- Instituto de Investigaciones Bioquímicas de La Plata (INIBIOLP), CCT La Plata Consejo Nacional de Investigaciones Científicas y Técnicas (CONICET) - Universidad Nacional de La Plata (UNLP), La Plata, Argentina
Triatomine insects are vectors of the protozoan Trypanosoma cruzi, the causative agent of Chagas disease. Although residual pyrethroid spraying has been a successful vector control strategy for many years, a growing number of pyrethroid-resistance foci is being documented, mainly in Triatoma infestans, that led to failures in vector elimination. Insecticide resistance is a multifactorial phenomenon that often implies a combination of three different mechanisms: increased insecticide detoxification, reduced affinity of the site of action, and reduced insecticide penetration through the cuticle. All three mechanisms were reported in pyrethroid-resistant T. infestans. Cytochrome P450s are enzymes involved in the metabolism of xenobiotics and endogenous chemicals. They are encoded by CYP genes and classified into different families and clans. In triatomines, the CYP4 clan is divided in two families, CYP3093 and CYP4, and both exhibit genome-wide, triatomine-specific gene expansions. Some members from each family have been reported to be involved in two of the mechanisms mentioned above, i.e., they participate in insecticide detoxification in different organs and tissues, and in the synthesis of cuticular hydrocarbons, which ultimately can contribute to a reduced insecticide penetration. The aim of this manuscript is to review the current state of knowledge of P450 genes belonging to the CYP4 clan in triatomines and to highlight their potential role in insecticide resistance.
Introduction
Chagas disease, also known as American trypanosomiasis, is a chronic parasitic infection caused by the protozoa Trypanosoma cruzi, which is transmitted to human mainly through the faeces of infected blood-sucking insects belonging to the subfamily Triatominae (Heteroptera: Reduviidae). Approximately 7 million people worldwide, mostly in Latin America, are estimated to be infected with this parasite (1). There is no treatment for the chronic forms of the disease; thus, interrupting vector transmission represents the best way to reduce the incidence of the disease. Vector control has been primarily based on spraying dwellings with contact insecticide formulations that led to the reduction of triatomine distribution and consequently the interruption of disease transmission in many areas from endemic regions. Pyrethroids have been widely used for triatomine control because of their low toxicity to humans, compared to organophosphate and organochlorine insecticides. However, in the last years it has been repeatedly reported the presence of triatomines, mainly Triatoma infestans, after spraying with pyrethroid insecticides, which was associated with the evolution of resistance to insecticides (2). Contact insecticides are lipophilic compounds that penetrate the cuticle after being sprayed, and then must reach the target site. In the case of pyrethroids, this is the voltage-gated sodium channel in the insect’s nervous system. Contact insecticides are transported through the insect body mainly by haemolymph, however, there are very few studies on the transport mechanisms involved during the initial stages of intoxication (3). Dulbecco et al. (4) evaluated the in vitro binding of deltamethrin to haemolymph’s lipoproteins of T. infestans, showing that lipophorin and very-high-density lipoprotein I (VHDLpI) are mainly responsible for deltamethrin transport.
Insecticide resistance is a multifactorial phenomenon involving i) mutations causing loss of sensitivity of the target site for the insecticide (knockdown resistance), ii) an enhanced inactivation of the insecticide produced by detoxifying enzymes (metabolic resistance), and iii) a diminished penetration of the insecticide through the integument (cuticular resistance factor). Other less studied resistance factors are the insecticide excretion by ATP-binding cassette (ABC) transporters (5), and the insecticide sequestration by olfactory proteins (6). Among mechanisms of deltamethrin resistance, the main three mentioned above were reported in deltamethrin-resistant T. infestans, i.e., increased activity of some detoxification enzymes (7, 8), point mutations in the sodium channel gene (9, 10), and a reduced insecticide penetration related to thickening of the cuticle together with larger amounts of cuticular hydrocarbons (11, 12).
Metabolic resistance to chemical insecticides occurs through increased biodegradation and/or sequestration of the insecticide, usually by overproduction of detoxification enzymes such as cytochrome P450 monooxygenases, glutathione S-transferases, and carboxyl/cholinesterases. Among them, P450s are the primary enzyme superfamily associated with resistance to most insecticides including pyrethroids. They constitute one of the largest superfamilies of enzymes found in nature, catalysing the monooxygenation of a broad range of xenobiotic and endogenous compounds. Cytochrome P450s are encoded by CYP genes. In insects, all CYPs described were classified into one of the following four clans: CYP2, CYP3, CYP4 and the mitochondrial CYP clan (13). Both mitochondrial and CYP2 clans participate in core functions related to development and physiology, whereas CYP3 and CYP4 clans have been associated with environmental interactions. New families belonging to CYP3 and CYP4 clans have been described in the Rhodnius prolixus genome (14) and were also found in transcriptome analyses of other triatomines species such as T. infestans, T. dimidata, and T. pallidipennis (15). Also, some insect-specific members of the CYP4 clan are known to be involved in the last step of cuticular hydrocarbon biosynthesis (16, 17) and have been proposed to contribute to the cuticular resistance factor (18, 19).
As P450s members belonging to CYP4 clan seem to be involved in two of the mentioned deltamethrin resistant mechanisms, i.e., increased insecticide detoxification, and the cuticle hydrocarbon biosynthesis conferring resistance via reducing insecticide uptake, the main objective of this mini review is to describe the current knowledge on CYP4 clan genes in triatomines, with emphasis in T. infestans and R. prolixus, and their relationship with pyrethroid resistance.
Cytochrome P450 Genes Belonging to CYP4 Clan in Triatomines
The CYP4 clan is very diverse in insect genomes (20), with some members involved in insecticide resistance and others with different roles, including biosynthesis of endogenous compounds and odor processing (21–23). In triatomines, it includes the family CYP4 and the triatomine-specific family CYP3093 (Figure 1). Based on the information known to date, both families include gene expansions, particularly in the subfamilies CYP3093A (14 genes, CYP3093A1 to A14, plus 40 gene fragments) and CYP4EM (8 genes, CYP4EM1 to EM7, and CYP4EM10) (14, 24). Expansions of CYP genes are not only restricted to hematophagous insects but are also evident in pyrethroid resistant herbivorous and omnivorous species. For instance, gene expansions within the CYP4 clan were detected in Anopheles gambiae (subfamilies 4D, 4H), Tribolium castaneum (subfamilies 351A, 4BN), and Acyrthosiphon pisum (subfamilies CYP380 and CYP4CJ) (Figure 1). All feeding strategies and their related habitats involve the exposure to specific environmental factors that may promote the evolutive selection of gene expansions, which in turn help the insects to better respond to them. In this regard, the expansions of CYP genes are hypothesized to be a response to environmental stimuli that might drive the potential to acquire insecticide resistance (13).
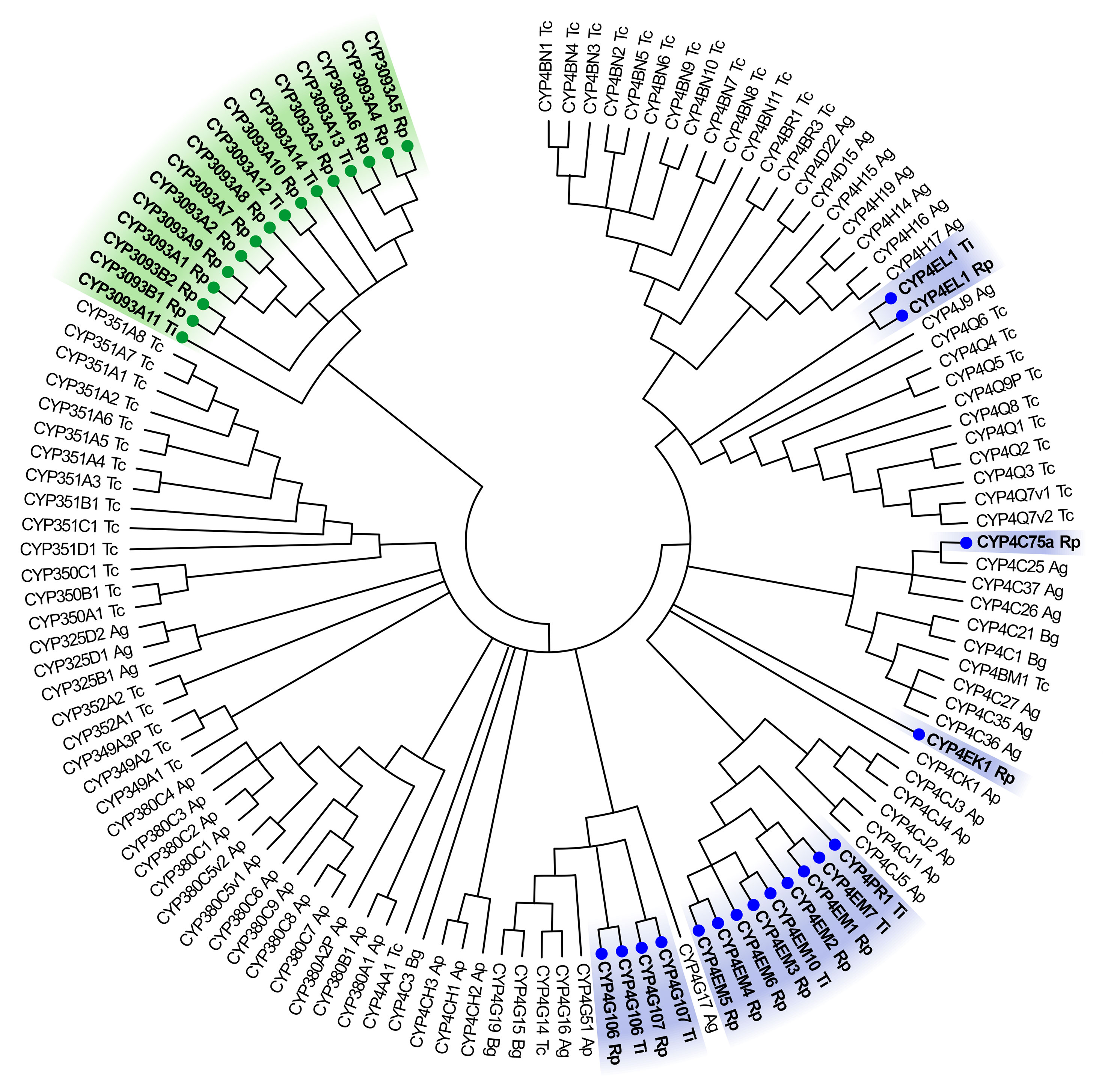
Figure 1 Neighbor-joining consensus tree of CYP4 clan. The phylogenetic tree was generated by MEGA X using the amino acid sequences from Triatoma infestans (Ti), Rhodnius prolixus (Rp), Anopheles gambiae (Ag), Blattella germanica (Bg), Acyrthosiphon pisum (Ap) and Tribolium castaneum (Tc). Triatomine genes are marked with a filled circle; members of CYP3093 family are highlighted in green and members of CYP4 family in blue. All nodes have significant bootstrap support based on 1,000 replicates. The trees were created with cut-off value of 50%.
In triatomines, the complete information about CYP genes (CYPome) is only available for R. prolixus, which is the only species of this group with whole genome available to date (14). It contains 11 genes belonging to the family CYP4, in turn classified into subfamilies C (CYP4C75), G (CYP4G106 and CYP4G107), EK (CYP4EK1), EL (CYP4EL1) and EM (six members: CYP4EM1 to EM6); and 12 genes belonging to the family CYP3093, subfamilies A (CYP3093A1 to A10) and B (CYP3093B1 and B2) (14) (Figure 1).
In T. infestans, the information available about CYP4 clan was recently reported by Dulbecco et al. (25), which mainly comes from two tissue-specific transcriptomes (26, 27). The CYP4 clan is represented by family CYP4, subfamilies EM (CYP4EM7 and EM10), the orthologous genes from subfamilies G (CYP4G106 and CYP4G107), EL (CYP4EL1), and the novel subfamily PR (CYP4PR1) (25). Subfamilies CYP4C and EK, despite being present in R. prolixus genome, had no orthologous members identified in the T. infestans transcriptomes analysed to date, but they could be represented by unnamed short sequences or not yet identified. The family CYP3093 is represented by 4 full-length sequences belonging to the subfamily CYP3093A (CYP3093A11 to A14) (24, 25) (Figure 1).
Traverso et al. (15) performed a normalized transcriptome analysis using eggs, nymphs, and adults of other epidemiologically relevant triatomine vector species, i.e., T. infestans, T. dimidiata and T. pallidipennis. By mining into CYP4 clan transcripts, these authors counted 22 transcripts in T. infestans, 19 in T. dimidiata and 30 in T. pallidipennis; however, no official names were provided in that study. Gene identification is not easy since in general transcriptomes offer short sequences that might correspond to either transcripts of the same gene or products of alternative splicing. Thus, in this review we will focus on full-length and officially named sequences by the P450 Nomenclature Committee (28). Their main characteristics and studies carried out on them are detailed in Table 1.
Roles of Triatomine CYP4 Clan in Insecticide Detoxification and Metabolic Resistance
Regarding insecticide detoxification, Dulbecco et al. (24) used a bioinformatics approach to model the docking of deltamethrin to the active site of all members of the subfamilies CYP3093A and CYPEM from T. infestans and R. prolixus, considering that the most probable pathway for deltamethrin metabolism in insects is the 4’ carbon-hydroxylation (32). All CYP4 clan members of T. infestans showed a favorable docking between the substrate and the active site, suggesting a possible role in deltamethrin metabolism. On the contrary, only 25% of CYP4 clan members of R. prolixus showed favorable interactions. Thus, the specific differences found in the composition of amino acids forming the enzyme’s active site might prevent a favorable interaction between deltamethrin and R. prolixus P450s (24). No functional assays were performed to date to confirm this hypothesis.
The role of three CYP4EM subfamily members (genes EM1, EM2 and EM3) was evaluated in vivo during R. prolixus blood meal and after deltamethrin exposure (29). A differential expression pattern of these genes was found in different insect organs and tissues; CYP4EM1 and CYP4EM2 were expressed in the integument, salivary glands, midgut, fat body and Malpighian tubules, whereas CYP4EM3 was expressed only in the integument, fat body and Malpighian tubules. Remarkably, the expression of CYP4EM1 was much higher in salivary gland than in any other organ/tissue, and it was modulated after insect blood meal. Knocking down of CYP4EM1 by RNAi induced deleterious effects in R. prolixus nymphs after topical application of deltamethrin, suggesting an important role in detoxification (29).
Regarding metabolic resistance, the first report about the potential role of CYP genes in resistance to deltamethrin was done by Grosso et al. (30). These authors characterized the expression profile of CYP4EM7, the same as other CYP3 clan members, in the fat body of four different laboratory strains of T. infestans, both resistant and susceptible nymphs. The colonies came originally from different areas of Argentina and Bolivia. Interestingly, they reported that only CYP4EM7 was highly overexpressed in resistant insects although the expression pattern was different between strains. They also performed a time-course expression profile after deltamethrin topical application. CYP4EM7 gene was inducible by deltamethrin in all the strains analysed (30). The expression differences between strains may have arisen in response to distinct insecticides applied in the places of origin of each population and/or by genetic variations between Argentinian and Bolivian populations. In insects, P450s are inducible by many lipophilic substrates from endogenous and exogenous sources. Differences in treatments with insecticides could have led to the selection of different mechanisms of resistance between populations of this insect vector (2).
Integument CYP and Metabolic Resistance
Detoxification processes are recognized to occur in the gut, Malpighian tubules, and mainly in insect fat body. Recently, cytochrome P450-based detoxification in the integument of deltamethrin-resistant T. infestans has been described for the first time (24). Furthermore, it was reported that most of CYP4 clan members that constitute gene expansions of subfamily CYP3093A and CYP4EM are mainly expressed in the integument tissue. Some of them have high constitutive expression levels in the integument of pyrethroid-resistant T. infestans compared to susceptible specimens, namely CYP3093A11 (130-fold), CYP3093A12 (4-fold) and CYP4EM10 (8-fold) (24). Also, CYP4PR1 (first member of a new subfamily described in triatomines) was 6-fold more expressed in resistant insects compared with susceptible ones (25). These genes did not show overexpression in other tissues, such as fat body, midgut, and Malpighian tubules. Expansions of the other members of both subfamilies showed slight or no expression differences between susceptible and resistant insects in all the tissues examined (24).
RNAi-based knockdown of either CYP3093A11 or CYP4EM10 in T. infestans did not show a clear phenotype after deltamethrin treatment; however, CYP4PR1 was overexpressed (13-fold) in CYP3093A11-silenced insects. Interestingly, subsequent knockdown of CYP4PR1 resulted in increased mortality after topical application of two doses of deltamethrin (25). These authors hypothesized a redundancy in the function of CYP genes, in which some members could compensate the loss of function of the targeted genes by masking the silenced phenotype as observed for both knockdown genes, CYP3093A11 and CYP4EM10.
As previously described, there are many pyrethroid-resistant foci in T. infestans, whereas there is only one low-resistance R. prolixus strain reported (33). The constitutive overexpression of CYP4EM10, CYP3093A11 and CYP4PR1 in T. infestans resistant insects (24), the absence of CYP4PR subfamily in R. prolixus genome (14, 25) and the distinctive interaction between CYP4-clan genes and deltamethrin exhibited by molecular docking in both species (24) are important facts regarding pyrethroid resistance in triatomines. All these results support the role of CYP4 clan members in metabolic resistance.
Roles of Triatomine CYP4 Clan in Hydrocarbon Formation and Cuticular Resistance Factor
The integument is the outer insect tissue, which is formed by the cuticle and the epidermis and plays an essential role in insect fitness and survival (34–36). The cuticle is covered by a complex blend of lipids, with a predominance of very long chain straight and methyl-branched hydrocarbons (HCs) (37). Triatomines synthesize a blend of HCs composed by branched mono-, di-, tri- and tetra-methyl alkanes of 27 to more than 42 carbons in the straight carbon skeleton, together with relatively minor amounts of straight chain alkanes and alkenes of 18 to 33 carbons (38–40). The biosynthesis of the cuticle lipids takes place in the oenocytes (41, 42), which are large ectodermic cells associated with the integument in triatomines (31, 34, 43). The last step in insect hydrocarbon biosynthesis is carried out by an insect-specific cytochrome P450 of the 4G subfamily (CYP4G) (17), catalysing the conversion of long chain aldehydes and alcohols to hydrocarbons (16, 44).
There are two CYP4G genes in most insects, with few exceptions of higher numbers or a single gene in some species (17). Their coded enzymes can show different subcellular localization as it has been shown in A. gambiae (45). R. prolixus has two CYP4G genes, CYP4G106 and G107 (14, 46), and two orthologs of these genes have been also found in a T. infestans integument transcriptome (27). Dulbecco et al. (31) demonstrated that CYP4G106 and CYP4G107 are expressed almost exclusively in the integument of R. prolixus; with very low expression of CYP4G106 in the nervous system. Their expression pattern in integument varies significantly depending on insect age and feeding status. RNAi-based knockdown of either CY4G106 or CYP4G107 and the subsequent analysis of the HCs amount and composition, both internal and cuticular, led the authors to report for the first time the in vivo specificity of CYP4G106 and CYP4G107 to make mostly straight or methyl-branched hydrocarbons, respectively. Molecular docking of the two enzymes with the corresponding long chain straight or methyl-branched hydrocarbon precursors gave further support to their biosynthetic specificity. Also, CYP4G107 was shown to be very important for cuticular lipid barrier formation that avoid insect desiccation (31).
Physiological adaptive changes in the cuticle thickness and the amount of cuticular HCs related to insecticide resistance (cuticle factor) were detected for the first time in T. infestans, where pyrethroid-resistant populations showed larger amounts of cuticle HCs together with a diminished insecticide penetration, compared to susceptible insects (11, 12). Later, it was also described in the mosquito Anopheles gambiae (47), and more recently in the cockroach Blattella germanica (48) and the brown planthopper, Nilaparvata lugens (49). In these species, the overexpression of CYP4G genes in insecticide-resistant strains has been reported (18, 19). Interestingly, the ortholog CYP4G106 of T. infestans was shown to be overexpressed in integument of pyrethroid-resistant insects (27). The higher amount of cuticle HCs, the role of CYP4Gs in their synthesis, and the overexpression in resistant population suggest the involvement of this CYP4 clan subfamily in the cuticular resistant factor. The putative role of CYP4G106 in the nervous system is currently unknown.
Concluding Remarks and Future Directions
The potential role of some CYP4 clan members of triatomines in two of the three main factors involved in insecticide resistance was reviewed. Their constitutive overexpression in specific organs and tissues in resistant populations, the capacity of being modulated by deltamethrin together with the deleterious effects observed in knockdown experiments after topical application of pyrethroids suggest the involvement of these genes in metabolic resistance. Detoxification processes are recognized to occur in the gut, Malpighian tubules, and mainly in the insect fat body. However, it was highlighted the importance of the integument tissue in metabolic resistance, acting not only as a physical barrier but also as the first line of metabolic defence in the insect surface. In addition to the well-known role of CYP4G subfamily members in the biosynthesis of hydrocarbons that waterproof the insect cuticle, the recently reported association with insecticide resistance in the insect surface opens an exciting scenario for further studies. Also, it would be of interest to elucidate the involvement of CYP4 clan members in other physiological activities, and whether these roles are affected in resistant populations, as it has been reported that some physiological functions are diminished by a compensation mechanism of energy expenditure that leads to a resistant phenotype.
P450 is a complex superfamily of enzymes, where several members of each family or clan might perform either similar or overlapping functions. Their expression profile can be distinctive in different tissues, and differentially inducible by the substrates. Both are important reasons why RNAi-based experiments are often not conclusive to determine the function of CYP4 members. In the case of triatomines, the heterologous expression of CYP4-clan proteins has not been carried out to conclusively demonstrate their proposed role. Therefore, it is very important to perform heterologous expression of these proteins to start biochemical assays that confirm their role in both deltamethrin detoxification and as metabolic and cuticular resistance factors. Their kinetics, tissue, and subcellular localization, the same as other important biochemical properties of the P450s encoded by the mentioned CYP genes should be explored in future studies.
Author Contributions
Original draft preparation, AD. Review and editing, AD, GC-F, and NP. Writing the manuscript, NP. All authors contributed to the article and approved the submitted version.
Funding
This research received financial support from CONICET (grant PIP11220150100390) and Agencia I+D+i (grant PICT 2019-01653) to GC-F. GC-F, and NP are members of the CONICET Researcher Career.
Conflict of Interest
The authors declare that the research was conducted in the absence of any commercial or financial relationships that could be construed as a potential conflict of interest.
Publisher’s Note
All claims expressed in this article are solely those of the authors and do not necessarily represent those of their affiliated organizations, or those of the publisher, the editors and the reviewers. Any product that may be evaluated in this article, or claim that may be made by its manufacturer, is not guaranteed or endorsed by the publisher.
Acknowledgments
The authors thank Mario Ramos for figure preparation and Rosana Del Cid for language revision.
References
1. WHO. Chagas Disease (American Trypanosomiasis) (2021). Available at: https://www.who.int/news-room/fact-sheets/detail/chagas-disease-(american-trypanosomiasis) (Accessed November 10, 2021).
2. Mougabure-Cueto G, Picollo MI. Insecticide Resistance in Vector Chagas Disease: Evolution, Mechanisms and Management. Acta Trop (2015) 149:70–85. doi: 10.1016/j.actatropica.2015.05.014
3. Gerolt P. Insecticides: Their Route of Entry, Mechanism of Transport and Mode of Action. Biol Rev (1983) 58:233–74. doi: 10.1111/j.1469-185x.1983.tb00389.x
4. Dulbecco AB, Mijailovsky SJ, Girotti JR, Juárez MP. Deltamethrin Binding to Triatoma Infestans (Hemiptera: Reduviidae) Lipoproteins. Analysis by Solvent Bar Microextraction Coupled to Gas Chromatography. J Med Entomol (2015) 52:1254–9. doi: 10.1093/jme/tjv141
5. Gott RC, Kunkel GR, Zobel ES, Lovett BR, Hawthorne DJ. Implicating ABC Transporters in Insecticide Resistance: Research Strategies and a Decision Framework. J Econ Entomol (2017) 110:667–77. doi: 10.1093/jee/tox041
6. Li L, Zhang WB, Shan YM, Zhang ZR, Pang BP. Functional Characterization of Olfactory Proteins Involved in Chemoreception of. Galeruca Daurica Front Physiol (2021) 12:678–98. doi: 10.3389/FPHYS.2021.678698
7. Picollo MI, Vassena C, Santo Orihuela P, Barrios S, Zaidemberg M, Zerba E. High Resistance to Pyrethroid Insecticides Associated With Ineffective Field Treatments in Triatoma Infestans (Hemiptera: Reduviidae) From Northern Argentina. J Med Entomol (2005) 42:637–42. doi: 10.1603/0022-2585
8. Santo Orihuela PL, Vassena CV, Zerba EN, Picollo MI. Relative Contribution of Monooxygenase and Esterase to Pyrethroid Resistance in Triatoma Infestans (Hemiptera: Reduviidae) From Argentina and Bolivia. J Med Entomol (2008) 45:298–306. doi: 10.1603/0022-2585
9. Fabro J, Sterkel M, Capriotti N, Mougabure-Cueto G, Germano M, Rivera-Pomar R, et al. Identification of a Point Mutation Associated With Pyrethroid Resistance in the Para-Type Sodium Channel of Triatoma Infestans, a Vector of Chagas’ Disease. Infect Genet Evol (2012) 12:487–91. doi: 10.1016/j.meegid.2011.12.006
10. Capriotti N, Mougabure-Cueto G, Rivera-Pomar R, Ons S. L925I Mutation in the Para-Type Sodium Channel is Associated With Pyrethroid Resistance in Triatoma Infestans From the Gran Chaco Region. PloS Negl Trop Dis (2014) 8:20. doi: 10.1371/journal.pntd.0002659
11. Pedrini N, Mijailovsky SJ, Girotti JR, Stariolo R, Cardozo RM, Gentile A, et al. Control of Pyrethroid-Resistant Chagas Disease Vectors With Entomopathogenic Fungi. PloS Negl Trop Dis (2009) 3:e434. doi: 10.1371/journal.pntd.0000434
12. Juárez MP, Pedrini N, Girotti JR. Pyrethroid Resistence in Chagas Disease Vectros: The Case of Triatoma Infestans Cuticle. Resist Pest Manag (2010) 19:59–61.
13. Feyereisen R. Arthropod CYPomes Illustrate the Tempo and Mode in P450 Evolution. Biochim Biophys Acta (2011) 1814:19–28. doi: 10.1016/j.bbapap.2010.06.012
14. Schama R, Pedrini N, Juárez MP, Nelson DR, Torres AQ, Valle D, et al. Rhodnius Prolixus Supergene Families of Enzymes Potentially Associated With Insecticide Resistance. Insect Biochem Mol Biol (2016) 69:91–104. doi: 10.1016/j.ibmb.2015.06.005
15. Traverso L, Lavore A, Sierra I, Palacio V, Martinez-Barnetche J, Latorre-Estivalis JM, et al. Comparative and Functional Triatomine Genomics Reveals Reductions and Expansions in Insecticide Resistance-Related Gene Families. PloS Negl Trop Dis (2017) 11:1–25. doi: 10.1371/journal.pntd.0005313
16. Qiu Y, Tittiger C, Wicker-Thomas C, Le Goff G, Young S, Wajnberg E, et al. An Insect-Specific P450 Oxidative Decarbonylase for Cuticular Hydrocarbon Biosynthesis. Proc Natl Acad Sci USA (2012) 109:14858–63. doi: 10.1073/pnas.1208650109
17. Feyereisen R. Origin and Evolution of the CYP4G Subfamily in Insects, Cytochrome P450 Enzymes Involved in Cuticular Hydrocarbon Synthesis. Mol Phylogenet Evol (2020) 143:106695. doi: 10.1016/j.ympev.2019.106695
18. Pridgeon JW, Zhang L, Liu N. Overexpression of CYP4G19 Associated With a Pyrethroid-Resistant Strain of the German Cockroach, Blattella Germanica (L.). Gene (2003) 314:157–63. doi: 10.1016/s0378-1119(03)00725-x
19. Ingham VA, Jones CM, Pignatelli P, Balabanidou V, Vontas J, Wagstaff SC, et al. Dissecting the Organ Specificity of Insecticide Resistance Candidate Genes in Anopheles Gambiae: Known and Novel Candidate Genes. BMC Genomics (2014) 15:1–9. doi: 10.1186/1471-2164-15-1018
20. Feyereisen R. Insect CYP Genes and P450 Enzymes. San Diego (California, USA): Elsevier B.V (2012). doi: 10.1016/B978-0-12-384747-8.10008-x
21. Maïbèche-Coisne M, Monti-Dedieu L, Aragon S, Dauphin-Villemant C. A New Cytochrome P450 From Drosophila Melanogaster, CYP4G15, Expressed in the Nervous System. Biochem Biophys Res Commun (2000) 273:1132–7. doi: 10.1006/bbrc.2000.3058
22. Maïbèche-Coisne M, Jacquin-Joly E, François MC, Nagnan-Le Meillour P. cDNA Cloning of Biotransformation Enzymes Belonging to the Cytochrome P450 Family in the Antennae of the Noctuid Moth Mamestra Brassicae. Insect Mol Biol (2002) 11:273–81. doi: 10.1046/j.1365-2583.2002.00335.x
23. Maïbèche-Coisne M, Merlin C, François MC, Porcheron P, Jacquin-Joly E. P450 and P450 Reductase cDNAs From the Moth Mamestra Brassicae: Cloning and Expression Patterns in Male Antennae. Gene (2005) 346:195–203. doi: 10.1016/j.gene.2004.11.010
24. Dulbecco AB, Moriconi DE, Calderón-Fernández GM, Lynn S, McCarthy A, Roca-Acevedo G, et al. Integument CYP Genes of the Largest Genome-Wide Cytochrome P450 Expansions in Triatomines Participate in Detoxification in Deltamethrin-Resistant Triatoma Infestans. Sci Rep (2018) 8:2–13. doi: 10.1038/s41598-018-28475-x
25. Dulbecco AB, Moriconi DE, Pedrini N. Knockdown of CYP4PR1, a Cytochrome P450 Gene Highly Expressed in the Integument Tissue of Triatoma Infestans, Increases Susceptibility to Deltamethrin in Pyrethroid-Resistant Insects. Pestic Biochem Physiol (2021) 173:1–7. doi: 10.1016/j.pestbp.2021.104781
26. Schwarz A, Medrano-Mercado N, Schaub GA, Struchiner CJ, Bargues MD, Levy MZ, et al. An Updated Insight Into the Sialotranscriptome of Triatoma Infestans: Developmental Stage and Geographic Variations. PloS Negl Trop Dis (2014) 8:e3372. doi: 10.1371/journal.pntd.0003372
27. Calderón-Fernández GM, Moriconi DE, Dulbecco AB, Patricia Juárez M. Transcriptome Analysis of the Triatoma Infestans (Hemiptera: Reduviidae) Integument. J Med Entomol (2017) 54:1531–42. doi: 10.1093/jme/tjx151
28. Nelson DR. The Cytochrome P450 Homepage. Hum Genomics (2009) 4:59–65. doi: 10.1186/1479-7364-4-1-59
29. Paim RMM, Pessoa GCD, Nascimento BWL, Nascimento AMD, Pinheiro LC, Koerich LB, et al. Effect of Salivary CYP4EM1 and CYP4EM2 Gene Silencing on the Life Span of Chagas Disease Vector Rhodnius Prolixus (Hemiptera, Reduviidae) Exposed to Sublethal Dose of Deltamethrin. Insect Mol Biol (2021) 31:49–59. doi: 10.1111/imb.12737
30. Grosso CG, Blariza MJ, Mougabure-Cueto G, Picollo MI, García BA. Identification of Three Cytochrome P450 Genes in the Chagas’ Disease Vector Triatoma Infestans: Expression Analysis in Deltamethrin Susceptible and Resistant Populations. Infect Genet Evol (2016) 44:459–70. doi: 10.1016/j.meegid.2016.07.027
31. Dulbecco AB, Moriconi DE, Lynn S, McCarthy A, Juárez MP, Girotti JR, et al. Deciphering the Role of Rhodnius Prolixus CYP4G Genes in Straight and Methyl-Branched Hydrocarbon Formation and in Desiccation Tolerance. Insect Mol Biol (2020) 29:431–43. doi: 10.1111/imb.12653
32. Stevenson BJ, Bibby J, Pignatelli P, Muangnoicharoen S, O’Neill PM, Lian LY, et al. Cytochrome P450 6M2 From the Malaria Vector Anopheles Gambiae Metabolizes Pyrethroids: Sequential Metabolism of Deltamethrin Revealed. Insect Biochem Mol Biol (2011) 41:492–502. doi: 10.1016/j.ibmb.2011.02.003
33. Vassena CV, Picollo MI, Zerba EN. Insecticide Resistance in Brazilian Triatoma Infestans and Venezuelan Rhodnius Prolixus. Med Vet Entomol (2000) 14:51–5. doi: 10.1046/j.1365-2915.2000.00203.x
34. Wigglesworth VB. The Physiology of the Cuticle and of Ecdysis in Rhodnius Prolixus (Triatomidae, Hemiptera); With Special Reference to the Function of the Oenocytes and of the Dermal Glands. J Cell Sci (1933) s2-76:269–318. doi: 10.1242/jcs.s2-76.302.269
35. Juárez MP, Calderón-Fernández GM. Cuticular Hydrocarbons of Triatomines. Comp Biochem Physiol A Mol Integr Physiol (2007) 147:711–30. doi: 10.1016/j.cbpa.2006.08.031
36. Gutierrez E, Wiggins D, Fielding B, Gould AP. Specialized Hepatocyte-Like Cells Regulate Drosophila Lipid Metabolism. Nature (2007) 445:275–80. doi: 10.1038/nature05382
37. Blomquist GJ. Biosynthesis of Cuticular Hydrocarbons. In: Blomquist GJ, Bagnère A-G, eds. Insect Hydrocarbons: Biology, Biochemistry, and Chemical Ecology. Cambridge, UK: Cambridge University Press (2010). p.35–52. doi: 10.1017/cbo9780511711909.004
38. Juárez P, Blomquist GJ, Schofield CJ. Hydrocarbons of Rhodnius Prolixus, a Chagas Disease Vector. Comp Biochem Physiol B Biochem Mol Biol (2001) 129:733–46. doi: 10.1016/S1096-4959(01)00380-3
39. Calderón-Fernández GM, Girotti JR, Juárez MP. Cuticular Hydrocarbons of Triatoma Dimidiata (Hemiptera: Reduviidae): Intraspecific Variation and Chemotaxonomy. J Med Entomol (2011) 48:262–71. doi: 10.1603/me10141
40. Calderón-Fernández GM, Juárez MP. The Cuticular Hydrocarbons of the Triatoma Sordida Species Subcomplex (Hemiptera: Reduviidae). Mem Inst Oswaldo Cruz (2013) 108:78–784. doi: 10.1590/0074-0276108062013015
41. Fan Y, Zurek L, Dykstra MJ, Schal C. Hydrocarbon Synthesis by Enzymatically Dissociated Oenocytes of the Abdominal Integument of the German Cockroach, Blattella Germanica. Naturwissenschaften (2003) 90:121–6. doi: 10.1007/s00114-003-0402-y
42. Wicker-Thomas C, Garrido D, Bontonou G, Napal L, Mazuras N, Denis B, et al. Flexible Origin of Hydrocarbon/Pheromone Precursors in Drosophila Melanogaster. J Lipid Res (2015) 56:2094–101. doi: 10.1194/jlr.m060368
43. Juárez P, Brenner RR. Fatty Acid Biosynthesis in the Integument Tissue of Triatoma Infestans. Comp Biochem Physiol – Part B Biochem (1989) 93:763–72. doi: 10.1016/0305-0491(89)90043-6
44. MacLean M, Nadeau J, Gurnea T, Tittiger C, Blomquist GJ. Mountain Pine Beetle (Dendroctonus Ponderosae) CYP4Gs Convert Long and Short Chain Alcohols and Aldehydes to Hydrocarbons. Insect Biochem Mol Biol (2018) 102:11–20. doi: 10.1016/j.ibmb.2018.09.005
45. Kefi M, Balabanidou V, Douris V, Lycett G, Feyereisen R, Vontas J. Two Functionally Distinct CYP4G Genes of Anopheles Gambiae Contribute to Cuticular Hydrocarbon Biosynthesis. Insect Biochem Mol Biol (2019) 110:52–9. doi: 10.1016/j.ibmb.2019.04.018
46. Majerowicz D, Calderón-Fernández GM, Alves-Bezerra M, De Paula IF, Cardoso LS, Juárez MP, et al. Lipid Metabolism in Rhodnius Prolixus: Lessons From the Genome. Gene (2017) 596:27–44. doi: 10.1016/j.gene.2016.09.045
47. Balabanidou V, Kampouraki A, Maclean M, Blomquist GJ, Tittiger C, Juárez MP, et al. Cytochrome P450 Associated With Insecticide Resistance Catalyzes Cuticular Hydrocarbon Production in Anopheles Gambiae. Proc Natl Acad Sci USA (2016) 113:9268–73. doi: 10.1073/pnas.1608295113
48. Chen N, Pei XJ, Li S, Fan YL, Liu TX. Involvement of Integument-Rich CYP4G19 in Hydrocarbon Biosynthesis and Cuticular Penetration Resistance in Blattella Germanica (L.). Pest Manag Sci (2020) 76:215–26. doi: 10.1002/ps.5499
Keywords: triatomine, Triatoma infestans, Rhodnius prolixus, insect integument, deltamethrin, hydrocarbon biosynthesis, metabolic resistance, cuticular factor
Citation: Dulbecco AB, Calderón-Fernández GM and Pedrini N (2022) Cytochrome P450 Genes of the CYP4 Clan and Pyrethroid Resistance in Chagas Disease Vectors. Front. Trop. Dis 3:823093. doi: 10.3389/fitd.2022.823093
Received: 26 November 2021; Accepted: 18 January 2022;
Published: 10 February 2022.
Edited by:
Nathan Daniel Burkett-Cadena, University of Florida, United StatesReviewed by:
Rajnikant Dixit, National Institute of Malaria Research (ICMR), IndiaCopyright © 2022 Dulbecco, Calderón-Fernández and Pedrini. This is an open-access article distributed under the terms of the Creative Commons Attribution License (CC BY). The use, distribution or reproduction in other forums is permitted, provided the original author(s) and the copyright owner(s) are credited and that the original publication in this journal is cited, in accordance with accepted academic practice. No use, distribution or reproduction is permitted which does not comply with these terms.
*Correspondence: Gustavo M. Calderón-Fernández, Z2NhbGRlcm9uZkBtZWQudW5scC5lZHUuYXI=; Nicolás Pedrini, bnBlZHJpbmlAbWVkLnVubHAuZWR1LmFy