- 1Department of Immunization, Vaccines and Biologicals, World Health Organization, Geneva, Switzerland
- 2PATH Essential Medicines, PATH, Seattle, WA, United States
- 3Drugs for Neglected Diseases initiative (DNDi), New Delhi, India
- 4Hilleman Laboratories, Singapore, Singapore
- 5Science and Innovation Unit, Assistant Regional Director Cluster, World Health Organization Africa Region, Brazzaville, Democratic Republic of Congo
- 6The Special Programme for Research and Training in Tropical Diseases and Department of Research for Health, The World Health Organization, Geneva, Switzerland
- 7Division of Gastrointestinal Sciences, Christian Medical College, Vellore, India
- 8Center for Immunization Research, Johns Hopkins Bloomberg School of Public Health, Baltimore, MD, United States
- 9Faculty of Medicine, National Autonomous University of Mexico, Mexico City, Mexico
- 10Instituto de Investigación Nutricional, Lima, Peru
- 11Department of Control of Neglected Tropical Diseases, World Health Organization, Geneva, Switzerland
The mRNA vaccine technology platform may enable rapid response to some emerging infectious diseases (EIDs), as demonstrated through the COVID-19 pandemic. Beyond the role it could play in future EID response, mRNA technology also could have an important role in accelerating the development of, and access to, vaccines for some neglected tropical diseases (NTDs), which occur mainly in impoverished regions of the world. Despite their significant disease burden, few vaccines against NTDs have been developed, in part because of the uncertain market and return on investment. In addition, the probability of technical and regulatory success is considered to be low for developing vaccines against multicellular parasites, or organisms that have sophisticated mechanisms for evading immunological surveillance, such as many of the NTD pathogens. The global 2021-2030 road map for neglected tropical diseases sets ambitious targets for the eradication, elimination, and control of NTDs. For some, effective interventions exist but are underutilized. For others, vaccines need to be developed or their use expanded to meet global targets on control and elimination. This article discusses the application of the mRNA technology platform to the development of vaccines for NTDs as well as EIDs, highlights the challenges in bringing these products to the market, and indicates potential areas which could be explored, including leveraging investment for vaccines with a more profitable market potential and enabling local manufacturing in regions where NTDs are endemic. Such regional production could include collaborations with the mRNA vaccine technology transfer hubs that are being established with the support of WHO and COVAX partners.
The Immunization Agenda 2030 - Research and Innovation
In 2020, the World Health Assembly of WHO endorsed the Immunization Agenda 2030 (IA2030): A Global Strategy to Leave No One Behind which includes a strategic priority on Research and Innovation (1). The overarching goal of this strategic priority is that prioritized innovations, as identified by national, regional, and global immunization stakeholders, are rapidly made available to all countries and communities, to decentralize their access and facilitate development and use at the national and regional levels.
Introduction to NTDs and Vaccine Development
Neglected tropical diseases (NTDs) include 20 diseases and disease groups, with a combined health, social, and economic burden on more than 1 billion people living predominantly in resource-poor communities in tropical and sub-tropical areas (2). The global road map for NTDs, 2021-2030, sets new targets related to the eradication, elimination and control for NTDs (2). For many of these diseases, effective interventions exist such as pharmaceuticals as well as surgical interventions, water, sanitation and hygiene, and vector and other environmental control; however, these interventions are not always widely available or easily accessible. In the case of therapeutic interventions, robust surveillance and case detection are needed, which is often lacking in low-income contexts.
For some NTDs, the development of vaccines or expansion in vaccine use is needed to meet global targets. As part of these targets, the road map identifies actions for the development or increased use of the following human vaccines: chikungunya; dengue; cutaneous leishmaniasis; visceral leishmaniasis; leprosy; rabies; and schistosomiasis (2). In addition to these diseases, vaccine development is also underway for hookworm disease and trachoma (Supplementary Table 1).
The development of vaccines against NTDs is challenging for several reasons. First, due to a limited or uncertain market, which is predominantly in low- and middle-income countries (LMICs), there is limited or unquantified economic incentive for developers to invest in the costly and lengthy development of these products. A limited market also means that, at small production scales, the cost of goods will be high when using traditional vaccine manufacturing. Second, the biological and scientific feasibility of developing a vaccine might be low, given that many NTD pathogens are bacterial or parasitic with complicated structures, or lifecycles, rendering it difficult to identify the protective antigen(s) to be included in the vaccine. Third, the regulatory pathway to market authorization may be challenging and/or uncertain, preclinical animal models may be lacking, and the ability to demonstrate statistically significant efficacy through appropriately sized pivotal clinical trials may be difficult, especially when the incidence of the primary clinical endpoint is low or unpredictable, and when correlates of protection have not been established. Fourth, other interventions may be available to prevent or treat these diseases that may have greater impact if they were optimally used or more broadly and equitably implemented. Fifth, some NTDs are not global in endemicity and the settings in which vaccines would be used are uncertain or limited, with use likely in specific epidemiologic settings and at-risk groups. These target groups and the strategy to deliver vaccines should they exist, are poorly defined, and as such the pathway to policy uncertain. Finally, for many NTDs, mortality is low which means that international policy makers and funders cannot rely on this traditional metric to prioritize vaccine development. However, some of these pathogens do kill and others cause significant morbidity, disability, mental health problems, reduce quality of life, and decrease socio-economic status of the populations they affect. These are critical parameters to quantify in order to articulate the potential public health value that vaccines can offer (3).
Introduction to Priority EIDs and Vaccine Development
Emerging infectious diseases (EIDs) are serious public health, social, and economic threats. They may be either newly identified diseases or previously known diseases that are rapidly expanding in incidence or geographic scope (4). EIDs are a growing threat due to increased travel, increasing population density, deforestation, and climate change (5). Many EIDs are zoonotic, such as Zika, avian influenza, coronaviruses, Ebola and Marburg, and some have the potential to cause epidemics and pandemics. This potential increases significantly when a new pathogen emerges to which humans have no prior immunity and human-to-human transmission occurs readily. Pharmaceutical interventions must be developed rapidly to mitigate the spread and impact of the pandemic, an effort that becomes especially difficult when the pathogen was previously unknown, and no prior research has been conducted. Vaccines are a critical tool in the battle against emerging infectious diseases but typically take years to develop (6). Generally, it is not until an epidemic or pandemic arises that political pressure and incentive to develop vaccines are realised.
The unprecedented speed of COVID-19 vaccine development resulted, in part, from decades of research on coronaviruses, genomics, antigen selection and design, and vaccine platform technologies (7). Global collaboration between the public and private sectors as well as the ability to conduct large efficacy trials in the midst of a pandemic with widespread, high incidence SARS-CoV-2 infection also contributed to the speed of development (8).
An example of the typical pace of vaccine development for EIDs is Ebola. Despite its discovery as the etiologic virus in 1976, and numerous outbreaks thereafter (9), there was limited political pressure or financial incentive to develop Ebola vaccines as outbreaks could be contained quickly without significant geographic spread. While some candidate Ebola vaccines advanced in preclinical studies, it was not until the West African Ebola epidemic of 2014-2016, the worst recorded Ebola outbreak in history (10), that vaccine development accelerated, and candidates were evaluated in clinical trials (11). The declaration of a public health emergency of international concern in August 2014, and the threat that the virus posed on a global scale, brought the scientific community, governments, regulators and funders together to accelerate vaccine development (12). In 2019, the first Ebola vaccine was licensed followed by a second in 2020 (13). Vaccination has now become an important tool in controlling subsequent outbreaks of Ebola (14). The 2014-2016 Ebola outbreak led to the formation of two key initiatives, the WHO R&D Blueprint for action to prevent epidemics and the Coalition for Epidemic Preparedness Innovations (CEPI) (15, 16). Both initiatives have since published pathogen lists for which development of interventions should be prioritized (Table 1). While both aim to advance the development of vaccines for these priority pathogens, the WHO R&D Blueprint also aims to advance other interventions including medicines and diagnostics.
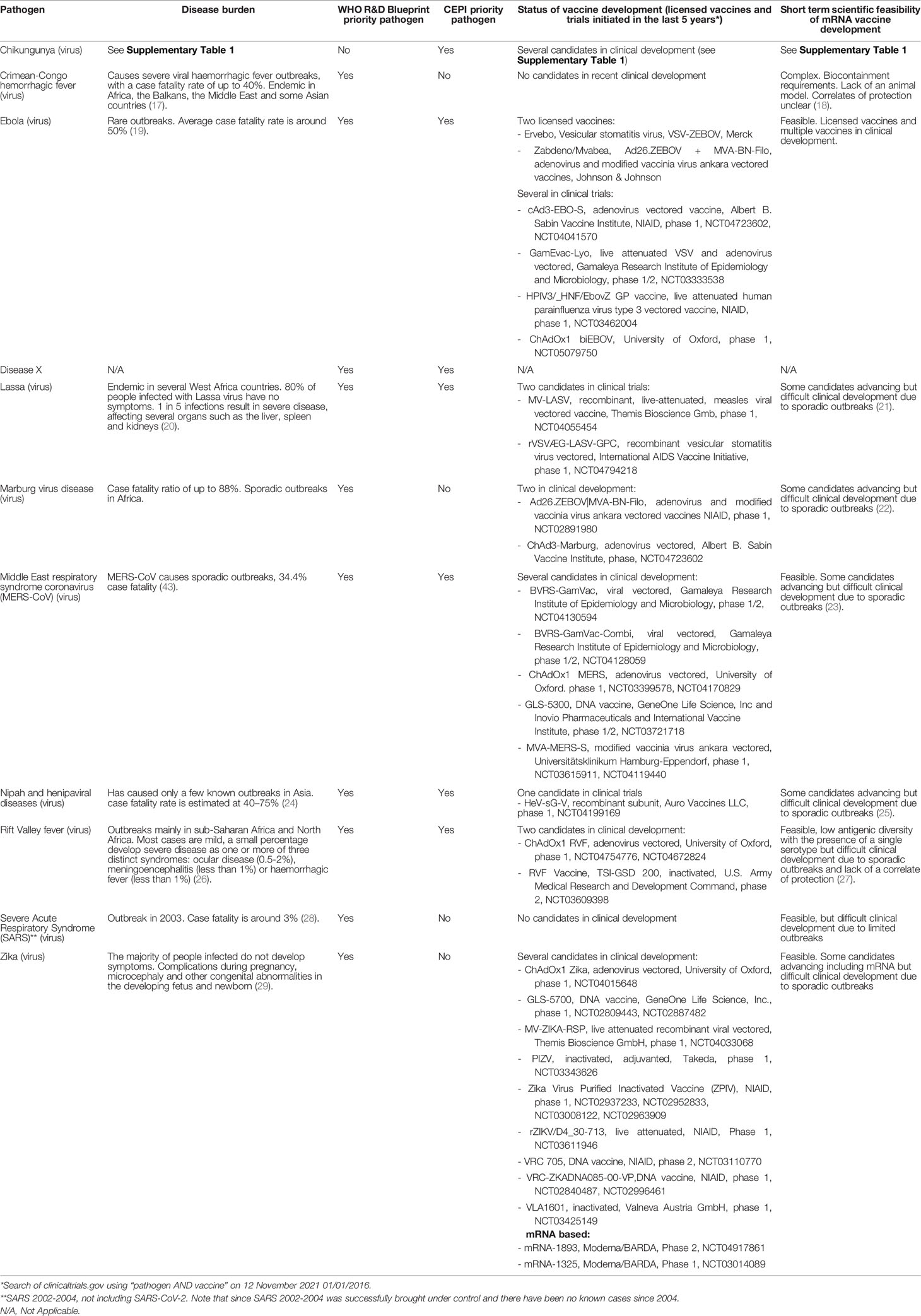
Table 1 List of Priority EIDs and vaccines under development, identified by WHO R&D Blueprint and/or CEPI.
Similar to NTDs, vaccine development for EIDs is challenging due to an uncertain market, lack of predictable and ongoing financial incentives, and a challenging regulatory pathway. However, one major difference is that priority EIDs (Table 1) are all viruses, while, apart from chikungunya, dengue, and rabies, NTDs are mostly parasitic or bacterial pathogens with more complex structures and/or lifecycles.
mRNA Technology Advantages and Potential Applications
To respond rapidly to the threats posed by a new EID with pandemic potential, vaccine production must not only be rapid, but also scalable, to quickly meet the needs of a region, or the global population. The recent advances in mRNA-based vaccines, exemplified by use against COVID-19, validated their viability as a platform technology for rapid, large-scale production of relatively well-tolerated, highly efficacious vaccines, in adults and children (8).
Because mRNA vaccines contain the genetic code, or sequence, for an antigen, they bypass the manufacturing production requirements of in-vitro expression and multi-step downstream processing, which can be costly and time consuming. Instead, antigen expression occurs in-vivo in the vaccinated individual. As such, mRNA-based vaccine production has several advantages over traditional cell-based, egg-based, or recombinant vaccine manufacturing. The production process is simpler with fewer steps so production yields are less variable, production is much faster, and facilities can be smaller (30). Furthermore, the costs to establish a facility, (i.e. capital costs), are potentially much lower than traditional vaccine manufacturing. A major advantage is that, being a platform technology, rapid changeover to produce mRNA encoding antigens from other pathogens in the event of an outbreak, epidemic, or pandemic is possible. Product-independent manufacturing also makes multi-production facilities feasible to operate because a single facility can be leveraged for rapid sequential small-scale production of vaccines against several pathogens. In addition, the rapid production of vaccine candidates using mRNA technology can accelerate candidate identification and optimization (especially if preclinical models are available) as well as initiation of early phase clinical studies.
mRNA vaccines induce both cellular and humoral immune responses and are capable of, in theory, encoding a large variety of proteins and even multiple proteins (31). Furthermore, unlike DNA vaccines, where optimal immunogenicity requires delivery using specific devices such as electroporation, microneedles or gene guns (32), mRNA vaccines can be delivered intramuscularly, subcutaneously, or intradermally via conventional needle and syringe (33). Presently mRNA vaccines are not given intranasally or intratracheally as, in their current form, they are susceptible to mucosal clearance and thus do not induce mucosal immunity (31).
The ability to rapidly manufacture, scale-up and respond to disease outbreaks with mRNA vaccines is critical for pandemic or any major epidemic response. However, to be commercially sustainable, an mRNA vaccine manufacturer will need to produce vaccines on an ongoing basis in its facility. For a country that is considering the application of the mRNA platform for EID or NTD vaccines, the expansion to also include manufacture of vaccines for non-pandemic, globally endemic diseases could off-set investment in the less lucrative vaccines that are important from a national and/or regional perspective. The development of mRNA vaccines is currently advancing with candidate mRNA vaccines in clinical development for respiratory syncytial virus, influenza, cytomegalovirus, human metapneumovirus and parainfluenza, rabies, chikungunya and Zika (34). Investment in an mRNA platform and facility for a portfolio of vaccines, particularly those intended for use in high-income countries, could off-set and support the development and production costs of the NTD or EID vaccines with limited commercial incentive.
General Challenges of the mRNA Vaccine Platform Technology
Despite the compelling advantages, the mRNA platform has limitations. One major limitation is the stability of mRNA, meaning that licensed mRNA COVID-19 vaccines currently require ultra-cold temperatures for long term storage (30, 35, 36). For vaccines intended for broad use in LMICs, mRNA vaccines will need to be able to be stored for long periods of time within the standard vaccine cold chain (2-8°C) or even outside of the cold chain for several days at temperatures of up to 40°C. Several promising next-generation technologies which may have improved thermostability are currently in development and have been reviewed elsewhere (35, 37).
Another potential limitation is that, unlike a whole killed or inactivated vaccine approach, protective target antigen/s must first be identified to produce the corresponding mRNA sequence.
Furthermore, significant evidence gaps exist as to the safety of these vaccines in infants and young children and duration of protection afforded by mRNA vaccines, which may differ by pathogen and formulation. For the moment, the safety database is small (albeit continuing to accumulate) for mRNA use in young children, which is the priority target group for many endemic, routine use vaccines. Continued demonstration of safety especially with regards to repeat doses of both the same antigen or diverse antigens, and in very young and infant populations is needed.
In the case of mRNA-based COVID-19 vaccines, waning immunity has been observed which may be an issue with the immune response to the spike protein of SARS-CoV-2 itself or the mRNA vaccine platform and needs further evaluation, including with alternative antigens (38–40). The effectiveness of mRNA in a heterologous prime boost strategy also needs further investigation, particularly when regimens require multiple doses, or boosters.
While the cost to establish facilities may be lower than traditional manufacturing, some of the raw materials and reagents used in mRNA vaccines production have been reported to be expensive and in short supply (30). Furthermore, downstream processing improvements are needed in order to improve the scalability and cost of production (30). Improvements in manufacturing processes, or other technologies, such as self-amplifying mRNA, may bring down production costs (34).
Finally, intellectual property and access to know-how are currently barriers for the development of mRNA vaccines for new producers.
mRNA Vaccines for NTDs
mRNA COVID-19 vaccines target a single viral protein while for some NTDs, it is likely that more than one antigen will be needed, which may increase the complexity of development, manufacture, and stability as well as production costs.
Currently the only NTD with candidate mRNA vaccines in development is rabies (Supplementary Table 1). Rabies is a viral pathogen, with first vaccines developed over a century ago (41). There are now 27 rabies vaccines on the global market (16). The development pathway for rabies vaccines is well-defined with established animal models and a correlate of protection (42). The development of vaccines against other NTDs, especially bacterial, fungal and parasitic pathogens is more uncertain and complicated, especially with regards to antigen design. For those NTDs with low or sporadic incidence, large-scale efficacy trials may not be feasible and vaccine development would need to be facilitated by innovative tools or clinical trial designs, when available, in lieu of full-scale clinical endpoint efficacy trials. These include the use of correlates of protection, animal challenge studies where the model recapitulates disease in humans, and Controlled Human Infection Models (CHIM). Alternative regulatory approval pathways such as the US FDA animal rule, accelerated approval, or emergency use listing may provide mechanisms to accelerate the availability of these vaccines. However, vaccine development is not needed for all NTDs and as stated above, many NTDs have other effective interventions available but their use needs to be scaled-up in order to increase their public health impact.
mRNA Vaccines for Priority EIDs
The potential of mRNA vaccine is most evident for EIDs where rapid response is often required. However, many of the challenges of NTD vaccine development mentioned above also apply to EIDs. The main difference is that all priority EID’s are viruses, with fewer antigen targets, which can make vaccine design simpler, however an antigen target capable of inducing protective immunity must first be known in order to produce an mRNA vaccine. mRNA vaccine development is ongoing for two priority EIDs, chikungunya and Zika.
Role of Local Production for mRNA-Based Priority EIDs and NTDs
Several initiatives to expand manufacturing capacity of mRNA-based vaccines to LMICs have launched, including the initiative of WHO, the Medicines Patent Pool (MPP), and COVAX partners (43, 44). The goal is to improve long-term health security in LMICs for vaccine production via two key objectives. The first objective is to expand the capabilities of existing LMICs manufacturers and the second to establish sustainable capacity in regions that previously had no significant capacity. As part of the WHO and MPP initiative, a global hub has been identified in South Africa and ‘spokes’ (technology transfer recipient manufacturers) are being identified in other regions through open calls for expression of interest. The hub is mandated to establish mRNA technology and to transfer that technology to LMIC manufacturers. Positive spin offs include strengthening the R&D and regulatory ecosystems in these regions. In addition, a call has also been announced for institutes interested to supply pharmaceutical grade reagents, raw materials and other inputs for mRNA vaccine production, and for manufacturers interested in receiving technology transfer (44, 45). To support this technology transfer as well as technology transfer for other vaccines and biologicals, WHO is also establishing a biomanufacturing workforce training center.
Regulatory agencies in producing countries will also receive support through these initiatives to strengthen their capacities. As part of this, WHO has published Regulatory considerations on the evaluation of the quality, safety and efficacy of messenger RNA vaccines for the prevention of infectious diseases (46).
Further capacity building in producing countries will be needed to strengthen preclinical and clinical development capacity, to enable both early- and late-stage studies and trials to be designed and implemented. The regional centers will also explore the development of new technologies to improve the thermostability of mRNA vaccines, increase efficacy for single-dose administration, and reduce production costs. Beyond COVID-19 vaccines, other vaccines will need to be developed to support the long-term sustainability of the local or regional mRNA vaccine production capacity. This model will enable the countries and regions to align on their R&D priorities, and determine which vaccines are to be developed/manufactured in these facilities, which may present an opportunity to develop vaccines for some NTDs and EIDs.
Conclusion
While mRNA vaccines can be produced faster than conventional vaccines, improvements in thermostability and cost and availability of reagents/raw materials are needed to ensure accessibility and affordability of these vaccines to LMICs. In addition, mRNA vaccines don’t necessarily solve issues related to antigen design and preclinical and clinical development challenges. Beyond COVID-19, further data are needed to confirm the duration of immunity for mRNA vaccines against other pathogens, and to confirm the safety and immunogenicity in very young children and infants. A significant advantage of the mRNA platform is the feasibility to rapidly produce multiple vaccines at small scale. However, while the mRNA platform offers a unique opportunity to ‘leapfrog’ the development of vaccines for NTDs and EIDs, it still remains critical to articulate the full public health value of these vaccines, and if favorable, to create effective incentives for the needed investments. Vaccine development for NTDs will likely need to leverage investments in vaccine manufacturing for other endemic diseases.
The establishment of mRNA vaccine production in the regions in which NTDs and EIDs occur most frequently may present an opportunity to accelerate vaccine development. Therefore, alignment of key stakeholders on the priority NTD and EID targets, including high-level regional political commitment, is imperative. Further assessment is needed of the potential pathogens for mRNA vaccine production based on local epidemiology at regional centers, biological and scientific feasibility of vaccine development, regulatory pathways to licensure, and policy and market considerations. Based on this assessment, regions that establish local mRNA vaccine manufacturing will need to prioritize pathogens for local vaccine production.
Author Contributions
All authors contributed technical content and reviewed the full draft. All comments consolidated. ES: Primary author, technical input. MH-A: clinical trial review, derivation of tables. DCK: technical input - platform, value. KS: technical input – NTDs. RR: technical input platform and NTDs. MC: technical input, production, LMICs. LEM: technical input, production, LMICs. JCR: technical input, NTDs. GK: technical input, EIDs, policy. RAK: technical input, platform, value. AC: technical input, policy. CFL: technical input, NTDs. MF: technical input, platform and manufacturing. BA-R: technical input, zoonotics, NTDs. AWS: technical input, zoonotics, NTDs. DD: technical input, zoonotics, NTDs. BG: secondary author, technical input all.
Author Disclaimer
The authors alone are responsible for the views expressed in this article and they do not necessarily represent the views, decisions or policies of the institutions with which they are affiliated.
Conflict of Interest
GK is on the board of CEPI.
The remaining authors declare that the research was conducted in the absence of any commercial or financial relationships that could be construed as a potential conflict of interest.
Publisher’s Note
All claims expressed in this article are solely those of the authors and do not necessarily represent those of their affiliated organizations, or those of the publisher, the editors and the reviewers. Any product that may be evaluated in this article, or claim that may be made by its manufacturer, is not guaranteed or endorsed by the publisher.
Supplementary Material
The Supplementary Material for this article can be found online at: https://www.frontiersin.org/articles/10.3389/fitd.2022.844039/full#supplementary-material
References
1. Immunization Agenda 2030: A Global Strategy to Leave No One Behind (IA2030). Available at: www.who.int/publications/m/item/immunization-agenda-2030-a-global-strategy-to-leave-no-one-behind (Accessed 30 August 2021).
2. Ending the Neglect to Attain the Sustainable Development Goals: A Road Map for Neglected Tropical Diseases 2021–2030 . Available at: https://apps.who.int/iris/handle/10665/338565 (Accessed 23 August 2021).
3. Bottazzi ME, Hotez PJ. “Running the Gauntlet”: Formidable Challenges in Advancing Neglected Tropical Diseases Vaccines From Development Through Licensure, and a “Call to Action”. Hum Vaccin Immunother (2019) 15(10):2235–42. doi: 10.1080/21645515.2019.1629254
4. McArthur DB. Emerging Infectious Diseases. Nurs Clin North Am (2019) 54(2):297–311. doi: 10.1016/j.cnur.2019.02.006
5. Bernasconi V, Kristiansen PA, Whelan M, Román RG, Bettis A, Yimer SA, et al. Developing Vaccines Against Epidemic-Prone Emerging Infectious Diseases. Bundesgesundheitsblatt Gesundheitsforschung Gesundheitsschutz (2020) 63(1):65–73. doi: 10.1007/s00103-019-03061-2
6. Excler JL, Saville M, Berkley S, Kim JH. Vaccine Development for Emerging Infectious Diseases. Nat Med (2021) 27(4):591–600. doi: 10.1038/s41591-021-01301-0
7. Rappuoli R, De Gregorio E, Del Giudice G, Phogat S, Pecetta S, Pizza M, et al. Vaccinology in the Post-COVID-19 Era. Proc Natl Acad Sci U S A. (2021) 118(3):1–7. doi: 10.1073/pnas.2020368118
8. Hogan MJ, Pardi N. Mrna Vaccines in the COVID-19 Pandemic and Beyond. Annu Rev Med (2021) 73:17–39. doi: 10.1146/annurev-med-042420-112725. Cited in: Pubmed
9. History of Ebola Virus Disease (EVD) Outbreaks . CDC website. Available at: www.cdc.gov/vhf/ebola/history/chronology.html (Accessed 30 August 2021).
10. Kamorudeen RT, Adedokun KA, Olarinmoye AO. Ebola Outbreak in West Africa, 2014 - 2016: Epidemic Timeline, Differential Diagnoses, Determining Factors, and Lessons for Future Response. J Infect Public Health (2020) 13(7):956–62. doi: 10.1016/j.jiph.2020.03.014
11. Higgs ES, Dubey SA, Coller BAG, Simon JK, Bollinger L, Sorenson RA, et al. Accelerating Vaccine Development During the 2013-2016 West African Ebola Virus Disease Outbreak. Curr Top Microbiol Immunol (2017) 2017:411:229–261. doi: 10.1007/82_2017_53
12. Venkatraman N, Silman D, Folegatti PM, Hill AVS. Vaccines Against Ebola Virus. Vaccine (2018) 36(36):5454–9. doi: 10.1016/j.vaccine.2017.07.054
13. Ebola Virus Disease: Vaccines. WHO website. Available at: www.who.int/news-room/q-a-detail/ebola-vaccines (Accessed 30 August 2021).
14. 19 June 2021- Ebola Outbreak in Guinea Declared Over. WHO website. Available at: https://www.afro.who.int/news/ebola-outbreak-guinea-declared-over (Accessed 30 August 2021).
15. Gouglas D, Christodoulou M, Plotkin SA, Hatchett R. CEPI: Driving Progress Toward Epidemic Preparedness and Response. Epidemiol Rev (2019) 41(1):28–33. doi: 10.1093/epirev/mxz012
16. Kieny MP. Lessons Learned From Ebola Vaccine R&D During a Public Health Emergency. Hum Vaccin Immunother (2018) 14(9):2114–5. doi: 10.1080/21645515.2018.1442161
17. WHO Health Topic: Crimean-Congo Haemorrhagic Fever. Available at: https://www.who.int/health-topics/crimean-congo-haemorrhagic-fever (Accessed 15 December 2021).
18. Mendoza EJ, Warner B, Safronetz D, Ranadheera C. Crimean-Congo Haemorrhagic Fever Virus: Past, Present and Future Insights for Animal Modelling and Medical Countermeasures. Zoonoses Public Health (2018) 65(5):465–80. doi: 10.1111/zph.12469
19. WHO Factsheets Ebola Virus Disease (2021). Available at: https://www.who.int/news-room/fact-sheets/detail/ebola-virus-disease (Accessed 16 December 2021). 23 February.
20. Who Health Topica, Lassa Fever . Available at: https://www.who.int/health-topics/lassa-fever (Accessed 16 December 2021).
21. CEPI Website: Largest Ever Lassa Fever Study Expands to More Countries in West Africa . Available at: https://cepi.net/news_cepi/largest-ever-lassa-fever-study-expands-to-more-countries-in-west-africa/ (Accessed 16 December 2021).
22. Wolfe DN, Sabourin CL, Merchlinsky MJ, Florence WC, Wolfraim LA, Taylor KL, et al. Selection of Filovirus Isolates for Vaccine Development Programs. Vaccines (Basel) (2021) 9(9). doi: 10.3390/vaccines9091045
23. Tai W, Zhang X, Yang Y, Zhu J, Du L. Advances in Mrna and Other Vaccines Against MERS-Cov. Transl Res (2021) 242:20–37. doi: 10.1016/j.trsl.2021.11.007
24. WHO Health Topics, Nipah Virus Infection . Available at: https://www.who.int/health-topics/nipah-virus-infection (Accessed 16 December 2021).
25. Amaya M, Broder CC. Vaccines to Emerging Viruses: Nipah and Hendra. Annu Rev Virol (2020) 7(1):447–73. doi: 10.1146/annurev-virology-021920-113833
26. WHO Health Topics, Rift Valley Fever . Available at: https://www.who.int/health-topics/rift-valley-fever (Accessed 16 December 2021).
27. Petrova V, Kristiansen P, Norheim G, Yimer SA. Rift Valley Fever: Diagnostic Challenges and Investment Needs for Vaccine Development. BMJ Glob Health (2020) 5(8):1–9. doi: 10.1136/bmjgh-2020-002694
28. Who Health Topics, Severe Acute Respiratory Syndrome (Sars) . Available at: https://www.who.int/health-topics/severe-acute-respiratory-syndrome (Accessed 16 December 2021).
29. WHO Health Topics, Zika Virus Disease . Available at: https://www.who.int/health-topics/zika-virus-disease (Accessed 16 December 2021).
30. Rosa SS, Prazeres DMF, Azevedo AM, Marques MPC. Mrna Vaccines Manufacturing: Challenges and Bottlenecks. Vaccine (2021) 39(16):2190–200. doi: 10.1016/j.vaccine.2021.03.038
31. Tang J, Cai L, Xu C, Sun S, Liu Y, Rosenecker J, et al. Nanotechnologies in Delivery of DNA and Mrna Vaccines to the Nasal and Pulmonary Mucosa. Nanomaterials (Basel) (2022) 12(2):1–28. doi: 10.3390/nano12020226
32. Shafaati M, Saidijam M, Soleimani M, Hazrati F, Mirzaei R, Amirheidari B, et al. A Brief Review on DNA Vaccines in the Era of COVID-19. Future Virol (2021) 17(1):49–66. doi: 10.2217/fvl-2021-0170
33. Liu MA (2019). A Comparison of Plasmid DNA and mRNA asVaccine Technologies. Vaccines (2019) 7(2):37. doi: 10.3390/vaccines7020037
34. Knezevic I, Liu MA, Peden K, Zhou T, Kang HN. Development of Mrna Vaccines: Scientific and Regulatory Issues. Vaccines (Basel) (2021) 9(2):1–10. doi: 10.3390/vaccines9020081
35. Zeng C, Zhang C, Walker PG, Dong Y. Formulation and Delivery Technologies for Mrna Vaccines. Curr Top Microbiol Immunol (2020) 1–40. doi: 10.1007/82_2020_217
36. Uddin MN, Roni MA. Challenges of Storage and Stability of Mrna-Based COVID-19 Vaccines. Vaccines (Basel) (2021) 9(9):1–9. doi: 10.3390/vaccines9091033
37. Zhang C, Maruggi G, Shan H, Li J. Advances in Mrna Vaccines for Infectious Diseases. Front Immunol (2019) 10:594. doi: 10.3389/fimmu.2019.00594
38. Bruxvoort KJ, Sy LS, Qian L, Ackerson BK, Luo Y, Lee GS, et al. Effectiveness of Mrna-1273 Against Delta, Mu, and Other Emerging Variants of SARS-Cov-2: Test Negative Case-Control Study. BMJ (2021) 375:e068848. doi: 10.1136/bmj-2021-068848
39. Hamady A, Lee J, Loboda ZA. Waning Antibody Responses in COVID-19: What can We Learn From the Analysis of Other Coronaviruses? Infection (2022) 50(1):11–25. doi: 10.1007/s15010-021-01664-z
40. Giannotta G, Giannotta N. Mrna COVID-19 Vaccines and Long-Lived Plasma Cells: A Complicated Relationship. Vaccines (Basel) (2021) 9(12):1055–65. doi: 10.3390/vaccines9121503
41. Saleh A, Qamar S, Tekin A, Singh R, Kashyap R. Vaccine Development Throughout History. Cureus (2021) 13(7):e16635. doi: 10.7759/cureus.16635
42. Plotkin SA. Correlates of Protection Induced by Vaccination. Clin Vaccine Immunol (2010) 17(7):1055–65. doi: 10.1128/CVI.00131-10
43. Towards Africa’s First Mrna Vaccine Technology Transfer Hub (2021). WHO AFRICA. Available at: https://www.afro.who.int/news/towards-africas-first-mrna-vaccine-technology-transfer-hub (Accessed 12 November 2021).
44. PAHO Selects Centers in Argentina, Brazil to Develop COVID-19 Mrna Vaccines (2021). WHO PAHO. Available at: https://www.paho.org/en/news/21-9-2021-paho-selects-centers-argentina-brazil-develop-covid-19-mrna-vaccines (Accessed 12 November 2021).
45. WHO Call for Expressions of Interest to Receive Technology Transfer for Mrna Vaccines and to Become a Mrna Vaccine Manufacturer (2021). WHO website. Available at: www.who.int/news-room/articles-detail/receive-technology-transfer-for-mrna-vaccines-and-to-become-a-mrna-vaccine-manufacturer (Accessed 12 November 2021).
46. Evaluation of the Quality, Safety and Efficacy of Messenger RNA Vaccines for the Prevention of Infectious Diseases: Regulatory Considerations (2021). WHO. Available at: www.who.int/publications/m/item/evaluation-of-the-quality-safety-and-efficacy-of-messenger-rna-vaccines-for-the-prevention-of-infectious-diseases-regulatory-considerations (Accessed 10 December 2021).
Keywords: vaccine, platform, LMICs, neglected tropical disease (NTD), emerging infectious disease (EID)
Citation: Sparrow E, Hasso-Agopsowicz M, Kaslow DC, Singh K, Rao R, Chibi M, Makubalo LE, Reeder JC, Kang G, Karron RA, Cravioto A, Lanata CF, Friede M, Abela-Ridder B, Solomon AW, Dagne DA and Giersing B (2022) Leveraging mRNA Platform Technology to Accelerate Development of Vaccines for Some Emerging and Neglected Tropical Diseases Through Local Vaccine Production. Front. Trop. Dis 3:844039. doi: 10.3389/fitd.2022.844039
Received: 27 December 2021; Accepted: 28 February 2022;
Published: 04 July 2022.
Edited by:
Raphael M. Zellweger, International Vaccine Institute, South KoreaReviewed by:
Ruklanthi De Alwis, Duke-NUS Medical School, SingaporePhilippe Buchy, GlaxoSmithKline, Belgium
Copyright © 2022 World Health Organization 2022. Licensee Frontiers Media SA. This is an open-access article distributed under the terms of the Creative Commons Attribution License (CC BY). The use, distribution or reproduction in other forums is permitted, provided the original author(s) and the copyright owner(s) are credited and that the original publication in this journal is cited, in accordance with accepted academic practice. No use, distribution or reproduction is permitted which does not comply with these terms.
*Correspondence: Erin Sparrow, Sparrowe@who.int