- 1Department of Chemistry and Physics, Sokoine University of Agriculture, Morogoro, Tanzania
- 2Department of Veterinary and Animal Sciences, University of Copenhagen, Frederiksberg C, Denmark
- 3Department of Biological and Pre-clinical Studies, Institute of Traditional Medicine, Muhimbili University of Health and Allied Sciences, Dar es Salaam, Tanzania
- 4Department of Biosciences, College of Natural and Applied Sciences, Sokoine University of Agriculture, Morogoro, Tanzania
- 5Department of Clinical Pharmacology and Therapeutics, Hubert Kairuki Memorial University, Dar es Salaam, Tanzania
- 6Department of Administration, Tanzania Industrial Research and Development Organisation, Dar es Salaam, Tanzania
- 7Department of Microbiology and Parasitology, School of Medicine and Dentistry, University of Dodoma, Dodoma, Tanzania
- 8Department of Veterinary Medicine and Public Health, Sokoine University of Agriculture, Morogoro, Tanzania
Introduction: Synadenium glaucescens has been used for the treatment of bacterial infections in many parts of the world. We investigated the antibacterial and cytotoxicity activities of secondary metabolites of this plant.
Methods: Hexane, dichloromethane, methanol, and water were used as extraction solvents. The extract of the root bark was fractionated with ethyl acetate and methanol. The isolation of compounds from root barks, leaves and stem wood extracts were carried out using column chromatography. Antibacterial activities were characterized based on growth curves, killing curves and MIC determinations. Haemolytic effect towards sheep red blood cells (RBCs) was analysed with spectrophotometer at the wavelength of 540nm.
Results and Discussion: Extracts from whole root and root bark showed strong activity against Staphylococcus aureus, and Streptococci and Enterococci species, and moderate to weak activity against Escherichia coli, Klebsiella pneumoniae, Pseudomonas aeruginosa, Salmonella species, Shigella sonnei and Yersinia enterocolitica. Staphylococcus aureus was the most susceptible, and E. coli and Klebsiella pneumonia were the least susceptible ones. Likewise, extracts, fractions, sub-fractions and epifriedelanol demonstrated bacteriostatic activity against S. aureus. The haemolytic activity of the extracts, fractions, sub-fractions and epifriedelanol was significantly low compared to the positive control, hydrogen peroxide. But extract from leaves showed high haemolytic effects at the concentrations of 500 μg/mL and 1000 μg/mL. Thus, extracts of S. glaucescens have antibacterial activity against several Gram-positive bacteria including Methicillin Resistant S. aureus with low haemolytic activity. At high concentrations, the extracts from leaves have toxicity risk. More studies for the active compounds are required for biological testing.
1. Introduction
Medicinal plants have always been important for disease management in humans and animals (1). They are normally used directly for therapeutic purposes, but they can also be seen as precursors for the synthesis of useful drugs (2). Synadenium glaucescens is a medicinal plants used to treat infectious diseases such as diarrhea, tuberculosis, sores, wounds and Newcastle (3).
S. glaucescens belongs to the family Euphorbiaceae and it is commonly known as milk bush plant in English, mvunjakongwa in Swahili and liyugi in Bena. Ethnomedical and ethnoveterinary use of the decoctions of leaves, roots and stems of this plant has been reported in Bena people of Tanzania for the treatment of different diseases including wound and skin diseases. The root decoction is used for the treatment of tooth ache, cough, tuberculosis, sexual transmitted infections and Human Immunodeficiency Virus, and the stem decoction is also used against Gastrointestinal worm and ringworms (4). S. glaucescens contains secondary metabolites such as terpenoids, steroids, alkaloids, flavonoids, anthraquinones, tannins, coumarins, glycosides (5, 6) which may have antimicrobial activity (7) and root extract of the plant has been reported in preliminary testings to act against Staphylococcus aureus and Pseudomonas aeruginosa (8). However, the activity has not been thouroughly characterized, including how activity differs between different extracts, fractions and compounds, the principle mode of action (bacteriocidal or bacteriostatic), and the spectrum of activity against different medically important bacteria.
Given that plants contain secondary metabolites, that may have toxic effects such as cytotoxicity, allergic reactions, irritation of the gastrointestinal tract, and injury to vital body organs such as the heart, liver, kidney, and it may be carcinogenicity (9). Toxicity assesment is a key factor during characterization of lead molecules during drug discovery, and haemolytic activity represents a useful first line information of such properties (10). Nevertheless, the haemolysis activity of S. glaucescens has never been investigated.
Thus, the aim of the current study was to characterize the antibacterial activities of extracts, fractions, sub-fractions and compounds of S. glaucescens with regard to strength, spectrum of activity, mode of action, and to determine putative cytotoxicity of these substances towards RBCs.
2. Materials and methods
2.1. Bacterial strains and growth media
Ninety five strains of bacteria were obtained from the Department of Veterinary and Animal Science, University of Copenhagen, Denmark, Department of Microbiology, Sokoine University of Agriculture, Tanzania, and Muhimbili University of Health and Allied Sciences, Tanzania (Supplementary Table S1). In addition, a set of reference strains were included: Staphylococcus aureus ATCC25923, Escherichia coli ATCC25922, Klebsiella pneumonia ATCC700603, Pseudomonas aeruginosa ATCC27853, Enterococcus faecium ATCC51559, Streptococcus uberis ATCC854 and Streptococcus agalactiae ATCC12403. Bacteria were propagated on Mueller Hinton agar (MHA) and Mueller Hinton broth (MHB) (Sigma Aldrich, St Louis, USA) at 37°C for 16-24 hours.
2.2. Plant collection, processing and preparation of extracts, fractions and compounds
Fresh plant samples of S. glaucescens were collected in Njombe district in the Southern Highlands of Tanzania (S08°47’08.5” E 34°53’22.2”). The voucher specimen number HOS/FM 3672 is deposited at the herbarium of Botany Department, College of Natural and Applied Sciences,University of Dar es Salaam, Tanzania. Plants names have been verified at http://www.theplantlist.org. The leaves, roots and stem of the target plants were collected. The roots were manually separated into root bark and rootwood and the stems into stem bark and stem wood. All plants parts were chopped into small pieces and dried at 20 °C at the Tanzania Tree Seed Agency Laboratory, Morogoro. The dried samples were milled to particle size of 2 mm using milling machine (Christy Hunt Engineering Ltd, England). Solvents of different polarities including hexane, dichloromethane and methanol (Sigma Aldrich, Saint Louis, USA) were used to obtain crude extracts from the plant parts using cold (total and sequential) and hot methods of extraction with some modification (11, 12). For cold-total extraction, powdered materials were soaked in a solvent for 48 hours then filtered and for cold-sequential method, residue from dichhloromethane were resoaked with methanol for 48 hours before filtered. The obtained liquid extracts were filtered using Whatman™qualitative filter paper1, 24.0 cm (GE Healthcare Life Sciences, China) in syntax glass. In hot method, powdered materials were boiled for 4 hours in a soxhlet extractor with solvents of different polarities and temperatures to obtain liquid extracts. For the decoction method, powdered samples were soaked in water for about 30 seconds; boiled in a charcoal burner for 30 minutes and then allowed to cool then filtered. All filtrates from organic solvents were concentrated in a rotary evaporator at 40-55 °C to get extracts. The filtrates from water were freezed at -20 °C and taken to the freeze drier (ATS company, Pennsylvania, USA) and other extracts were completely frozen and placed in a freeze-dryer to remove traces of solvents. The dried extracts were stored at -20°C before running bioassays. The matrix of extracts from different parts of the plant (leaves, roots and stems) and with different extraction solvents are summarized in Supplementary Table S2. Further, the methanol root bark from cold-total extraction was fractionated sequentially with hexane, ethyl acetate and methanol solvents by vaccum liquid chromatography through Buchner funnel. The resulting fractions were subjected to column chromatograph to obtain sub-fractions, which were then purified to yield two compounds. The compounds were epifriedelanol (1) (Figure 1) and euphol (2) isolated from ethyl acetate fraction. Other compounds used were isolated from leaves extract tetracosane (3), hexadecyl palmitate (4), octacosane (5), tetracosanol (6) and β-sitosterol (7); stem wood extract were 1-hexacosene (8), campesterol (9) and tetracosanoic acid (10). These compounds were kindly donated by Frank Rewgoshora and David Credo and were published (13, 14).
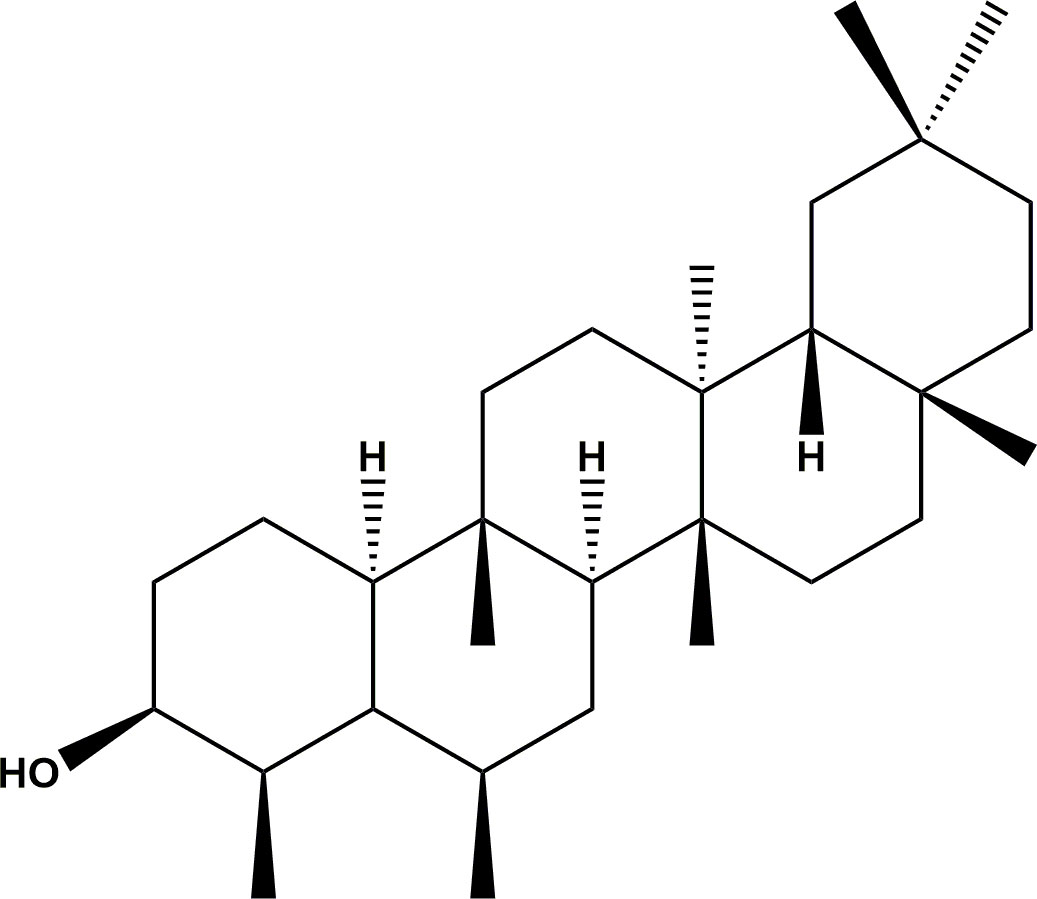
Figure 1 The structure of epifriedelanol. The structure was drawn by using ChemDraw Professional 16.0 software.
2.3. Growth inhibition assay
Growth inhibition assay was performed in honey comb multi well plate using the Bioscreen C machine (Thermo Fischer Scientific, Finland). Bacteria were grown in MHB overnight, and the broth was diluted by adding MHB to the desired Optical Density (OD600) equal to 0.10 (108 CFU/mL). The extracts were dissolved in Dimethyl-Sulphoxide (DMSO) (Sigma Aldrich, Germany) to make concentrationsof 1500 µg/mL and 150µg/mL in MHB, which was mixed with the bacteria in MHB. The plates were incubated for 24 hours with continuous shaking at 37°C and Optical Density (OD600) readings at intervals of 20 minutes. The experiment was performed in triplicates, and negative controls in the form of wells with bacteria and solvent used to dissolve the extract and wells with broth and bacteria alone were included in the plates. Bacteria were also added to wells with tetracycline (4 µg/mL) and gentamicin (4 µg/mL) (Sigma Aldrich, Germany). Growth curves of Log10 optical density against time were visualized using GraphpadPrism8 software (San Diego, USA).
2.4. Minimum inhibitory concentration
Minimum Inhibitory Concentration (MIC) was determined in duplicates by two folds micro-dilution tests performed in a 96-well microtitre plates (15). In brief, test organisms in concentrations equivalent to 0.5 McFarland (1.5 x 108 CFU/mL) were diluted to 100 folds to reach a concentration of 106 CFU/mL. The inoculum was added to well containing 2 fold dilutions of extracts (range 1500 µg/mL to 0.00075 µg/mL) or the antimicrobial gentamicin (range 0.016 µg/mL to 0.000004 µg/mL). Two additional rows were used, one as sterility control, in which, neither drug nor bacteria were added, and one as growth control in which only bacteria and broth were added. The microtitre plates were incubated at 37°C for 16-18 hours. The MIC was taken visually from the well of the lowest concentration showing no bacteria turbidity. The antimicrobial activity of extracts was classified as strong if the MIC range was <500 µg/mL, moderate if MIC range was >500 to 1500 µg/mL and weak if the MIC range was >1500 µg/mL (16).
2.5. Time kill assay
Time-kill assay of Staphylococcus aureus (ATCC25923) and Escherichia coli (ATCC25922) were carried out according to the procedures described by (17) with slight modifications. One mL of MHB was added to test tubes 1-9. Then 1mL of plant extracts was added to test tube 1 followed by two-fold serial dilutions starting with the 2nd tube and continued to the 5th tube leaving a volume of 1 mL in each test tube. A total of 1mL of gentamicin (4 µg/mL) was added in tube 6. Tube 7, 8 and 9 were reserved as controls for the broth with no bacteria, bacteria alone and bacteria with solvent (DMSO) respectively. Then, 1 mL of MHB was added to each tube to make a volume of 2 mL. The turbidity of bacteria was adjusted and visually compared to 0.5 McFarland corresponding to approximately 1.5 x108 CFU/mL and then diluted 100 folds. One hundred µl of the bacteria suspension was added to all tubes except tube 7 and mixed by vortex at low speed. This resulted into an initial inoculum size of approximately 3.5 x105 CFU/mL. The number of colony-forming units (CFU) was determined by plating serial dilutions in Phosphate Buffer Saline on MHA at times 0, 2, 4, 6, 8, 10, 12 and 24 hours after inoculation. Plates were incubated over night at 37 °C. Graphs of the Log10 CFU/mL were plotted against time using GraphpadPrism8 software (San Diego, USA). Time kill curves were determined in triplicates.
2.6. Haemolysis assay
Haemolysis activity was measured according to (18). Briefly, sheep blood was collected in vacuutainer tubes. The blood was centrifuged at 3500 rpm followed by three times wash for 5 minutes to obtain red blood cells (RBCs). The obtained RBCs suspension were prepared at 2% in phosphate buffer pH= 7.4. Extracts were prepared at concentrations of 100, 200, 500, 1000 µg/mL. Two mL of RBCs suspension, PBS and plant extract were added to reach the final volume of 4 mL. Hydrogen peroxide (H2O2) at a concentration of 3% was used as control to induce total haemolysis. The solvent (DMSO) was used as negative control. After 30 minutes of incubation at room temperature, the mixtures were centrifuged at 3500 rpm for 10 minutes and the resulting supernatant was removed and used to evaluate haemolytic activity using a spectrophotometer at the absorbance wavelength of 540 nm. All the experiments were done in three independent experiments. Determination of a percentage haemolysis was calculated by the substraction of the negative control absorbance from the extracts absorbance relative to the positive control absorbance. The final result was multiplied by 100. The interpretation of percentage haemolysis was compared to positive control and considered as low haemolytic activity between 0-40% (18).
2.7. Statistical analysis
The MIC data were expressed as medians and interquartile ranges (IQR). In cases where there were growths in all dilutions, the MIC value was set to the highest tested concentration for statistical purposes. Shapiro-Wilk test was used to test the normality of the data. Comparison of the mean between groups was performed using a Mann-Whitney U test. Comparison of more than two groups was performed by the Kruskal-Wallis test. The IBM Statistical Package for Social Sciences (SPSS) programme, version 20 was used. A two-tailed p-value of < 0.05 level was considered statistically significant.
3. Results
3.1. Growth inhibition assay
All plant extracts were first tested for their ability to inhibit the growth of two reference bacteria; the Gram-positive S. aureus ATCC25923 and the Gram-negative E.coli ATCC25922. For the first 5 hours, growth in all samples resembled the growth of the controls. After this time point, the growth of S. aureus was inhibited by cold–total methanol extract of root bark and leaves, and cold-sequential methanol extract of the whole root of S. glaucescens at concentrations of 150 µg/mL. No growth was observed in controls treated with tetracycline or gentamicin (Figure 2A). None of the extracts inhibited the growth of E. coli at a concentration of 150 µg/mL and 1500 µg/mL (Figure 2B). Full data with inactivation curves for all extracts in different concentrations appear in Supplementary Figure S1.
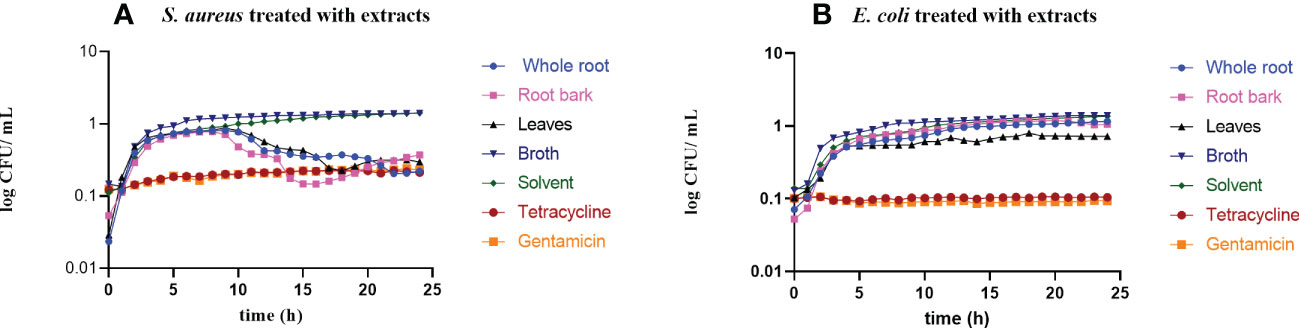
Figure 2 Growth curves of S. aureus ATCC25923 (A) and E. coli ATCC25922 (B) in MHB with active extracts at the concentration of 150 µg/mL. Solvent controls consisted of DMSO, and tetracycline and gentamicin in the concentrations 4 µg/mL were included for comparison.
3.2. Minimum inhibitory concentration
Active extracts selected from the growth inhibition assay were subjected to MIC determination against a wider collection of Gram-positive (Staphyllococcus aureus, Streptococci species and Enterococci species) and Gram-negative bacteria (Escherichia coli, Pseudomonas aeruginosa, Klebsiella pneumoniae, Salmonella species, Shigella species, Yersinia enterocolitica). The median MIC of extract was significantly lower for Gram-positive bacteria [90 µg/mL (IQR 30-750 µg/mL), n = 114] than for Gram-negative bacteria [3000 µg/mL (IQR 1500->3000 µg/mL), n = 171], P<0.0001. The extracts from the whole root [MIC = 40 µg/mL (IQR 30-210 µg/mL)] and root bark [MIC =20 µg/mL (IQR 10-190 µg/mL)] exhibited higher activity against Gram-positive bacteria than extracts from leaves [MIC =380 µg/mL (IQR 380-1500 µg/mL)] (P<0.001). The whole root and root bark extracts exhibited strong activity against the Gram-positive bacteria, S. aureus, Enterococci spp. and Streptococci spp., while extracts from leaves demonstrated moderate activity against these bacteria.
When tested against Gram-negative bacteria, extracts of the whole root and root bark exhibited moderate activity against Shigella species and Pseudomonas aeruginosa while they displayed weak activity against Escherichia coli and Klebsiella pneumoniae. Similarly, extracts from leaves displayed weak activity except against P. aeruginosa, where it demonstated moderate activity. In addition, the extracts from whole root and root bark showed strong activity against Yersinia enterocolitica, while the extract from leaves exhibited moderate activity. However, the number of strains tested in this species was low (n = 2). The MIC values of all extracts were significantly higher than that of gentamicin for the same bacteria (p<0.0001) (Table 1).
An extended study was done on determination of MIC of the most active extract (root bark with MIC 20 µg/mL (IQR 10-190 µg/mL)), its fractions, sub-fractions and the isolated compounds against three strains S. aureus and an E. Ccoli. The fractions, sub-fractions and the compound epifriedelanol (1) demonstrated the same activity against the tested S. aureus strains as the crude extract, suggested that the activity was related to these fractions and the specific compound. Other compounds displayed weak activity against S. aureus. All fractions, sub-fractions and compounds (1-10) exhibited weak activity against E. coli, similar to the crude extract (Table 2).
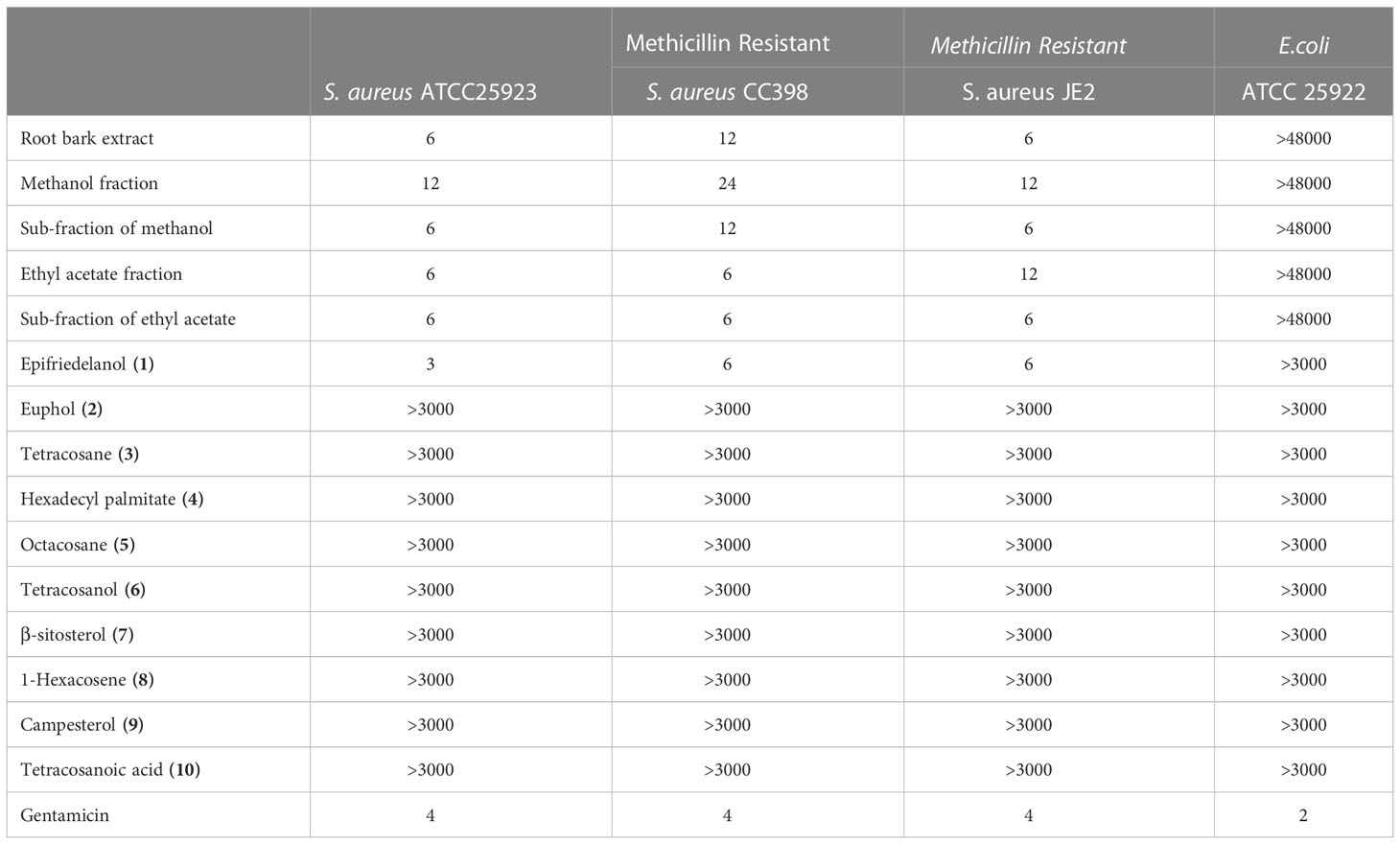
Table 2 MIC (µg/mL) of root bark, its fractions, sub-fractions and the compounds against S. aureus strains and E. coli.
3.3. Time kill kinetics
The kinetic effect (bacteriostatic versus bactericidal) of the three active extracts (whole root, root bark, and leaves), fractions, sub-fractions and epifriedelanol (1) were determined against Staphylococcus aureus (ATCC25923) and Escherichia coli (ATCC25922). The time kill curves revealed that the effect was static, such as, in the situation where antibactial activity was observed, it caused stop of growth, but it did not kill the bacteria. There was a significant growth inhibition in CFU/mL after 24 hours for the whole root and root bark extracts at a concentration of 3000-1500 µg/mL, root bark fractions, sub-fractions and a compound epifriedelanol at the concentration of 3000-750 µg/mL. Extracts from leaves also exhibited significant inhibition at the concentrations of 6000-3000 µg/mL when compared to the untreated groups. In contrast at lower concentration, extracts, fractions, sub-fractions and active compound allowed growth of the bacteria, slightly higher than in the untreated groups. However, the difference was not statistically significant (p> 0.05). Treatment with gentamicin resulted in fast reduction of CFU, and no colonies were formed after 4 hours of treatment with this drug. Full account of the comparison within and between treated group against control groups are shown in Figures 3A–H and Suplementary Tables S3 and S4. For E. coli, only the root bark of S. glaucescens was tested at the concentrations of 24000, 12000, 6000 and 3000 µg/mL, and, no significant difference to the growth curve of the untreated control was observed (data not shown).
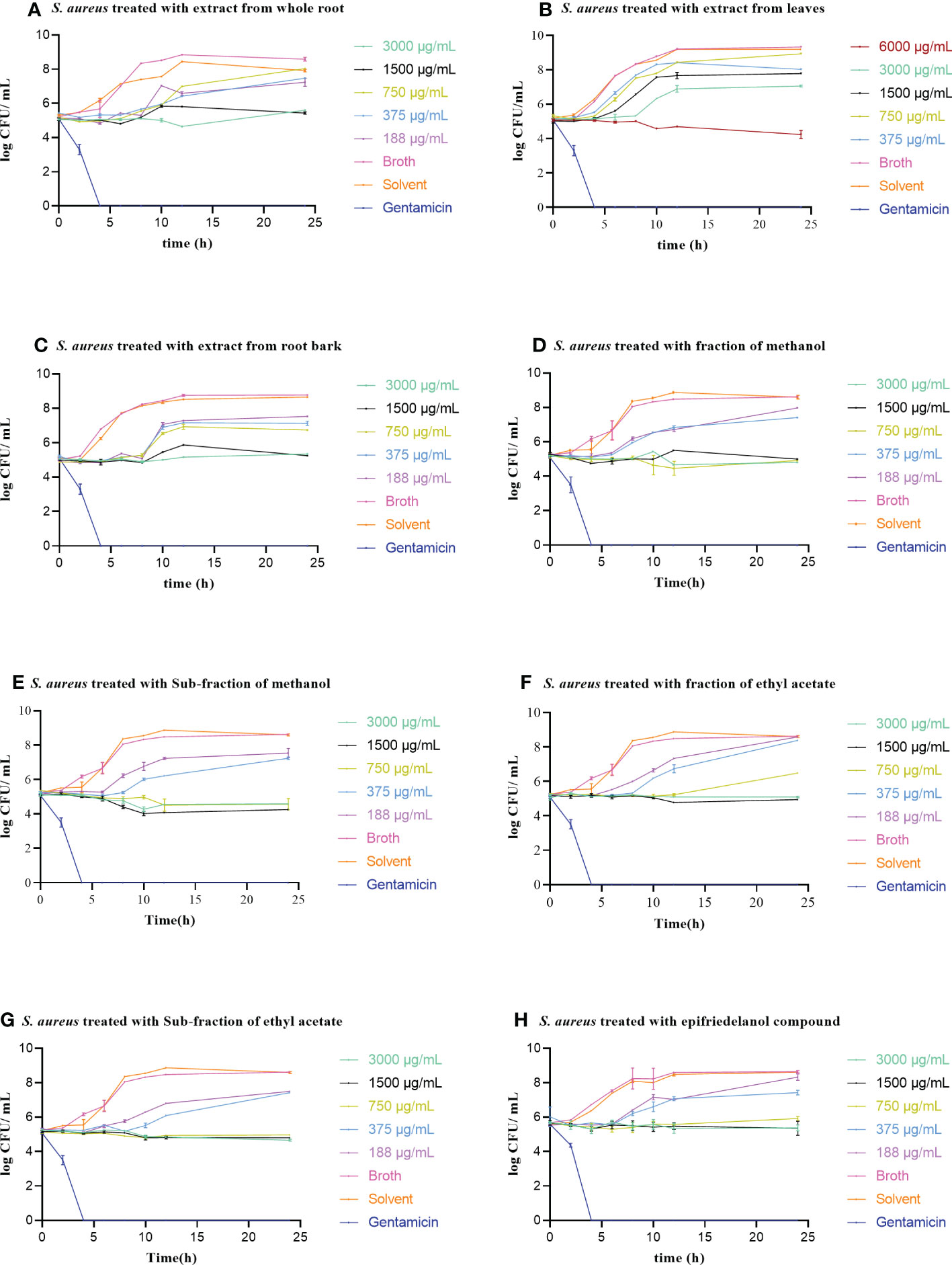
Figure 3 (A-H) Time-kill curves of S. aureus (ATCC25213) treated with increasing concentrations of extracts of S. glaucescens. Treatment with gentamicin at a concentration of 4 µg/mL was included for comparison. Error bars represent standard error of the mean of three independent experiments.
3.4. Haemolytic activity
The three active extracts (whole root, root bark, and leaves), fractions, sub-fractions and epifriedelanol tested showed haemolytic activity which was significantly above the negative control (Figures 4A-H). No haemolytic activity was observed for the solvent control. Eventhough, the haemolytic activities were above the negative control, the haemolytic activities were below 40% when compared to the positive control (H202), except for extracts from the leaves at the concentration of 500 and 1000 µg/mL (Figure 5). The percentage haemolysis indicated low haemolytic activities of these extracts, fractions, sub-fractions and the compound. Furthermore, the minimum inhibitory concentration observed in the antimicrobial activity for the extracts (whole root, root bark, and leaves), fractions, sub-fractions against S. aureus was < 100 µg/mL, such that the percentage haemolysis at this concentration for the however was less than 14.04%. In contrast, extracts of leaves showed high haemolysis activity (75.01%) at the highest concention of 1000 µg/mL. Generally, the percentage haemolysis showed concentration dependent.
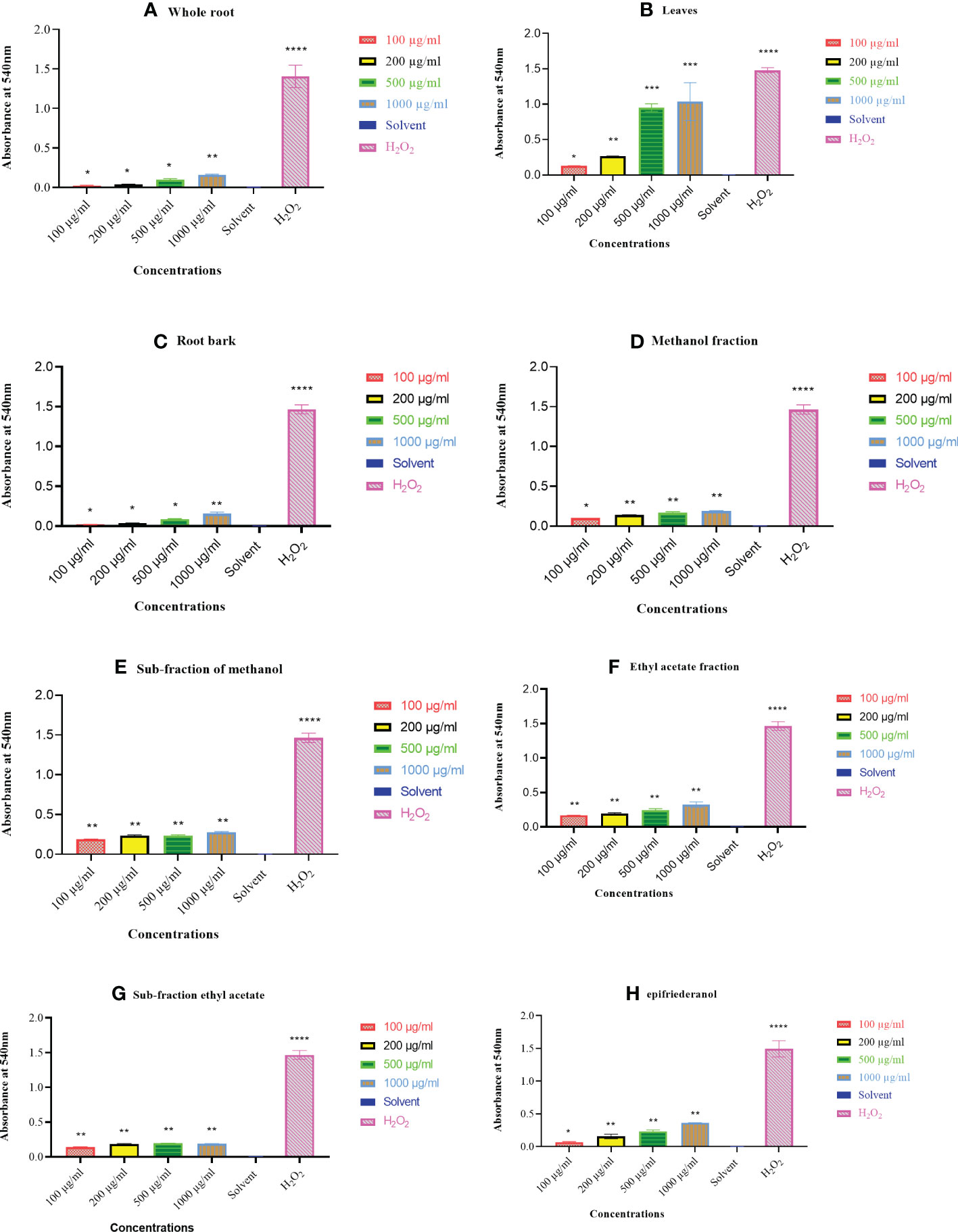
Figure 4 (A-H) Haemolytic activity of extracts, fractions, sub-fractions and epifriedelanol against RBCs as compared to the negative (DMSO) control (one way ANOVA, *P<0.05, **P<0.001, ***P<0.0001, ****P<0.00001). Error bars represent standard deviation from the mean from three independent experiments.
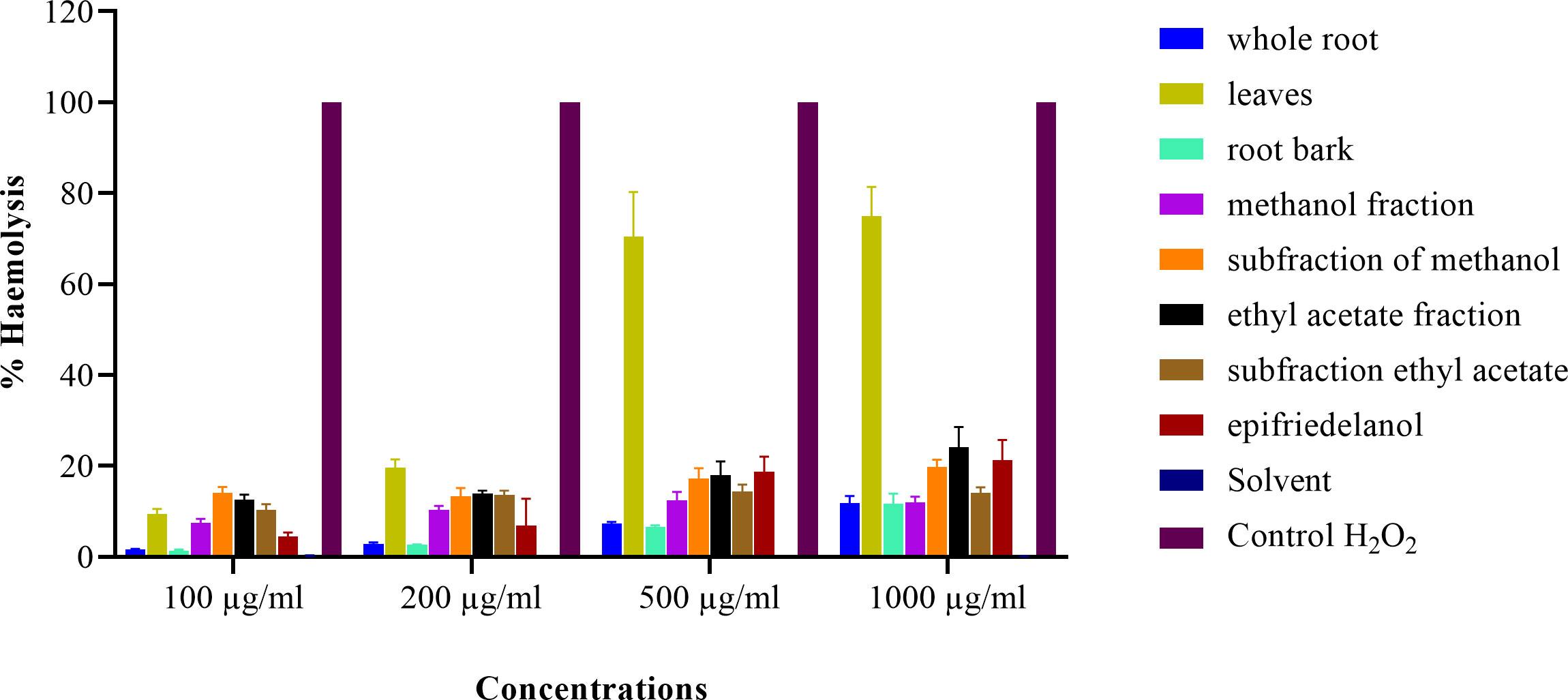
Figure 5 Percentage haemolysis of RBCs treated with whole root, leaves, root bark, methanol fraction, methanol sub-fraction, ethyl acetate fraction, sub-fraction of ethyl acetate and epifriedelanol from S. glaucescens tested in different concentrations. The haemolysis is shown in percentage with respect to positive control (H2O2), and error bars represent standard error of the mean of three independent experiments.
4. Discussion
The study investigated the antibacterial activity of crude extracts from S. glaucescens against medically important bacteria. Based on the growth inhibition curves, it was evident that cold methanol extract of whole root, root bark and leaves of S. glaucescens inhibited S. aureus at relatively low concentrations. This is in contrast with the activity against E. coli used even in higher concentration. However, from this observation we cannot conclude that, the plant parts are devoid of substances with activity against Gram-negative bacteria. Sometimes, active compound in crude extracts may be present in too low concentrations to show activity without enrichment (19, 20), or antagonistic effect may hinder the activity, for example, by binding to the same receptors as the active compound(s) (21).
In accordance with the initial indications from growth inhibition assay, on one strain of S. aureus and E. coli, the median MIC in Gram-positive was lower than Gram-negative species when testing a wider collection of bacteria. The findings of the current study thus corroborate the traditional use of this plants in treating infections caused by Gram-positive bacteria, while the current use of such extracts against infections caused by Gram-negative bacteria are not indicated. Previous report on antibacterial activity of S. glaucescens (8), showed to have activity on P. aeruginosa, though the report rely on only one bacteria tested on mice skin, and we cannot confirm this activity.
Extracts from whole root and root bark demonstrated higher activity than extracts of leaves. This suggests that a dedicated search for the active substances should concentrate on the root parts of the plants. However, from a plant conservation point of view; the selection of extracts of roots may be sub-optimal. Others studies have found that Gram-positive bacteria are more susceptible towards plants extracts than Gram-negative bacteria (22) probably due to the difference in cell wall structure between the two groups of bacteria (23). Since the pipeline for antimicrobial drugs with good activity against Gram-negative bacteria is dry (24), this is worrisome. Even though the plant extracts exhibited relatively moderate to weak activity toward Gram-negative bacteria, they may be used as a source of parent compounds which upon molecular modification (semi-synthetic) may extend the spectrum to include Gram-negative bacteria. The most well-known example of the conversion of Gram-positive only antibacterial is Penicillin G to Ampicillin, which is a broad-spectrum agent as a result of the addition of amine group (25).
Within the group of Gram-positive bacteria, activity was good against all tested species for at least one extract, suggesting either that different active substance(s) of different extracts may have cellular target in this group of bacteria, or that different extracts contain the same active substances. The order of susceptibility was S. aureus>Enterococci spp.>Streptococci spp. The findings suggest that S. glauscences can be used as a source of drug developing compound capable of combating different infectious diseases caused by such bacteria. Of the Gram-negative bacteria tested, E. coli, P. aeruginosa, and K. pneumoniae were the least susceptible to S. glaucescens, indicating that the active substances in the two plants are less suited for the treatment of diseases caused by these species. Extended Spectrum Beta-Lactamase (ESBL) variants of E. coli and carbapenase-producing P. aeruginosa are on the WHO list of bacteria for which novel antimicrobials are in particularly needed (24), hence, there is a need to search for more active substances to complement current antimicrobials with active substances from plants.
The antibacterial activity of fractions/sub-fractions and epifriedelanol (1) had approximately similar potency against S. aureus strains as the crude extracts. This similarity possibly indicate that, the major bioactive compound in root bark extract, its fractions and sub-fractions is epifriedelanol (1) and it indicates that, there is no contribution from other compounds to its activity. The strong activity of this compound concide with the previous work done by (26, 27) that reported a comparable activity for related friedelanol compound against S. aureus. The potency of epifriedelanol (1) against S. aureus was almost equivalent to the potency of gentamicin, indicating a high potential for use of this compound against S. aureus, and it was noteworthy that the activity was equally good against MRSA as against the non-MRSA strains tested. The weak activity demonstrated by compounds (2-10), is related to previous reported findings on related compounds (28, 29).
Time kill kinetics defines time and concentration dependency of the activity of an antimicrobial agent. The time kill profile showed that extracts, fractions, sub-fractions and the active compound (epifriedelanol) of S. glaucescens exerted a bacteriostatic effect on S. aureus as it did not allow the growth of bacteria, but on the other hand did not cause reduction in CFU. The inhibition mechanism of bacteriostatic agents function by inhibition of bacterial protein synthesis or metabolic pathways (30). However, it can be difficult in practice to distinguish between a bactericidal and bacteriostatic antimicrobial agent, because in some cases, the bacteriostatic agent may become bactericidal at higher concentrations (31).
Haemolytic activity is commonly used as initial assessment of membrane toxicity. The findings indicated that extracts, fractions, sub-fractions and epifriedelanol had low haemolytic effect at the relevant concentrations as compared to positive control. Even though the effect was significantly higher over the negative control. Extacts with <40% haemolytic activity have been considered to have no clinical significance in other works (18). Contrary to the root extracts, extracts from leaves demostrated >40% haemolysis at high concentrations. Consequently, the most active parts of the plant (root bark) and the isolated active compound paradoxically had the lowest haemolytic effect, supporting their potential for use as antimicrobial drugs.
In conclusion, this study demonstrated a strong activity of extracts of S. glaucescens, as well as fractions, sub-fractions and the compound epifriedelanol purified from sub-fractions against against Gram-positive bacteria, while the activity against Gram-negative bacteria was weak. In general, the extracts from the root presented the highest activity over other tested extracts. The time kill curves indicated that the effect of against S. aureus was bacteriostatic. Moreover, the roots parts of the plant showed to have low haemolytic effect. The present article showed that, S. glaucescens contained an antibacterial compound that can be useful for the development of novel antibacterial drugs.
Data availability statement
The original contributions presented in the study are included in the article/Supplementary Material. Further inquiries can be directed to the corresponding author.
Author contributions
ZM contributed on plant collection, extraction, biological experiments, data analysis, wrote and reviewed the manuscript. FR and DC provided the fractions and compounds. MM assisted in data analysis. JM assisted in biological experiments. FM, BJM, MMM, LK, RM, and JO provided professional advice, reviewed and edited the manuscript, provided supervision and solicited the funding for the research. All authors contributed to the article and approved the submitted version.
Funding
This work was supported by the Danish Development Agency (DANIDA) through the Green Resource Products for Livelihood Improvement (GRILI) Project; (DFC file no. 18-3-TAN).
Acknowledgments
The authors acknowledge the DANIDA-GRILI ptoject, Danish Fellowship Centre, Sokoine University of Agriculture in collaboration with the University of Copenhagen and Muhimbili University of Health and Allied Sciences is acknowledged. Also a botanist Mr. Mbagho from Botany Department of the University of Dar es salaam is acknowledged for his assistance in plant identification and collection.
Conflict of interest
The authors declare that the research was conducted in the absence of any commercial or financial relationships that could be construed as a potential conflict of interest.
Publisher’s note
All claims expressed in this article are solely those of the authors and do not necessarily represent those of their affiliated organizations, or those of the publisher, the editors and the reviewers. Any product that may be evaluated in this article, or claim that may be made by its manufacturer, is not guaranteed or endorsed by the publisher.
Supplementary material
The Supplementary Material for this article can be found online at: https://www.frontiersin.org/articles/10.3389/fitd.2022.1104543/full#supplementary-material
Abbreviations
DCM, Dichloromethane; ESBL, Extended Spectrum Beta Lactamase; ATCC, American type culture collection; CFU, colony forming unit; MHA, Mueller-Hinton Agar; MIC, Minimum Inhibitory Concentration; NCCLS, The National Committee for Clinical Laboratory Standards; 95% CI, 95 percent confidence intervals; %: Percentage; IQR, Interquartile range.
References
1. Sofowora A, Ogunbodede E, Onayade A. The role and place of medicinal plants in the strategies for disease prevention. Afr J Tradit Complement Altern Med (2013) 10(5):210–29. doi: 10.4314/ajtcam.v10i5.2
2. Abegaz BM, Kinfe HH. Secondary metabolites, their structural diversity, bioactivity, and ecological functions: An overview. Phys Sci Rev (2019) 4(6):1–30. doi: 10.1515/psr-2018-0100
3. Mabiki FP, Mdegela RH, Mosha RD, Magadula JJ. In ovo antiviral activity of Synadenium glaucescens (pax) crude extracts on Newcastle disease virus. J Med Plants Res (2013) 7(14):863–70. doi: 10.5897/JMPR12.684
4. Mabiki FP. Bioactivity potential of extracts from synadenium glaucescens pax (Euphorbiaceae) (2013).
5. Nyigo VA, Peter X, Mabiki F, Malebo HM, Mdegela RH, Fouche G. Isolation and identification of euphol and β-sitosterol from the dichloromethane extracts of Synadenium glaucescens. J Phytopharm (2016) 5(3):100–4. doi: 10.31254/phyto.2016.5302
6. Mabiki F, Magadula J, Mdegela R, Mosha R. Optimization of extraction conditions and phytochemical screening of root extract of Synadenium glaucescens pax. Int J Chem (2013) 5(4):103–12. doi: 10.5539/ijc.v5n4p103
7. Othman L, Sleiman A, Abdel-Massih RM. Antimicrobial activity of polyphenols and alkaloids in middle eastern plants. Front Microbiol (2019) 10(911). doi: 10.3389/fmicb.2019.00911
8. Max R, Mwageni C, Bakari GG. Effect of crude root extract from Synadenium glaucescens on selected bacterial infections in albino mice (Mus musculus). J Med Plants Res (2014) 8(26):915–23. doi: 10.5897/MPR2014.5468
9. Nondo RSO, Moshi MJ, Erasto P, Zofou D, Njouendou AJ, Wanji S, et al. Evaluation of the cytotoxic activity of extracts from medicinal plants used for the treatment of malaria in kagera and lindi regions, Tanzania. J Appl Pharm Sci (2015) 5(4):7–12. doi: 10.7324/JAPS.2015.50402
10. Greco I, Molchanova N, Holmedal E, Jenssen H, Hummel BD, Watts JL, et al. Correlation between hemolytic activity, cytotoxicity and systemic in vivo toxicity of synthetic antimicrobial peptides. Sci Rep (2020) 10(1):1–13. doi: 10.1038/s41598-020-69995-9
11. Ingle KP, Deshmukh AG, Padole DA, Dudhare MS, Moharil MP, Khelurkar VC. Phytochemicals: Extraction methods, identification and detection of bioactive compounds from plant extracts. J Pharmacogn Phytochem (2017) 6(1):32–6.
12. Azwanida NN. A review on the extraction methods use in medicinal plants, principle, strength and limitation. Med Aromat Plants (2015) 4(3):196. doi: 10.4172/2167-0412.1000196
13. Rwegoshora F, Mabiki F, Machumi F, Chacha M, Styrishave B, Cornett C. Isolation and toxicity evaluation of feruloyl ester and other triterpenoids from Synadenium glaucescens pax. J phytoparmarc (2022) 11(3672):347–52. doi: 10.31254/phyto.2022.11506
14. Credo D, Mabiki FP, Machumi F, Cornett C. Structural elucidation and toxicity evaluation of bioactive compounds from the leaves and stem woods of synadenium glaucescens. Pharmac Sci Res (2022) 9(2):59–66.
15. Wiegand I, Hilpert K, Hancock REW. Agar and broth dilution methods to determine the minimal inhibitory concentration (MIC) of antimicrobial substances. Nat Protoc (2008) 3(2):163–75. doi: 10.1038/nprot.2007.521
16. Aligiannis N, Kalpoutzakis E, Mitaku S, Chinou IB. Composition and antimicrobial activity of the essential oils of two Origanum species. J Agric Food Chem (2001) 49:4168–70. doi: 10.1021/jf001494m
17. NCCLS. Methods for determining bactericidal activity of antimicrobial agents; approved guideline. NCCLS docu Vol. 19. . West Valley Road, Suite 1400, Wayne, Pennsylvania 19087 USA: NCCLS (1999), ISBN: ISBN 1-56238-384-1. NCCLS document M26-A.
18. de Souza-Melo WO, Figueiredo-Júnior EC, Freire JCP, Costa BP, Lira AB, Freires IA, et al. Phytochemistry, antifungal and antioxidant activity, and cytotoxicity of Byrsonima gardneriana (A. juss) extract. Arch Oral Biol (2021) 123(January). doi: 10.1016/j.archoralbio.2020.104994
19. Taylor JLS, Rabe T, Mcgaw LJ, Jäger AK, Van Staden J. Towards the scientific validation of traditional medicinal plants. Plant Growth Regul (2001) 34(1):23–37. doi: 10.1023/A:1013310809275
20. Chanda S, Baravalia Y. Screening of some plant extracts against some skin diseases caused by oxidative stress and microorganisms. Afr J Biotechnol (2010) 9(21):3210–7.
21. Jager Anna K, Hutchings Anne. van SJ. Screening of Zulu medicinal plants for prostaglandin-synthesis inhibitors. J Ethnopharmacol (1996) 8741(96):95–100.
22. Parekh J, Chanda S. In-vitro antimicrobial activities of extracts of Launaea procumbens roxb. (Labiateae), Vitis vinifera l. (Vitaceae) and Cyperus rotundus l. (Cyperaceae. Afr J BioMed Res (2006) 9(2):89–93.
23. Wu Y, Bai J, Zhong K, Huang Y, Qi H, Jiang Y GH. Antibacterial activity and membrane-disruptive mechanism of 3- p - trans -Coumaroyl-2-hydroxyquinic acid, a novel phenolic compound from pine needles of Cedrus deodara, against staphylococcus aureus. Molecules (2016) 21(8):1084. doi: 10.3390/molecules21081084
24. World Health Organization. Prioritization of pathogens to guide discovery, research and development of new antibiotics for drug-resistant bacterial infections, including tuberculosis. World Health Organization (2017). https://apps.who.int/iris/handle/10665/311820.
25. Richter MF, Hergenrother PJ. The challenge of converting gram-positive-only compounds into broad-spectrum antibiotics. Ann N Y Acad Sci (2019) 1435(1):18–38. doi: 10.1111/nyas.13598
26. Tamokou JDD, Tala MF, Wabo HK, Kuiate JR, Tane P. Antimicrobial activities of methanol extract and compounds from stem bark of vismia rubescens. J Ethnopharmacol (2009) 124(3):571–5. doi: 10.1016/j.jep.2009.04.062
27. Kemboi D, Langat MK, Siwe-Noundou X, Krause RWM, Isaacs ML, Tembu VJ. In vitro antibacterial and cytotoxic effects of Euphorbia grandicornis blanc chemical constituents. BMC Complement Med Ther (2022) 22(1):1–10. doi: 10.1186/s12906-022-03571-8
28. Voukeng IK, Nganou BK, Sandjo LP, Celik I, Veronique P, Tane P, et al. Antibacterial activities of the methanol extract, fractions and compounds from Elaeophorbia drupifera (Thonn.). BMC Complement Altern Med (2017) 17(28):1–9. doi: 10.1186/s12906-016-1509-y
29. Kitahara T, Koyama N, Matsuda J, Aoyama Y, Hirakata Y, Kamihira S, et al. Antimicrobial activity of saturated fatty acids and fatty amines against methicillin-resistant staphylococcus aureus. Biol Pharm Bull (2004) 27(9):1321–6. doi: 10.1248/bpb.27.1321
30. Ullah H, Ali S. Classification of anti-bacterial agents and their functions. In: Antibacterial agents (2017).
Keywords: medicinal plants, bacteriostatic activity, haemolytic activity, infectious diseases, extracts
Citation: Msengwa Z, Rwegoshora F, David C, Mwesongo J, Mafuru M, Mabiki FP, Mwang’onde BJ, Mtambo MM, Kusiluka LJM, Mdegela RH and Olsen JE (2023) Epifriedelanol is the key compound to antibacterial effects of extracts of Synadenium glaucescens (Pax) against medically important bacteria. Front. Trop. Dis 3:1104543. doi: 10.3389/fitd.2022.1104543
Received: 21 November 2022; Accepted: 29 December 2022;
Published: 19 January 2023.
Edited by:
Victorien Tamègnon Dougnon, University of Abomey-Calavi, BeninReviewed by:
Cynthia A. Danquah, Kwame Nkrumah University of Science and Technology, GhanaYaw Duah Boakye, Kwame Nkrumah University of Science and Technology, Ghana
Copyright © 2023 Msengwa, Rwegoshora, David, Mwesongo, Mafuru, Mabiki, Mwang’onde, Mtambo, Kusiluka, Mdegela and Olsen. This is an open-access article distributed under the terms of the Creative Commons Attribution License (CC BY). The use, distribution or reproduction in other forums is permitted, provided the original author(s) and the copyright owner(s) are credited and that the original publication in this journal is cited, in accordance with accepted academic practice. No use, distribution or reproduction is permitted which does not comply with these terms.
*Correspondence: Zaituni Msengwa, emFpdHVuaS5tc2VuZ3dhQHN0dWRlbnQuc3VhbmV0LmFjLnR6; bXNlbmd3YV96QHlhb28uY29t