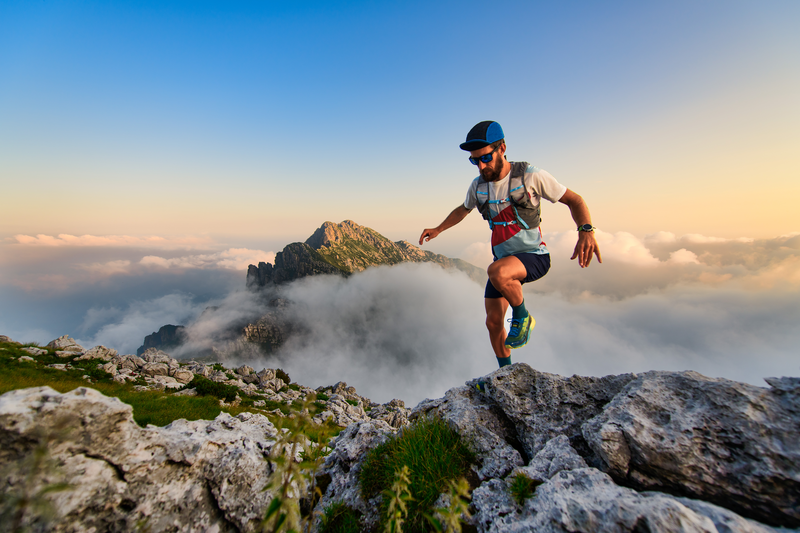
95% of researchers rate our articles as excellent or good
Learn more about the work of our research integrity team to safeguard the quality of each article we publish.
Find out more
ORIGINAL RESEARCH article
Front. Trop. Dis. , 06 April 2022
Sec. Vector Biology
Volume 3 - 2022 | https://doi.org/10.3389/fitd.2022.875031
This article is part of the Research Topic Mosquitoes and Their Role in Disease Transmission: Recent Advances and Innovations View all 9 articles
The (re) emergence of arboviruses around the world is a public health concern once severe outbreaks are usually associated with these infections. The co-circulation of Dengue (DENV), Zika (ZIKV) and Chikungunya (CHIKV) viruses in the past few years has caused a unique epidemic situation in Brazil. The northeast region of the country was the most affected by clinical complications from such arboviruses’ infections, including neurological disorders caused by ZIKV. In this particular region, Aedes mosquitoes are the main vectors of DENV, ZIKV and CHIKV, with Culex quinquefasciatus also considered as a potential vector of ZIKV. Therefore, virological surveillance in mosquitoes contributes to understanding the epidemiological profile of these diseases. Here, we report the circulation of DENV, ZIKV and CHIKV in Aedes spp. and Cx. quinquefasciatus female mosquitoes collected in areas with a high arbovirus circulation in humans in the Metropolitan Region of Recife, Pernambuco, Brazil, during the triple-epidemics of 2015-17. All the field-caught mosquitoes were sent to the laboratory for arbovirus screening after RNA extraction and RT-PCR/RT-qPCR. A total of 6,227 females were evaluated and, as a result, DENV, ZIKV and CHIKV were identified in Ae. aegypti, Ae. taeniorhynchus and Cx. quinquefasciatus mosquito pools. In addition, DENV and ZIKV were isolated in C6/36 cells. In conclusion, it is important to highlight that arbovirus surveillance performed in mosquitoes from DENV-ZIKV-CHIKV hotspots areas can serve as an early-warning system to target vector control actions more efficiently in each studied area.
Vector-borne diseases have expanded its geographical distribution in the past decades due to a myriad of factors, such as globalization, climate changes and the lack of specific therapeutics or vaccines (1, 2). Dengue virus (DENV), an arbovirus mainly transmitted to humans through the bite of Aedes aegypti and Aedes albopictus mosquitoes, was estimated to annually infect 80-100 million humans in 1998 (1, 3) and now is predicted to infect nearly 400 million people per year (4). Currently, Brazil is the country with the largest number of dengue fever cases in the world (5) with expected outbreak cycles occurring every three years (6). In 2015, Brazilian authorities reported a triple-arboviral epidemics of Dengue, Zika (ZIKV) and Chikungunya (CHIKV) viruses (7). With over 200,000 cases reported in 2016 (8), ZIKV infection was associated to neurological complications such as Guillain-Barré Syndrome in adults and Congenital Zika Syndrome (CZS) (9, 10). The Brazilian Northeast region was the most affected area by ZIKV and, the city of Recife (capital of Pernambuco state) reported the highest number of CZS cases in the country (9, 10). Recife displays favorable conditions for arboviruses outbreaks: poor sanitation structure, high densities of mosquito vectors, unsatisfactory healthcare structure, informal low-income housing, among others (11).
Regarding high densities of mosquito vectors, monitoring viruses in field-collected mosquito vectors is a key element in arboviruses surveillance programs, that can serve as a warning system to guide the appropriate measures prior to virus notification in humans (12–14). Molecular epidemiology studies can also be carried out based on entomological surveillance with virus detection, which allows the investigation of viral evolution or adaptation, as well as identifying new strains (15, 16). In addition, massive screening of arboviruses in mosquito species may also provide data over which vectors are participating in the local virus dynamics (14, 17). Since the public health emergency of international concern caused by the broad ZIKV circulation, several groups have reported a large dataset of diversified results of arbovirus detection in mosquitoes from around the world. In Brazil, for instance, Ae. aegypti mosquitoes from Rio de Janeiro were found naturally-infected with ZIKV during surveillance conducted in 2015-16, with no evidence of infection in Ae. albopictus and Culex quinquefasciatus (18). Arbovirus-surveillance from two independent datasets of field-collected mosquitoes from Recife (Northeast Brazil) and Vitória (Southeast Brazil) in 2016 showed the circulation of ZIKV not only in Ae. aegypti, but also in Cx. quinquefasciatus with no signs of recent bloodmeal (19, 20).
The proliferation of vectors, such as Ae. aegypti and Cx. quinquefasciatus, have been associated with environmental, ecological and socioeconomic factors, as arbovirus transmission has increased over the years in several parts of the world (21). In Brazil, regions with the highest risk of arbovirus transmission display all the above-mentioned factors, coupled with inadequate vector control strategies (22). In an attempt to reduce arbovirus circulation in the country, public health authorities usually rely on the massive use of chemical insecticides as the main strategy, which results in the selection of resistant mosquitoes (23, 24).
The main goal of the present study was to conduct an extensive arbovirus surveillance of DENV, ZIKV and CHIKV in natural populations of Aedes and Culex mosquitoes, collected in hotspots (areas previously known that have a higher probability of arbovirus circulation) from the Metropolitan Region of Recife (MRR), during the triple-epidemics reported from 2015 to 2017. In order to understand the dynamics of transmission, direct vector control and monitor the evolution of detected viruses.
The study was conducted in the Metropolitan Region of Recife (MRR), in 236 collection sites distributed in six municipalities: Recife, Jaboatão dos Guararapes, Olinda, Camaragibe, Paulista and São Lourenço da Mata (Figure 1). Climate conditions in these municipalities are predominantly hot and humid, with temperatures ranging from 25°C to 32°C and humidity varies between 60-70%. Most of the collection sites were located in the state capital, Recife, which has approximately a population of 1,537,704 habitants and 218,435 km² of area (25). All the selected cities have large urban settings, except São Lourenço da Mata.
Figure 1 Maps of the Metropolitan Region of Recife with collection sites and densities of infected mosquitoes. (A) Map of Brazil with Pernambuco state in red; (B) Map of Pernambuco state with MRR in red; (C) Study area with collection sites; (D) Density of DENV infected; (E) Density of CHIKV infected mosquitoes; (F) Density of ZIKV infected mosquitoes.
Viral stocks used as positive controls for the assays were ZIKV BRPE243/2015 (KX197192), CHIKV BRPE408/2016, DENV-1 42735/BR-PE (EU259529), DENV-2 3808/BR-PE (EU259569), DENV-3 85469/BRPE (EU259607) and DENV-4 1385 (U18425) (were all kindly provided by Dr. Marli Tenório, LAVITE-AMI). DENV positive control for conventional RT-PCR was obtained by RNA extraction from the viral stocks, while the standard curve of ZIKV and CHIKV used in RT-qPCR was synthesized by in vitro transcription using MEGAscript T7 kit (Ambion, catalog #AM1334, Carlsbad, CA, USA). Each virus standard was quantified in Nanodrop 2000 and RNA concentration was converted in RNA copy number (26).
Adult mosquitoes were obtained using battery-operated aspirators (Horst Armadilhas Ltd, São Paulo, Brazil) between January/2015 and December/2017, from houses with suspected arboviral human cases (either suspected or laboratory-confirmed) and public places, such as universities, public squares and government buildings. Adult mosquitoes were also collected from hospitals and Emergency Care Units (Unidades de Pronto Atendimento - UPA) which are places considered reference in clinical care and monitoring of arboviruses.
Field-caught mosquitoes were immediately sent in aspiration bags to the Entomology Laboratory – Aggeu Magalhães Institute (AMI), where individuals were kept at -20°C for 30 minutes and, morphologically identified on ice-chilled Petri dishes using taxonomic keys (27). Mosquito specimen were sorted in pools (up to 10 mosquitoes) by locality, species, sex and feeding status (blood-engorged and non-engorged). And then they were transferred to 1.5 ml DNAse/RNAse free microtubes and stored at -80°C until further usage. Prior to RNA extraction, each mosquito pool was homogenized as described in Barbosa et al. (28).
RNA extraction protocol was carried out as described in Guedes et al. (20). Two approaches were used for arbovirus detection, conventional RT-PCR for DENV and multiplex RT-qPCR for ZIKV and CHIKV. DENV molecular detection was performed using a single-tube Multiplex RT-PCR, as described in Barbosa et al. (28). Each PCR run was performed using a positive control and two negative controls (one comprising all RT- PCR reagents except RNA template and the other one, the negative control from RNA extraction). PCR products were run for 50 minutes at 120V on a 1.5% agarose gel stained with ethidium bromide and visualized on a UV transilluminator. Due to the recent introduction of both ZIKV and CHIKV in the northeastern region in 2015, laboratory diagnosis protocols were adapted to increase the sensitivity of the reaction and to simultaneous detection of arbovirus in the same sample. A quantitative RT-PCR for both ZIKV and CHIKV (Duplex) was assayed in a QuantiStudio 5 system (Applied BioSystems, Foster City, CA, USA). Reactions were performed using the QuantiNova Probe RT-PCR kit (Qiagen, catalog #208354, Hilden, Germany) in a 15 μl final volume, with primers of both viruses in a 0.8 μM final concentration and probes in a 0.1 μM final concentration. Primers and probes are described in Table 1. All samples were tested in duplicates, using a standard curve consisting of a serial dilution of previously quantified RNA transcript and negative controls (all reagents except RNA and negative control from RNA extraction). RT-qPCR results were analyzed using QuantStudio Design and Analysis Software 1.3.1 (Thermo Fisher Scientifc) with automatic threshold and baseline. Samples that produced Cq values of ≤ 38.5 in both duplicates were considered positive.
Table 1 Primers and probes sequences used in DENV, CHIKV and ZIKV with field-caught mosquito collected from 2015 to 2017 in Pernambuco, Brazil.
After DENV, ZIKV and CHIKV detection in mosquito pools, we calculated the Minimum Infection Rate (MIR) as the following formula: (the number of positive pools/total number of mosquitoes tested) × 1,000, for each virus and species, according to Chow et al. (32).
To confirm the feeding status in each pool of mosquitoes identified as positive for any arbovirus tested, we use a well-stablished PCR protocol based on mitochondrial DNA identification (33). DNA was extracted from a 50 μl aliquot of the stored homogenates using an alcohol precipitation protocol described by Ayres et al. (34). Each PCR run contained a positive control (DNA from a human blood sample) and negative controls (all reagents except DNA and negative control from DNA extraction). Confirmation of the amplified fragments was performed on 1.5% agarose gel for 50 minutes at 120V, stained with ethidium bromide and visualized in U.V. After confirmation, PCR products were sequenced on ABI 3500xL Genetic Analyzer sequencer (Applied Biosystems), using BigDye® (Applied Biosystems Foster City, CA, USA). The sequencing was carried out at the AMI/FIOCRUZ Technological Platforms Nucleus. Sequences were then edited, contigs assembled and aligned with CodonCode Analyzer program (v.3.7.1) and compared with the sequences previously archived in GenBank, through the Basic Local Alignment Search Tool (BLAST) (https://blast.ncbi.nlm.nih.gov/blast.cgi).
For virus isolation from positive mosquito pools, twenty microliters of arbovirus-confirmed homogenates (samples with Cq values that ranged from 13.2 to 38.3 are described in Table 2) were inoculated into confluent monolayers C6/36 cells for 1 hour, in 10 cm² cell culture tubes (TTP®, Techno Plastic Products AG, Trasadingen, Switzerland) with Leibovitz Medium (L-15, Gibco, catalog #41300-039, Carlsbad, CA, USA) supplemented with 2% of fetal bovine serum and 1% of antifungal (Fungizone) (Gibco, catalog # 15290-018, Carlsbad, CA, USA) and antibiotic (Penicillin/Streptomycin) (Gibco, catalog #15140-122, Carlsbad, CA, USA). Cell culture flasks were incubated at 27°C, during seven days for cytopathic effects (CPE) visualization. After CPE visualization, samples were frozen and stored at −80°C for further usage. This procedure was repeated for four passages. Viral isolation was monitored by RT-qPCR in each passage (Table 2), as previously described for both ZIKV and CHIKV. DENV reactions were assayed using the QuantiTect SYBR® Green RT-PCR Kit (Qiagen, catalog # 204243, German) in a 20 μl final volume with primers described by Kong et al. (26) in a 0.2 μM final concentration.
Table 2 Description of 32 positive pools for DENV, ZIKV and CHIKV inoculated in C6/36 cell culture during arbovirus surveillance in Pernambuco, Brazil from 2015 to 2017.
For confirmation of viral isolation, samples that resulted in CPE or increased viral load on the RT-qPCR assay were submitted to an indirect immunofluorescence assay (IFA). At the 4th (CHIKV) and 6th day (DENV and ZIKV) after inoculation in cell culture flasks, an aliquot of 10 μl of each cell suspension, obtained from cell scraping the vials, was deposited on IFA slides. IFA slides were dry at room temperature, and then fixed with acetone P.A for 30 minutes at - 20°C. The primary antibody (Anti-DENV 2, Anti-4G2 for ZIKV and Anti-CHIKV) was added to IFA slides and then incubated for one hour in a humid chamber at 37°C. Slides were then washed twice with 1x PBS and the secondary antibody (Anti-mouse) was added, following incubation of one-hour in a humid 37°C chamber. After incubation, slides were again washed twice with 1x PBS and dried for 5 minutes. After drying, slides were mounted in VECTASHIELD® Medium with DAPI (Vector Laboratories, California, USA) under the coverslip. Slides were visualized in a Fluorescence microscope (Leica Microsystems, Germany) using the green filter (FIT C) for virus fluorescence and the blue filter (DAPI) for cell nuclei fluorescence. The images were captured by the microscope software on the 400x objective.
From 2015 to 2017, 9,834 adult mosquitoes were obtained from which 6,227 were identified as females. All the females were pooled in a total of 1,049 pools (497 of Ae. aegypti and 549 of Cx. quinquefasciatus) that were assayed for conventional and quantitative RT-PCRs. Ae. albopictus, Aedes taeniorhynchus and Aedes scapularis specimens were also collected and assayed as one pool each. After molecular analysis, a total of 219 pools (20.8%) were positive for at least one of the tested arboviruses. In summary, DENV was detected in 19 pools (89.5% DENV2 and 10.5% DENV4), ZIKV was detected in 194 pools (Cq values described in Figure 2) and CHIKV in six pools. Cq values found ranged from 13.2 to 38.3 (equivalent to 5,22E+19 and 1,89E+07 RNA copy number). Out of the 219 positive-pools, five pools of Ae. aegypti and four of Cx. quinquefasciatus resulted in double infection of DENV2 and ZIKV. In addition, a simultaneous DENV2 and DENV4 infection was detected in a single pool of Cx. quinquefasciatus. No virus was detected in Ae. scapularis and Ae. albopictus samples. The distribution of the positive samples is mapped in Figure 1. From the total of positive samples, 150 were identified as blood-fed females after PCR confirmation (Supplementary Figure 1). The sequencing analysis of the pools that contained engorged females revealed the presence of human blood in all samples.
Figure 2 Cq values of positive Ae. aegypti and Cx. quinquefasciatus pools for ZIKV plotted using GraphPad Prism 9 software. The red traced line represents the threshold used for positive samples (Cq = 38.5).
Concerning DENV surveillance in field-caught mosquitoes, pools were tested by conventional RT-PCR. In 2015, DENV was detected in 10 pools of Ae. aegypti samples. In 2016, four pools of Ae. aegypti and four pools of Cx. quinquefasciatus were positive for DENV. In 2017 only one pool was positive for DENV. Minimum infection rate (MIR) for DENV ranged from 0.68 to 13.77 (Table 3). With regards to ZIKV in 2015, 30 pools of Ae. aegypti and one pool of Ae. taeniorhynchus from a hospital were positive. In 2016, 40 pools of Ae. aegypti and 69 pools of Cx. quinquefasciatus were ZIKV positive. In 2017, 27 pools of Ae. aegypti and 27 pools of Cx. quinquefasciatus were ZIKV-positive, with a MIR ranging from 18.59 to 41.32 (Table 3). CHIKV was detected in two pools of Ae. aegypti in 2015 and in four pools of Ae. aegypti in 2017. The MIR observed for both years was 2.75 (Table 3). For virus isolation, all positive samples for DENV and CHIKV and samples with the lowest Cq values for ZIKV were selected (Table 1). A total of 32 samples out of 219 positive sample were inoculated in a C6/36 cells monolayer. After inoculation, virus isolation was confirmed by cytopathic effects (CPE) visualization in three positive samples for DENV (13DE/2015RA, 17F/2015HA and 18/2015HA) and in one sample for ZIKV (1A/2015HA). RT-qPCR and indirect immunofluorescence assays also confirmed the isolation of DENV and ZIKV (Figure 3).
Table 3 Description of results of arbovirus infection obtained from mosquito samples collected in the metropolitan region of recife in three distinct years.
Figure 3 ZIKV visualization in C6/36 cells by immunofluorescence assay and isolated from field-caught mosquitoes. Image captured with 400x objective. (A–C) Immunofluorescence of non-infected C6/36 cells; (D–F) Immunofluorescence of C6/36 cells inoculated with the sample 1A / 2015HA and (G–I) Immunofluorescence of infected C6/36 cells with the positive control of ZIKV. ZIKV is labelled in green (FIT C) and the nucleus is labelled in blue (DAPI).
Environmental and ecological settings, combined with areas of poor living conditions such as: overcrowding, inadequate sewage system and discontinuing water supply forge the Metropolitan Region of Recife (MRR) as a year-long hotspot for arbovirus transmission, resulting in a permanent vector control issue. In our current surveillance study, we collected, during a triple epidemic of DENV-ZIKV-CHIKV from 2015 to 2017, adult mosquitoes in different sites located at the Metropolitan Region of Recife (MRR), Pernambuco State, Brazil where arbovirus-suspected cases were reported in the human population (hotspots). From over 1,049 pools screened (over 6,000 females), 219 were RT-PCR/RT-qPCR positive for at least one of the viruses (DENV, ZIKV and CHIKV). The spatial distribution of these positive samples showed a higher risk of DENV, ZIKV and CHIKV transmission in low and medium low class neighborhoods of MRR. Our findings for ZIKV corroborate with the study by Souza and collaborators (2018) (11), which demonstrated a higher prevalence of ZIKV and microcephaly in environments of Recife with a high level of poverty. This critical scenario indicates the need to put even more effort into environmental health improvements.
Considering DENV data, while our results showed the circulation of DENV4 in those mosquitoes, not a single infection with this particular serotype was confirmed in 2016-17 in the human population from Recife (35). DENV2 and DENV3 were the only serotypes confirmed in the human population during 2016 unlike what we found in mosquito’s population (35). Such results acted as an early warning as DENV2 became the most frequent serotype circulating in Brazil in 2019 (two years after our study) (36). In addition to DENV, CHIKV was also detected in Ae. aegypti collected in the different sites from the MRR. Our results of CHIKV MIR are similar to those reported by Cevallos and collaborators (2018) (37), who conducted an arbovirus surveillance in Ae. aegypti from active epidemic urban areas. However, mosquitoes were collected during the epidemics, the MIR value of CHIKV was lower than those reported in Ae. aegypti samples from Maranhão and Aracaju (northeast Brazil) and, Yucatan (Mexico) (38–40). We were unable to detect CHIKV in pools from mosquito collections performed in 2016 and, CHIKV positive samples from 2015 and 2017 were all from engorged pools and, did not result in any CPE in C6/36 cells. We did not detect the circulation of CHIKV in Cx. quinquefasciatus from the MRR, although two independent studies reported the virus circulation in this species during outbreaks of CHIKV in the Amazon region (Brazil) and Bangkok (Thailand) (41, 42). Regarding ZIKV data, we found higher ZIKV MIR values in Ae. aegypti than those reported in studies conducted during the same period in Rio de Janeiro (Brazil) (18, 43), as well as in Chiapas and Jalisco states (Mexico) (44, 45). However, these studies were performed with random collections, where MIR values are expected to be lower. Similar to our results, ZIKV was also detected in Ae. taeniorhynchus collected from the city of Vitória (southeast Brazil) during 2016 (19), but the role of this species in transmitting ZIKV still needs elucidation (46). We identified 96 ZIKV-positive pools of Cx. quinquefasciatus from the MRR, but even years after the 2015 ZIKV epidemics, the role of this species in transmitting this particular virus is still pondered (47–50). Yet, natural ZIKV infection has also been reported in salivary glands from wild-caught Cx. coronator and Cx. tarsalis from Guadalajara (Mexico) (44), in whole-body field-collected Cx. quinquefasciatus females from Recife, Vitória (Brazil) and Medellin (Colombia) (19, 20, 51).
Parameters such as vector competence, mosquito populational density and blood feeding status added to arboviral detection in field mosquitoes are important aspects taken into consideration when studying the transmission cycle in field mosquitoes (52, 53). Once an outbreak occurs in an urban area, the species with the highest density in the region should be included in the investigation, in order to assess the epidemiological situation of each location (52). In the present study, we detected DENV in Cx. quinquefasciatus samples. Among 549 Cx. quinquefasciatus pools from the MRR screened, DENV was detected in six samples. Studies conducted in Cuiaba (Western Brazil) and São Paulo (southern Brazil) have also reported DENV circulation in field-caught Culex. spp (54, 55). Although Culex mosquitoes are not considered competent in transmitting DENV (56), the data obtained from this species may provide valuable information over the transmission of pathogens in areas where Cx. quinquefasciatus is found in high densities. Therefore, it can be considered an epidemiological indicator of which arbovirus are circulating in humans during outbreaks and epidemics. We reinforce here the importance of implement arbovirus surveillance in Culex species, which is not commonly performed (57). Characteristics such as high population density and high anthropophilic behavior of Cx. quinquefasciatus in Brazil can increase the chance of collecting specimens that contain blood in your organism. In this way, increase the probability of identifying circulating arboviruses in the vector population (13, 58, 59).
RT-PCR and RT-qPCR are ideal techniques for massive screening of mosquitoes during virus surveillance, reducing diagnosis time in relation to viral isolation and decrease the possibility of cross-reaction when compared to serological methods (60, 61). RT-qPCR is considered the gold standard method for arboviruses detection in humans and mosquito samples and produces high quality results (31, 60) and new nucleic acid tests can be used to detect arboviruses, such as Loop-mediated isothermal amplification (LAMP), a point-of-care assay, which has already been used to detect DENV, ZIKV and CHIKV in patient and mosquito samples (62–64). In our study, from a total of 32 RT-PCR/RT-qPCR positive samples inoculated in C6/36 cells, CPE was observed in three samples containing pools of Ae. aegypti mosquitoes with DENV2 and in one sample comprised of Ae. aegypti positive mosquitoes for ZIKV. The difficulty in isolating arboviruses in C6/36 cells observed here could be related to samples that are improperly transported to the lab, thawed more than once or have a low viral titer. In addition, these factors also directly influence the detection of a low number RNA copies number in RT-qPCR (38, 39).
In conclusion, since strategies implemented by control programs in the MRR have historically struggled in preventing arbovirus transmission, the implementation of a specific mosquito collection protocol for arbovirus surveillance in epidemic and inter-epidemic periods serves as an early-warning system to implement control actions before the surge of human cases. These studies must be conducted in accordance with the needs and peculiarities of each location, as well as having focus on precarious areas. The implementation of such surveillance in mosquitoes from the Metropolitan Region of Recife resulted in the identification of DENV, ZIKV and CHIKV circulating in Ae. aegypti, Ae. taeniorhynchus and Cx. quinquefasciatus collected from 2015-17.
The original contributions presented in the study are included in the article/Supplementary Material. Further inquiries can be directed to the corresponding author.
The study was performed based on caught-field mosquitoes in houses and was approved by the Research Ethics Committees of the Aggeu Magalhães Institute (FIOCRUZ-PE) under the registration number CAAE 51012015.9.0000.5190 and PlatBr 1.547.598.
LK, MP, and DG wrote the manuscript. CA, MP, DG, and LK conceived and planned the study. LK and DG performed laboratory experiments. LK, DG, MP, and AO performed data analysis. RB, DA, and CP planned and super-vised the mosquito collections. CA, MP, and DG supervised and administered the study. CA, MP, and RB financed the project. All authors contributed to the article and approved the submitted version.
This work was partially supported by the Fundação de Amparo à Pesquisa do Estado de Pernambuco (FACEPE): APQ-1608-2.13/15 and APQ-0085-2.13/16 to CA, APQ-0725-2.13/17 to MP and APQ- 1630-2.01/15 to RB. CA is supported by a productivity fellowship from the Brazilian National Council for Research and Development (CNPq).
The authors declare that the research was conducted in the absence of any commercial or financial relationships that could be construed as a potential conflict of interest.
All claims expressed in this article are solely those of the authors and do not necessarily represent those of their affiliated organizations, or those of the publisher, the editors and the reviewers. Any product that may be evaluated in this article, or claim that may be made by its manufacturer, is not guaranteed or endorsed by the publisher.
All the experiments were performed using viral isolates kindly provided by Dr. Marli Tenório (LAVITE, IAM/FIOCRUZ). We would like to thank Dr. Danielle Maria Nascimento Moura (Immunology Department, IAM/FIOCRUZ) for IFA image capturing and, the staff of the Pernambuco State Health Department and Entomology Department for collecting mosquitoes in all different locations.
The Supplementary Material for this article can be found online at: https://www.frontiersin.org/articles/10.3389/fitd.2022.875031/full#supplementary-material
1. Bhatt S, Gething PW, Brady OJ, Messina JP, Farlow AW, Moyes CL, et al. The Global Distribution and Burden of Dengue. Nature (2013) 496(7446):504–7. doi: 10.1038/nature12060
2. Wu P, Yu X, Wang P, Cheng G. Arbovirus Lifecycle in Mosquito: Acquisition, Propagation and Transmission. Expert Rev Mol Med (2019) 21:e1. doi: 10.1017/erm.2018.6
3. Mazarin N, Rosenthal J-M, Devenge J. [Mother-Infant Dengue Transmission During the 2009-2010 Dengue Epidemic: Report of Four Cases]. Arch Pediatr Organe (2014) 21(7):745–9. doi: 10.1016/j.arcped.2014.04.009
4. WHO. Dengue and Severe Dengue (2020). Available at: https://www.who.int/news-room/fact-sheets/detail/dengue-and-severe-dengue.
5. Nunes PCG, Daumas RP, Sánchez-Arcila JC, Nogueira RMR, Horta MAP, Dos Santos FB. 30 Years of Fatal Dengue Cases in Brazil: A Review. BMC Public Health (2019) 19(1):329. doi: 10.1186/s12889-019-6641-4
6. Brathwaite Dick O, San Martín JL, Montoya RH, del Diego J, Zambrano B, Dayan GH. The History of Dengue Outbreaks in the Americas. Am J Trop Med Hyg (2012) 87(4):584–93. doi: 10.4269/ajtmh.2012.11-0770
7. Donalisio MR, Freitas ARR, Zuben APBV. Arboviroses Emergentes No Brasil: Desafios Para a Clínica E Implicações Para a Saúde Pública. Rev Saúde Pública (2017) 51:1–6. doi: 10.1590/S1518-8787.2017051006889
8. Ministério da Saúde. Monitoramento Dos Casos De Arboviroses Urbanas Transmitidas Pelo Aedes Aegypti (Dengue, Chikungunya E Zika), Semanas Epidemiológicas 1 a 50, 2020. Bol Epidemiol (2020) 51:1–11.
9. de Albuquerque M deFPM, de Souza WV, Araújo TVB, Braga MC, Miranda Filho D deB, Ximenes RA deA, et al. The Microcephaly Epidemic and Zika Virus: Building Knowledge in Epidemiology. Cad Saude Publica (2018) 34(10):e00069018. doi: 10.1590/0102-311X00069018
10. Brito C. Zika Virus: A New Chapter in the History of Medicine. Acta Med Port (2015) 28(6):679–80. doi: 10.20344/amp.7341
11. de Souza WV, de Albuquerque M deFPM, Vazquez E, Bezerra LCA, Mendes A daCG, Lyra TM, et al. Microcephaly Epidemic Related to the Zika Virus and Living Conditions in Recife, Northeast Brazil. BMC Public Health (2018) 18(1):130. doi: 10.1186/s12889-018-5039-z
12. Gu W, Unnasch TR, Katholi CR, Lampman R, Novak RJ. Fundamental Issues in Mosquito Surveillance for Arboviral Transmission. Trans R Soc Trop Med Hyg (2008) 102(8):817–22. doi: 10.1016/j.trstmh.2008.03.019
13. Ndiaye EH, Diallo D, Fall G, Ba Y, Faye O, Dia I, et al. Arboviruses Isolated From the Barkedji Mosquito-Based Surveillance System, 2012-2013. BMC Infect Dis (2018) 18(1):642. doi: 10.1186/s12879-018-3538-2
14. Ramírez AL, van den Hurk AF, Meyer DB, Ritchie SA. Searching for the Proverbial Needle in a Haystack: Advances in Mosquito-Borne Arbovirus Surveillance. Parasit Vectors (2018) 11(1):320. doi: 10.1186/s13071-018-2901-x
15. Faria NR, Sabino EC, Nunes MRT, Alcantara LCJ, Loman NJ, Pybus OG. Mobile Real-Time Surveillance of Zika Virus in Brazil. Genome Med (2016) 8(1):97. doi: 10.1186/s13073-016-0356-2
16. Grubaugh ND, Ladner JT, Lemey P, Pybus OG, Rambaut A, Holmes EC, et al. Tracking Virus Outbreaks in the Twenty-First Century. Nat Microbiol (2019) 4(1):10–9. doi: 10.1038/s41564-018-0296-2
17. van den Hurk AF, Hall-Mendelin S, Johansen CA, Warrilow D, Ritchie SA. Evolution of Mosquito-Based Arbovirus Surveillance Systems in Australia. J BioMed Biotechnol (2012) 2012:e325659. doi: 10.1155/2012/325659
18. Ferreira-de-Brito A, Ribeiro IP, de Miranda RM, Fernandes RS, Campos SS, da Silva KAB, et al. First Detection of Natural Infection of Aedes Aegypti With Zika Virus in Brazil and Throughout South America. Mem Inst Oswaldo Cruz (2016) 111(10):655–8. doi: 10.1590/0074-02760160332
19. Ayres CFJ, Guedes DRD, Paiva MHS, Morais-Sobral MC, Krokovsky L, Machado LC, et al. Zika Virus Detection, Isolation and Genome Sequencing Through Culicidae Sampling During the Epidemic in Vitória, Espírito Santo, Brazil. Parasit Vectors (2019) 12(1):220. doi: 10.1186/s13071-019-3461-4
20. Guedes DR, Paiva MH, Donato MM, Barbosa PP, Krokovsky L, Rocha SWDS, et al. Zika Virus Replication in the Mosquito Culex Quinquefasciatus in Brazil. Emerg Microbes Infect (2017) 6(8):e69. doi: 10.1038/emi.2017.59
21. Whiteman A, Loaiza JR, Yee DA, Poh KC, Watkins AS, Lucas KJ, et al. Do Socioeconomic Factors Drive Aedes Mosquito Vectors and Their Arboviral Diseases? A Systematic Review of Dengue, Chikungunya, Yellow Fever, and Zika Virus. One Health Amst Neth (2020) 11:100188. doi: 10.1016/j.onehlt.2020.100188
22. Almeida LS, Cota ALS, Rodrigues DF. Sanitation, Arboviruses, and Environmental Determinants of Disease: Impacts on Urban Health. Ciênc Saúde Coletiva (2020) 25:3857–68. doi: 10.1590/1413-812320202510.30712018
23. Lopes RP, Lima JBP, Martins AJ. Insecticide Resistance in Culex Quinquefasciatus Say, 1823 in Brazil: A Review. Parasit Vectors (2019) 12:591. doi: 10.1186/s13071-019-3850-8
24. Rahman RU, Cosme LV, Costa MM, Carrara L, Lima JBP, Martins AJ. Insecticide Resistance and Genetic Structure of Aedes Aegypti Populations From Rio De Janeiro State, Brazil. PLoS Negl Trop Dis (2021) 15(2):e0008492. doi: 10.1371/journal.pntd.0008492
25. IBGE. Instituto Brasileiro De Geografia E Estatística, Censo 2010. (2010). Rio de Janeiro: IBGE-Instituto Brasileiro de Geografia e Estatística
26. Kong YY, Thay CH, Tin TC, Devi S. Rapid Detection, Serotyping and Quantitation of Dengue Viruses by TaqMan Real-Time One-Step RT-PCR. J Virol Methods (2006) 138(1–2):123–30. doi: 10.1016/j.jviromet.2006.08.003
27. Forattini OP. Principais Mosquitos De Importância Sanitária No Brasil. Cad Saúde Pública. (1995) 11(1):157–8. doi: 10.1590/S0102-311X1995000100027
28. Barbosa PP, Guedes DRD, Melo-Santos M, Cordeiro MT, Acioli RV, Batista C, et al. Vector Surveillance for Dengue Virus Detection in the Archipelago of Fernando De Noronha, Brazil. J Med Entomol (2016) 53(3):613–9. doi: 10.1093/jme/tjw034
29. Lanciotti RS, Calisher CH, Gubler DJ, Chang GJ, Vorndam AV. Rapid Detection and Typing of Dengue Viruses From Clinical Samples by Using Reverse Transcriptase-Polymerase Chain Reaction. J Clin Microbiol (1992) 30(3):545–51. doi: 10.1128/jcm.30.3.545-551.1992
30. Lanciotti RS, Kosoy OL, Laven JJ, Panella AJ, Velez JO, Lambert AJ, et al. Chikungunya Virus in US Travelers Returning From India, 2006. Emerg Infect Dis (2007) 13(5):764–7. doi: 10.3201/eid1305.070015
31. Lanciotti RS, Kosoy OL, Laven JJ, Velez JO, Lambert AJ, Johnson AJ, et al. Genetic and Serologic Properties of Zika Virus Associated With an Epidemic, Yap State, Micronesia, 2007. Emerg Infect Dis (2008) 14(8):1232–9. doi: 10.3201/eid1408.080287
32. Chow VT, Chan YC, Yong R, Lee KM, Lim LK, Chung YK, et al. Monitoring of Dengue Viruses in Field-Caught Aedes Aegypti and Aedes Albopictus Mosquitoes by a Type-Specific Polymerase Chain Reaction and Cycle Sequencing. Am J Trop Med Hyg (1998) 58(5):578–86. doi: 10.4269/ajtmh.1998.58.578
33. Kocher TD, Thomas WK, Meyer A, Edwards SV, Pääbo S, Villablanca FX, et al. Dynamics of Mitochondrial DNA Evolution in Animals: Amplification and Sequencing With Conserved Primers. Proc Natl Acad Sci U S A (1989) 86(16):6196–200. doi: 10.1073/pnas.86.16.6196
34. Ayres CFJ, Melo-santos MAV, Solé-Cava AM, Furtado AF. Genetic Differentiation of Aedes Aegypti (Diptera: Culicidae), the Major Dengue Vector in Brazil. J Med Entomol (2003) 40(4):430–5. doi: 10.1603/0022-2585-40.4.430
35. Secretaria Estatual de Saúde/PE. Casos Confirmados De Dengue No Estado De Pernambuco. Recife, Brasil:SES-PE-Secretaria de Saúde de Pernambuco (2021).
36. Secretaria Estatual de Saúde/PE. Vigilância Em Saúde, Boletim Arboviroses. Recife: SES-PE-Secretaria de Saúde de Pernambuco (2019).
37. Cevallos V, Ponce P, Waggoner JJ, Pinsky BA, Coloma J, Quiroga C, et al. Zika and Chikungunya Virus Detection in Naturally Infected Aedes Aegypti in Ecuador. Acta Trop (2018) 177:74–80. doi: 10.1016/j.actatropica.2017.09.029
38. Aragão CF, Cruz ACR, Nunes Neto JP, Monteiro HA de O, da Silva EVP, da Silva SP, et al. Circulation of Chikungunya Virus in Aedes Aegypti in Maranhão, Northeast Brazil. Acta Trop (2018) 186:1–4. doi: 10.1016/j.actatropica.2018.06.022
39. Cigarroa-Toledo N, Blitvich BJ, Cetina-Trejo RC, Talavera-Aguilar LG, Baak-Baak CM, Torres-Chablé OM, et al. Chikungunya Virus in Febrile Humans and Aedes Aegypti Mosquitoes, Yucatan, Mexico. Emerg Infect Dis (2016) 22(10):1804–7. doi: 10.3201/eid2210.152087
40. Costa-da-Silva AL, Ioshino RS, de Araújo HRC, Kojin BB, Zanotto PM de A, Oliveira DBL, et al. Laboratory Strains of Aedes Aegypti are Competent to Brazilian Zika Virus. PLoS One (2017) 12(2):e0171951. doi: 10.1371/journal.pone.0171951
41. Phumee A, Intayot P, Sor-suwan S, Jittmittraphap A, Siriyasatien P. Molecular Detection of Indian Ocean Lineage Chikungunya Virus RNA in Field Collected Culex Quinquefasciatus Say From Bangkok, Thailand But No Evidence of Virus Replication. PLoS One (2021) 16(1):e0246026. doi: 10.1371/journal.pone.0246026
42. Ribeiro Cruz AC, Pinto Nunes Neto J, Patroca da Silva S, Vieira Pinto da Silva E, Juscely Galvão Pereira G, Maia Santos M, et al. Chikungunya Virus Detection in Aedes Aegypti and Culex Quinquefasciatus During an Outbreak in the Amazon Region. Viruses (2020) 12(8):853–62. doi: 10.3390/v12080853
43. Ayllón T, Campos R de M, Brasil P, Morone FC, Câmara DCP, Meira GLS, et al. Early Evidence for Zika Virus Circulation Among Aedes Aegypti Mosquitoes, Rio De Janeiro, Brazil. Emerg Infect Dis (2017) 23(8):1411–2. doi: 10.3201/eid2308.162007
44. Elizondo-Quiroga D, Medina-Sánchez A, Sánchez-González JM, Eckert KA, Villalobos-Sánchez E, Navarro-Zúñiga AR, et al. Zika Virus in Salivary Glands of Five Different Species of Wild-Caught Mosquitoes From Mexico. Sci Rep (2018) 8(1):809. doi: 10.1038/s41598-017-18682-3
45. Guerbois M, Fernandez-Salas I, Azar SR, Danis-Lozano R, Alpuche-Aranda CM, Leal G, et al. Outbreak of Zika Virus Infection, Chiapas State, Mexico, 2015, and First Confirmed Transmission by Aedes Aegypti Mosquitoes in the Americas. J Infect Dis (2016) 214(9):1349–56. doi: 10.1093/infdis/jiw302
46. Hart CE, Roundy CM, Azar SR, Huang JH, Yun R, Reynolds E, et al. Zika Virus Vector Competency of Mosquitoes, Gulf Coast, United States. Emerg Infect Dis (2017) 23(3):559–60. doi: 10.3201/eid2303.161636
47. Fernandes RS, Campos SS, Ferreira-de-Brito A, de Miranda RM, Barbosa da Silva KA, Castro MG de, et al. Culex Quinquefasciatus From Rio De Janeiro Is Not Competent to Transmit the Local Zika Virus. PLoS Negl Trop Dis (2016) 10(9):e0004993. doi: 10.1371/journal.pntd.0004993
48. Gomard Y, Lebon C, Mavingui P, Atyame CM. Contrasted Transmission Efficiency of Zika Virus Strains by Mosquito Species Aedes Aegypti, Aedes Albopictus and Culex Quinquefasciatus From Reunion Island. Parasit Vectors (2020) 13(1):398. doi: 10.1186/s13071-020-04267-z
49. Gutiérrez-Bugallo G, Piedra LA, Rodriguez M, Bisset JA, Lourenço-de-Oliveira R, Weaver SC, et al. Vector-Borne Transmission and Evolution of Zika Virus. Nat Ecol Evol (2019) 3(4):561–9. doi: 10.1038/s41559-019-0836-z
50. MacLeod HJ, Dimopoulos G. Detailed Analyses of Zika Virus Tropism in Culex Quinquefasciatus Reveal Systemic Refractoriness. mBio (2020) 11(4):1–19. doi: 10.1128/mBio.01765-20
51. Pérez-Pérez J, Rojo-Ospina R, Henao-Correa E, García-Huertas P, Triana-Chavez O, Rúa-Uribe GL. Natural Infection of Aedes Aegypti, Ae. Albopictus and Culex Spp. With Zika Virus in Medellin, Colombia. CES Med (2019) 33(3):175–81. doi: 10.21615/cesmedicina.33.3.2
52. Ayres CFJ. Identification of Zika Virus Vectors and Implications for Control. Lancet Infect Dis (2016) 16(3):278–9. doi: 10.1016/S1473-3099(16)00073-6
53. Shaw WR, Catteruccia F. Vector Biology Meets Disease Control: Using Basic Research to Fight Vector-Borne Diseases. Nat Microbiol (2019) 4(1):20–34. doi: 10.1038/s41564-018-0214-7
54. Barrio-Nuevo KM, Cunha MS, Luchs A, Fernandes A, Rocco IM, Mucci LF, et al. Detection of Zika and Dengue Viruses in Wild-Caught Mosquitoes Collected During Field Surveillance in an Environmental Protection Area in São Paulo, Brazil. PLoS One (2020) 15(10):1–13. doi: 10.1371/journal.pone.0227239
55. Serra OP, Cardoso BF, Ribeiro ALM, dos Santos FAL, Slhessarenko RD. Mayaro Virus and Dengue Virus 1 and 4 Natural Infection in Culicids From Cuiabá, State of Mato Grosso, Brazil. Mem Inst Oswaldo Cruz (2016) 111(1):20–9. doi: 10.1590/0074-02760150270
56. Vazeille-Falcoz M, Rosen L, Mousson L, Rodhain F. Replication of Dengue Type 2 Virus in Culex Quinquefasciatus (Diptera: Culicidae). Am J Trop Med Hyg (1999) 60(2):319–21. doi: 10.4269/ajtmh.1999.60.319
57. Dos Reis IC, Gibson G, Ayllón T, de Medeiros Tavares A, de Araújo JMG, da Silva Monteiro E, et al. Entomo-Virological Surveillance Strategy for Dengue, Zika and Chikungunya Arboviruses in Field-Caught Aedes Mosquitoes in an Endemic Urban Area of the Northeast of Brazil. Acta Trop (2019) 197:105061. doi: 10.1016/j.actatropica.2019.105061
58. Brinkmann A, Nitsche A, Kohl C. Viral Metagenomics on Blood-Feeding Arthropods as a Tool for Human Disease Surveillance. Int J Mol Sci (2016) 17(10):1743–57. doi: 10.3390/ijms17101743
59. Shi C, Liu Y, Hu X, Xiong J, Zhang B, Yuan Z. A Metagenomic Survey of Viral Abundance and Diversity in Mosquitoes From Hubei Province. PLoS One (2015) 10(6):e0129845. doi: 10.1371/journal.pone.0129845
60. Waggoner JJ, Pinsky BA. Zika Virus: Diagnostics for an Emerging Pandemic Threat. J Clin Microbiol (2016) 54(4):860–7. doi: 10.1128/JCM.00279-16
61. Felix AC, Souza NCS, Figueiredo WM, Costa AA, Inenami M, da Silva RMG, et al. Cross Reactivity of Commercial Anti-Dengue Immunoassays in Patients With Acute Zika Virus Infection. J Med Virol (2017) 89(8):1477–9. doi: 10.1002/jmv.24789
62. Lopez-Jimena B, Bekaert M, Bakheit M, Frischmann S, Patel P, Simon-Loriere E, et al. Development and Validation of Four One-Step Real-Time RT-LAMP Assays for Specific Detection of Each Dengue Virus Serotype. PLoS Negl Trop Dis (2018) 12(5):e0006381. doi: 10.1371/journal.pntd.0006381
63. Lopez-Jimena B, Wehner S, Harold G, Bakheit M, Frischmann S, Bekaert M, et al. Development of a Single-Tube One-Step RT-LAMP Assay to Detect the Chikungunya Virus Genome. PLoS Negl Trop Dis (2018) 12(5):e0006448. doi: 10.1371/journal.pntd.0006448
64. da Silva SJR, Paiva MHS, Guedes DRD, Krokovsky L, de Melo FL, da Silva MAL, et al. Development and Validation of Reverse Transcription Loop-Mediated Isothermal Amplification (RT-LAMP) for Rapid Detection of ZIKV in Mosquito Samples From Brazil. Sci Rep (2019) 9(1):4494. doi: 10.1038/s41598-019-40960-5
Keywords: vector surveillance, arbovirus, RT-PCR, RT-qPCR, Aedes, Culex
Citation: Krokovsky L, Paiva MHS, Guedes DRD, Barbosa RMR, de Oliveira ALS, Anastácio DB, Pontes CR and Ayres CFJ (2022) Arbovirus Surveillance in Field-Collected Mosquitoes From Pernambuco-Brazil, During the Triple Dengue, Zika and Chikungunya Outbreak of 2015-2017. Front. Trop. Dis 3:875031. doi: 10.3389/fitd.2022.875031
Received: 13 February 2022; Accepted: 14 March 2022;
Published: 06 April 2022.
Edited by:
Indra Vythilingam, University of Malaya, MalaysiaReviewed by:
Anayansi Valderrama Cumbrera, Gorgas Memorial Institute of Health Studies, PanamaCopyright © 2022 Krokovsky, Paiva, Guedes, Barbosa, de Oliveira, Anastácio, Pontes and Ayres. This is an open-access article distributed under the terms of the Creative Commons Attribution License (CC BY). The use, distribution or reproduction in other forums is permitted, provided the original author(s) and the copyright owner(s) are credited and that the original publication in this journal is cited, in accordance with accepted academic practice. No use, distribution or reproduction is permitted which does not comply with these terms.
*Correspondence: Constância F. J. Ayres, Y29zbnRhbmNpYS5heXJlc0BmaW9jcnV6LmJy
†These authors share first authorship
Disclaimer: All claims expressed in this article are solely those of the authors and do not necessarily represent those of their affiliated organizations, or those of the publisher, the editors and the reviewers. Any product that may be evaluated in this article or claim that may be made by its manufacturer is not guaranteed or endorsed by the publisher.
Research integrity at Frontiers
Learn more about the work of our research integrity team to safeguard the quality of each article we publish.