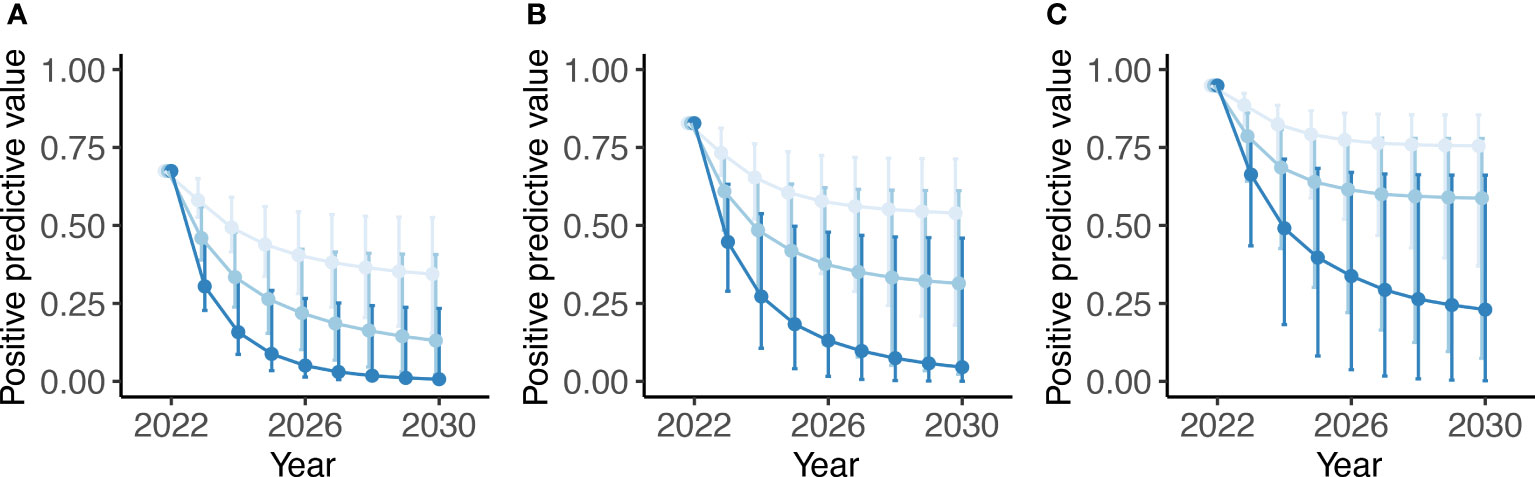
Figure 6 Modelled positive predictive value dynamics for detecting bovine schistosomiasis during a test-and-treat (TnT) intervention between 2022 and 2030 using either n =1 (light blue), n = 2 (baby blue) or n = 4 (sapphire blue) POC-CCA tests administered per TnT round. Dynamics are shown for a baseline prevalence of (A) 50%, (B) 70% and (C) 90% with uncertainty generated by 250 parameter sets yielding a baseline prevalence ± 1% of the target. In each panel, the point depicts the mean and the vertical bars the 95% credible interval.
Discussion
The recently launched WHO NTD 2021-2030 Roadmap and WHO guideline for the control and elimination of human schistosomiasis include within their strategy a consideration of potential control of infection in animal reservoirs (8, 30, 31). Here, we have explored the potential effectiveness of a TnT strategy (Figure 1) targeting bovine schistosomiasis motivated by epidemiological data collected in northern Senegal where the prevalence in bovines can be greater than 90% (Table 2). We show that a hypothetical herd-level TnT intervention running from 2022 through 2030 could have a substantial impact on bovine schistosomiasis, averting up to 85% of case-years (Figure 4) and, in lower prevalence settings, effecting a substantial chance of local elimination/interruption of transmission (Figure 5). Hence, if TnT was used at scale and targeted at multiple livestock species (i.e., whichever key hosts are playing a role in transmission within the area, such as goats, sheep and beyond, as well as cattle as simulated here), it could not only facilitate the WHO elimination goals for human schistosomiasis—by limiting spillover infection from animal reservoirs to humans and concurrent opportunities for interspecific hybridization—but also enhance productivity and livelihoods, further helping to lift people out of poverty.
Notwithstanding the clear potential benefits of treating livestock and reducing the burden of schistosomiasis, an equally important objective behind the TnT strategy is to avoid unnecessary treatment of uninfected herds. Achieving this objective also critically depends on the specificity of the diagnostic test used. We modelled TnT here using the POC-CCA diagnostic which was originally developed and validated for the detection of S. mansoni in humans (43), but that has also been recently shown to have moderate sensitivity (~62%) and specificity (~70%) for detecting intestinal livestock schistosomes caused by S. bovis and S. curassoni (35). The use of a POC test—as opposed to more specific traditional faecal parasitology—is important to facilitate the ease of implementing TnT strategies at scale. Moreover, our data show clearly that parasitology based on KK of livestock stool has very poor sensitivity (see Table 2 and Figure 2A). However, the estimated specificity of currently available POC-CCA may be too low to satisfy confidence requirements to avoid initiation and continuation of TnT in uninfected herds, thus ongoing work to enhance specificity for livestock usage should be prioritized, even at the cost of sensitivity. With improved specificity, adaptive strategies could also be developed to help meet the dual TnT objectives. For example, the use of a single test to identify and initiate TnT would minimize the risk of an initial false positive and subsequent testing could be implemented only after several completed treatment rounds to lower the number of potentially missed treatments. Alternatively, initial testing could aim to quantify herd-level prevalence (adjusting for sensitivity and specificity) and mathematical modelling used to project the likely number of treatment rounds required to reach pre-defined objectives.
Modelling the transmission dynamics of human schistosomiasis has been used extensively in recent years to evaluate the impact of MDA and to inform decision making on strategies to reach the WHO’s elimination goals (44–46). Modelling has, however, been seldom applied to livestock schistosomiasis, although some key modelling analyses have quantified contributions of zoonotic reservoirs and human-animal schistosome hybrids to infection dynamics in humans (10, 25). Nonetheless, there remains great uncertainty in our understanding of the population biology and transmission dynamics of livestock schistosomiasis, which we have tried to reflect in the modelling presented here. Some uncertainties are common to both human and livestock infection. For example, the role of acquired immunity, while long suspected to contribute to the epidemiological patterns observed in human schistosomiasis (47, 48), has not been elucidated completely in either humans or animals and its effects may alter the effectiveness of MDA (49). Moreover, a recent eco-evolutionary hypothesis linking worm-directed concomitant immunity and epidemiological patterns in humans presents further (potential) consequences for schistosome control (50). Other processes that regulate schistosome populations—such as density-dependent fecundity—are also debated and incompletely understood in both humans and animals (51). The impact on the transmission breakpoint (i.e., the minimum infection level that can sustain parasite transmission) of repeated MDA rounds has, however, been explored in a recent mathematical analysis of helminth burdens within a human population (42). Treatment non-compliance was highlighted as a key challenge that increases parasite overdispersion (smaller k) and further complicates elimination (by increasing parasite population resilience) (42). We have incorporated this important phenomenon into our model via a dynamic overdispersion parameter that varies through repeated TnT rounds. In addition, modelling schistosome dynamics in livestock is complicated by the often-fragmented nature of livestock herds in small rural communities, the impacts of seasonality on transmission risk and livestock migration (particularly amongst transhumance populations), as well as the limited understanding of environmental exposure (i.e., waterbody interaction) among dispersed populations.
Given these uncertainties, the modelling presented here should therefore be viewed as illustrative of the potential impact of TnT. Animals within herds are assumed to experience similar infection risk as they will be exposed to potentially contaminated water sources as a group. In the proposed simulated strategy, a representative small number of animals are tested to determine whether the herd is likely to be infected, and the whole herd is treated when an infected member of the group is detected. For simplicity and as proof-of-principle, we have only modelled schistosome burden in one animal host (bovines) within a perennial transmission location, given that in the Northern Senegal study area upon which model parameters are based, bovines are thought to act as the primary zoonotic reservoir of S. bovis (25). Notwithstanding, it is apparent, that both large and small ruminants can be heavily infected with blood flukes across Africa, and with Schistosoma species that can infect humans through viable hybridization with S. haematobium. In addition to this, wildlife, notably rodents and non-human primates can also play a significant role in zoonotic transmission of both intestinal and urogenital schistosomes to humans (52, 53). Thus, we have deliberately simplified many structural (e.g., inter-connected nature of herds) and biological (e.g., role of acquired and/or concomitant immunity; role of multiple animal reservoirs in schistosome transmission) complexities that would alter the transmission dynamics. Nonetheless, the clear contribution of cattle in spillover to humans in SSA in conjunction to the feasibility and numerous benefits of implementing control measures in these domestic ruminants (public and veterinary health benefits plus economic benefits) justify efforts to design a coherent and comprehensive strategy that includes them.
Nevertheless, there are numerous practical and logistical challenges that must be overcome to make TnT viable. Appropriate veterinary dosages and commercially available products tailored to livestock alongside stakeholder education would contribute to avoiding the current ad hoc use (and misuse) at livestock owners discretion (26) and minimize the risk of PZQ resistance. Critically, within Africa, PZQ is currently donated for human use only and while there may be some market to purchase PZQ by larger farmers because of the productivity benefits—particularly for small ruminants (27)—it is highly unlikely that a livestock-centric schistosomiasis program would be sustainable without some form of third-party donation.
Yet while there remain major challenges to the implementation of TnT, the evidence is now incontrovertible that livestock act as reservoirs for schistosome hybridization (9, 15–17, 20) and that livestock schistosomiasis causes significant economic and productivity losses (26, 27), compounding further the lives and livelihoods of impoverished populations. The need to address this challenge has been articulated by the WHO (8, 30). The findings we present here confirm that a TnT approach has the potential to be an effective strategy in controlling livestock schistosomiasis within a broader One Health approach eliminating NTDs.
Data availability statement
The original contributions presented in the study are publicly available. Code for dynamic transmission model and associated data available at https://github.com/martwalker/schistoTnT and for the statistical model at https://github.com/martwalker/trueprev. Further inquiries can be directed to the corresponding authors.
Ethics statement
The animal study was reviewed and approved by: (i) Imperial College London (London, UK) application 03.36; (ii) the CRERB at the Royal Veterinary College (London, UK) application URN20151327; and (iii) the Comité National d’Ethique pour la Recherche en Santé (Dakar, Senegal) application SEN15/68. Written informed consent was obtained from the owners for the participation of their animals in this study.
Author contributions
JW and MW conceptualized the work. MW, SL, MN, and AD developed the mathematical methodology. MW and AD carried out the formal analysis. EL, AB, ND, and JW, collected and analyzed the samples and the data that this work is based on, with fieldwork and permissions facilitated by MS. AD, MW, and JW wrote the original draft of the manuscript. All authors contributed to the article and approved the submitted version.
Funding
AD was funded by a Biotechnology and Biological Sciences Research Council (BBSRC) (London Interdisciplinary Doctoral Training Program funding studentship (grant number BB/M009513/1) under the supervision of JW and MW. The larger project that this work was embedded within was funded by the Biotechnology and Biological Sciences Research Council, the Department for International Development, the Economic & Social Research Council, the Medical Research Council, the Natural Environment Research Council and the Defence Science & Technology Laboratory, under the Zoonoses and Emerging Livestock Systems (ZELS : SR) program (grant number BB/S013822/1: ‘CATTLES - Control And Targeted Treatment for Livestock Emerging Schistosomiasis’); PI: JW, co-I MW, co-Is within Senegal: MS and ND), and a Research England grant: The Bloomsbury SET - Connecting Capability to Combat Infectious Disease and Antimicrobial Resistance project grant (grant number CCF-17-7779; PI: JW, co-Is MW and EL). The funders played no role in the writing of this manuscript nor in the decision to submit for publication.
Acknowledgments
We are thankful to the Senegalese communities, local facilitators and field teams involved in the ZELS project, particularly the late Cheikh Thiam, his family, and Alassane Ndiaye. Without their assistance in the collection and analysis of the samples, the data on which this work is based upon, would not have been created. We also thank Dr. Benjamin Collyer for discussions on modelling the overdispersion dynamics.
Conflict of interest
The authors declare that the research was conducted in the absence of any commercial or financial relationships that could be construed as a potential conflict of interest.
Publisher’s note
All claims expressed in this article are solely those of the authors and do not necessarily represent those of their affiliated organizations, or those of the publisher, the editors and the reviewers. Any product that may be evaluated in this article, or claim that may be made by its manufacturer, is not guaranteed or endorsed by the publisher.
References
1. WHO. Informal consultation on expanding schistosomiasis control in africa. facilitating PZQ access to scale up schistosomiasis control. Geneva: World Health Organization (2010).
2. WHO. Schistosomiasis: number of people treated worldwide in 2014. weekly epidemiological record. Switzerland: World Health Organization (2016).
3. WHO. Schistosomiasis: World health organization (2022). Available at: https://www.who.int/news-room/fact-sheets/detail/schistosomiasis.
4. Lai YS, Biedermann P, Ekpo UF, Garba A, Mathieu E, Midzi N, et al. Spatial distribution of schistosomiasis and treatment needs in sub-Saharan Africa: A systematic review and geostatistical analysis. Lancet Infect Dis (2015) 15(8):927–40. doi: 10.1016/S1473-3099(15)00066-3
5. Webster JP, Molyneux DH, Hotez PJ, Fenwick A. The contribution of mass drug administration to global health: past, present and future. Philos Trans R Soc B: Biol Sci (2014) 369(1645):20130434. doi: 10.1098/rstb.2013.0434
6. Deol AK, Fleming FM, Calvo-Urbano B, Walker M, Bucumi V, Gnandou I, et al. Schistosomiasis - assessing progress toward the 2020 and 2025 global goals. New Engl J Med (2019) 381(26):2519–28. doi: 10.1056/NEJMoa1812165
7. Kokaliaris C, Garba A, Matuska M, Bronzan RN, Colley DG, Dorkenoo AM, et al. Effect of preventive chemotherapy with praziquantel on schistosomiasis among school-aged children in sub-Saharan Africa: A spatiotemporal modelling study. Lancet Infect Dis (2022) 22(1):136–49. doi: 10.1016/S1473-3099(21)00090-6
8. WHO. Ending the neglect to attain the sustainable development goals: A road map for neglected tropical diseases 2021–2030. Geneva, Switzerland:World Health Organization (2020).
9. Léger E, Webster JP. Hybridizations within the genus Schistosoma: implications for evolution, epidemiology and control. Parasitology (2017) 144(1):65–80. doi: 10.1017/S0031182016001190
10. Rudge JW, Webster JP, Lu DB, Wang TP, Fang GR, Basáñez MG. Identifying host species driving transmission of schistosomiasis japonica, a multihost parasite system, in China. Proc. Natl. Acad. Sci. U.S.A. (2013) 110(28):11457–62. doi: 10.1073/pnas.1221509110
11. Wang W, Bergquist R, King CH, Yang K. Elimination of schistosomiasis in China: Current status and future prospects. PloS Negl Trop Dis (2021) 15(8):e0009578. doi: 10.1371/journal.pntd.0009578
12. Zhang LJ, Dai SM, Xue JB, Li YL, Lv S, Xu J, et al. The epidemiological status of schistosomiasis in p. r. China after the world bank loan project, 2002-2017. Acta Trop (2019) 195:135–41. doi: 10.1016/j.actatropica.2019.04.030
13. De Bont J, Vercruysse J. Schistosomiasis in cattle. Adv Parasitol (1998) 41:285–364. doi: 10.1016/S0065-308X(08)60426-1
14. Webster BL, Diaw OT, Seye MM, Webster JP, Rollinson D. Introgressive hybridization of Schistosoma haematobium group species in Senegal: Species barrier break down between ruminant and human schistosomes. PloS Negl Trop Dis (2013) 7(4):e2110. doi: 10.1371/journal.pntd.0002110
15. Webster BL, Alharbi MH, Kayuni S, Makaula P, Halstead F, Christiansen R, et al. Schistosome interactions within the Schistosoma haematobium group, Malawi. Emerg Infect Dis (2019) 25(6):1245–7. doi: 10.3201/eid2506.190020
16. Léger E, Borlase A, Fall CB, Diouf ND, Diop SD, Yasenev L, et al. Prevalence and distribution of schistosomiasis in human, livestock, and snail populations in northern Senegal: A one health epidemiological study of a multi-host system. Lancet Planet Health (2020) 4(8):e330–e42. doi: 10.1016/S2542-5196(20)30129-7
17. Savassi B, Mouahid G, Lasica C, Mahaman SK, Garcia A, Courtin D, et al. Cattle as natural host for Schistosoma haematobium (Bilharz, 1852) weinland, 1858 x schistosoma bovis sonsino, 1876 interactions, with new cercarial emergence and genetic patterns. Parasitol Res (2020) 119(7):2189–205. doi: 10.1007/s00436-020-06709-0
18. Angora EK, Allienne JF, Rey O, Menan H, Toure AO, Coulibaly JT, et al. High prevalence of Schistosoma haematobium x Schistosoma bovis hybrids in schoolchildren in Côte d'Ivoire. Parasitology (2020) 147(3):287–94. doi: 10.1017/S0031182019001549
19. Onyekwere AM, Rey O, Allienne JF, Nwanchor MC, Alo M, Uwa C, et al. Population genetic structure and hybridization of Schistosoma haematobium in Nigeria. Pathogens (2022) 11(4):425. doi: 10.3390/pathogens11040425
20. Léger E, Garba A, Hamidou AA, Webster BL, Pennance T, Rollinson D, et al. Introgressed animal schistosomes Schistosoma curassoni and S. bovis naturally infecting humans. Emerg Infect Dis (2016) 22(12):2212–4. doi: 10.3201/eid2212.160644
21. Fall CB, Lambert S, Léger E, Yasenev L, Garba AD, Diop SD, et al. Hybridized Zoonotic Schistosoma infections result in hybridized morbidity profiles: A clinical morbidity study amongst Co-infected human populations of Senegal. Microorganisms (2021) 9(8):1776. doi: 10.3390/microorganisms9081776
22. Boissier J, Grech-Angelini S, Webster BL, Allienne JF, Huyse T, Mas-Coma S, et al. Outbreak of urogenital schistosomiasis in Corsica (France): An epidemiological case study. Lancet Infect Dis (2016) 16(8):971–9. doi: 10.1016/S1473-3099(16)00175-4
23. Wellinghausen N, Mone H, Mouahid G, Nebel A, Tappe D, Gabriel M. A family cluster of schistosomiasis acquired in Solenzara river, Corsica (France) - Solenzara river is clearly a transmission site for schistosomiasis in Corsica. Parasitol Res (2022) 121(8):2449–52. doi: 10.1007/s00436-022-07574-9
24. Oleaga A, Rey O, Polack B, Grech-Angelini S, Quilichini Y, Pérez-Sánchez R, et al. Epidemiological surveillance of schistosomiasis outbreak in Corsica (France): Are animal reservoir hosts implicated in local transmission? PloS Negl Trop Dis (2019) 13(6):e0007543. doi: 10.1371/journal.pntd.0007543
25. Borlase A, Rudge JW, Léger E, Diouf ND, Fall CB, Diop SD, et al. Spillover, hybridization, and persistence in schistosome transmission dynamics at the human-animal interface. Proc Natl Acad Sci U.S.A. (2021) 118(41):e2110711118. doi: 10.1073/pnas.2110711118
26. Gower CM, Vince L, Webster JP. Should we be treating animal schistosomiasis in Africa? the need for a one health economic evaluation of schistosomiasis control in people and their livestock. Trans R Soc Trop Med Hyg (2017) 111(6):244–7. doi: 10.1093/trstmh/trx047
27. Adeyemo P, Léger E, Hollenberg E, Diouf N, Séne M, Webster JP, et al. Estimating the financial impact of livestock schistosomiasis on traditional subsistence and transhumance farmers keeping cattle, sheep and goats in northern Senegal. Parasites Vectors (2022) 15(1):101. doi: 10.1186/s13071-021-05147-w
28. Calavas D, Martin PM. Schistosomiasis in cattle in Corsica, France. Emerg Infect Dis (2014) 20(12):2163–4. doi: 10.3201/eid2012.141474
29. Vercruysse J. Schistosomiasis: MSD veterinary manual (2015). Available at: https://www.msdvetmanual.com/circulatory-system/blood-parasites/schistosomiasis.
30. WHO. WHO guideline on control and elimination of human schistosomiasis. Geneva: World Health Organization (2022).
31. Lo NC, Bezerra FSM, Colley DG, Fleming FM, Homeida M, Kabatereine N, et al. Review of 2022 WHO guidelines on the control and elimination of schistosomiasis. Lancet Infect Dis (2022) S1473–3099(22):00221–3. doi: 10.1016/S1473-3099(22)00221-3
32. Vercruysse J, Claerebout E. Resistance to anthelmintics: MSD veterinary manual (2014). Available at: https://www.msdvetmanual.com/pharmacology/anthelmintics/resistance-to-anthelmintics.
33. van Lieshout L, Polderman AM, Deelder AM. Immunodiagnosis of schistosomiasis by determination of the circulating antigens CAA and CCA, in particular in individuals with recent or light infections. Acta Trop (2000) 77(1):69–80. doi: 10.1016/S0001-706X(00)00115-7
34. Colley DG, Bustinduy AL, Secor WE, King CH. Human schistosomiasis. Lancet (2014) 383(9936):2253–64. doi: 10.1016/S0140-6736(13)61949-2
35. Calvo-Urbano B, Léger E, Gabain I, De Dood CJ, Diouf ND, Borlase A, et al. Sensitivity and specificity of human point-of-care circulating cathodic antigen (POC-CCA) test in African livestock for rapid diagnosis of schistosomiasis: A Bayesian latent class analysis. bioRxiv (2022). doi: 10.1101/2022.08.17.504243
36. Anderson RM, May RM. Helminth infections of humans: mathematical models, population dynamics, and control. Adv Parasitol (1985) 24:1–101. doi: 10.1016/S0065-308X(08)60561-8
37. WHO. Assessing the efficacy of anthelminthic drugs against schistosomiasis and soil-transmitted helminthiases Vol. 2013. Geneva: World Health Organization (2013).
38. Plummer M. Rjags: Bayesian graphical models using MCMC: R package version 4-12. (2021) Vienna, Austria:R Foundation for Statistical Computing
39. R Core Team. R: A language and environment for statistical computing. Vienna, Austria: R Foundation for Statistical Computing (2020).
40. May RM. Togetherness among schistosomes: Its effects on the dynamics of the infection. Math Biosci (1977) 35:301–43. doi: 10.1016/0025-5564(77)90030-X
41. Anderson RM, May RM. Infectious diseases of humans: dynamics and control. Oxford: Oxford University Press (1991).
42. Collyer BS, Anderson RM. Probability distributions of helminth parasite burdens within the human host population following repeated rounds of mass drug administration and their impact on the transmission breakpoint. J R Soc Interface (2021) 18(177):20210200. doi: 10.1098/rsif.2021.0200
43. Colley DG, Binder S, Campbell C, King CH, Tchuem Tchuente LA, N'Goran EK, et al. A five-country evaluation of a point-of-care circulating cathodic antigen urine assay for the prevalence of Schistosoma mansoni. Am J Trop Med Hyg (2013) 88(3):426–32. doi: 10.4269/ajtmh.12-0639
44. Kura K, Hardwick RJ, Truscott JE, Toor J, Hollingsworth TD, Anderson RM. The impact of mass drug administration on Schistosoma haematobium infection: What is required to achieve morbidity control and elimination? Parasites Vectors (2020) 13(1):554. doi: 10.1186/s13071-020-04409-3
45. Toor J, Rollinson D, Turner HC, Gouvras A, King CH, Medley GF, et al. Achieving elimination as a public health problem for Schistosoma mansoni and S. haematobium: When is community-wide treatment required? J Infect Diseases (2020) 221(Suppl 5):S525–S30. doi: 10.1093/infdis/jiz609
46. NTD Modelling Consortium Schistosomiasis Group. Insights from quantitative and mathematical modelling on the proposed WHO 2030 goal for schistosomiasis. Gates Open Res (2019) 19(3):1517. doi: 10.12688/gatesopenres.13052.2
47. Mitchell KM, Mutapi F, Savill NJ, Woolhouse ME. Explaining observed infection and antibody age-profiles in populations with urogenital schistosomiasis. PloS Comput Biol (2011) 7(10):e1002237. doi: 10.1371/journal.pcbi.1002237
48. Woolhouse ME. Patterns in parasite epidemiology: the peak shift. Parasitol Today (1998) 14(10):428–34. doi: 10.1016/S0169-4758(98)01318-0
49. Kura K, Hardwick RJ, Truscott JE, Anderson RM. What is the impact of acquired immunity on the transmission of schistosomiasis and the efficacy of current and planned mass drug administration programmes? PloS Negl Trop Dis (2021) 15(12):e0009946. doi: 10.1371/journal.pntd.0009946
50. Buck JC, De Leo GA, Sokolow SH. Concomitant immunity and worm senescence may drive schistosomiasis epidemiological patterns: An eco-evolutionary perspective. Front Immunol (2020) 11:160. doi: 10.3389/fimmu.2020.00160
51. Neves MI, Gower CM, Webster JP, Walker M. Revisiting density-dependent fecundity in schistosomes using sibship reconstruction. PloS Negl Trop Dis (2021) 15(5):e0009396. doi: 10.1371/journal.pntd.0009396
52. Webster JP, Gower CM, Knowles SC, Molyneux DH, Fenton A. One health - an ecological and evolutionary framework for tackling neglected zoonotic diseases. Evol Appl (2016) 9(2):313–33. doi: 10.1111/eva.12341
Keywords: modelling, schistosomiasis, livestock, test-and-treat, One Health
Citation: Díaz AV, Lambert S, Neves MI, Borlase A, Léger E, Diouf ND, Sène M, Webster JP and Walker M (2022) Modelling livestock test-and-treat: A novel One Health strategy to control schistosomiasis and mitigate drug resistance. Front. Trop. Dis 3:893066. doi: 10.3389/fitd.2022.893066
Received: 09 March 2022; Accepted: 20 July 2022;
Published: 07 September 2022.
Edited by:
Stephen R. Doyle, Wellcome Sanger Institute (WT), United KingdomReviewed by:
Hugues C. Nana Djeunga, Centre for Research on Filariasis and other Tropical Diseases (CRFilMT), CameroonPedro Manuel Ferreira, New University of Lisbon, Portugal
Tiago M. F. Mendes, State University of Campinas, Brazil
Copyright © 2022 Díaz, Lambert, Neves, Borlase, Léger, Diouf, Sène, Webster and Walker. This is an open-access article distributed under the terms of the Creative Commons Attribution License (CC BY). The use, distribution or reproduction in other forums is permitted, provided the original author(s) and the copyright owner(s) are credited and that the original publication in this journal is cited, in accordance with accepted academic practice. No use, distribution or reproduction is permitted which does not comply with these terms.
*Correspondence: Adriana V. Díaz, YWRpYXpAcnZjLmFjLnVr; Joanne P. Webster, am93ZWJzdGVyQHJ2Yy5hYy51aw==; Martin Walker, bXdhbGtlckBydmMuYWMudWs=
†These authors have contributed equally to this work and share senior authorship