- 1International Vaccine Institute, Seoul, South Korea
- 2Swiss Tropical and Public Health Institute, Basel, Switzerland
- 3University of Basel, Basel, Switzerland
- 4Cambridge Institute of Therapeutic Immunology and Infectious Disease, University of Cambridge School of Clinical Medicine, Cambridge, United Kingdom
- 5University of Antananarivo, Antananarivo, Madagascar
- 6PAI Life Sciences Inc., Seattle, WA, United States
- 7Center for Tropical Medicine and Infectious Diseases, Texas Tech University Health Sciences Center, Lubbock, TX, United States
- 8Department of Internal Medicine, School of Medicine, Texas Tech University Health Sciences Center, Lubbock, TX, United States
Schistosomiasis remains a neglected tropical disease of major public health concern with high levels of morbidity in various parts of the world. Although considerable efforts in implementing mass drug administration programs utilizing praziquantel have been deployed, schistosomiasis is still not contained. A vaccine may therefore be an essential part of multifaceted prevention control efforts. In the 1990s, a joint United Nations committee promoting parasite vaccines shortlisted promising candidates including for schistosomiasis discussed below. After examining the complexity of immune responses in human hosts infected with schistosomes, we review and discuss the antigen design and preclinical and clinical development of the four leading vaccine candidates: Sm-TSP-2 in Phase 1b/2b, Sm14 in Phase 2a/2b, Sm-p80 in Phase 1 preparation, and Sh28GST in Phase 3. Our assessment of currently leading vaccine candidates revealed some methodological issues that preclude a fair comparison between candidates and the rationale to advance in clinical development. These include (1) variability in animal models - in particular non-human primate studies - and predictive values of each for protection in humans; (2) lack of consensus on the assessment of parasitological and immunological parameters; (3) absence of reliable surrogate markers of protection; (4) lack of well-designed parasitological and immunological natural history studies in the context of mass drug administration with praziquantel. The controlled human infection model - while promising and unique - requires validation against efficacy outcomes in endemic settings. Further research is also needed on the impact of advanced adjuvants targeting specific parts of the innate immune system that may induce potent, protective and durable immune responses with the ultimate goal of achieving meaningful worm reduction.
Introduction
Disease Burden
Schistosomes are digenea trematodes within the clade of platyhelminths. Human pathogenic species include Schistosoma haematobium (Sh) and S. mansoni (Sm) occurring throughout Africa and the Middle East. The former is also found in Mediterranean Europe and the latter also in South America. S. japonicum (Sj) and S. mekongi occur in Central, East and South-East Asia (1–3). Schistosomiasis is a neglected tropical disease (4) that is estimated to affect more than 240 million people (5, 6). The 2016 Global Burden of Disease study suggested that of all diseases assessed, schistosomiasis revealed the most pronounced reduction in age-standardized years lived with disability (YLD) between 2006 and 2016. Low infection intensities with minimal clinical symptoms likely underestimate the true burden of infected individuals leading to a hypothetical correction of one egg-negative case for each egg-positive case and an adjusted 400 and 600 million infected people globally (7, 8). Initial infections occur in endemic settings with typical transmission patterns among young children two years of age with increasing infection intensities during the following 10 years of life, peaking among young adolescents and decreasing during adulthood. An estimated 60-80% schoolchildren and 20-40% adults have persistent infections (9, 10). Sh-HIV co-infections, especially among women suffering from genital mucosal inflammation and ulceration, and Sm-Plasmodium spp. co-infections are considered important contributors to the HIV and malaria epidemics in Africa (11–14). Genital schistosomiasis caused by Sh is a common gynecologic condition affecting an estimated 40 million African women (15).
Parasite Pathogenicity
Definitive mammalian hosts acquire infection via skin penetration during contact with fresh water infested with infectious larval cercariae released from intermediate species-specific snail hosts. Cercariae transform to juvenile schistosomes or schistosomula before entering the vascular system, where they migrate to their venous destination to mature and mate with their sexual counterparts (16). Adult worms spend much of their lives in copula and are capable of existing in immunocompetent hosts for decades. The adult worms migrate to the mesenteric venules of the intestine, i.e. Sm and Sj, or the venous plexus of the bladder, i.e. Sh, where females residing in the gynaecophoric canal of males produce hundreds of eggs daily that are subsequently fertilized by males. Ova released via feces for Sm and Sj or urine for Sh continue their lifecycle upon hatching in fresh water as miracidia and multiplication by asexual replication in snail hosts prior to transmission to definitive hosts.
Clinical disease in humans can be divided into acute and chronic manifestations. Acute schistosomiasis is seen among individuals without previous parasitic exposure and presents as debilitating febrile illness at six to eight weeks post infection. Chronic schistosomiasis is caused by inflammatory, immunopathological host responses and organ damage due to bleeding, scarring, and formation of granulomata and fibrosis around eggs retained in tissues. Schistosomiasis clinical features are parasite species-dependent with intestinal, hepatic and urinary pathology including malignancies, e.g., squamous cell carcinomas in the bladder or sandy patches in the female genital areas. It may affect other organs such as the central nervous system through ectopic ova deposition, and lead to cognitive and physical impairment - especially among children (7, 17, 18).
Schistosomes co-exist in immunocompetent hosts for decades since they adapt, modulate, and evade cellular and humoral immune defense mechanisms though with parasite species-specific differences (9, 19). Their pivotal component for switching from a young immune-sensitive to an adult immune-refractory state is the tegument. It is a syncytial matrix of fused cells overlaid by a lipoidal membranous bilayer on the parasite outer surface, and essential for metabolic processes, movement, and external interchange (16, 20–22). Tegumental mechanisms to evade host defense components include biophysical membrane adaptations with rapid turnover, and alterations or masking of surface antigens and immunomodulatory molecules (21).
Host Immunity
Upon infection in humans, the immune system is confronted with multiple moieties exposed during the various lifecycle stages. Intact adult worms are impervious to immune attacks, whereas the migrating skin- and especially lung-stage schistosomula are targets of the cellular and humoral host defense (9, 10, 23). Generally, hosts react in the following general manner against schistosomal infections: (i) development of age-dependent partial protective immunity to reinfection from repeated adult worm death that releases immunologically active molecules that induce adaptive immunity and (ii) initiation of immunopathogenic and/or immunoregulatory mechanism against parasitic antigens released from tissue-trapped ova (7, 17, 24, 25). In endemic settings, immune responses and disease severity are influenced by multiple host factors, e.g., infection intensity, cumulative treatment history, co-infection status, genetics/genetic pre-disposition, and in utero transplacental and postnatal oral antigen sensitization resulting in phenotypically similar maternal and fetal responses (18, 26). Interestingly, newborns of mothers with schistosomiasis have a pre-existing anti-inflammatory T-helper 2 (Th2) response, IgM and/or IgE anti-schistosome antibodies, and cord blood mononuclear cells proliferating with anti-egg antibodies that induce altered regulated responses to infections in these children (10, 18, 26).
The acute disease phase is characterized by pro-inflammatory CD4+ T-helper 1 (Th1) or CD4+ Th1/T-helper 17 (Th17) responses against migrating schistosomula with elevated production of tumor necrosis factor alpha (TNF-α) and interferon gamma (IFN-γ) triggering innate phagocytic antigen-presenting and epithelial cells to release larvicidal molecules and other cytokines (18, 23, 27–30). For instance, interleukin (IL)-17-activated neutrophils release neutrophilic extracellular traps (NETs) to sequester schistosomula in the bloodstream; IL-10-regulated IL-12 is essential for Th1-cell differentiation (23, 31). Regulatory CD4+ T-cells (Treg) provide an essential regulatory arm to stabilize the immune response and limit immunopathology. Tregs and cytokine decoy receptors serve to limit the extent of immune-mediated pathology during schistosomiasis (32). However, in Sh-infected individuals Treg proportions rise significantly with increasing infection in younger age groups. In contrast, Treg were negatively correlated to infection intensity in older age groups (32, 33). The chronic disease stage is defined by anti-inflammatory CD4+ Th2 responses against egg epitopes with antigen-presenting cells, members of the B7 superfamily, and cytokines to downregulate the pro-inflammatory response (18). IL-10, which has a role in T-cell regulation with IL-4, prevents damages from Th1/Th2-mediated pathologies, and polarizes Th1/Th2 responses to enhance resistance to pathogens, and mitigate disease severity, thus improving host survival (27, 28, 34).
Extreme polarization is detrimental; the “happy valley” hypothesis assumes best anti-parasite protection at either the Th1- or the Th2-pole, and least protection at a well-mixed Th1/Th2 balance where parasites seem “happiest” (27). IL-4 activates phagocytic antigen-presenting and cytokine-producing leukocytes and interacts with other cells presenting antigens in endemic populations impacting vaccine efficacy and disease susceptibility. Whether persistent schistosomiasis affects HIV susceptibility or both agents interact is uncertain though viral replication is higher when Th2-cells are present (18). Though Th2-cells stimulate natural but only partial non-sterile resistance to reinfection, they contribute long-term to disease chronicity with granulomatous-fibrotic formations mediated by IL-4, IL-5 and IL-13, and STAT6 (signal transducer and activator of transcription 6) pathways (32).
The B-cell contribution to parasite clearance and prevention of disease severity is not well understood. IgE and IgA antibodies confer protective immunity with resistance to reinfection, and larval killing through antibody-dependent cell-mediated cytotoxicity (ADCC) along with blood effector cells (27, 35–37). However, IgE antibodies develop slowly due to long-lived schistosomes and antigen-exposure occurring only during parasitic death. In contrast, IgG4, IgG2 and IgM against parasitic carbohydrate epitopes are associated with susceptibility to reinfection and disease severity along with mediators, e.g., IFN-γ, Treg and IL-10, thus blocking protective antibodies (10, 35–37). Whether resistance to schistosomiasis is due to a balanced mix of antibodies or to the presence or absence of a particular antibody is unclear (35, 36). Vaccine-induced hypersensitivity due to pre-existing IgE from past infections are seen in other worms, e.g., for the Brazilian APS-based hookworm vaccine in its Phase I trial (38, 39).
Prevention and Treatment
Preventive measures are multifaceted and include avoiding contact with infectious water, improved access to uncontaminated water, improved hygiene and sanitation, elimination of intermediate freshwater snail hosts, and provision of health education to high-risk populations, especially in settings with dam construction, irrigation systems, and internally/externally displaced persons (refugee camps) (40, 41). The drug of choice remains praziquantel (PZQ) (42). It is efficacious against adult worms by changing irreversibly the tegumental permeability and stability but works poorly against schistosome larva (9). Its full efficacy is impacted by host factors, e.g., immune defense, infection intensity, exposure history, gut microbiota, and physiological disposition and bioavailability, and parasite factors, e.g., localization in bloodstream leading to damage or recovery (9, 19, 20, 24, 42–45). Anti-worm rather than anti-egg antibodies are reported to increase after PZQ treatment. Initially, IgG4 leads to higher susceptibility to re-infection due to IgE blocking but a decrease in susceptibility with regular repeated treatment is seen due to decreased IgG4 levels (46, 47). The immunoregulatory role of PZQ is driven by the promotion of CD4+ T-cells and differentiation of Type 1 regulatory cells to maintain immune hemostasis and effector cytokine secretion - in particular IL-4, IL-5 and IL-10 (10, 45). However, since PZQ cannot prevent reinfection, regular repeated large-scale mass drug administration (MDA) is needed in endemic settings, which requires large infrastructure and financial investment. About 105 million people received preventive treatment in 2019, though at least 237 million people were in need (1, 44). The ability of PZQ to cure adult worm infection and reduce egg excretion is important, but concern over the potential of emerging resistance has arisen because of its exclusive and extensive use over decades (7–9, 39, 42, 48, 49).
Advanced Vaccine Candidates
Given the limitations of MDA alone, a hypothesis is that complementing a comprehensive approach with a safe and effective vaccine would more likely be successful in dramatically reducing the incidence of schistosomiasis (7). Current research builds largely on the radiation-attenuated cercarial vaccine considered as gold standard in terms of immunological response in experimental models, but difficult to be translated for human use. Single percutaneous administration yields 60-70% protection against subsequent challenge infection starting about two weeks post-immunization and persisting for about 15 weeks in various animal species and for several Schistosoma species (31, 50).
Preferred product characteristics for a schistosomiasis vaccine have been proposed (7, 8). Since schistosomes do not replicate in their definitive host, a prophylactic vaccine against schistosomiasis should ultimately result in reduction of disease manifestations as well as blocking transmission, thus reducing force of transmission (7, 8). The goal is non-sterilizing immunity with a long-term decline in tissue eggs and egg excretion - preferably through killing of female worms - while preserving concomitant natural immunity induced by non-pathogenic male worms (8, 51–53). A candidate vaccine should be co-administrable with PZQ in MDA programs and with existing national vaccination programs. In the 1990s, a joint UNICEF, UNDP, World Bank, WHO-TDR program promoting vaccines against parasitic diseases shortlisted some promising candidates; only few have progressed to pre-clinical and clinical stages: Sm-TSP-2 which is in Phase 1b/2b, Sm14 in Phase 2a/2b, Sm-p80 which is being submitted to the US FDA for Phase 1 clinical trial approval, and Sh28GST which has completed Phase 3 trials (51, 54, 55). We present findings from the literature and clinical trials registered at https://clinicaltrials.gov. For each of the four leading vaccine candidates, we discuss the antigen, and preclinical and clinical studies as applicable. Details on antigens, formulations, study designs and findings are summarized in Supplementary Tables S1–S4.
S. mansoni Tetraspanin: Sm-TSP-2/Sm-TSP-2/Al®
The characteristics of tetraspanin (TSP) are summarized in Figure 1 (56–61); detailed pre-clinical and clinical investigations of Sm-TSP-2 are presented in Supplementary Table S1.
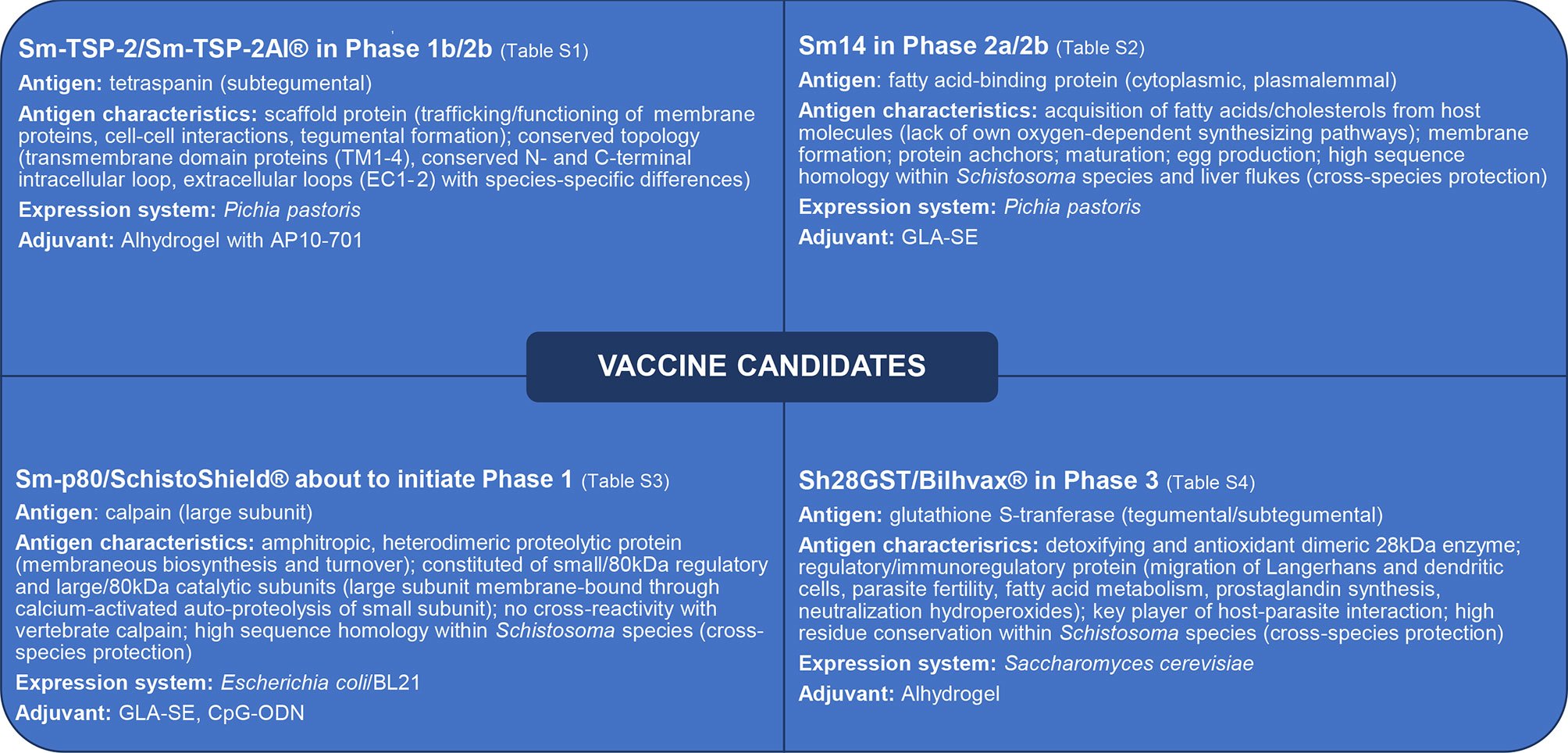
Figure 1 Antigen characteristics of advanced schistosomiasis vaccine candidates which progressed to pre-clinical and clinical development (details in Supplementary Tables S1–S4). Sm, Schistosoma mansoni; Sh, Schistosoma haematobium; TSP, tetraspanin; GST, glutathione S-transferase; cGMP, current Good Manufacturing Practice; AP10-701, glucopyranosyl lipid A (aqueous formulation); GLA-SE, glucopyranosyl lipid A in stable emulsion (TLR4 agonist); CpG-ODN, oligodeoxynucleotides with unmethylated CpG dinucleotides (TLR9 agonist).
TSPs are scaffold proteins regulating the trafficking, functioning of membrane proteins, cell-cell interactions, and tegumental formation; they share a topology of an intracellular N- and C-terminal loop, and extracellular loops EC1 and EC2 with species-specific phylogenetic differences stimulating differential protection (56–60). TSP’s species-specific genetic polymorphism - especially within the EC2 external loop - and subclass diversity impact its host range and suitability as a target of protective immunity in addition to cross-species protection (62). Gene sequences of the EC2-domain from Kenyan Sm male worms revealed the greatest degree of polymorphism found in Schistosoma spp (58). The sub-tegumental Sm-TSP-2 of newly transformed schistosomula is an easy target of protective immunity and uniquely recognized by IgG1 and IgG3 antibodies from individuals with acquired resistance to schistosomiasis compared to chronically-infected subjects. Krautz-Peterson et al. showed that a limited number of dominant conformational epitopes on five major tegumental surface membrane proteins, i.e., Sm-TSP-2, Sm23, Sm29, SmLy6B and SmLy6F, are primary targets of serum antibodies from mice, rats and humans infected with Sm. However, neither infecting schistosomula nor mature adult schistosomes are substantively impacted by the robust circulating antibodies specific to these antigens (62). By contrast, Th1 responses to these antigens can be harmful to schistosomes and are responsible for the reduction in parasite load following vaccination (63, 64).
Screening sera of individuals from endemic areas of the state of Minas Gerais, Brazil revealed higher anti-TSP-2 IgG1 and IgG3 antibodies among putatively resistant individuals, though no anti-TSP-1 antibodies were found. However, among chronically-infected individuals, all IgG subclasses and IgE were detected against soluble egg and worm antigens (65). Injection of mice with schistosomula after electroporation with Sm-TSP-1 and Sm-TSP-2 dsRNAs resulted in 61% and 83% reductions in the numbers of parasites recovered from the mesenteric veins four weeks later when compared to dsRNA-treated controls. These results imply that TSPs play important structural roles impacting tegument development, maturation, or stability (61).
TSP orthologs in Sh and Sj with >90% sequence homology indicate potential cross-species protection (66, 67). Protection based on subclass diversity with differential expression of transcripts across the parasitic lifecycle stages and higher expression in female than male worms was investigated within Sj-TSP-2. Immunizing mice with Sj-TSP-2 subclass C or recombinant Sj-TSP-2 subclasses A-G resulted in no protection (56, 66); however, administering Sj-TSP-2 subclass E or D adjuvanted with or without complete Freund’s adjuvant (CFA) or incomplete Freund’s adjuvant (IFA) resulted in reductions of 54% hepatic and 69% fecal eggs, and 53% hepatic and 52% fecal eggs, respectively (56, 66, 68). Strong total IgG (IgG1 and IgG2a) antibody responses with weak IgA, IgE and IgM levels were detected, indicating an overall marked Th1/Th2 response (65).
Variations in the Sj-TSP-2 EC2 sequence may alter the affinity or avidity to hosts’ immune responses and stimulate different levels of protective efficacy. Experiments in mice with Sm-TSP-2 encoding EC2 or Sm-TSP-2/5B chimera (hookworm vaccine candidate) co-administered with CpG/alum reduced worm burden by 25-27% and 54-58%, and liver eggs by 20-27% and 48-56%, respectively, and induced higher titers of total IgG, IgG1 and IgG2 antibodies in the Sm-TSP-2/5B group (65, 69). Analysis of sera of chronically-infected individuals from Sm and hookworm endemic Minas Gerais, Brazil, did not reveal Sm-TSP-2-specific (and Na-APR-1/5B) IgE antibodies despite high IgE titers to crude schistosome soluble egg antigen.
Additional pre-clinical experiments combining Sm-TSP-2 with Sm29 adjuvanted with CFA/ICA conferred increased protective efficacy (63). Similar to the aforementioned studies, individuals from endemic areas in Brazil showed higher IgG against Sm-TSP-2/Sm29 in naturally-resistant individuals, suggesting that a multivalent vaccine might be better recognized by individuals with a resistant phenotype (63). Murine experiments with Sm-TSP-2/Sm29 vaccination resulted in higher reductions of worm burden and granulomata, with elicitation of total IgG, IgG1 and IgG2a antibodies with IFN-γ and TNFα cytokine production across groups, and a Th1 response with increasing antibody titers following additional booster immunizations (63). Mice immunized with DNA-based Sm29, Sm-TSP-2, Sm29 N/C-terminus/Sm-TSP-2 chimera or Sm29+Sm-TSP-2 had worm reductions of 17-22%, 22%, 31-32% or 24-32% and decreases in hepatic granuloma of 28%, 30%, 37% and 26% respectively (70). High total IgG to N/C-terminus/SmTSP-2 chimera and Sm29+rSm-TSP-2 along with high production of IFN-γ and TNF-α -indicative of a Th1-biased profile - were detected following cercarial challenge. DNA-based immunization resulted in a lower protection rate compared to recombinant protein formulations. A chimeric Sm multi-epitope (Sm14, Sm21.7, Sm23, Sm29, Smp80, Smcb, and Sm-tsp-2), subunit vaccine formulated with a novel TLR4 agonist induced humoral and cellular immune responses suggestive of its potential as a prophylactic or therapeutic vaccine (71). Taken together, these findings justified taking Sm-TSP-2 (made in Pichia pastoris) forward in clinical trials.
Sm-TSP-2/Alhydrogel (Sm-TSP-2/Al®) with or without Glucopyranosyl Lipid Adjuvant in an Aqueous Formulation (“GLA-AF”) was first tested in a Phase 1 dose-escalation trial among healthy adults from a Sm non-endemic area (NCT02337855) (49, 72). The vaccine was well tolerated with only mild local and systemic reactogenicity and no vaccine-related serious adverse events reported. The proportion of vaccinees responding 14 days post vaccination was 30%, 50%, and 89% for 10µg, 30µg and 100µg antigen doses, respectively, suggesting a dose-dependent response. IgG antibodies peaked at day 127 post vaccination in the 30μg and 100μg cohorts, but were not detectable in the 10μg cohort, and decreased by day 293 across cohorts. Magnitude and longevity of responses to Sm-TSP-2/Al® with GLA/AF (AP10-701) were assessed in a subsequent dose-escalation Phase Ib trial in healthy adults from a Sm endemic Brazilian setting (NCT03110757), but the findings are yet to be published (73). Safety, immunogenicity, efficacy and cross-species protection against Sh of Sm-TSP-2/Al® with AP10-701 are being investigated in Phase I and Phase IIb trials among healthy adults from a Sm endemic setting in Uganda (NCT03910972) (74).
S. mansoni Fatty Acid-Binding Protein: Sm14
The characteristics of FABP are provided in Figure 1 (75–79); pre-clinical and clinical investigations of Sm14 are in Supplementary Table S2.
FABPs are expressed in all parasitic life cycle stages. Cytoplasmic FABPs allow schistosomes to acquire fatty acids and cholesterol from the host due to a lack of schistosome oxygen-dependent synthetic pathways essential for membrane formation, protein anchoring, maturation, and egg production (75–79). Sm FABP shares 91%, 45%, 39% and 49% sequence homology with FABPs of Sj, Echinococcus granulosus, Fasciola hepatica, and Clonorchis sinensis, respectively; phylogenetic proximity may suggest potential for cross-species protection (80–83).
Sm14-vaccinated mice exhibited a 41% reduction in worms compared to unvaccinated controls; immunizing outbred rodents with Sm14 formulated with or without CFA reduced Sm worms by 66%-89% and Fasciola hepatica metacercariae by 100% (84–87). Administering adjuvanted Sm14 to ungulates resulted in high cross-species protection against Fasciola hepatica parasites and liver damage due to T-lymphocyte infiltration, with strong IgM and IgG responses (88–90) suggesting Sm14 as a vaccine candidate with cross-species potential (91, 92). Goats immunized with synthetic Sm14 adjuvanted with RIBI/Al(OH)3 developed cross-species cellular responses and strong humoral protection also against Fasciola hepatica with declines in liver and gallbladder worms of 46%, and a reduction of gross liver damage of 55% due to low infiltration of CD2+, CD4+ and CD8+ T lymphocytes (87–90). Early murine experiments showed worm reductions ranging from 20-67%, and strong Th1-predominant responses without impacting granulomatous-fibrotic reactions (92–100). Further rodents immunized with Sm14 with CFA or pRSET-His-rSm14, showed 72% protection and prevention of parasite maturation and hepatic tissue damage by 100% (79, 84–87, 101).
Naturally resistant individuals in endemic areas demonstrate a Th1 immune response to Sm14. Assessment of cell-mediated responses among subjects from endemic areas in Brazil using synthetic multi-epitope peptides of Sm14 (1-18, 32-48, and 53-69) indicated that Sm14 epitopes 32-48 and 53-69 were recognized by the majority of individuals tested and preferentially by resistant patients compared to non-infected, infected and susceptible subjects. A marked Th1 immune response profile corresponded with decreased granulomatous fibrotic pathology (99, 102–105). Mixtures of synthetic peptides of Sm14 and paramyosin with CFA/IFA induced a Th1/Th2 immune response with noticeable reductions of worms, intestinal eggs, and hepatic granuloma suggesting that multi-epitope-based vaccines increase the frequency of responders in genetically distinct populations. Multi-epitope and multi-valent (Sm14 and Sm29) adjuvanted vaccine candidates elicited Th2-dominant protection and a decline in granulomatous-fibrotic pathologies in different animal models (102–105). Sm14 antigens from different expression systems, e.g., Mycobacterium spp., Clostridium spp., and Salmonella spp., were of moderate efficacy (101, 106–110). Subsequent optimization of expression and fusion systems and adjuvant formulations elicited strong total IgG and IgG subclass responses with induction of cells positive for IFN-γ, TNF-α and other cytokines associated with reductions in worms, hepatic granuloma, and egg counts (92–101, 106–110). Similarly, bivalent constructs (Sm14/Sm28 GST expressed in Escherichia coli, Sm14/Sm29, and FSm14/29 adjuvanted with poly(I:C), administered in murine models resulted in declines in adult worms, hepatic and intestinal eggs, and hepatic granuloma size and frequency, and elicitation of Th1 immune responses (87, 103–106, 110).
Sm14 expressed in Pichia pastoris and adjuvanted with GLA in a stable emulsion (GLA-SE) advanced into a safety and immunogenicity Phase 1 clinical trial among healthy adults from a non-endemic Brazilian area (NCT01154049) (111–113). The vaccine given intramuscularly at weeks 0, 4 and 8 was safe and well tolerated, and no vaccine-related serious adverse event was reported. Sm14-specific total IgG and IgG1-4, were induced from day 30 until day 90 together with Th-1 and Th-2 cytokines; no IgE were detected suggestive of reduced risk of vaccine-induced hypersensitivity. Safety and immunogenicity of Sm14/GLA-SE were assessed in a Phase 2a trial among adults with history of Sm and/or Sh infections from the hyperendemic Senegal River Basin, Senegal (114). Adults received one dose of PZQ three weeks prior to vaccination (NCT03041766) (113, 114). Preliminary results suggest that Sm14/GLA-SE was safe and well tolerated and resulted in 92% seroconversion after the second booster dose. Sm14-specific antibody titers were detected up to 12 months after initial immunization (113). A Phase 2b 3-arm self-contained open-label controlled randomized trial is ongoing among healthy and Sm- and/or Sh-infected schoolchildren from the Senegal River Basin receiving a single dose of PZQ prior vaccination to further assess the safety and immunogenicity of Sm14/GLA-SE (NCT03799510) (113, 115).
S. mansoni Large-Subunit Calpain: Sm-p80/SchistoShield®
The characteristics of calpain are summarized in Figure 1 (116–125); detailed pre-clinical results using Sm-p80 are in Supplementary Table S3.
Calpain is a proteolytic protein for membranous biosynthesis consisting of a catalytic and a regulatory subunit. The large subunit, Sm-p80, changes to a membrane-bound status through calcium-activated auto-proteolysis by the small subunit (124, 125). It shows no immunological cross-reactivity with calpains in vertebrates; Sm-p80 is expressed in all schistosome lifecycle stages though with a 2.5-fold higher expression in females compared to male adult worms, and plays a pivotal role in membranous biosynthesis and turnover (116–123). Sm-p80 induced cross-species protection against Sh with reductions of 48% worms, and 66% and 63% hepatic and intestine eggs in Syrian hamsters, respectively, and reductions of 25% worms, and 64% urinary bladder, 40% fecal and 53% urinary eggs in Papio anubis baboons with robust immune responses indicative of balanced Th1/Th17 immunity though slightly biased to a Th2 response (125, 126). Cross-species protection was also shown against Sj using GLA-SE-adjuvanted Sm-p80, and against Sh using a Sm-p80-VR1020 DNA prime and CpG-ODN-adjuvanted protein boost approach with reductions of 47% of worms and 5% of hepatic eggs in mice, and reductions of 27% worms but no effects on hepatic, intestinal and urinary eggs in hamsters, respectively, and a balanced Th1/Th2 immune response (122, 126).
Sm-p80 expressed in baculovirus and adjuvanted with CFA resulted in 67% worm reduction in immunized mice challenged with Sm (124). Neither subcutaneous nor intranasal delivery of recombinant vaccinia virus-expressed protein-based Sm-p80 decreased adult worms, while DNA immunization via gene gun provided 60% protection with a Th1-based immune response against cercarial challenge (127). Sm-p80 DNA administered intramuscularly with IL-2 or IL-12 to mice at weeks 0, 4 and 8 followed by cercarial challenge resulted in 57% and 45% decreases in adult worms, respectively. Total IgG, IgG2a and IgG2b antibodies were enhanced in the presence of either interleukin indicative of a Th1 response, but no IgA, IgE and IgM antibodies were detected, which is a hallmark of Sm-p80 (52). The same DNA construct co-administered with the granulocyte-macrophage colony-stimulating factor (GM-CSF) or IL-4 conferred similar worm reductions of 44% and 42% compared to IL-2 or IL-12, respectively; Sm-p80-DNA given with GM-CSF or IL-4 resulted in the augmentation of both Th1 and Th2 responses, but no IgA, IgE and IgM antibodies (116). Subcutaneous immunization of mice with Sm-p80 DNA alone or adjuvanted with GM-CSF, IL-4, IL-12, or IL-2 followed by Sm cercarial challenge confirmed protective effects with decreased worm burden as in earlier experiments. Mouse splenocytes showed high in vitro proliferation indicative of a protective Th1 immune response (128). Increasing the frequency of DNA boosts resulted in 59% and 84% decrease of worm and egg burdens, respectively, with distinct total IgG, IgG1, IgG2a, IgG2b, IgG3 titers mediated by IFN-γ and IL-2 even without added adjuvants (129).
Vaccinating mice in a Sm-p80 DNA prime and protein boost versus Sm-p80 alone co-administered with Resiquimod (R848, TLR7/8 agonist) resulted in 49% and 50% worm as well as 30% and 16% egg reductions, respectively. In contrast, the same prime-boost regimen versus recombinant protein alone co-administered with CpG-ODN conferred decreases of 57% and 70% in adult worms, and 71% (65% anti-fecundity) and 75% (77% anti-fecundity) reduction in egg counts, respectively (30). The use of R848 resulted in high titers of IgM, IgA, total IgG and IgG1, IgG2a, IgG2b, and IgG3 together with pro-inflammatory cytokines in addition to IL-15 and IL-16 indicating a Th1 type immune response (130) - similar to the immune response seen in the CpG-ODN experiment though titers were higher with Sm-p80 alone. Both approaches elicited strong mixed Th1/Th17 and Th2 biased cellular responses (30).
Papio anubis baboons which develop a schistosomiasis syndrome similar to humans and are natural hosts for various Schistosoma species were vaccinated intramuscularly with 500μg Sm-p80 DNA alone or IL-2-adjuvanted followed by three boosts at 4-week intervals (8, 131). Immunized animals exhibited a Th1 response with 21-34% complement-dependent killing of schistosomula. A proof-of-concept study in non-human primates assessed the safety and prophylactic anti-fecundity potential of non-adjuvanted DNA immunization followed by a subcutaneous challenge with 1,000 Sm cercariae. The vaccine was well tolerated and showed 38% reduction in worms (30% males and 14% females), 50% reduction of paired worms, 6% reduction in immature worms, and 32% reduction hepatic and intestinal eggs, and a mixed Th1/Th2 response with Th1 dominance (118, 132–134).
Sm-p80 DNA cloned in vector VR1020 administered to mice resulted in a decrease in 47% adult worm and high total IgG and IgG2b titers following cercarial challenge indicative of a Th1/Th17 immune response (118). Baboons immunized with Sm-p80-VR1020 showed similar reductions in adult worms of 46%, and of hepatic and intestinal eggs of 28%, and a mixed Th1/Th2 response (133). The co-administration of Sm-p80-VR1020 or Sm-p80 protein with alum in mice saw reductions of 61% and 55% adult worms and 23% and 21% eggs counts, respectively, coupled with a robust Th1/Th2 response with strong IgM, total IgG, IgG1, IgG2 and IgG3 levels, and upregulation of pro-/anti-inflammatory cytokines affecting Th17 and Treg functions (134). Immunization of non-human primates with Sm-p80-VR1020 followed by two boosts 4 weeks apart with Sm-p80 protein adjuvanted with CpG-ODN or R848 resulted in adult worm declines of 47% and 38% versus 58% and 52% with protein alone, respectively. Total IgG, IgG1, IgG2 and IgA antibodies coupled with IFN-γ and IL-2 expression in peripheral blood mononuclear cells, splenocytes and lymph node cells, but no IgG3 and IgG4 were elicited in either group, indicative of a mixed/balanced Th1/Th17 and Th2 response (39).
Human correlate studies revealed Sm-p80 reactivity with immunoglobulin G in human serum samples from schistosome-infected individuals. In addition, a complete lack of prevailing Sm-p80–specific immunoglobulin E in high-risk or infected populations was observed, thus minimizing the risk of hypersensitivity reaction following vaccination with Sm-p80 in humans (39). This study provided the proof-of-concept to move Sm-p80 forward into further translational development leading to human clinical trials.
In order to dissect the role(s) of antibodies in Sm-p80 mediated protection, pooled sera from mice immunized with Sm-p80-DNA or purified IgG from baboons immunized with Sm-p80-DNA were transferred intravenously into naïve mice, respectively, prior to challenge with cercariae. Passive transfer of sera or purified IgG antibodies from mice and baboons vaccinated with Sm-p80 DNA into naïve mice with subsequent cercarial challenge resulted in decreases in adult worms, hepatic eggs and egg hatching from tissues, suggesting that antibodies play a significant role in Sm-p80-mediated protection (135). Sera from immunized baboons were able to kill a significant proportion of schistosomula in a complement-dependent manner (135, 136). Naïve versus antibody gene locus knockout mice immunized with Sm-p80 with CpG-ODN showed reductions of 63% and 18% adult worms, and 47% and 36% eggs, respectively; this decrease in protection demonstrates that antibodies in addition to complement are an essential component -but not the only one - of Sm-p80-mediated protection. Immunization of wild-type and complement-3-deficient mice with Sm-p80 with CpG-ODN showed that complement plays a minimal protective role in vitro though it may impact the development of egg-induced pathology. Worms were reduced by 53% and 34%, respectively, while 15 to 25-fold lower levels of trapped hepatic and intestinal tissue eggs were seen in complement-3-deficient than wild-type mice (119). ADCC protection using lung lavage and lung cells was assessed in mice vaccinated with Sm-p80 with CpG-ODN (137). High levels of larvicidal killing and cellular attachments of macrophages, lymphocytes, and endothelial cells activated by cytokines were found suggesting that at least in mice lung cells play pivotal roles in Sm-p80-mediated immunity to schistosomiasis. Sm-p80-specific IgG antibodies were detected up to 60 weeks in mice and 5-8 years in baboons, including maternal co-protection through placental transfer, colostrum or lactation after administration of Sm-p80 with GLA-SE and Sm-p80-DNA with IL-2, respectively (137, 138).
Immunization of chronically infected baboons with Sm-p80 adjuvanted with GLA-SE resulted in declines of 36% adult worms, 54% tissue eggs, and 33% fecal eggs; immunizing baboons with a Sm-p80-VR1020 DNA prime and Sm-p80 protein boost adjuvanted with alum or CpG-ODN led to reductions of 10% worms, 10% tissue eggs and 15% fecal eggs, and of 23% worms, 57% tissue eggs and 13% fecal eggs, respectively (139). Total IgG and IgM were detected in all groups, but the expression of IgA and IgG subclasses, and cytokines differed; balanced Th1/Th17 and weak Th2 profiles were potent to kill established worms, and to reduce egg retention and expulsion (133, 139, 140). Immunofluorescence studies on Sm-p80 show it is highly expressed on eggs while still in the uterus of females, which may suggest that eggs may be damaged by Sm-p80-specific antibodies through tegument permeation. Correspondingly, infected baboons immunized with Sm-p80 with GLA-SE had a 68% reduction in liver eggs with 86% decline in the hatching rate (141). Following vaccination of baboons with Sm-p80 with GLA-SE, egg levels were reduced in liver (91%), small intestine (87%), and large intestine (91%). Female adult worms and egg hatching into miracidia decreased by 93% and 82%, respectively (123, 126). The retention of some non-pathogenic male worms of stunted growth and shorter life spans due to lack of pairing in the absence of female worms may be of benefit in recurrent boosting of the natural immunity and resistance to schistosomiasis. Livers had soft, smooth texture with fewer, smaller granulomas and necrosis surrounding trapped eggs compared to control animals (123).
Sm-p80-based vaccine efficacy was evaluated in a baboon model of infection and disease. The efficacy study aimed to replicate the scenario of implementing Sm-p80 in endemic settings subsequent to drug treatment of infected individuals. Sm-p80-based vaccination reduced hepatic- (38%), small intestine- (72.2%) and large intestine-egg burdens (49.4%). Sm-p80 also reduced hatching rates by 60.4%, 48.6%, and 82.3%, respectively (142). Observed declines in egg maturation and hatching rates were supported by immunofluorescence and confocal microscopy revealing unique differences in Sm-p80 expression in worms of both sexes and matured eggs. Immunizing baboons resulted in 64.5% reduction of the urine schistosome circulating anodic antigen, a parameter that reflects worm numbers and health status of infected hosts. Total IgG titers were unchanged during trickle infection but increased following PZQ treatment and vaccination. Transcriptomes of peripheral blood mononuclear cells, and secondary lymphoid tissues among vaccinated baboons exhibited induction, activation and proliferation of innate and adaptive humoral and cellular elements, including memory immune responses (142). Phase 1 clinical trials are proceeding now in US adults (first-in-human) followed by dose-escalation among African adults with future age-de-escalation to children with SchistoShield® (Sm-p80 expressed in E. coli and adjuvanted using GLA-SE).
S. haematobium Glutathione S-Transferase: Sh28GST (Bilharvax®)
The characteristics of GST are summarized in Figure 1 (143–151); detailed pre-clinical and clinical studies of the candidate are provided in Supplementary Table S4.
Comparative analyses on sera from exposed individuals before and after PZQ treatment known for altering schistosome-specific immune reactions qualitatively and quantitatively were performed to identify and characterize immunogenic Sh proteins (152). These studies show that GST is involved in detoxification and antioxidant pathways (144, 151). The protein is expressed on the tegument and sub-tegument of adult schistosomes of many species and plays a pivotal role in modulating the host’s immune response during infection. Inhibition of its enzymatic activities through neutralizing antibodies following immunization may be detrimental to the parasite. As key player of host-parasite interactions 28GST is an attractive vaccine candidate (143–151). It is a dimer of two very similar monomers, each having N- and C-terminal domains (144). Comparisons of Sh28GST and Sb28GST show that 75% of all residues are fully conserved among Sm, Sj, Sh, and S. bovis (Sb), suggesting a close evolutionary relationship (145, 151).
Initial research revealed that the passive transfer of 28GST-specific IgM antibodies with blocking effect of the enzymatic activity of Sm28GST in rodents resulted in worm decline, impairments in the female fecundity, and reduced egg viability related to laying, hatching and tissue deposition, suggesting that blocking effects of enzyme activities are detrimental to parasites (153). Interestingly, though PZQ has no pharmacological effect on the functional enzymatic activities of Sh28GST, its binding to Sj26GST results in a steric inhibition and altered immune responses and transport for large ligands. Whether upregulation or mutagenesis of parasitic GST could confer resistance to PZQ is debatable but may suggest the need for additional anti-schistosomal drugs (144, 151, 152, 154, 155).
Early pre-clinical experiments of Sh-infected Papio anubis baboons and Erythrocebus patas monkeys immunized with Sm28GST followed by homologous Sm or heterologous Sh challenge, and cattle vaccinated with Sm28GST with or without muramyl-di-peptide (MDP) or Sb28GST with or without MDP followed by Sh and S. mattheei challenge, showed heterologous immunity and protection post challenge with anti-fecundity and improved organ pathology. This suggests Sm28GST may have potential as polyvalent cross-protective vaccine candidate (145–148, 156, 157). In mice, Sh28GST elicited stronger IgA, IgG and IgE antibody titers compared to Sh-infected mice (158–160). Similar immune responses with predominant IgA, IgG and IgE antibodies were detected among infected humans from highly endemic Senegalese and Kenyan settings. IgE was positively correlated with older, lightly-infected subjects and negatively correlated with younger, highly-infected Zimbabweans (158–164). Naturally Sh-exposed Zimbabwean children from an endemic area demonstrated the highest and lowest Th2-cytokines among children aged 4-9 and 10-12 years of age, respectively, prior to PZQ treatment, and the highest Th1-/Th2-/Th17-cytokine levels among children aged 4-9 years six weeks post PZQ treatment (146).
Experiments using different expression vectors, e.g., Bordetella pertussis, Mycobacterium bovis, Saccharomyces cerevisiae, and Salmonella Typhimurium, and administration routes (intranasal, intraperitoneal and oral), among rodent and monkey models induced mixed Th1/Th2 responses with dose-dependent IgG1, IgG2a, IgG2b and IgA antibodies, and ADCC (148, 149, 164–167). Wild-caught Erythrocebus patas monkeys immunized with Sh28GST with CFA/IFA but not Sh28GST with Bacillus Calmette-Guérin adjuvant developed IgG and IgA titers with homogeneous anti-pathology processes, such as acute eosinic and chronic sclerotic inflammations, compared to heterogeneous processes in the alternate cohort (148). Dose-escalation toxicological assessments of Sh28GST adjuvanted with alum induced transient local inflammatory reactions and no clinical, anatomical or physiological modifications among rodents (168).
An initial Phase 1a trial assessed the safety, tolerability and immunogenicity of 100μg Sh28GST expressed in Saccharomyces cerevisiae and adjuvanted with alum followed by two booster doses on day 28 and 150 in healthy adult Caucasians 18-30 years of age (NCT01512277). A dose-escalation open-label trial of 300μg Sh28GST in alum with a single booster dose 4 weeks later was performed (168, 169). The vaccine was safe and well tolerated with only mild vaccine-related adverse events. Th2-type Sh28GST antibodies were induced by additional booster doses in a dose-independent manner. IgG1, IgG2 and IgG3 subclass antibodies, weak IgA and IgG4 titers, and no IgE antibodies, as well as a Th-1/Th-2 cytokine expression response, were detected. Inhibition of GST enzymatic activity was seen among all vaccine recipients and enhanced with additional doses coupled with the production of IgG1 and IgG3 subclass antibodies (143, 146, 168, 170). A subsequent Phase 1b trial in non-infected children 6-10 years of age (115) from schistosomiasis endemic Saint-Louis, Senegal, confirmed the vaccine safety and tolerability and the induction of high antibody titers (115, 143, 170). Bilharvax® in combination with PZQ treatment was also safe in infected adults and children (171). These preliminary clinical findings led to the implementation of an efficacy trial.
A randomized, placebo-controlled Phase 3 trial investigated the safety, efficacy, long-term recurrences of clinical and parasitological manifestations, and immunogenicity of Bilharvax® among Sh-infected school children 6-9 years of age in the Saint-Louis region of the Senegal River Basin, with high endemicity (>60%) (171, 172). Subsequent to clearance of ongoing schistosomiasis infection with two doses of PZQ prior and post immunization at week 44, children were randomized to receive three subcutaneous injections of either Bilharvax® or Alhydrogel® alone serving as control group at weeks 0, 4, and 8 followed by a booster dose at week 52, one year after the first injection (168, 171, 172). The primary endpoint was efficacy, evaluated as delay of recurrence of urinary schistosomiasis, and defined by a microhematuria associated with at least one living Sh egg from baseline to week 152. Bilharvax® combined with PZQ was safe and well tolerated. No disease recurrence was observed among vaccinees during a median follow-up period of 76 and 92 weeks and 76 weeks among the vaccine and the control groups, respectively. However, at week 152, at least one recurrence of urinary schistosomiasis without differences in morbidity and parasitological manifestations assessed by ultrasonography was experienced by 86% and 85% of recipients in each group. Sh28GST-specific total IgG, IgG1, IgG2, IgG4 and modest IgE antibodies were elevated in the vaccine group, but unexpectedly IgG3 and IgA titers were absent in both groups. The immune response generated inhibited the enzymatic activity of Sh28GST preventing schistosomiasis pathology; ≥70% of sera in the vaccine group compared to 8% in controls had antibodies. The absence of specific IgG3 antibodies in the vaccine group, and the low IgA and IgE levels might represent a key factor involved in the lack of efficacy of the vaccine in this trial. Indeed, previous studies have shown elevated Sh28GST IgG3, IgE, and IgA antibodies compared to IgG1 in association with acquired immunity against reinfection to urinary schistosomiasis (173). Immuno-epidemiological studies in human populations indicate that the presence of IgG3 antibodies correlates with naturally acquired protective immunity against schistosomiasis (174). A Phase 1 clinical trial conducted in healthy subjects (168) revealed that Sh28GST induced IgG1 and high IgG3 titers, an effect that was associated previously with reduced egg production and decreased urinary tract pathology among Sh-infected individuals. Future trials should thus reassess Bilharvax® in the absence of PZQ, and preferably in non-infected younger children utilizing another pro-Th1 adjuvant.
Methodological Considerations
Animal Models and Predictive Value of Protection in Humans
Among the experiments presented within the scope of this review, there are notable differences between the animal models, and parasitological and immunological assessments used - in particular for non-human primate challenge studies. These differences render comparisons between vaccine candidates and experimental outcomes difficult. For example, the Sh28GST candidate failed to confer efficacy in Phase 3 trials in humans while protection was demonstrated in the Erythrocebus patas monkey model, which in this case showed no positive predictive value. How would the Papio anubis baboons challenge model used for Sm-p80 compare to the Erythrocebus patas monkey model in terms of species susceptibility, cercarial challenge method, and immunogenicity assessment?
With the exception of a few antigens, the prophylactic efficacy of the majority of schistosome vaccine candidates has been evaluated only in the murine model which, inherently, appears to have an apparent ceiling of 40-50% protection (175). It is therefore advisable that while designing immunization regimens for clinical trials, data generated in the murine model should be used with caution. Baboons may serve as a useful bridge between mouse and human studies (8).
It is also noteworthy that two out of three vaccines candidates that progressed to advanced clinical development were not tested in non-human primate challenge models. The Sm14 vaccine entering into Phase 2 has not been tested in non-human primates so far. It is not clear whether Sm14 will undergo a challenge model experiment before entering an efficacy trial. Sm-TSP-2 vaccine was also not tested in non-human primates and it does not appear that this is being considered in preparation of a Phase 2b trial in Uganda.
Parasitological Assessments
Across the evaluation of different schistosomiasis vaccines, there does not seem to be a general consensus about parameters to assess and the methods of measurement for non-human primate challenge experiments and efficacy trials in humans.
Parasitological post-challenge outcomes as primary efficacy endpoints are also difficult to interpret. In efficacy trials, it is assumed that egg output and hatching percentage would be a surrogate of worm burden to assess efficacy endpoints. The determination of worm burden is only possible in non-human primate models after portal perfusion. However, fecal egg output and/or circulating antigen levels are surrogate markers in humans. The sensitivity of these surrogates, the correlation between worm burden and egg output, and the validation of these surrogates in humans need further research.
In addition, the duration of protection is unknown since all baboons in the reported trials were sacrificed. In a challenge study in baboons vaccinated with Sm-p80, post challenge there are still ~7% of active female worms producing eggs with little pathogenicity (123). It remains unclear how this would change after longer periods of observation or repeated exposure.
Immune Response Assessment and PZQ Impact
Across the evaluation of the different vaccine candidates, there does not seem to be consensus about the assessment of the following parameters: immune responses, including which variables to measure, and time points; in addition, consensus is needed on the method of measurement for non-human primate challenge experiments and efficacy trials in humans in terms of assay validation, standardization of reagents, and repository. As highlighted in the Krautz-Peterson’s study (62), using conformationally-native immunogens may be important when preparing antibodies for detection of schistosome tegumental antigens under natural conditions, such as for in situ localization or live worm staining.
PZQ may modulate transient and long-term immunological effects, which in turn may augment or antagonize vaccine-induced immune responses and skew towards a more pro-inflammatory response (47, 146, 176). In Zhang’s challenge study (123), the Sm-p80 vaccine exhibited potent prophylactic efficacy against transmission of Sm infection and was associated with significantly less egg-induced pathology compared to unvaccinated control animals. Specifically, the vaccine resulted in a 93.5% reduction female worms and impacted significantly the major clinical manifestations of hepatic and intestinal schistosomiasis by reducing the tissue egg-load by 90%. A 35-fold decrease in fecal egg excretion in vaccinated animals combined with an 81.5% reduction of egg hatching into the snail-infective stage (miracidia) demonstrates the parasite transmission-blocking potential of the vaccine. Higher Sm-p80 expression in female worms and Sm-p80-specific antibodies in vaccinated baboons appear to play an important role in vaccine-mediated protection. The immune correlates of protection are, however, not known (potential Th1 response with IFN-γ, IgG1 and IgG3)?. It must be noted that Ahmad’s work in non-human primates describes a mixed Th1-Th2 response without IgG3 antibodies. Little was learnt on immune correlates of protection however. Cell-mediated immune responses were not assessed.
Vaccination neither induces IgG4 nor a pro-inflammatory response after PZQ treatment and cercarial challenge. However, the reduction of worms and eggs after PZQ before challenge was not assessed (142). A clearer picture of these parasitological and immunological parameters might be generated from the comparison of the following groups: group 1 chronic infection, group 2 chronic infection receiving PZQ, group 3 chronic infection receiving PZQ with subsequent cercarial challenge, and group 4 chronic infection receiving PZQ followed by Sm-p80 vaccination and cercarial challenge.
To better understand field conditions of prior infection with schistosomes and post treatment with PZQ, baboons underwent Sm cercariae trickle infections over five weeks allowing the development of chronic disease and were treated subsequently with PZQ. After initial infection and during chronic disease, there was an increase in NK and NKT cells while the CD4:CD8 T-cell ratio inverted from 2:1 to 1:2.5. The cytokine expression in peripheral blood mononuclear cells after trickle infections was polarized more towards a Th2 response with a gradual increase in the Th1 profile in the chronic disease stage. Following PZQ treatment, immune cell populations reverted back towards naïve pre-treatment levels with the exception of an increase in B cells; however, expression of Th1, Th2, and Th17 cytokines was significantly increased. The implications of such findings for vaccine studies in baboons and humans remain unclear (177).
Natural history studies in areas targeted for efficacy trials seem essential, in particular in the context of PZQ MDA. Using well-established Brazilian cohorts of putative resistant and chronically-infected individuals stratified by the intensity of their Sm infection, arrays for IgG subclass and IgE responses were probed to these antigens to detect antibody signatures that were reflective of protective vs. non-protective immune responses. Moreover, probing for IgE responses allowed identifying antigens that might induce potentially deleterious hypersensitivity responses if used as subunit vaccines in endemic populations. Using multi-dimensional cluster analysis, the authors showed that putatively resistant individuals mounted a distinct and robust IgG1 response to a small set of newly discovered and well characterized surface tegument antigens compared to chronically infected individuals who mounted strong IgE and IgG4 responses to many antigens (178).
In another set of studies, Sm-infected individuals recruited from a schistosomiasis endemic area in Uganda, were treated with PZQ and followed up from five weeks to one-year post-treatment. Pre-treatment and five weeks post-treatment IgE, IgG1 and IgG4 levels against recombinant schistosomula antigens SmKK7, SmLy6A, SmLy6B and SmTSP7 were measured. Being male was associated with higher pre-treatment IgG1 levels to SmKK7, SmLy6a and Sm adult worms. There was no consistent association between the detectable five weeks post-treatment antibody responses against schistosomula antigens and reinfection intensity one year after PZQ treatment. Sm-infected individuals exhibited detectable antibody responses to schistosomula antigens that were affected by treatment. These findings indicate that schistosomula antigens induce highly heterogeneous antibody responses following treatment besides prior exposure as well as poly-parasitism and other co-infections. Some antigens induce an immune response and give rise to increased antibodies, while other antigens do not, and this could have implications for vaccine development (47, 179). More natural infection studies are needed to elucidate the immune responses before and after PZQ administration in geographical areas where vaccine efficacy trials are planned. Finally, although the latest non-human primate studies published (123, 142) use sophisticated immune assessments, the statistical analysis of baboon immunological data may benefit from new tools such as COMPASS, a computational framework for unbiased combinatorial polyfunctionality analysis of antigen-specific T-cell subsets, used in HIV vaccine trials (180).
Controlled Human Infection Model
Controlled human infection models (CHIM) are gaining recognition as an approach to accelerating vaccine development, for use in both non-endemic and endemic populations. CHIM could possibly guide the identification of a promising candidate vaccines for further trials and advance the understanding of protective immunity. The Sm CHIM model developed in the Netherlands (181) might be an important vaccine assessment tool providing guidance without being a gatekeeper of clinical efficacy trials. Because responses to infections and candidate vaccines are likely to differ between endemic and non-endemic settings, it has been proposed to establish a Sm-CHIM in Uganda where also Sh is endemic (182). A first dose-escalating clinical safety trial in 17 volunteers using male Sm cercariae, which do not produce eggs and therefore do not cause lasting pathology, as a challenge was recently conducted in Leiden, the Netherlands (NCT02755324). The primary endpoints were adverse events and infectivity. A dose-related increase in adverse events due to acute schistosomiasis syndrome, occurred in nine of 17 volunteers. Overall, five volunteers reported severe adverse events (SAEs). Infection with 20 Sm cercariae led to SAEs in 18% of volunteers and high infection rates. Worm-derived circulating anodic antigen, the biomarker of the primary infection endpoint, peaked in 82% of volunteers during 3 to 10 weeks post exposure. All volunteers showed IgM and IgG1 seroconversion and worm-specific cytokine production by CD4+ T cells. All volunteers were cured with PZQ provided at 12 weeks after exposure (183).
This model is not validated and its positive and negative predictive values of the efficacy outcome of vaccines in humans remain unclear. Also, it does not reflect the situation of endemic areas in terms of age of target population, repeated natural exposure, and PZQ rollout. It remains unclear what parasitological and immunological parameters would be assessed with the exception of serum circulating anodic antigen.
Conclusions
Schistosomiasis remains a neglected tropical disease of major public health concern with high levels of morbidity globally. Despite considerable efforts in MDA programs, schistosomiasis is still not contained. A schistosomiasis vaccine may be a critical component of a multifaceted prevention control approach. Four major vaccine candidates have entered advanced pre-clinical and clinical development, but only one reached Phase 3 in Africa and failed to confer meaningful efficacy. Several other promising candidates are in preclinical and early clinical development. This assessment of current candidates revealed some methodological issues that preempt a predictive comparison between leads. These include variability in animal models, in particular, non-human primate studies, and their predictive value of protection in humans, lack of consensus on essential parasitological and immunological assessment parameters and of reliable surrogate markers of protection, and the need for better designed parasitological and immunological natural history studies in the context of PZQ. Although the CHIM model is a great initiative, it needs to be validated for its positive and negative predictive values for vaccine efficacy in endemic settings and should not be considered as a gatekeeper of field trials in humans.
More research is also needed to test new potential vaccine antigens such as cholinesterases regulating the neurotransmission of Schistosoma (184), or new tegumental proteins identified by microarray and other modern technologies (178, 185–189), and more potent adjuvants targeting specific parts of the innate immune system to tailor a protective immune response for lead schistosome vaccine candidates with the long-term aim to achieve high levels of worm reduction and ultimately elimination of this terrible disease.
Author Contributions
UP, J-LE, and JK conceived, designed and analyzed the available data. UP, J-LE, and JK wrote the initial manuscript, and FM, DC, and AS reviewed it. All authors contributed to the article and approved the submitted version.
Funding
Bill and Melinda Gates Foundation [Grant # OPP1097535; INV-006395; INV-027122; INV-004809 ID52625 and Global Health Vaccine Accelerator Platform (GH-VAP)]; NIH/NIAID (2R44AI103983); European Union Horizon 2020 Program (Grant # DLV-815643); and Wellcome Trust (Grant # 218454/Z/19/Z) are acknowledged for their support of Sm-p80-based vaccine development effort.
Author Disclaimer
The findings and conclusions of this review are those of the authors and do not necessarily represent official funder or institutional positions.
Conflict of Interest
DC is an inventor of the GLA-based adjuvants GLA-AF, GLA-Alum, and GLA-SE and CEO of PAI Life Sciences Inc. which has a license to SchistoShield®. AS is an inventor of the Sm-p80 antigen.
The remaining authors declare that the research was conducted in the absence of any commercial or financial relationships that could be construed as a potential conflict of interest.
Publisher’s Note
All claims expressed in this article are solely those of the authors and do not necessarily represent those of their affiliated organizations, or those of the publisher, the editors and the reviewers. Any product that may be evaluated in this article, or claim that may be made by its manufacturer, is not guaranteed or endorsed by the publisher.
Acknowledgments
The authors would like to thank Ms. Ji Yeon Park for her invaluable bibliography assistance.
Supplementary Material
The Supplementary Material for this article can be found online at: https://www.frontiersin.org/articles/10.3389/fitd.2021.719369/full#supplementary-material
References
1. World Health Organization. Schistosomiasis: Key Facts (2019). Geneva, Switzerland. Available at: https://www.who.int/news-room/fact-sheets/detail/schistosomiasis (Accessed Last accessed on 31/05/2021).
2. French MD, Evans D, Fleming FM, Secor WE, Biritwum NK, Brooker SJ, et al. Schistosomiasis in Africa: Improving Strategies for Long-Term and Sustainable Morbidity Control. PloS Negl Trop Dis (2018) 12(6):e0006484. doi: 10.1371/journal.pntd.0006484
3. Gordon CA, Kurscheid J, Williams GM, Clements ACA, Li Y, Zhou XN, et al. Asian Schistosomiasis: Current Status and Prospects for Control Leading to Elimination. Trop Med Infect Dis (2019) 4(1). doi: 10.3390/tropicalmed4010040
4. Hotez PJ, Alvarado M, Basanez MG, Bolliger I, Bourne R, Boussinesq M, et al. The Global Burden of Disease Study 2010: Interpretation and Implications for the Neglected Tropical Diseases. PloS Negl Trop Dis (2014) 8(7):e2865. doi: 10.1371/journal.pntd.0002865
5. Collaborators, G.D.a.I.I.a.P. Global, Regional, and National Incidence, Prevalence, and Years Lived With Disability for 354 Diseases and Injuries for 195 Countries and Territories, 1990-2017: A Systematic Analysis for the Global Burden of Disease Study 2017. Lancet (2018) 392:1789–858. doi: 10.1016/S0140-6736(18)32279-7
6. Deol AK, Fleming FM, Calvo-Urbano B, Walker M, Bucumi V, Gnandou I, et al. Schistosomiasis - Assessing Progress Toward the 2020 and 2025 Global Goals. N Engl J Med (2019) 381(26):2519–28. doi: 10.1056/NEJMoa1812165
7. Molehin AJ, Rojo JU, Siddiqui SZ, Gray SA, Carter D, Siddiqui AA. Development of a Schistosomiasis Vaccine. Expert Rev Vaccines (2016) 15(5):619–27. doi: 10.1586/14760584.2016.1131127
8. Siddiqui AA, Siddiqui SZ. Sm-P80-Based Schistosomiasis Vaccine: Preparation for Human Clinical Trials. Trends Parasitol (2017) 33(3):194–201. doi: 10.1016/j.pt.2016.10.010
9. Colley DG, Bustinduy AL, Secor WE, King CH. Human Schistosomiasis. Lancet (2014) 383(9936):2253–64. doi: 10.1016/S0140-6736(13)61949-2
10. Colley DG, Secor WE. Immunology of Human Schistosomiasis. Parasite Immunol (2014) 36(8):347–57. doi: 10.1111/pim.12087
11. Kjetland EF, Hegertun IE, Baay MF, Onsrud M, Ndhlovu PD, Taylor M. Genital Schistosomiasis and its Unacknowledged Role on HIV Transmission in the STD Intervention Studies. Int J STD AIDS (2014) 25(10):705–15. doi: 10.1177/0956462414523743
12. Ndeffo Mbah ML, Kjetland EF, Atkins KE, Poolman EM, Orenstein EW, Meyers LA, et al. Cost-Effectiveness of a Community-Based Intervention for Reducing the Transmission of Schistosoma Haematobium and HIV in Africa. Proc Natl Acad Sci U S A (2013) 110(19):7952–7. doi: 10.1073/pnas.1221396110
13. Ndeffo Mbah ML, Skrip L, Greenhalgh S, Hotez P, Galvani AP. Impact of Schistosoma Mansoni on Malaria Transmission in Sub-Saharan Africa. PloS Negl Trop Dis (2014) 8(10):e3234. doi: 10.1371/journal.pntd.0003234
14. Yegorov S, Joag V, Galiwango RM, Good SV, Okech B, Kaul R. Impact of Endemic Infections on HIV Susceptibility in Sub-Saharan Africa. Trop Dis Travel Med Vaccines (2019) 5:22. doi: 10.1186/s40794-019-0097-5
15. Hotez PJ, Engels D, Gyapong M, Ducker C, Malecela MN. Female Genital Schistosomiasis. N Engl J Med (2019) 381(26):2493–5. doi: 10.1056/NEJMp1914709
16. Fonseca CT, Braz Figueiredo Carvalho G, Carvalho Alves C, de Melo TT. Schistosoma Tegument Proteins in Vaccine and Diagnosis Development: An Update. J Parasitol Res (2012) 2012:541268. doi: 10.1155/2012/541268
17. Mo AX, Agosti JM, Walson JL, Hall BF, Gordon L. Schistosomiasis Elimination Strategies and Potential Role of a Vaccine in Achieving Global Health Goals. Am J Trop Med Hyg (2014) 90(1):54–60. doi: 10.4269/ajtmh.13-0467
18. Pearce EJ, MacDonald AS. The Immunobiology of Schistosomiasis. Nat Rev Immunol (2002) 2(7):499–511. doi: 10.1038/nri843
19. Meurs L, Mbow M, Vereecken K, Menten J, Mboup S, Polman K. Epidemiology of Mixed Schistosoma Mansoni and Schistosoma Haematobium Infections in Northern Senegal. Int J Parasitol (2012) 42(3):305–11. doi: 10.1016/j.ijpara.2012.02.002
20. Skelly PJ, Alan Wilson R. Making Sense of the Schistosome Surface. Adv Parasitol (2006) 63:185–284. doi: 10.1016/S0065-308X(06)63003-0
21. Van Hellemond JJ, Retra K, Brouwers JF, van Balkom BW, Yazdanbakhsh M, Shoemaker CB, et al. Functions of the Tegument of Schistosomes: Clues From the Proteome and Lipidome. Int J Parasitol (2006) 36(6):691–9. doi: 10.1016/j.ijpara.2006.01.007
22. Loukas A, Tran M, Pearson MS. Schistosome Membrane Proteins as Vaccines. Int J Parasitol (2007) 37(3-4):257–63. doi: 10.1016/j.ijpara.2006.12.001
23. El Ridi R, Tallima H, Mahana N, Dalton JP. Innate Immunogenicity and In Vitro Protective Potential of Schistosoma Mansoni Lung Schistosomula Excretory–Secretory Candidate Vaccine Antigens. Microbes Infect (2010) 12(10):700–9. doi: 10.1016/j.micinf.2010.04.012
24. Fukushige M, Mutapi F, Woolhouse MEJ. Woolhouse, Population Level Changes in Schistosome-Specific Antibody Levels Following Chemotherapy. Parasite Immunol (2019) 41(1):e12604. doi: 10.1111/pim.12604
25. McManus DP, Bergquist R, Cai P, Ranasinghe S, Tebeje BM, You H. Schistosomiasis-From Immunopathology to Vaccines. Semin Immunopathol (2020) 42(3):355–71. doi: 10.1007/s00281-020-00789-x
26. Attallah AM, Ghanem GE, Ismail H, El Waseef AM. Placental and Oral Delivery of Schistosoma Mansoni Antigen From Infected Mothers to Their Newborns and Children. Am J Trop Med Hyg (2003) 68(6):647–51. doi: 10.4269/ajtmh.2003.68.647
27. Wynn TA, Hoffmann KF. Defining a Schistosomiasis Vaccination Strategy - Is it Really Th1 Versus Th2? Parasitol Today (2000) 16(11):497–501. doi: 10.1016/S0169-4758(00)01788-9
28. Stadecker MJ, Asahi H, Finger E, Hernandez HJ, Rutitzky LI, Sun J. The Immunobiology of Th1 Polarization in High-Pathology Schistosomiasis. Immunol Rev (2004) 20:168–79. doi: 10.1111/j.0105-2896.2004.00197.x
29. Kalantari P, Bunnell SC, Stadecker MJ. The C-Type Lectin Receptor-Driven, Th17 Cell-Mediated Severe Pathology in Schistosomiasis: Not All Immune Responses to Helminth Parasites Are Th2 Dominated. Front Immunol (2019) 10:26. doi: 10.3389/fimmu.2019.00026
30. Ahmad G, Zhang W, Torben W, Haskins C, Diggs S, Noor Z, et al. Prime-Boost and Recombinant Protein Vaccination Strategies Using Sm-P80 Protects Against Schistosoma Mansoni Infection in the Mouse Model to Levels Previously Attainable Only by the Irradiated Cercarial Vaccine. Parasitol Res (2009) 105(6):1767–77. doi: 10.1007/s00436-009-1646-z
31. Hewitson JP, Hamblin PA, Mountford AP. Immunity Induced by the Radiation-Attenuated Schistosome Vaccine. Parasite Immunol (2005) 27(7-8):271–80. doi: 10.1111/j.1365-3024.2005.00764.x
32. Wilson MS, Mentink-Kane M, Pesce JT, Ramalingam TR, Thompson R, Wynn TA. Immunopathology of Schistosomiasis. Immunol Cell Biol (2007) 85(2):148–54. doi: 10.1038/sj.icb.7100014
33. Nausch N, Midzi N, Mduluza T, Maizels RM, Mutapi F. Regulatory and Activated T Cells in Human Schistosoma Haematobium Infections. PloS One (2011) 6(2):e16860. doi: 10.1371/journal.pone.0016860
34. Fairfax K, Nascimento M, Huang SC, Everts B, Pearce EJ. Th2 Responses in Schistosomiasis. Semin Immunopathol (2012) 34(6):863–71. doi: 10.1007/s00281-012-0354-4
35. Negrao-Correa D, Fittipaldi JF, Lambertucci JR, Teixeira MM, Antunes CM, Carneiro M. Association of Schistosoma Mansoni-Specific IgG and IgE Antibody Production and Clinical Schistosomiasis Status in a Rural Area of Minas Gerais, Brazil. PloS One (2014) 9(2):e88042. doi: 10.1371/journal.pone.0088042
36. Vereecken K, Naus CW, Polman K, Scott JT, Diop M, Gryseels B, et al. Associations Between Specific Antibody Responses and Resistance to Reinfection in a Senegalese Population Recently Exposed to Schistosoma Mansoni. Trop Med Int Health (2007) 12(3):431–44. doi: 10.1111/j.1365-3156.2006.01805.x
37. Garraud O, Perraut R, Riveau G, Nutman TB. Class and Subclass Selection in Parasite-Specific Antibody Responses. Trends Parasitol (2003) 19(7):300–4. doi: 10.1016/S1471-4922(03)00139-9
38. Hotez PJ, Bethony JM, Diemert DJ, Pearson M, Loukas A. Developing Vaccines to Combat Hookworm Infection and Intestinal Schistosomiasis. Nat Rev Microbiol (2010) 8(11):814–26. doi: 10.1038/nrmicro2438
39. Ahmad G, Zhang W, Torben W, Ahrorov A, Damian RT, Wolf RF, et al. Preclinical Prophylactic Efficacy Testing of Sm-P80-Based Vaccine in a Nonhuman Primate Model of Schistosoma Mansoni Infection and Immunoglobulin G and E Responses to Sm-P80 in Human Serum Samples From an Area Where Schistosomiasis Is Endemic. J Infect Dis (2011) 204(9):1437–49. doi: 10.1093/infdis/jir545
40. Nelwan ML. Schistosomiasis: Life Cycle, Diagnosis, and Control. Curr Ther Res Clin Exp (2019) 91:5–9. doi: 10.1016/j.curtheres.2019.06.001
41. Sokolow SH, Wood CL, Jones IJ, Lafferty KD, Kuris AM, Hsieh MH, et al. To Reduce the Global Burden of Human Schistosomiasis, Use ’Old Fashioned’ Snail Control. Trends Parasitol (2018) 34(1):23–40. doi: 10.1016/j.pt.2017.10.002
42. Vale N, Gouveia MJ, Rinaldi G, Brindley PJ, Gartner F, Correia da Costa JM. Praziquantel for Schistosomiasis: Single-Drug Metabolism Revisited, Mode of Action, and Resistance. Antimicrob Agents Chemother (2017) 61(5). doi: 10.1128/AAC.02582-16
43. Schneeberger PHH, Coulibaly JT, Panic G, Daubenberger C, Gueuning M, Frey JE, et al. Investigations on the Interplays Between Schistosoma Mansoni, Praziquantel and the Gut Microbiome. Parasit Vectors (2018) 11(1):168. doi: 10.1186/s13071-018-2739-2
44. Mutapi F, Maizels R, Fenwick A, Woolhouse M. Human Schistosomiasis in the Post Mass Drug Administration Era. Lancet Infect Dis (2017) 17(2):e42–8. doi: 10.1016/S1473-3099(16)30475-3
45. Eyoh E, McCallum P, Killick J, Amanfo S, Mutapi F, Astier AL. The Anthelmintic Drug Praziquantel Promotes Human Tr1 Differentiation. Immunol Cell Biol (2019) 97(5):512–8. doi: 10.1111/imcb.12229
46. Chisango TJ, Ndlovu B, Vengesai A, Nhidza AF, Sibanda EP, Zhou D, et al. Benefits of Annual Chemotherapeutic Control of Schistosomiasis on the Development of Protective Immunity. BMC Infect Dis (2019) 19(1):219. doi: 10.1186/s12879-019-3811-z
47. Driciru E, Koopman JPR, Cose S, Siddiqui AA, Yazdanbakhsh M, Elliott AM, et al. Immunological Considerations for Schistosoma Vaccine Development: Transitioning to Endemic Settings. Front Immunol (2021) 12:635985. doi: 10.3389/fimmu.2021.635985
48. McWilliam HE, Driguez P, Piedrafita D, McManus DP, Meeusen EN. Novel Immunomic Technologies for Schistosome Vaccine Development. Parasite Immunol (2012) 34(5):276–84. doi: 10.1111/j.1365-3024.2011.01330.x
49. Keitel WA, Potter GE, Diemert D, Bethony J, El Sahly HM, Kennedy JK, et al. A Phase 1 Study of the Safety, Reactogenicity, and Immunogenicity of a Schistosoma Mansoni Vaccine With or Without Glucopyranosyl Lipid A Aqueous Formulation (GLA-AF) in Healthy Adults From a non-Endemic Area. Vaccine (2019) 37(43):6500–9. doi: 10.1016/j.vaccine.2019.08.075
50. El Ridi R, Tallima H. Why the Radiation-Attenuated Cercarial Immunization Studies Failed to Guide the Road for an Effective Schistosomiasis Vaccine: A Review. J Adv Res (2015) 6(3):255–67. doi: 10.1016/j.jare.2014.10.002
51. Merrifield M, Hotez PJ, Beaumier CM, Gillespie P, Strych U, Hayward T, et al. Advancing a Vaccine to Prevent Human Schistosomiasis. Vaccine (2016) 34(26):2988–91. doi: 10.1016/j.vaccine.2016.03.079
52. Siddiqui AA, Phillips T, Charest H, Podesta RB, Quinlin ML, Pinkston JR, et al. Enhancement of Sm-P80 (Large Subunit of Calpain) Induced Protective Immunity Against Schistosoma Mansoni Through Co-Delivery of Interleukin-2 and Interleukin-12 in a DNA Vaccine Formulation. Vaccine (2003) 21(21-22):2882–9. doi: 10.1016/S0264-410X(03)00159-2
53. Mo AX, Colley DG. Workshop Report: Schistosomiasis Vaccine Clinical Development and Product Characteristics. Vaccine (2016) 34(8):995–1001. doi: 10.1016/j.vaccine.2015.12.032
54. Anisuzzaman, Tsuji N. Schistosomiasis and Hookworm Infection in Humans: Disease Burden, Pathobiology and Anthelmintic Vaccines. Parasitol Int (2020) 75:102051. doi: 10.1016/j.parint.2020.102051
55. Hotez PJ, Bottazzi ME, Bethony J, Diemert DD. Advancing the Development of a Human Schistosomiasis Vaccine. Trends Parasitol (2019) 35(2):104–8. doi: 10.1016/j.pt.2018.10.005
56. Zhang W, Li J, Duke M, Jones MK, Kuang L, Zhang J, et al. Inconsistent Protective Efficacy and Marked Polymorphism Limits the Value of Schistosoma Japonicum Tetraspanin-2 as a Vaccine Target. PloS Negl Trop Dis (2011) 5(5):e1166. doi: 10.1371/journal.pntd.0001166
57. Curti E, Kwityn C, Zhan B, Gillespie P, Brelsford J, Deumic V, et al. Expression at a 20L Scale and Purification of the Extracellular Domain of the Schistosoma Mansoni TSP-2 Recombinant Protein: A Vaccine Candidate for Human Intestinal Schistosomiasis. Hum Vaccin Immunother (2013) 9(11):2342–50. doi: 10.4161/hv.25787
58. Cupit PM, Steinauer ML, Tonnessen BW, Eric Agola L, Kinuthia JM, Mwangi IN, et al. Polymorphism Associated With the Schistosoma Mansoni Tetraspanin-2 Gene. Int J Parasitol (2011) 41(12):1249–52. doi: 10.1016/j.ijpara.2011.07.007
59. Charrin S, Jouannet S, Boucheix C, Rubinstein E. Tetraspanins at a Glance. J Cell Sci (2014) 127(Pt 17):3641–8. doi: 10.1242/jcs.154906
60. Jia X J, Schulte L, Loukas A, Pickering D, Pearson M, Mobli M, et al. Solution Structure, Membrane Interactions, and Protein Binding Partners of the Tetraspanin Sm-TSP-2, a Vaccine Antigen From the Human Blood Fluke Schistosoma Mansoni. J Biol Chem (2014) 289(10):7151–63. doi: 10.1074/jbc.M113.531558
61. Tran MH, Freitas TC, Cooper L, Gaze S, Gatton ML, Jones MK, et al. Suppression of mRNAs Encoding Tegument Tetraspanins From Schistosoma Mansoni Results in Impaired Tegument Turnover. PloS Pathog (2010) 6(4):e1000840. doi: 10.1371/journal.ppat.1000840
62. Krautz-Peterson G, Debatis M, Tremblay JM, Oliveira SC, Da’dara AA, Skelly PJ, et al. Schistosoma Mansoni Infection of Mice, Rats and Humans Elicits a Strong Antibody Response to a Limited Number of Reduction-Sensitive Epitopes on Five Major Tegumental Membrane Proteins. PloS Negl Trop Dis (2017) 11(1):e0005306. doi: 10.1371/journal.pntd.0005306
63. Pinheiro CS, Ribeiro AP, Cardoso FC, Martins VP, Figueiredo BC, Assis NR, et al. A Multivalent Chimeric Vaccine Composed of Schistosoma Mansoni SmTSP-2 and Sm29 was Able to Induce Protection Against Infection in Mice. Parasite Immunol (2014) 36(7):303–12. doi: 10.1111/pim.12118
64. Cardoso FC, Macedo GC, Gava E, Kitten GT, Mati VL, de Melo AL, et al. Schistosoma Mansoni Tegument Protein Sm29 Is Able to Induce a Th1-Type of Immune Response and Protection Against Parasite Infection. PloS Negl Trop Dis (2008) 2(10):e308. doi: 10.1371/journal.pntd.0000308
65. Tran MH, Pearson MS, Bethony JM, Smyth DJ, Jones MK, Duke M, et al. Tetraspanins on the Surface of Schistosoma Mansoni Are Protective Antigens Against Schistosomiasis. Nat Med (2006) 12(7):835–40. doi: 10.1038/nm1430
66. Cai P, Bu L, Wang J, Wang Z, Zhong X, Wang H. Molecular Characterization of Schistosoma Japonicum Tegument Protein Tetraspanin-2: Sequence Variation and Possible Implications for Immune Evasion. Biochem Biophys Res Commun (2008) 372(1):197–202. doi: 10.1016/j.bbrc.2008.05.042
67. Rinaldi G, Okatcha TI, Popratiloff A, Ayuk MA, Suttiprapa S, Mann VH, et al. Genetic Manipulation of Schistosoma Haematobium, the Neglected Schistosome. PloS Negl Trop Dis (2011) 5(10):e1348. doi: 10.1371/journal.pntd.0001348
68. Yuan C, Fu YJ, Li J, Yue YF, Cai LL, Xiao WJ, et al. Schistosoma Japonicum: Efficient and Rapid Purification of the Tetraspanin Extracellular Loop 2, a Potential Protective Antigen Against Schistosomiasis in Mammalian. Exp Parasitol (2010) 126(4):456–61. doi: 10.1016/j.exppara.2010.05.018
69. Pearson MS, Pickering DA, McSorley HJ, Bethony JM, Tribolet L, Dougall AM, et al. Enhanced Protective Efficacy of a Chimeric Form of the Schistosomiasis Vaccine Antigen Sm-TSP-2. PloS Negl Trop Dis (2012) 6(3):e1564. doi: 10.1371/journal.pntd.0001564
70. Goncalves de Assis NR, Batistoni de Morais S, Figueiredo BC, Ricci ND, de Almeida LA, da Silva Pinheiro C, et al. DNA Vaccine Encoding the Chimeric Form of Schistosoma Mansoni Sm-TSP2 and Sm29 Confers Partial Protection Against Challenge Infection. PloS One (2015) 10(5):e0125075. doi: 10.1371/journal.pone.0125075
71. Rahmani A, Baee M, Rostamtabar M, Karkhah A, Alizadeh S, Tourani M, et al. Development of a Conserved Chimeric Vaccine Based on Helper T-Cell and CTL Epitopes for Induction of Strong Immune Response Against Schistosoma Mansoni Using Immunoinformatics Approaches. Int J Biol Macromol (2019) 141:125–36. doi: 10.1016/j.ijbiomac.2019.08.259
72. Medicine/ClinicalTrials.gov USNLo. A Phase I Study of the Safety, Reactogenicity, and Immunogenicity of Sm-TSP-2/Alhydrogel® With or Without GLA-AF for Intestinal Schistosomiasis in Healthy Adults [Nct02337855] (2017). Available at: https://clinicaltrials.gov/ct2/show/NCT02337855?term=TSP&cond=Schistosomiasis&rank=1 (Accessed Last accessed on 30/05/2021).
73. Medicine/ClinicalTrials.gov USNLo. A Phase Ib Study of the Safety, Reactogenicity, and Immunogenicity of Sm-TSP-2/Alhydrogel)(R) With or Without AP 10-701 for Intestinal Schistosomiasis in Healthy Exposed Adults [Nct03110757] (2018). Available at: https://clinicaltrials.gov/ct2/show/NCT03110757?term=TSP&cond=Schistosomiasis&rank=3 (Accessed Last accessed on 30/05/2021).
74. Medicine/ClinicalTrials.gov USNLo. Sm-TSP-2 Schistosomiasis Vaccine in Healthy Ugandan Adults [Nct03910972] (2019). Available at: https://clinicaltrials.gov/ct2/show/NCT03910972?term=TSP&cond=Schistosomiasis&rank=2 (Accessed Last accessed on 30/05/2021).
75. Moser D, Tendler M, Griffiths G, Klinkert MQ. A 14-kDa Schistosoma Mansoni Polypeptide Is Homologous to a Gene Family of Fatty Acid Binding Proteins. J Biol Chem (1991) 266(13):8447–54. doi: 10.1016/S0021-9258(18)92995-9
76. Rodriguez-Perez J, Rodriguez-Medina JR, Garcia-Blanco MA, Hillyer GV. Fasciola Hepatica: Molecular Cloning, Nucleotide Sequence, and Expression of a Gene Encoding a Polypeptide Homologous to a Schistosoma Mansoni Fatty Acid-Binding Protein. Exp Parasitol (1992) 74(4):400–7. doi: 10.1016/0014-4894(92)90202-L
77. Becker MM, Kalinna BH, Waine GJ, McManus DP. Gene Cloning, Overproduction and Purification of a Functionally Active Cytoplasmic Fatty Acid-Binding Protein (Sj-FABPC) From the Human Blood Fluke Schistosoma Japonicum. Gene (1994) 148(2):321–5. doi: 10.1016/0378-1119(94)90706-4
78. Brito CF, Oliveira GC, Oliveira SC, Street M, Riengrojpitak S, Wilson RA, et al. Sm14 Gene Expression in Different Stages of the Schistosoma Mansoni Life Cycle and Immunolocalization of the Sm14 Protein Within the Adult Worm. Braz J Med Biol Res (2002) 35(3):377–81. doi: 10.1590/S0100-879X2002000300014
79. Thaumaturgo N, Vilar MM, Diogo CM, Edelenyi R, Tendler M. Preliminary Analysis of Sm14 in Distinct Fractions of Schistosoma Mansoni Adult Worm Extract. Mem Inst Oswaldo Cruz (2001) 96 Suppl:79–83. doi: 10.1590/S0074-02762001000900011
80. Scott JC, Kennedy MW, McManus DP. Molecular and Immunological Characterisation of a Polymorphic Cytosolic Fatty Acid Binding Protein From the Human Blood Fluke of Humans, Schistosoma Japonicum. Biochim Biophys Acta (2000) 1517(1):53–62. doi: 10.1016/S0167-4781(00)00254-2
81. Thaumaturgo N, Vilar MM, Edelenyi R, Tendler M. Characterization of Sm14 Related Components in Different Helminths by Sodium Dodecyl Sulphate-Polyacrylamide Gel Electrophoresis and Western Blotting Analysis. Mem Inst Oswaldo Cruz (2002) 97(Suppl 1):115–6. doi: 10.1590/S0074-02762002000900024
82. Lee JS, Yong TS. Expression and Cross-Species Reactivity of Fatty Acid-Binding Protein of Clonorchis Sinensis. Parasitol Res (2004) 93(5):339–43. doi: 10.1007/s00436-004-1139-z
83. Espino AM, Hillyer GV. Identification of Fatty Acid Molecules in a Fasciola Hepatica Immunoprophylactic Fatty Acid-Binding Protein. J Parasitol (2001) 87(2):426–8. doi: 10.1645/0022-3395(2001)087[0426:IOFAMI]2.0.CO;2
84. Ribeiro F, Vieira Cdos S, Fernandes A, Araujo N, Katz N. The Effects of Immunization With Recombinant Sm14 (Rsm14) in Reducing Worm Burden and Mortality of Mice Infected With Schistosoma Mansoni. Rev Soc Bras Med Trop (2002) 35(1):11–7. doi: 10.1590/S0037-86822002000100003
85. Tendler M, Vilar MM, Brito CA, Freire NM, Katz N, Simpson A. Vaccination Against Schistosomiasis and Fascioliasis With the New Recombinant Antigen Sm14: Potential Basis of a Multi-Valent Anti-Helminth Vaccine? Mem Inst Oswaldo Cruz (1995) 90(2):255–6. doi: 10.1590/S0074-02761995000200022
86. Tendler M, Brito CA, Vilar MM, Serra-Freire N, Diogo CM, Almeida MS, et al. A Schistosoma Mansoni Fatty Acid-Binding Protein, Sm14, Is the Potential Basis of a Dual-Purpose Anti-Helminth Vaccine. Proc Natl Acad Sci U S A (1996) 93(1):269–73. doi: 10.1073/pnas.93.1.269
87. Mendes RE, Zafra R, Perez-Ecija RA, Buffoni L, Martinez-Moreno A, Tendler M, et al. Evaluation of Local Immune Response to Fasciola Hepatica Experimental Infection in the Liver and Hepatic Lymph Nodes of Goats Immunized With Sm14 Vaccine Antigen. Mem Inst Oswaldo Cruz (2010) 105(5):698–705. doi: 10.1590/S0074-02762010000500017
88. Zafra R, Buffoni L, Martinez-Moreno A, Perez-Ecija A, Martinez-Moreno FJ, Perez J. A Study of the Liver of Goats Immunized With a Synthetic Peptide of the Sm14 Antigen and Challenged With Fasciola Hepatica. J Comp Pathol (2008) 139(4):169–76. doi: 10.1016/j.jcpa.2008.06.004
89. Zafra R, Buffoni L, Perez-Ecija RA, Mendes RE, Martinez-Moreno A, Martinez-Moreno FJ, et al. Study of the Local Immune Response to Fasciola Hepatica in the Liver and Hepatic Lymph Nodes of Goats Immunised With a Peptide of the Sm14 Antigen. Res Vet Sci (2009) 87(2):226–32. doi: 10.1016/j.rvsc.2009.02.013
90. Almeida MS, Torloni H, Lee-Ho P, Vilar MM, Thaumaturgo N, Simpson AJ, et al. Vaccination Against Fasciola Hepatica Infection Using a Schistosoma Mansoni Defined Recombinant Antigen, Sm14. Parasite Immunol (2003) 25(3):135–7. doi: 10.1046/j.1365-3024.2003.00619.x
91. Tendler M, Simpson AJ. The Biotechnology-Value Chain: Development of Sm14 as a Schistosomiasis Vaccine. Acta Trop (2008) 108(2-3):263–6. doi: 10.1016/j.actatropica.2008.09.002
92. Tendler M, Almeida M, Simpson A. Development of the Brazilian Anti Schistosomiasis Vaccine Based on the Recombinant Fatty Acid Binding Protein Sm14 Plus GLA-SE Adjuvant. Front Immunol (2015) 6:218. doi: 10.3389/fimmu.2015.00218
93. Ramos CR, Vilar MM, Nascimento AL, Ho PL, Thaumaturgo N, Edelenyi R, et al. R-Sm14 - pRSETA Efficacy in Experimental Animals. Mem Inst Oswaldo Cruz (2001) 96(Suppl):131–5. doi: 10.1590/S0074-02762001000900019
94. Damasceno L, Ritter G, Batt CA. Process Development for Production and Purification of the Schistosoma Mansoni Sm14 Antigen. Protein Expr Purif (2017) 134:72–81. doi: 10.1016/j.pep.2017.04.002
95. Ramos CR, Figueredo RC, Pertinhez TA, Vilar MM, do Nascimento AL, Tendler M, et al. Gene Structure and M20T Polymorphism of the Schistosoma Mansoni Sm14 Fatty Acid-Binding Protein. Molecular, Functioanl, and Immunoprotection Analysis. J Biol Chem (2003) 278(15):12745–51. doi: 10.1074/jbc.M211268200
96. Ramos CR, Spisni A, Oyama S Jr., Sforca ML, Ramos HR, Vilar MM, et al. Stability Improvement of the Fatty Acid Binding Protein Sm14 From S. Mansoni by Cys Replacement: Structural and Functional Characterization of a Vaccine Candidate. Biochim Biophys Acta (2009) 1794(4):655–62. doi: 10.1016/j.bbapap.2008.12.010
97. Fonseca CT, Brito CF, Alves JB, Oliveira SC. IL-12 Enhances Protective Immunity in Mice Engendered by Immunization With Recombinant 14 kDa Schistosoma Mansoni Fatty Acid-Binding Protein Through an IFN-Gamma and TNF-Alpha Dependent Pathway. Vaccine (2004) 22(3-4):503–10. doi: 10.1016/j.vaccine.2003.07.010
98. Fonseca CT, Cunha-Neto E, Kalil J, Jesus AR, Correa-Oliveira R, Carvalho EM, et al. Identification of Immunodominant Epitopes of Schistosoma Mansoni Vaccine Candidate Antigens Using Human T Cells. Mem Inst Oswaldo Cruz (2004) 99(5 Suppl 1):63–6. doi: 10.1590/S0074-02762004000900011
99. Fonseca CT, Cunha-Neto E, Goldberg AC, Kalil J, de Jesus AR, Carvalho EM, et al. Human T Cell Epitope Mapping of the Schistosoma Mansoni 14-kDa Fatty Acid-Binding Protein Using Cells From Patients Living in Areas Endemic for Schistosomiasis. Microbes Infect (2005) 7(2):204–12. doi: 10.1016/j.micinf.2004.10.012
100. Fonseca CT, Pacifico LG, Barsante MM, Rassi T, Cassali GD, Oliveira SC. Co-Administration of Plasmid Expressing IL-12 With 14-kDa Schistosoma Mansoni Fatty Acid-Binding Protein cDNA Alters Immune Response Profiles and Fails to Enhance Protection Induced by Sm14 DNA Vaccine Alone. Microbes Infect (2006) 8(9-10):2509–16. doi: 10.1016/j.micinf.2006.06.008
101. Varaldo PB, Leite LC, Dias WO, Miyaji EN, Torres FI, Gebara VC, et al. Recombinant Mycobacterium Bovis BCG Expressing the Sm14 Antigen of Schistosoma Mansoni Protects Mice From Cercarial Challenge. Infect Immun (2004) 72(6):3336–43. doi: 10.1128/IAI.72.6.3336-3343.2004
102. Garcia TC, Fonseca CT, Pacifico LG, Duraes Fdo V, Marinho FA, Penido ML, et al. Peptides Containing T Cell Epitopes, Derived From Sm14, But Not From Paramyosin, Induce a Th1 Type of Immune Response, Reduction in Liver Pathology and Partial Protection Against Schistosoma Mansoni Infection in Mice. Acta Trop (2008) 106(3):162–7. doi: 10.1016/j.actatropica.2008.03.003
103. Ewaisha RE, Bahey-El-Din M, Mossallam SF, Amer EI, Aboushleib HM, Khalil AM. Combination of the Two Schistosomal Antigens Sm14 and Sm29 Elicits Significant Protection Against Experimental Schistosoma Mansoni Infection. Exp Parasitol (2014) 145:51–60. doi: 10.1016/j.exppara.2014.07.010
104. Ewaisha RE, Bahey-El-Din M, Mossallam SF, Khalil AM, Aboushleib HM. Successful Detection, Expression and Purification of the Alternatively Spliced Truncated Sm14 Antigen of an Egyptian Strain of Schistosoma Mansoni. J Helminthol (2015) 89(6):764–8. doi: 10.1017/S0022149X14000571
105. Mossallam SF, Amer EI, Ewaisha RE, Khalil AM, Aboushleib HM, Bahey-El-Din M. Fusion Protein Comprised of the Two Schistosomal Antigens, Sm14 and Sm29, Provides Significant Protection Against Schistosoma Mansoni in Murine Infection Model. BMC Infect Dis (2015) 15:147. doi: 10.1186/s12879-015-0906-z
106. Varaldo PB, Miyaji EN, Vilar MM, Campos AS, Dias WO, Armoa GR, et al. Mycobacterial Codon Optimization of the Gene Encoding the Sm14 Antigen of Schistosoma Mansoni in Recombinant Mycobacterium Bovis Bacille Calmette-Guerin Enhances Protein Expression But Not Protection Against Cercarial Challenge in Mice. FEMS Immunol Med Microbiol (2006) 48(1):132–9. doi: 10.1111/j.1574-695X.2006.00133.x
107. Abreu PA, Miyasato PA, Vilar MM, Dias WO, Ho PL, Tendler M, et al. Sm14 of Schistosoma Mansoni in Fusion With Tetanus Toxin Fragment C Induces Immunoprotection Against Tetanus and Schistosomiasis in Mice. Infect Immun (2004) 72(10):5931–7. doi: 10.1128/IAI.72.10.5931-5937.2004
108. Pacheco LG, Mati VL, Castro TL, Dorella FA, Oliveira SC, Miyoshi A, et al. Oral Immunization With Salmonella Harboring a Sm14-Based DNA Vaccine Does Not Protect Mice Against Schistosoma Mansoni Infection. Parasitol Int (2008) 57(4):506–8. doi: 10.1016/j.parint.2008.04.010
109. Pacheco LG, Zucconi E, Mati VL, Garcia RM, Miyoshi A, Oliveira SC, et al. Oral Administration of a Live Aro Attenuated Salmonella Vaccine Strain Expressing 14-kDa Schistosoma Mansoni Fatty Acid-Binding Protein Induced Partial Protection Against Experimental Schistosomiasis. Acta Trop (2005) 95(2):132–42. doi: 10.1016/j.actatropica.2005.05.007
110. Espindola MS, Frantz FG, Soares LS, Masson AP, Tefe-Silva C, Bitencourt CS, et al. Combined Immunization Using DNA-Sm14 and DNA-Hsp65 Increases CD8+ Memory T Cells, Reduces Chronic Pathology and Decreases Egg Viability During Schistosoma Mansoni Infection. BMC Infect Dis (2014) 14:263. doi: 10.1186/1471-2334-14-263
111. Medicine/ClinicalTrials.gov USNLo. Phase 1 Study to Evaluate the Safety of the Vaccine Prepared Sm14 Against Schistosomiasis [NCT01154049] (2014). Available at: https://clinicaltrials.gov/ct2/show/NCT01154049?term=Sm14&cond=Schistosomiasis&rank=1 (Accessed Last accessed on 30/05/2021).
112. Santini-Oliveira M, Coler RN, Parra J, Veloso V, Jayashankar L, Pinto PM, et al. Schistosomiasis Vaccine Candidate Sm14/GLA-SE: Phase 1 Safety and Immunogenicity Clinical Trial in Healthy, Male Adults. Vaccine (2016) 34(4):586–94. doi: 10.1016/j.vaccine.2015.10.027
113. Tendler M, Almeida MS, Vilar MM, Pinto PM, Limaverde-Sousa G. Current Status of the Sm14/GLA-SE Schistosomiasis Vaccine: Overcoming Barriers and Paradigms Towards the First Anti-Parasitic Human(itarian) Vaccine. Trop Med Infect Dis (2018) 3(4):121. doi: 10.3390/tropicalmed3040121
114. Medicine/ClinicalTrials.gov USNLo. Safety and Immunogenicity Evaluation of the Vaccine Candidate Sm14 in Combination With the Adjuvant Glucopyranosyl Lipid A (GLA-SE) in Adults Living in Endemic Regions for S. Mansoni and S. Haematobium in Senegal. A Comparative, Randomized, Open-Label Trial [Nct03041766] (2016). Available at: https://clinicaltrials.gov/ct2/show/NCT03041766?term=Sm14&cond=Schistosomiasis&rank=3 (Accessed Last accessed on 30/05/2021).
115. Medicine/ClinicalTrials.gov USNLo. Safety and Immunogenicity Evaluation of the Vaccine Candidate Sm14 Against Schistosomiasis in Senegalese School Children Healthy or Infected With S. Mansoni and/or S. Haematobium. A Comparative, Randomized, Controlled, Open-Label Trial [Nct03799510] (2018). Available at: https://clinicaltrials.gov/ct2/show/NCT03799510?term=Sm14&cond=Schistosomiasis&rank=2 (Accessed Last accessed on 30/05/2021).
116. Siddiqui AA, Phillips T, Charest H, Podesta RB, Quinlin ML, Pinkston JR, et al. Induction of Protective Immunity Against Schistosoma Mansoni via DNA Priming and Boosting With the Large Subunit of Calpain (Sm-P80): Adjuvant Effects of Granulocyte-Macrophage Colony-Stimulating Factor and Interleukin-4. Infect Immun (2003) 71(7):3844–51. doi: 10.1128/IAI.71.7.3844-3851.2003
117. Karcz SR, Podesta RB, Siddiqui AA, Dekaban GA, Strejan GH, Clarke MW. Molecular Cloning and Sequence Analysis of a Calcium-Activated Neutral Protease (Calpain) From Schistosoma Mansoni. Mol Biochem Parasitol (1991) 49(2):333–6. doi: 10.1016/0166-6851(91)90078-K
118. Zhang W, Ahmad G, Torben W, Siddiqui AA. Sm-P80-Based DNA Vaccine Made in a Human Use Approved Vector VR1020 Protects Against Challenge Infection With Schistosoma Mansoni in Mouse. Parasite Immunol (2010) 32(4):252–8. doi: 10.1111/j.1365-3024.2009.01181.x
119. Karmakar S, Zhang W, Ahmad G, Alam MU, Winn R, Torben W, et al. Complement Plays a Minimal Role in Sm-P80-Mediated Protection Against Schistosoma Mansoni. Hum Vaccin Immunother (2014) 10(3):640–7. doi: 10.4161/hv.27576
120. Siddiqui AA, Zhou Y, Podesta RB, Karcz SR, Tognon CE, Strejan GH, et al. Characterization of Ca(2+)-Dependent Neutral Protease (Calpain) From Human Blood Flukes, Schistosoma Mansoni. Biochim Biophys Acta (1993) 1181(1):37–44. doi: 10.1016/0925-4439(93)90087-H
121. Jankovic D, Aslund L, Oswald IP, Caspar P, Champion C, Pearce E, et al. Calpain Is the Target Antigen of a Th1 Clone That Transfers Protective Immunity Against Schistosoma Mansoni. J Immunol (1996) 157(2):806–14.
122. Molehin AJ, Sennoune SR, Zhang W, Rojo JU, Siddiqui AJ, Herrera KA, et al. Cross-Species Prophylactic Efficacy of Sm-P80-Based Vaccine and Intracellular Localization of Sm-P80/Sm-P80 Ortholog Proteins During Development in Schistosoma Mansoni, Schistosoma Japonicum, and Schistosoma Haematobium. Parasitol Res (2017) 116(11):3175–88. doi: 10.1007/s00436-017-5634-4
123. Zhang W, Molehin AJ, Rojo JU, Sudduth J, Ganapathy PK, Kim E, et al. Sm-P80-Based Schistosomiasis Vaccine: Double-Blind Preclinical Trial in Baboons Demonstrates Comprehensive Prophylactic and Parasite Transmission-Blocking Efficacy. Ann N Y Acad Sci (2018) 1425(1):38–51. doi: 10.1111/nyas.13942
124. Hota-Mitchell S, Siddiqui AA, Dekaban GA, Smith J, Tognon C, Podesta RB. Protection Against Schistosoma Mansoni Infection With a Recombinant Baculovirus-Expressed Subunit of Calpain. Vaccine (1997) 15(15):1631–40. doi: 10.1016/S0264-410X(97)00081-9
125. Karmakar S, Zhang W, Ahmad G, Torben W, Alam MU, Le L, et al. Cross-Species Protection: Schistosoma Mansoni Sm-P80 Vaccine Confers Protection Against Schistosoma Haematobium in Hamsters and Baboons. Vaccine (2014) 32(11):1296–303. doi: 10.1016/j.vaccine.2013.12.057
126. Molehin AJ, Gray SA, Turner C, Davis J, Zhang W, Khatoon S, et al. Process Development of Sj-P80: A Low-Cost Transmission-Blocking Veterinary Vaccine for Asiatic Schistosomiasis. Front Immunol (2020) 11:578715. doi: 10.3389/fimmu.2020.578715
127. Hota-Mitchell S, Clarke MW, Podesta RB, Dekaban GA. Recombinant Vaccinia Viruses and Gene Gun Vectors Expressing the Large Subunit of Schistosoma Mansoni Calpain Used in a Murine Immunization-Challenge Model. Vaccine (1999) 17(11-12):1338–54. doi: 10.1016/S0264-410X(98)00391-0
128. Siddiqui AA, Pinkston JR, Quinlin ML, Kavikondala V, Rewers-Felkins KA, Phillips T, et al. Characterization of Protective Immunity Induced Against Schistosoma Mansoni via DNA Priming With the Large Subunit of Calpain (Sm-P80) in the Presence of Genetic Adjuvants. Parasite (2005) 12(1):3–8. doi: 10.1051/parasite/2005121003
129. Ahmad G, Torben W, Zhang W, Wyatt M, Siddiqui AA. Sm-P80-Based DNA Vaccine Formulation Induces Potent Protective Immunity Against Schistosoma Mansoni. Parasite Immunol (2009) 31(3):156–61. doi: 10.1111/j.1365-3024.2008.01091.x
130. Ahmad G, Zhang W, Torben W, Noor Z, Siddiqui AA. Protective Effects of Sm-P80 in the Presence of Resiquimod as an Adjuvant Against Challenge Infection With Schistosoma Mansoni in Mice. Int J Infect Dis (2010) 14(9):e781–7. doi: 10.1016/j.ijid.2010.02.2266
131. Siddiqui AA, Pinkston JR, Quinlin ML, Saeed Q, White GL, Shearer MH, et al. Characterization of the Immune Response to DNA Vaccination Strategies for Schistosomiasis Candidate Antigen, Sm-P80 in the Baboon. Vaccine (2005) 23(12):1451–6. doi: 10.1016/j.vaccine.2004.09.018
132. Ahmad G, Zhang W, Torben W, Damian RT, Wolf RF, White GL, et al. Protective and Antifecundity Effects of Sm-P80-Based DNA Vaccine Formulation Against Schistosoma Mansoni in a Nonhuman Primate Model. Vaccine (2009) 27(21):2830–7. doi: 10.1016/j.vaccine.2009.02.096
133. Zhang W, Ahmad G, Torben W, Noor Z, Le L, Damian RT, et al. Sm-P80-Based DNA Vaccine Provides Baboons With Levels of Protection Against Schistosoma Mansoni Infection Comparable to Those Achieved by the Irradiated Cercarial Vaccine. J Infect Dis (2010) 201(7):1105–12. doi: 10.1086/651147
134. Zhang W, Ahmad G, Torben W, Siddiqui AA. Schistosoma Mansoni Antigen Sm-P80: Prophylactic Efficacy of a Vaccine Formulated in Human Approved Plasmid Vector and Adjuvant (VR 1020 and Alum). Acta Trop (2011) 118(2):142–51. doi: 10.1016/j.actatropica.2011.01.010
135. Torben W, Ahmad G, Zhang W, Siddiqui AA. Role of Antibodies in Sm-P80-Mediated Protection Against Schistosoma Mansoni Challenge Infection in Murine and Nonhuman Primate Models. Vaccine (2011) 29(12):2262–71. doi: 10.1016/j.vaccine.2011.01.040
136. Zhang W, Le L, Ahmad G, Molehin AJ, Siddiqui AJ, Torben W, et al. Fifteen Years of Sm-P80-Based Vaccine Trials in Nonhuman Primates: Antibodies From Vaccinated Baboons Confer Protection In Vivo and In Vitro From Schistosoma Mansoni and Identification of Putative Correlative Markers of Protection. Front Immunol (2020) 11:1246. doi: 10.3389/fimmu.2020.01246
137. Torben W, Ahmad G, Zhang W, Nash S, Le L, Karmakar S, et al. Role of Antibody Dependent Cell Mediated Cytotoxicity (ADCC) in Sm-P80-Mediated Protection Against Schistosoma Mansoni. Vaccine (2012) 30(48):6753–8. doi: 10.1016/j.vaccine.2012.09.026
138. Zhang W, Ahmad G, Le L, Rojo JU, Karmakar S, Tillery KA, et al. Longevity of Sm-P80-Specific Antibody Responses Following Vaccination With Sm-P80 Vaccine in Mice and Baboons and Transplacental Transfer of Sm-P80-Specific Antibodies in a Baboon. Parasitol Res (2014) 113(6):2239–50. doi: 10.1007/s00436-014-3879-8
139. Karmakar S, Zhang W, Ahmad G, Torben W, Alam MU, Le L, et al. Use of an Sm-P80-Based Therapeutic Vaccine to Kill Established Adult Schistosome Parasites in Chronically Infected Baboons. J Infect Dis (2014) 209(12):1929–40. doi: 10.1093/infdis/jiu031
140. Le L, Zhang W, Karmakar S, Ahmad G, Torben W, Siddiqui AA. Simultaneous Priming With DNA Encoding Sm-P80 and Boosting With Sm-P80 Protein Confers Protection Against Challenge Infection With Schistosoma Mansoni in Mice. Parasitol Res (2014) 113(3):1195–200. doi: 10.1007/s00436-014-3757-4
141. Le L, Molehin AJ, Nash S, Sennoune SR, Ahmad G, Torben W, et al. Schistosoma Egg-Induced Liver Pathology Resolution by Sm-P80-Based Schistosomiasis Vaccine in Baboons. Pathology (2018) 50(4):442–9. doi: 10.1016/j.pathol.2018.01.004
142. Siddiqui AJ, Molehin AJ, Zhang W, Ganapathy PK, Kim E, Rojo JU, et al. Sm-P80-Based Vaccine Trial in Baboons: Efficacy When Mimicking Natural Conditions of Chronic Disease, Praziquantel Therapy, Immunization, and Schistosoma Mansoni Re-Encounter. Ann N Y Acad Sci (2018) 1425(1):19–37. doi: 10.1111/nyas.13866
143. Capron A, Riveau G, Capron M, Trottein F. Schistosomes: The Road From Host-Parasite Interactions to Vaccines in Clinical Trials. Trends Parasitol (2005) 21(3):143–9. doi: 10.1016/j.pt.2005.01.003
144. Johnson KA, Angelucci F, Bellelli A, Herve M, Fontaine J, Tsernoglou D, et al. Crystal Structure of the 28 kDa Glutathione S-Transferase From Schistosoma Haematobium. Biochemistry (2003) 42(34):10084–94. doi: 10.1021/bi034449r
145. Trottein F, Godin C, Pierce RJ, Sellin B, Taylor MG, Gorillot I, et al. Inter-Species Variation of Schistosome 28-kDa Glutathione S-Transferases. Mol Biochem Parasitol (1992) 54(1):63–72. doi: 10.1016/0166-6851(92)90095-2
146. Bourke CD, Nausch N, Rujeni N, Appleby LJ, Trottein F, Midzi N, et al. Cytokine Responses to the Anti-Schistosome Vaccine Candidate Antigen Glutathione-S-Transferase Vary With Host Age and Are Boosted by Praziquantel Treatment. PloS Negl Trop Dis (2014) 8(5):e2846. doi: 10.1371/journal.pntd.0002846
147. Boulanger D, Warter A, Trottein F, Mauny F, Bremond P, Audibert F, et al. Vaccination of Patas Monkeys Experimentally Infected With Schistosoma Haematobium Using a Recombinant Glutathione S-Transferase Cloned From S. Mansoni. Parasite Immunol (1995) 17(7):361–9. doi: 10.1111/j.1365-3024.1995.tb00903.x
148. Boulanger D, Warter A, Sellin B, Lindner V, Pierce RJ, Chippaux JP, et al. Vaccine Potential of a Recombinant Glutathione S-Transferase Cloned From Schistosoma Haematobium in Primates Experimentally Infected With an Homologous Challenge. Vaccine (1999) 17(4):319–26. doi: 10.1016/S0264-410X(98)00202-3
149. Lee JJ, Sinha KA, Harrison JA, de Hormaeche RD, Riveau G, Pierce RJ, et al. Tetanus Toxin Fragment C Expressed in Live Salmonella Vaccines Enhances Antibody Responses to its Fusion Partner Schistosoma Haematobium Glutathione S-Transferase. Infect Immun (2000) 68(5):2503–12. doi: 10.1128/IAI.68.5.2503-2512.2000
150. Scott JC, McManus DP. Molecular Cloning and Enzymatic Expression of the 28-kDa Glutathione S-Transferase of Schistosoma Japonicum: Evidence for Sequence Variation But Lack of Consistent Vaccine Efficacy in the Murine Host. Parasitol Int (2000) 49(4):289–300. doi: 10.1016/S1383-5769(00)00058-1
151. Mitchell GF. Glutathione S-Transferases - Potential Components of Anti-Schistosome Vaccines? Parasitol Today (1989) 5(2):34–7. doi: 10.1016/0169-4758(89)90185-3
152. Mutapi F, Burchmore R, Mduluza T, Foucher A, Harcus Y, Nicoll G, et al. Praziquantel Treatment of Individuals Exposed to Schistosoma Haematobium Enhances Serological Recognition of Defined Parasite Antigens. J Infect Dis (2005) 192(6):1108–18. doi: 10.1086/432553
153. Xu CB, Verwaerde C, Grzych JM, Fontaine J, Capron A. A Monoclonal Antibody Blocking the Schistosoma Mansoni 28-kDa Glutathione S-Transferase Activity Reduces Female Worm Fecundity and Egg Viability. Eur J Immunol (1991) 21(8):1801–7. doi: 10.1002/eji.1830210804
154. McTigue MA, Williams DR, Tainer JA. Tainer, Crystal Structures of a Schistosomal Drug and Vaccine Target: Glutathione S-Transferase From Schistosoma Japonica and its Complex With the Leading Antischistosomal Drug Praziquantel. J Mol Biol (1995) 246(1):21–7. doi: 10.1006/jmbi.1994.0061
155. Milhon JL, Thiboldeaux RL, Glowac K, Tracy JW. Schistosoma Japonicum GSH S-Transferase Sj26 Is Not the Molecular Target of Praziquantel Action. Exp Parasitol (1997) 87(3):268–74. doi: 10.1006/expr.1997.4231
156. Webbe G, James C, Nelson GS, Ismail MM, Shaw JR. Cross Resistance Between Schistosoma Haematobium and S. Mansoni in the Baboon. Trans R Soc Trop Med Hyg (1979) 73(1):42–54. doi: 10.1016/0035-9203(79)90128-7
157. Chippaux JP. [The Center for Research on Meningitis and Schistosomiasis (CERMES), Naimey, Niger]. Med Trop (Mars) (1998) 58(2):199–203.
158. Wolowczuk I, Auriault C, Bossus M, Boulanger D, Gras-Masse H, Mazingue C, et al. Antigenicity and Immunogenicity of a Multiple Peptidic Construction of the Schistosoma Mansoni Sm-28 GST Antigen in Rat, Mouse, and Monkey. 1. Partial Protection of Fischer Rat After Active Immunization. J Immunol (1991) 146(6):1987–95.
159. Grezel D, Capron M, Grzych JM, Fontaine J, Lecocq JP, Capron A. Protective Immunity Induced in Rat Schistosomiasis by a Single Dose of the Sm28GST Recombinant Antigen: Effector Mechanisms Involving IgE and IgA Antibodies. Eur J Immunol (1993) 23(2):454–60. doi: 10.1002/eji.1830230223
160. Lane A, Boulanger D, Riveau G, Capron A, Wilson RA. Murine Immune Responses to Schistosoma Haematobium and the Vaccine Candidate rSh28GST. Parasite Immunol (1998) 20(8):359–67.
161. Auriault C, Gras-Masse H, Pierce RJ, Butterworth AE, Wolowczuk I, Capron M, et al. Antibody Response of Schistosoma Mansoni-Infected Human Subjects to the Recombinant P28 Glutathione-S-Transferase and to Synthetic Peptides. J Clin Microbiol (1990) 28(9):1918–24. doi: 10.1128/jcm.28.9.1918-1924.1990
162. Pierce RJ, Balloul JM, Grzych JM, Dissous C, Auriault C, Boulanger D, et al. GP38, P28-I and P28-II: Candidates for a Vaccine Against Schistosomiasis. Mem Inst Oswaldo Cruz (1987) 82(Suppl 4):111–4. doi: 10.1590/S0074-02761987000800018
163. Ndhlovu P, Cadman H, Vennervald BJ, Christensen NO, Chidimu M, Chandiwana SK. Age-Related Antibody Profiles in Schistosoma Haematobium Infections in a Rural Community in Zimbabwe. Parasite Immunol (1996) 18(4):181–91. doi: 10.1046/j.1365-3024.1996.d01-78.x
164. Riveau G, Poulain-Godefroy OP, Dupre L, Remoue F, Mielcarek N, Locht C, et al. Glutathione S-Transferases of 28kDa as Major Vaccine Candidates Against Schistosomiasis. Mem Inst Oswaldo Cruz (1998) 93(Suppl 1):87–94. doi: 10.1590/S0074-02761998000700012
165. Kremer L, Dupre L, Riveau G, Capron A, Locht C. Systemic and Mucosal Immune Responses After Intranasal Administration of Recombinant Mycobacterium Bovis Bacillus Calmette-Guerin Expressing Glutathione S-Transferase From Schistosoma Haematobium. Infect Immun (1998) 66(12):5669–76. doi: 10.1128/IAI.66.12.5669-5676.1998
166. Kremer L, Riveau G, Baulard A, Capron A, Locht C. Neutralizing Antibody Responses Elicited in Mice Immunized With Recombinant Bacillus Calmette-Guerin Producing the Schistosoma Mansoni Glutathione S-Transferase. J Immunol (1996) 156(11):4309–17.
167. Dupre L, Poulain-Godefroy O, Ban E, Ivanoff N, Mekranfar M, Schacht AM, et al. Intradermal Immunization of Rats With Plasmid DNA Encoding Schistosoma Mansoni 28 kDa Glutathione S-Transferase. Parasite Immunol (1997) 19(11):505–13. doi: 10.1046/j.1365-3024.1997.d01-163.x
168. Riveau G, Deplanque D, Remoue F, Schacht AM, Vodougnon H, Capron M, et al. Safety and Immunogenicity of rSh28GST Antigen in Humans: Phase 1 Randomized Clinical Study of a Vaccine Candidate Against Urinary Schistosomiasis. PloS Negl Trop Dis (2012) 6(7):e1704. doi: 10.1371/journal.pntd.0001704
169. Medicine/Clinical/Trial.gov USNLo. Phase 1 Study Evaluating Safety and Immunological Criteria of Efficacy of the Recombinant Vaccine Candidate Bilhvax Against Schistosomiasis [Nct01512277] (1999). Available at: https://clinicaltrials.gov/ct2/show/NCT01512277?term=rsh28GST&cond=Schistosomiasis&draw=2&rank=1 (Accessed Last accessed on 30/05/2021).
170. Capron A, Capron M, Dombrowicz D, Riveau G. Vaccine Strategies Against Schistosomiasis: From Concepts to Clinical Trials. Int Arch Allergy Immunol (2001) 124(1-3):9–15. doi: 10.1159/000053656
171. Riveau G, Schacht AM, Dompnier JP, Deplanque D, Seck M, Waucquier N, et al. Safety and Efficacy of the rSh28GST Urinary Schistosomiasis Vaccine: A Phase 3 Randomized, Controlled Trial in Senegalese Children. PloS Negl Trop Dis (2018) 12(12):e0006968. doi: 10.1371/journal.pntd.0006968
172. Medicine/ClinicalTrials.gov USNLo. Efficacy and Safety Evaluation of the Therapeutic Vaccine Candidate Sh28GST in Association With Praziquantel (PZQ) for Prevention of Clinical and Parasitological Recurrences of S. Haematobium Infection in Children [Nct00870649] (2012). Available at: https://clinicaltrials.gov/ct2/show/NCT00870649?term=sh28GST&cond=Schistosomiasis&draw=2&rank=1 (Accessed Last accessed on 30/05/2021).
173. Remoue F, To Van D, Schacht AM, Picquet M, Garraud O, Vercruysse J, et al. Gender-Dependent Specific Immune Response During Chronic Human Schistosomiasis Haematobia. Clin Exp Immunol (2001) 124(1):62–8. doi: 10.1046/j.1365-2249.2001.01495.x
174. Mutapi F, Mduluza T, Gomez-Escobar N, Gregory WF, Fernandez C, Midzi N, et al. Immuno-Epidemiology of Human Schistosoma Haematobium Infection: Preferential IgG3 Antibody Responsiveness to a Recombinant Antigen Dependent on Age and Parasite Burden. BMC Infect Dis (2006) 6:96. doi: 10.1186/1471-2334-6-96
175. Wilson RA, Li XH, Castro-Borges W. Do Schistosome Vaccine Trials in Mice Have an Intrinsic Flaw That Generates Spurious Protection Data? Parasit Vectors (2016) 9:89. doi: 10.1186/s13071-016-1369-9
176. Bourke CD, Nausch N, Rujeni N, Appleby LJ, Mitchell KM, Midzi N, et al. Integrated Analysis of Innate, Th1, Th2, Th17, and Regulatory Cytokines Identifies Changes in Immune Polarisation Following Treatment of Human Schistosomiasis. J Infect Dis (2013) 208(1):159–69. doi: 10.1093/infdis/jis524
177. Melkus MW, Le L, Siddiqui AJ, Molehin AJ, Zhang W, Lazarus S, et al. Elucidation of Cellular Responses in Non-Human Primates With Chronic Schistosomiasis Followed by Praziquantel Treatment. Front Cell Infect Microbiol (2020) 10:57. doi: 10.3389/fcimb.2020.00057
178. Gaze S, Driguez P, Pearson MS, Mendes T, Doolan DL, Trieu A, et al. An Immunomics Approach to Schistosome Antigen Discovery: Antibody Signatures of Naturally Resistant and Chronically Infected Individuals From Endemic Areas. PloS Pathog (2014) 10(3):e1004033. doi: 10.1371/journal.ppat.1004033
179. Egesa M, Lubyayi L, Jones FM, van Diepen A, Chalmers IW, Tukahebwa EM, et al. Antibody Responses to Schistosoma Mansoni Schistosomula Antigens. Parasite Immunol (2018) 40(12):e12591. doi: 10.1111/pim.12591
180. Lin L, Finak G, Ushey K, Seshadri C, Hawn TR, Frahm N, et al. COMPASS Identifies T-Cell Subsets Correlated With Clinical Outcomes. Nat Biotechnol (2015) 33(6):610–6. doi: 10.1038/nbt.3187
181. Elliott AM, Roestenberg M, Wajja A, Opio C, Angumya F, Adriko M, et al. Ethical and Scientific Considerations on the Establishment of a Controlled Human Infection Model for Schistosomiasis in Uganda: Report of a Stakeholders’ Meeting Held in Entebbe, Uganda. AAS Open Res (2018) 1:2. doi: 10.12688/aasopenres.12841.2
182. Koopman JP, Egesa M, Wajja A, Adriko M, Nassuuna J, Nkurunungi G, et al. Risk Assessment for the Implementation of Controlled Human Schistosoma Mansoni Infection Trials in Uganda. AAS Open Res (2019) 2:17. doi: 10.12688/aasopenres.12972.1
183. Langenberg MCC, Hoogerwerf MA, Koopman JPR, Janse JJ, Kos-van Oosterhoud J, Feijt C, et al. A Controlled Human Schistosoma Mansoni Infection Model to Advance Novel Drugs, Vaccines and Diagnostics. Nat Med (2020) 26(3):326–32. doi: 10.1038/s41591-020-0759-x
184. Tedla BA, Pickering D, Becker L, Loukas A, Pearson MS. Vaccination With Schistosoma Mansoni Cholinesterases Reduces the Parasite Burden and Egg Viability in a Mouse Model of Schistosomiasis. Vaccines (Basel) (2020) 8(2). doi: 10.3390/vaccines8020162
185. Pearson MS, Becker L, Driguez P, Young ND, Gaze S, Mendes T, et al. Of Monkeys and Men: Immunomic Profiling of Sera From Humans and non-Human Primates Resistant to Schistosomiasis Reveals Novel Potential Vaccine Candidates. Front Immunol (2015) 6:213. doi: 10.3389/fimmu.2015.00213
186. De Sousa KP, Doolan DL. Immunomics: A 21st Century Approach to Vaccine Development for Complex Pathogens. Parasitology (2016) 143(2):236–44. doi: 10.1017/S0031182015001079
187. Pinheiro CS, Martins VP, Assis NR, Figueiredo BC, Morais SB, Azevedo V, et al. Computational Vaccinology: An Important Strategy to Discover New Potential S. Mansoni Vaccine Candidates. J BioMed Biotechnol (2011) 2011:503068. doi: 10.1155/2011/503068
188. Castro-Borges W, Dowle A, Curwen RS, Thomas-Oates J, Wilson RA. Enzymatic Shaving of the Tegument Surface of Live Schistosomes for Proteomic Analysis: A Rational Approach to Select Vaccine Candidates. PloS Negl Trop Dis (2011) 5(3):e993. doi: 10.1371/journal.pntd.0000993
Keywords: Schistosoma, schistosomiasis, vaccines, protective immunity, praziquantel, non-human primate model, controlled human infection model, efficacy endpoints
Citation: Panzner U, Excler J-L, Kim JH, Marks F, Carter D and Siddiqui AA (2021) Recent Advances and Methodological Considerations on Vaccine Candidates for Human Schistosomiasis. Front. Trop. Dis 2:719369. doi: 10.3389/fitd.2021.719369
Received: 02 June 2021; Accepted: 12 August 2021;
Published: 26 August 2021.
Edited by:
Michael A. Eller, Division of AIDS (NIAID), United StatesReviewed by:
William Evan Secor, Centers for Disease Control and Prevention (CDC), United StatesAlexander Kwarteng, Kwame Nkrumah University of Science and Technology, Ghana
Akram Dadarah, Tufts University, United States
Copyright © 2021 Panzner, Excler, Kim, Marks, Carter and Siddiqui. This is an open-access article distributed under the terms of the Creative Commons Attribution License (CC BY). The use, distribution or reproduction in other forums is permitted, provided the original author(s) and the copyright owner(s) are credited and that the original publication in this journal is cited, in accordance with accepted academic practice. No use, distribution or reproduction is permitted which does not comply with these terms.
*Correspondence: Ursula Panzner, dXBhbnpuZXJAaXZpLmludA==