- Division of Blood and Marrow Transplantation and Cellular Therapy, Department of Medicine, Stanford University, Stanford, CA, United States
Invariant Natural Killer T cell therapy is an emerging platform of immunotherapy for cancer treatment. This unique cell population is a promising candidate for cell therapy for cancer treatment because of its inherent cytotoxicity against CD1d positive cancers as well as its ability to induce host CD8 T cell cross priming. Substantial evidence supports that iNKT cells can modulate myelomonocytic populations in the tumor microenvironment to ameliorate immune dysregulation to antagonize tumor progression. iNKT cells can also protect from graft-versus-host disease (GVHD) through several mechanisms, including the expansion of regulatory T cells (Treg). Ultimately, iNKT cell-based therapy can retain antitumor activity while providing protection against GVHD simultaneously. Therefore, these biological properties render iNKT cells as a promising “off-the-shelf” therapy for diverse hematological malignancies and possible solid tumors. Further the introduction of a chimeric antigen recetor (CAR) can further target iNKT cells and enhance function. We foresee that improved vector design and other strategies such as combinatorial treatments with small molecules or immune checkpoint inhibitors could improve CAR iNKT in vivo persistence, functionality and leverage anti-tumor activity along with the abatement of iNKT cell dysfunction or exhaustion.
1 Introduction
Invariant Natural Killer T cells (iNKT cells) belong to a subset of innate lymphocytes that express an invariant T-cell receptor (TCR) that recognizes specific lipid antigens presented by cells expressing the MHC-like molecule CD1d (1). iNKT cells are known to have cytotoxicity against cancer cells as well as immunoregulatory like properties where unlike T cells, iNKT cells do not cause GVHD (2). Furthermore, there are several lines of evidence supporting the suppression or prevention of GVHD by iNKT cells through the expansion of CD4 + CD25 + FOXP3 + regulatory T cells (Treg) (3). These unique biological properties render iNKT cells as an ideal “off-the-shelf” product for allogeneic adoptive cell therapy for various hematologic malignancies and perhaps other cancers (4). Chimeric antigen receptors (CARs) can be introduced into iNKT cells to augment cytotoxicity (4–8). Further advantages of CAR iNKT cells are that they are distinct cells that can be isolated and expanded, recognize a unique marker CD1d that is frequently expressed on hematological malignancies and have cytotoxic capacity that may be augmented through other ligands (1, 5, 9). Interestingly, these engineered iNKT cells can exert both direct antitumor activity as well as an indirect activation of host CD8 mediated immunity and do not cause untoward effects such as GVHD (10). In this review, we discuss the biology of this unique cell population, their interaction with other immune cells, and the potential strategies to harness their biological properties to abate immunological dysregulation in hematological malignancies and their complications (1) such as GVHD and to treat disease and prevent relapse.
2 iNKT—basic biology
iNKT cells are a unique cell population that shares properties of both T cells and natural killer cells (1, 11). They express an invariant TCR (define mouse and human) defined by the specific interaction between their semi-conserved T cell receptors and either self or exogenous lipid antigen presented by CD1d (a conserved polymorphic MHC class I like molecule) on antigen presenting cell such as dendritic cells (1, 12). CD1d restriction defines this cell population (1, 2). In mice, iNKT cells TCR expression includes Vα14Jα18 chain paring with a limited Vβ repertoire (Vβ2, Vβ7, Vβ8.1, Vβ8.2 or Vβ8.3) (13, 14). In humans, Vα24Jα18 chain pairs almost exclusively with Vβ11. Like conventional T cells, iNKT cells develop in the thymus (1, 15). The double positive (CD4+ CD8+) thymocytes act as progenitor cells for all lymphocytes that belong to the αβ T cell lineage (16). Thymic selection shapes the development and differentiation of functional iNKT cells through the expression of promyelocytic leukemia zinc finger protein or PLZF (17). In C57 BL/6 mice, iNKT cells represent about 1%–2% of lymphocytes in the spleen or liver (13, 14). Mature iNKT cells are widely distributed in various tissues such as bone marrow, gastrointestinal tract, liver, and adipose tissue (13, 18). However, iNKT cells rarely recirculate as compared to MHC restricted T cells (1). In humans, iNKT cells consist of 0.1%–0.2% of T cells and are enriched in omentum (19–22). There are two fundamental lipid antigens that stimulate iNKT cells (1)—(1) glycosphingolipids or ceremide-based glycolipids and (2) glycerol-based lipids such as membrane phospholipids as self-antigens (1). The glycosphingolipid has α-orientation of glycosidic linkage between the carbohydrate head group and the lipid backbone, and this is not known to exist in mammals (1). However, this configuration is found in various pathogens (1, 23–26). This may explain how iNKT cells are stimulated during infection (1, 26). The lipid antigens exert their interaction with CD1d and TCR by regulating the strength of binding of this complex rather than the specificity of interaction (1). The first well characterized lipid antigen is α-galactosylceramide (α-GalCer) which is derived from a murine sponge (27). This is a well-known compound used to stimulate and enhance the expansion of iNKT cells in vitro (5). Unfortunately, a-GalCer has shown limited therapeutic efficacy in several clinical trials of cancer treatment but may be an effective strategy to reduce or ameliorate acute GVHD (28–30). Subsequently, there is a newly synthesized non-glycosidic analog—Threitolceremide-6 which potentially enhances anti-tumor activity and renders a potent stimulation of iNKT cells (31). This compound has now been investigated in clinical trials as a potential strategy to enhance the antitumor activity of iNKT cells (32, 33).
CD1d, it is a transmembrane protein like MHC class I that binds non-covalently to β-microglobulin (1). CD1d expression is found mainly on immune cells such as dendritic cells, macrophages, granulocytes, and B cells (34–36). CD1d is also frequently and strongly expressed on various hematological malignancies such as acute myeloid leukemia (AML) (37–39). This hypothesizes that iNKT cells may be an excellent candidate for adoptive cell therapy for hematological malignancies since all individuals have identical CD1d molecules (2). Further, iNKT cells are amendable to the adoptive cell transfer across MHC barriers without significant alloreactive consequences (2). This cell population has a unique immunoregulatory role that redirects immune response to both internal and exogenous signals (1). Several lines of evidence support the concept that iNKT cells can elicit hybrid immune responses encompassing both innate and adaptive immunity (1). iNKT cells have direct cytotoxicity against cancer (1, 2, 4, 40). Furthermore, the transcriptional profiles of iNKT cells are comparable to those of both innate and adaptive immune cells depending on the antigens or stimuli (1). Substantial compelling evidence indicates that iNKT cells can prime CD8 T cells (2, 10). iNKT cells can also interact with other immune cells such as dendritic cells, macrophages, and B cells to mediate the complex adaptive-innate immunological interactions in response to various antigens such as pathogens, cancer cells or other antigens (1). In the next section, we describe iNKT cells subsets and their development in details.
3 iNKT cell subsets and their development
The heterogeneity of iNKT cells has been extensively investigated in rodents which suggest three primary iNKT sublineages: iNKT1, iNKT2, and iNKT17 (41). iNKT cells differentiate during murine thymic development into these three distinct subsets that are characterized by their transcriptomic and epigenomic differences (42). iNKT1 cells can be identified by their production of interferon-gamma (IFN-γ) and dependence on the cytokine IL-15 for survival (43). iNKT1 cells express the transcription factor T-bet and are characterized by cell surface expression of CXCR3, CCR5, and VLA-1. iNKT2 cells are characterized by their production of interleukin-4 (IL-4) and expression of Gata-3 transcription factor as well as CCR4 and CCR9 surface receptors. iNKT17 cells produce IL-17 and express the ROR-γ transcription factor as well as the cell surface markers CCR6, Itgb4, Itgb5, and Itgb7 (44). While the differences in transcription factors maintain cellular identity, the differences in molecular phenotype drive unique tissue distributions (45). Murine iNKT1 cells are generally found in the liver and spleen, iNKT2 cells are most prevalent in the spleen and lymph nodes, and iNKT17 cells are generally located in the lymph nodes and lungs (46). Tissue residence of iNKT cells is long lived, enabling a rapid specific response to local stimuli.
The development of iNKT cell subsets and the signals regulating the commitment of these subsets have been thoroughly reviewed (47–50). Thymic positive selection of iNKT cell precursors depends on recognition of “self” lipid-CD1d complexes by their TCR or presentation of endogenous ligands α-galactosylceramide (α-GalCer) and α-glucosylceramide. iNKT cells are positively selected at the CD4+ CD8+ double-positive (DP) stage by CD1d-expressing DP thymocytes leading to “stage 0” which is characterized by CD69+ CD24hi cells with high Egr2 expression (48, 49). As these cells continue to mature they reach stage 1 characterized by down regulation of CD24 and CD69 and expression of high levels of PLZF (48, 51, 52). These stage 1 iNKT cells can be differentiated based on expression levels of IL-17RB which is selectively expressed on mature iNKT2 and iNKT17 cells but not iNKT1 cells (53). Stage 2 iNKT cells are characterized by acquisition of memory-like CD44hi phenotype, where they differentiate into distinct sub-populations (stage 3): iNKT1 cells, which cease proliferation and acquire T-bet and NK-like characteristics (NK1.1+ and CD122hi) with downregulation of PLZF and GATA3, iNKT2 cells, which exhibit retention of PLZF and high expression of GATA3 and produce IL4 and IL13 upon stimulation, and iNKT17 cells, which are characterized by upregulated expression of RORgt, intermediary expression of PLZF and downregulated expression of T-bet and produce IL-17 upon stimulation (48). The stages of development of thymic iNKT cells have been summarized in Table 1. Despite exhaustive studies into iNKT cell development and identification of roles of different signaling pathways and transcription factors, the knowledge regarding mechanisms controlling the differentiation of the iNKT subsets remains elusive.
4 iNKT cell activation
The semi-invariant TCR enables iNKT cells to swiftly respond to specific antigens presented by CD1d molecules (54). CD1d-mediated lipid antigen presentation is a critical process in iNKT cell activation (55, 56). While TCR recognition of glycolipids by CD1d remains central to iNKT cell activation, non-TCR signaling, including cytokine-mediated activation and direct glycolipid binding to CD1d, plays an essential role in modulating iNKT cell responses (46, 57). Understanding the multifaceted mechanisms underlying iNKT cell activation is crucial for harnessing the potential of these unique immune cells in therapeutic and immunomodulatory applications.
4.1 CD1d mediated lipid antigen presentation
The nature of the lipid antigens presented by CD1 molecules necessitates specific mechanisms for their uptake by antigen-presenting cells (APCs) and loading onto CD1 molecules (58). Lipid transfer proteins, including apolipoprotein E and fatty acid amide hydrolase could facilitate antigen presentation by CD1d (59–61). Similar to MHC antigens, to generate bioactive fragments lipid antigens need be processed in the lysosome (62). CD1d can present a wide range of antigens, including synthetic antigens (62), microbial antigens (63), and self-antigens (59). α-Galactosylceramide (α-GalCer), a synthetic glycolipid derived from galactosylceramides found in the marine sponge Agelas mauritianus, serves as a potent agonist and is widely used to investigate CD1d-mediated iNKT cell activation (64, 65). Studies using CD1d-deficient mice demonstrated the indispensable role of CD1d in presenting α-GalCer to iNKT cells (12, 66). Thus, a-Galcer is a tool to study and optimize CD1d-mediated iNKT activation.
iNKT cells, recognized by their expression of the NK cell receptor NK1.1, exhibit a repertoire of receptors akin to natural killer (NK) cells (67). This characteristic led to their initial classification as “NK T cells.” This nomenclature created confusion concerning the involvement of cytokines like IL-12, IL-18, and IFN-α in iNKT cell stimulation, given the dependence of NK cell activation on these cytokines (68). However, extensive in vivo and in vitro data indicate that initial iNKT cell activation is cytokine-independent. Tools such as CD1d tetramers have enabled the discrimination between iNKT cells and NKT cells, providing clear evidence that inflammatory cytokines and co-stimulatory molecules are dispensable for iNKT cell activation (69, 70). In vitro studies using soluble CD1d coated on plates demonstrated that agonist glycolipids bound to CD1d were sufficient to activate iNKT cells (71). Microbial glycolipids from α-proteobacteria could also directly activate iNKT cells without TLR or IL12 (23, 24, 26, 72). It is important to note that excessive iNKT cell activation with analogs can lead to iNKT cell anergy (73–75).
4.2 Activation of iNKT cells by non -TCR signaling
iNKT cells express various cytokine receptors, such as IL-2R, IL-7R, IL-12R, and IL-18R, and are capable of rapid cytokine production due to the presence of cytokine transcripts (57, 76, 77). iNKT cells can release IFN-γ when stimulated by cytokines like IL-12 and IL-18 without TCR involvement, suggesting a potential NK-like cell role for iNKT cells (78, 79). During certain infections, iNKT cells can become activated, characterized by the upregulation of CD25 and robust IFN-γ production (80, 81). The invariant TCR recognition mediated by CD1d plays a crucial role in this process as either anti-CD1d antibodies or iNKT cell adoptive transfer into CD1d−/− hosts could diminish activation (82). Infections like MCMV activate dendritic cells via TLR9, leading to IL-12 production, which subsequently activates iNKT cells to produce IFN-γ (81). TLR9 deficiency in mice infected with MCMV does not lead to significant iNKT cell activation, emphasizing the role of TLR9 in this type of activation (81). Similar findings have shown that CpG-induced iNKT cell activation is dependent on IL-12, with CD1d playing a minimal role (83). Additionally, LPS, another TLR ligand, has shown that CD1d is not always essential in all conditions (84). To sum up, iNKT cells can effectively respond to some pathogens with minimal TCR engagement.
IL-33, a member of the interleukin-1 family, has been shown to promote iNKT cell activation in both humans and murine models (85–87). This cytokine promotes IFN-γ production by iNKT cells in the presence of CD1d-mediated α-GalCer stimulation. Notably, when IL-12 is introduced into the system, IL-33 can induce IFN-γ production from iNKT cells even without TCR engagement.
5 Interaction of iNKT cells with other immune cells
iNKT cells, characterized by their distinctive T Cell Receptor (TCR) composition, possess a remarkable ability to engage with CD1d-expressing antigen-presenting cells (APCs), including dendritic cells (DCs), macrophages, neutrophils, and B cells. This interaction is not only characterized by the recognition of specific antigens but also involves the intricate interplay of cytokines, chemokines, and surface molecules. These multifaceted interactions empower iNKT cells to participate actively in the immune regulatory network (Figure 1A).
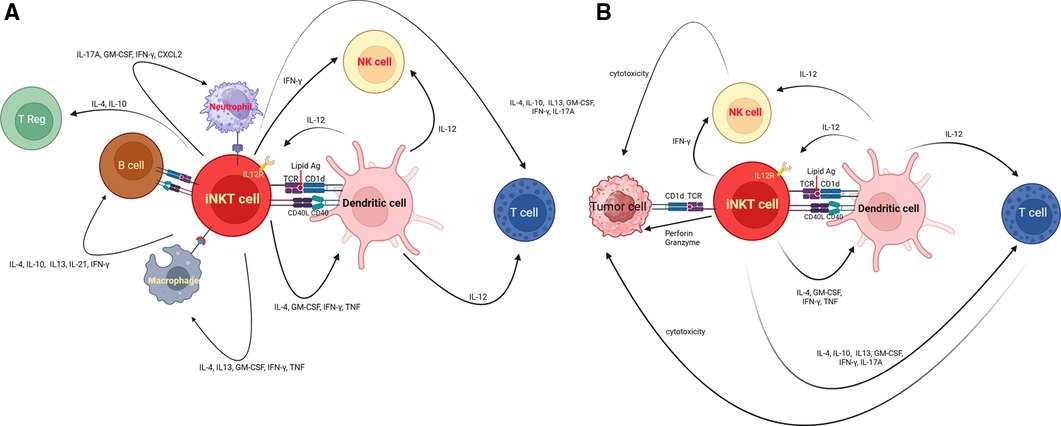
Figure 1. (A) Illustrates the interaction between iNKT cells, and other immune cells. iNKT cells possess T-cell receptor (TCR) that recognizes specific lipid antigens presented by cells expressing CD1d. Non-TCR signaling, including cytokine-mediated activation and direct glycolipid binding to CD1d, are sine qua non of modulating iNKT cell responses. In addition, iNKT cells express a variety of receptors, including cytokine receptors such as IL-12R, as well as modulatory molecules like PD-1 and CD40l. iNKT cells interact with other cells such as dendritic cells, their reciprocal interaction results in activation of dendritic cells, triggering IL-12 production and an upregulation in stimulatory lipid antigen presentation for iNKT cells. Upon recognition of self or foreign lipid antigens presented by CD1d on APCs, iNKT cells engage in CD40–CD40 ligand interactions, further enhancing IL-12 production by dendritic cells. The interaction between iNKT cells and other immune cells results in the complex immune response such as NK cell transactivation, T cell activation/differentiation, recruitment/regulation of neutrophils, activation of B cells, regulation of macrophages in tumor microenvironment, or expansion of T regulatory cells. Taken together, iNKT cells act as the mediator of adaptive and innate immune response to either exogenous or self-antigen. (B) iNKT cells and immune dysregulation in hematological cancers. iNKT cells eliminate cancer by two main mechanisms. The first mechanism is to directly recognize and eliminate cancer cells harboring CD1d via perforin/ granzyme. The other main mechanism that iNKT cells use to control tumor is indirect crosstalk with other immune cells in the tumor microenvironment (TME). iNKT cells can promote dendritic cell maturation. iNKT cells can also secrete IL-12 which activates anti-tumor CD4+ and CD8+ T cells.
5.1 Interactions with dendritic cells
Dendritic cells, a vital component of the immune system, constitutively express CD1d, making them pivotal in mediating iNKT cell activation, especially within the spleen (88, 89). Upon presentation of lipid antigens to iNKT cells, an immune response ensues characterized by robust IFNγ production and NK cell transactivation. Notably, a specific subset of dendritic cells, the CD8a + DEC-205+ DCs, stands out for their ability to capture and present diverse glycolipid antigens, including various forms of a-galactosylceramide, leading to distinct cytokine responses (90). Post glycolipid presentation, these DCs dynamically modulate their expression of costimulatory and coinhibitory molecules, a process intricately dependent on antigen structure.
The interaction between these two cell types is bidirectional, particularly during infection. Signals from pattern-recognition receptors activate DCs, triggering IL-12 production and an upregulation in stimulatory lipid antigen presentation for iNKT cells (91, 92). Upon recognition of self or foreign lipid antigens presented by CD1d on APCs, iNKT cells engage in CD40–CD40ligand interactions, further enhancing IL-12 production by DCs. Resting iNKT cells express the IL-12 receptor, with its expression intensifying in response to dendritic cell-derived IL-12 (93, 94). This interaction cascade leads to NK cell transactivation (95), heightened responses to protein antigens by MHC-restricted CD4+ and CD8+ T cells (96), and the licensing of dendritic cell cross-presentation (97).
These bidirectional interactions highlight the cooperative synergy between iNKT cells and dendritic cells, amplifying innate and adaptive immune responses. The ability of activated iNKT cells to facilitate adaptive T cell responses holds significant clinical implications, underscoring the importance of understanding these intricate immunological mechanisms for therapeutic advancements.
5.2 Interactions with macrophages
The intricate communication between iNKT cells and macrophages is orchestrated through multiple molecular interactions. The semi-invariant TCR of iNKT cells engages with lipid-loaded CD1d molecules on macrophages (98). This interaction is complemented by co-stimulatory signals mediated through CD40-CD40l and CD80/CD86-CD28 axes (94, 99). Additionally, both cell types secrete a spectrum of pro- and anti-inflammatory cytokines, further enhancing their communication network. In the liver, Kupffer cells, specialized macrophages, are pivotal for iNKT cell activation and clearance of pathogens, exemplified during B. burgdorferi infection, a microorganism producing an α-linked lipid antigen recognized by iNKT cells (100). Lymph node macrophages could also mediate iNKT cell activation. For example, CD169+ subcapsular sinus macrophages present lipids derived from particulate antigens to iNKT cells in the paracortex (101). Thymic macrophages play essential roles in activating and shaping effector functions of thymic iNKT cells (102), demonstrated by the decrease in NKT2 abundance and IL-4 production upon deficiency of thymic F4/80+ Mertk + macrophages or abrogation of CD1d expression in thymic CD11c + cells (103). In the intestine, macrophages derived from murine embryonic and non-bone marrow sources influence the local establishment of iNKT cells in the colon during early life, impacting susceptibility or resistance to iNKT cell-associated mucosal disorders in later stages (104).
On the flip side, activated iNKT cells exert regulatory control over macrophages. In the lean state, iNKT cells aid in dampening adipose inflammation by polarizing macrophages towards an M2 phenotype, a regulatory function impaired in obesity (105, 106). Moreover, iNKT cells modulate tumor growth, partly by altering the phenotype of tumor-associated macrophages, a cell population crucial for the growth of certain neoplasms (107, 108). This bidirectional crosstalk between iNKT cells and macrophages underscores their intricate regulatory roles in immune responses, inflammation, and disease progression.
5.3 Interactions with B cells
iNKT cells play a crucial role in regulating B cell functions through cytokine release and CD40 ligand signaling (102, 109). Notably, all B cells express CD1d, with marginal zone B cells (MZBs) in both mice and humans showing particularly high CD1d expression (110). B cells can present foreign or self-lipids on CD1d following B cell receptor-mediated uptake. iNKT cells offer cognate B cell help when their stimulatory lipid antigen is linked to a specific B cell epitope (111). This interaction facilitates the internalization of the antigen complex, enabling the presentation of the stimulatory lipid by CD1d on the same B cell. Additionally, in an inflammatory environment, iNKT cells can provide non-cognate B cell help (112). For instance, co-administration of αGalCer with a protein antigen allows iNKT cells to recognize lipids separately from B cell antigen recognition, ensuring efficient B cell help.
Moreover, iNKT cells contribute to protection against autoimmunity by supporting regulatory B cell (Breg) responses. Activation of iNKT cells by αGalCer expands innate IL-10 producing MZBs, which suppress autoimmunity through IL-10 secretion (113, 114). In a reciprocal manner, Bregs induce iNKT cells to produce IFNγ and modulate cytokine production, reducing Th1 and Th17 responses and consequently ameliorating experimental arthritis (115). The essential role of B cell presentation in iNKT cell regulatory responses is highlighted by studies in mice lacking CD1d on B cells, which exhibited exacerbated arthritis, emphasizing the contribution of activated B cells in engaging the iNKT regulatory pathway. Human studies corroborate these findings, underscoring the clinical relevance of these regulatory interactions (116).
5.4 Interactions with neutrophils
In certain infections, iNKT cells recruit neutrophils to infected tissues, a process facilitated by the secretion of chemokines like CXCL2 (117, 118). Moreover, iNKT cells have demonstrated their regulatory function in neutrophil recruitment during events such as ischemia–reperfusion injury and exposure to ozone (119). Additionally, activated iNKT cells exhibit rapid release of IL-4, which enhances neutrophil survival and contributes to hepatitis (120). Paradoxically, these activated iNKT cells also produce IFN-γ in a sequential manner, initiating a negative feedback loop that ameliorates iNKT hepatitis by inducing neutrophil apoptosis. Conversely, heightened concentrations of neutrophils suppress the iNKT cell response in both mice and humans (121). Peripheral Vα14iNKT cells from mice with spontaneous neutrophilia exhibited diminished cytokine production in response to the model iNKT cell antigen αGalCer. Furthermore, these cells displayed lower expression levels of the transcription factors T-bet and GATA3 compared to wild-type controls. Similarly, iNKT cells from the human peritoneal cavity exhibited reduced transcription factor levels during neutrophilic peritonitis, underscoring the intricate interplay between iNKT cells and neutrophils in the immune response (121).
5.5 iNKT cells as the mediator of adaptive and innate immune response
The immune system safeguards the organism by detecting and regulating various danger signals, whether originating from within the body or from external sources. This intricate defense mechanism requires a harmonious coordination between the innate and adaptive immune responses, with each equipped to protect the organism in its unique way. iNKT cells, a subset of innate-like T cells, serve as vital mediators bridging the innate and adaptive arms of the immune system. iNKT cells have features of both T cells and Natural Killer (NK) cells as they express an invariant TCR that can interact with the CD1d receptor, as well as NK1.1 and Ly49 receptors (56).
This unique feature allows iNKT cells to transcend the conventional boundaries between innate and adaptive responses due to their diverse surface marker expression. Furthermore, iNKT cells express a variety of receptors, including cytokine receptors such as IL-12R (122), as well as modulatory molecules like PD-1 and CD40l (123, 124). Additionally, they harbor cytotoxic molecules such as granzymes and perforins, providing them with a wide array of response capabilities tailored to specific contexts. iNKT cells play pivotal roles in modulating both innate and adaptive immune responses. They can activate or inhibit various cell populations on either side of the immune spectrum. Moreover, iNKT cells secrete distinct sets of cytokines and induce the proliferation of different subsets of cells, showcasing their remarkable versatility and significance in orchestrating the immune defense mechanisms.
6 iNKT cells and immune dysregulation in hematological cancers
Increased iNKT cells are found in various infectious diseases (1). Rarely, other pathological processes result in increased iNKT cells except in sickle cell crises (125, 126). Decreased iNKT cells correlate with the severity of several autoimmune diseases, cancer and GVHD (127–130). Of interest, decreased iNKT cells are associated with the poor outcome of bone marrow transplant (131), including the severity of GVHD although it is difficult to draw a conclusion whether the changes in number of iNKT cells are the cause or the consequence of these clinical settings (1).
From the perspective of tumor immunology, iNKT cells eliminate cancer by two main mechanisms (Figures 1B). The first mechanism is to directly recognize and eliminate cancer cells harboring CD1d via perforin/ granzyme, TNFα, TRAIL dependent apoptosis, FasL dependent apoptosis, or B cell mediated cytolysis (2, 39, 40, 66, 132–135). Particularly, CD1d is more commonly expressed in hematological malignancies as compared to non-hematological malignancies (39, 107, 136, 137). However, CD1d expression can be lost or diminished during disease progression (138, 139). This may have an unfavorable impact on tumor control. The other main mechanism that iNKT cells use to control tumor is indirectly crosstalk with other immune cells in the tumor microenvironment (TME) (2, 140). iNKT cells can promote dendritic cell maturation (1). iNKT cells can also secrete IL-12 which activates anti-tumor CD4+ and CD8+ T cells (1). Ultimately, iNKT cells can modulate myelomonocytic populations of tumor microenvironment to antagonize tumor progression (1, 2). Tumor associated macrophages (TAMs) can either promote anti-tumor activity (M1) or augment immune dysregulation (M2) leading to tumor progression or metastasis (99, 141). The dysregulation of TME can accelerate disease progression, render the survival advantages for cancer cells such as angiogenesis, invasion or proliferation, and interfere with or even negate therapeutic effect of cancer treatment including immunotherapy (2). The balance between tumor infiltrating T cells along with NK cells and immunosuppressing Treg shapes the outcome of immunologic interaction between immune cells and cancer cells (142). More importantly, 50% of tumor mass consists of tumor associated leukocytes such as tumor associated neutrophils (TANs), and tumor infiltrating myeloid-derived suppressor cells (MDSC) (143). iNKT cells can selectively eliminate CD1d expressing M2 like macrophages in a transgenic mouse model of CLL that delayed disease progression and improved survival (141, 144). On the contrary, tumors can evade immune response by upregulating the inhibitory NK receptor Ly49C/F/H/I resulting in unresponsive iNKT cells in murine prostate cancer model (145). The coadministration of IL-12 and α-GalCer can overcome this inhibitory signal (2). The upregulation of PD-1 on tumor unresponsive iNKT cells could also be thwarted by PD-1 or PD-L1 blockade. The coadministration of PD-1 or PD-L1 blockade along with iNKT agonist like Threitolceremide-6 is under investigation in the clinic for the treatment of patients with non-small cell lung carcinoma and melanoma (32). The PD1-CD28 CAR containing extracellular domain of PD1 fused to the intracellular co-stimulatory CD28 could also render the higher antitumor activity against PDLI+ lymphoma cells (146). Binding this CAR and its PDL1 transmits activating signals instead of inhibitory signals in lymphoma (146). Similar strategies addressing immune dysregulation associated with TME can also be potentially investigated in hematological malignancies to enhance anti-tumor activity.
iNKT cell therapy has been explored in the context of several autoimmune diseases. One aspect of potential therapeutic applications of iNKT based cell therapy is to utilize iNKT cells or engineered iNKT cells as either a preventive or treatment modality of graft-vs.-host disease (GVHD)—a life-threatening immunological complication that can occur after hematopoietic stem cell transplants (147). Donor derived T cells recognize and target recipient tissues, resulting in alloreactivity, tissue injury and destruction (147). Pro-inflammatory cytokines such as interferon-gamma (IFN-gamma) and tumor necrosis factor-alpha (TNF-alpha) aggravate GVHD whereas interleukin-4 (IL-4) and interleukin-10, which are anti-inflammatory cytokines suppress GVHD. Treg suppresses GVHD by producing interleukin-10 and transforming growth factor beta which can abrogate the activation and proliferation of T cells (148, 149). More importantly, there is growing evidence supporting the suppression or prevention of GVHD by iNKT cells through the expansion of Treg (150). The adoptive transfer of iNKT cells was 10–50x more potent than Treg in protecting mice from lethal GVHD in an identical model across major histocompatibility barriers (3). Further, the adoptive transfer of iNKT cells was effective in reducing pathology in a murine model of chronic GVHD (151). As such, iNKT based cell therapy can be potentially used to treat hematological malignancies and prevent or even treat GVHD simultaneously (152).
7 Potential clinical applications of iNKT cell therapy in hematological cancers
7.1 Dendritic cell vaccine (DC vaccine) and tumor-based vaccine
To overcome the limited therapeutic efficacy of administration of only α-GalCer due to anergy and unresponsive iNKT cells, the ex vivo stimulation and α-GalCer pulsed DC with or without tumor cells have been explored in several hematologic malignancies including multiple myeloma and lymphoma in murine models (152–156). This strategy improved survival and in vivo persistence of iNKT cells in a murine model. However, limited clinical benefits were observed in a clinical study (153). The administration of α-GalCer loaded tumor vaccines improved survival in murine models of B cell lymphoma, acute myeloid leukemia and multiple myeloma (153, 157–161). In addition, the tumor vaccine given after chemotherapy prevented the relapse of leukemia (161). Ultimately, clinical trials are required to endorse the clinical efficacy and validity of this approach.
7.2 Engineered iNKT cells utilizing chimeric antigen receptors (CAR)
The utilization of adoptive transfer of in vitro expanded iNKT cells has been explored in both murine models and patients with hematological malignancies Tables 2, 3 (162–170) Figure 2 given the fact that it is feasible to expand iNKT cells in vitro and iNKT cells have inherent antitumor activity against CD1d cancer cells as well as immunosuppressing immune cells such as macrophages in TME (2, 4, 5, 140). Interestingly, Watarai and colleagues developed induced-pluripotent stem cells or iPSC—derived iNKT cells which retained the biological properties and their antitumor activity (171). Human CD34+ HSC derived iNKT (HSC-iNKT) cells also maintained their biological properties (172). In addition, these HSC-iNKT cells could protect against multiple myeloma and melanoma that expressed CD1d (172). Further investigation in clinical trials is required to confirm its clinical application which is poised to begin in 2024. iNKT cells have some unique beneficial biological properties which make them the ideal candidate for adoptive cell transfer approach to treat cancer: (1) they inhibit immunosuppressive myelomonocytic cells in TME via CD1d- cognate recognition (2), (2) the suppression of inhibitory signals from TME, and elimination of CD1d harboring cancer cells along with specific antitumor activity through CAR signaling likely to enhance therapeutic efficacy of CAR-iNKT cells through these synergistic mechanisms (2, 140). Rotolo and colleagues demonstrated that CAR iNKT cells against CD19 could exert better tumor control as compared to CAR T cells against CD19 through the synergistic interaction with CD1d and CD19 on lymphoma (173). In addition, CAR iNKT cells directed against CD19 could render the control of brain lymphomas (173). To maximize antitumor activity of iNKT cells, the adoptive transfer of CAR-iNKT has been investigated in several preclinical and phase I clinical studies (5, 7, 8, 173) (Figure 2).
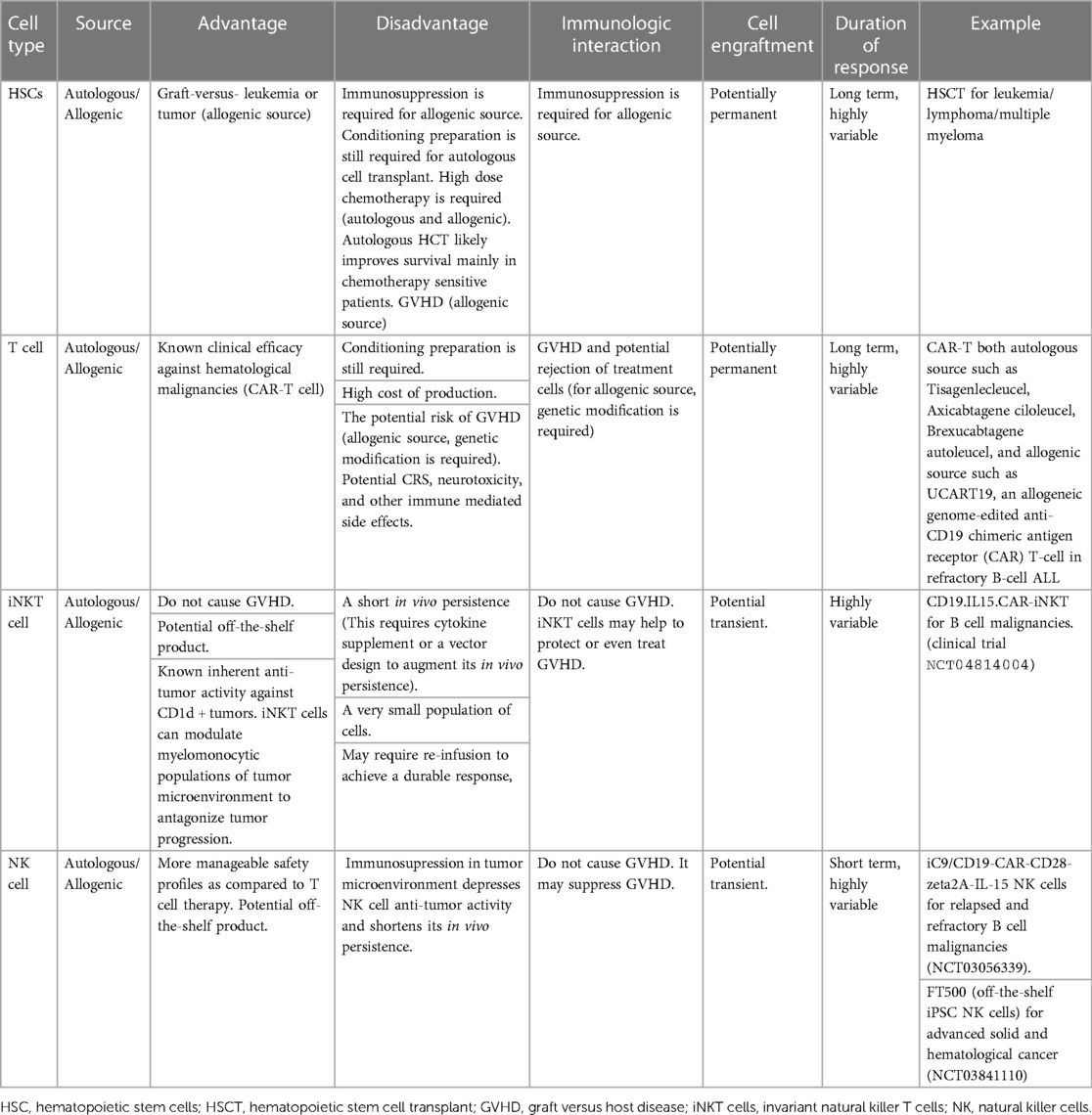
Table 2. The advantages and disadvantages of utilization of various sources of cell-based therapies in hematological malignancies.
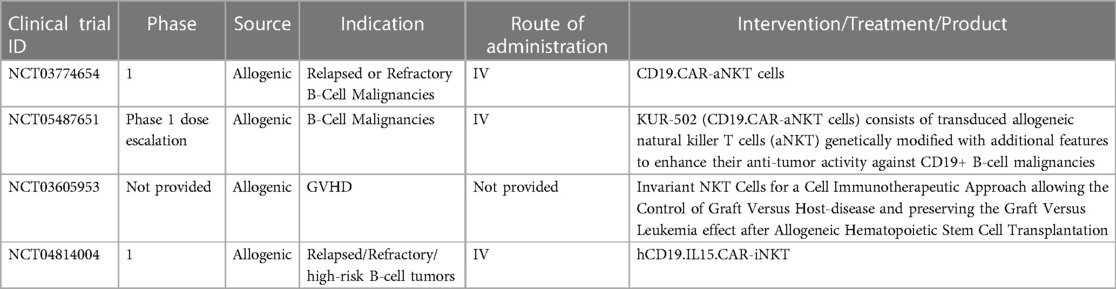
Table 3. Current clinical trials of iNKT/ engineered iNKT/CAR-iNKT for hematological malignancies and related diseases.
Our group and others have demonstrated that the adoptive cell therapy approach utilizing CAR-iNKT cells could render survival benefits in murine models in hematological malignancies such as B cell lymphoma and multiple myeloma (10, 174). Simonetta and colleagues demonstrated that allogenic CAR iNKT could induce host CD8 T cell cross priming (10). As a result, this rendered durable antitumor activity beyond the physical presence of transferred CAR iNKT cells and this also improved tumor control in murine model (10).
CD62l+ CAR iNKT cells appeared to have prolonged in vivo survival and they were highly effective against B cell lymphoma, and neuroblastoma in NSG mice (5). Further, Ngai and colleagues found that the administration of IL-21 could protect and enhance CD62+ CAR iNKT's antitumor activity and increase survival in a murine model of lymphoma (6). CD4+ T cells and iNKT cells produce IL-21 (175, 176). IL-21 can abrogate apoptosis by increasing the expression of BCL-2. This improves survival of iNKT cells. IL21 also binds with motif YXXQ on IL-21R, then activates STAT3 pathway resulting in generating more memory cells and effector cells (6, 175, 177). Several studies demonstrated that CAR iNKT cells were effective against multiple myeloma and they prolonged survival in murine models. O'Neal and colleague showed that the co-administration of long-acting IL-7: rhIL-7-hyFc and CAR iNKT targeting BMCA improved anti-myeloma activity in a preclinical study (178). CAR iNKT cells were demonstrated to be safe and able to infiltrate tumors in patients with neuroblastoma in the recent update of a phase I clinical trial (8). In this clinical trial, autologous anti-GD2 CAR iNKT cell therapy against neuroblastoma exploiting the co-expression of GD2 and IL15 appeared to be safe with acceptable adverse events (8). Like fourth generation CAR T cells, CAR constructs can be designed to secrete additional cytokine to enhance antitumor activity, and in this clinical trial the co-expressing IL-15 promoted the development, expansion, and in vivo persistence of central memory iNKT cells (8, 163). The CAR-iNKT cell therapy in this clinical trial showed anti-tumor activity with two partial responses (2/12) and one complete response (1/12) in relapsed or refractory neuroblastoma (8). iNKT cells can also be engineered to acquire a second specific antigen by expressing recombinant TCRS that recognize tumor associated antigens, for example, human iNKT cells could be engineered with TCR specific for an HLA-2-restricted peptide epitope derived from melanoma such as MART or PRAME (2, 179). This iNKT-TCR demonstrated HLA-restricted antitumor activity in a xenogenic murine model (179). To further leverage the unique immunotherapeutic application of CAR-iNKT cells in hematological malignancies, a novel universal CAR platform can also be designed (Figure 3). In general, this universal CAR-iNKT cell system consists of the flexible antigen binding component (e.g., svFC, monoclonal antibody or tumor specific ligand), and the iNKT cell signaling component (180). This off-the-shelf, universal CAR platform is flexible, scalable, and amendable to diverse and multiple antigen specificity. The current FDA approved CAR T cells for hematological malignancies are autologous CAR T cells and CAR iNKT cells may be particularly attractive as an allogeneic product.
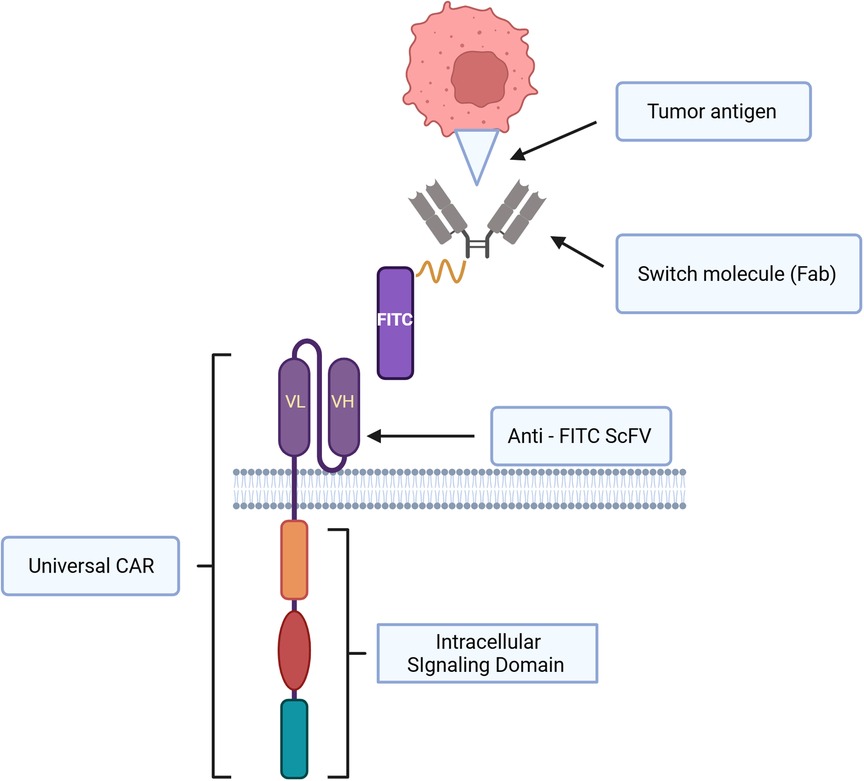
Figure 3. Schematic representation of universal CAR system design and immunological synapse of universal CAR, switch molecule and tumor antigen. This universal CAR-iNKT like CAR-T cell system consists of the flexible antigen binding component (e.g. svFC, monoclonal antibody or tumor specific ligand), and the iNKT cell signaling component.
In the context of stem cell transplantation, iNKT cells mitigate the severity of GVHD and they maintain antitumor activity (151, 152). No evidence of GVHD was demonstrated in several murine models of hematological malignancies (152). Of interest, further modification to lower MHC expression on iNKT cells may be required to prevent host immunity from rejecting adoptive transferred allogenic iNKT cells, and this has been investigated in clinical trial of B cell lymphoma (2). Furthermore, the complete absence in MHC expression on allogenic gene edited iNKT cells may provoke “missing self” response by NK cell, and this will compromise the survival of allogenic gene edited iNKT cells (2). Hence, allogenic gene edited iNKT cells may require to be engineered to express ligands for the NK inhibitory receptors or they may require modification of CD47/SIRPα pathway to protect them from phagocytosis (2).
Like CAR T cell therapy—one of the historic successful trailblazers in cell therapy for hematological malignancies leading to the first FDA approved CAR T cell for precursor B cell acute lymphoblastic leukemia (pre-B ALL) (181) then followed by a number of other FDA approved treatments for pre-B ALL, B cell lymphoma, and multiple myeloma (182), CAR-iNKT cell based therapy can be adopted to enhance antitumor activity, improve survival, mitigate serious or decrease adverse reactions (2, 4, 8, 40, 180). We foresee that the advancement of vector design and refined treatment schedule to improve CAR iNKT in vivo persistence, and functionality resulting in better clinical outcomes. Further investigation to combine CAR iNKT based cell therapy with other treatment modalities to leverage its therapeutic efficacy may be warranted. For example, the combination of CAR iNKT cell-based therapy with traditional hematopoietic stem cell transplantation to achieve synergistic antitumor activity and minimize or prevent immunological complications such as GVHD (2, 152, 183). The combination of CAR iNKT cells with immune checkpoint blockade or small molecule such as ibrutinib to mitigate iNKT cell dysfunction and enhance anti-tumor activity like in CAR T cell therapy may increase therapeutic efficacy (163, 184, 185). The expected adverse effects such as cytokine release syndrome, neurotoxicity, or other unforeseen adverse effects in CAR -iNKT cells-based therapy and confirmation of their clinical application require additional further investigations in clinical trials.
7.3 iNKT cell therapy to prevent and treat GVHD
Graft-vs.-host disease (GVHD) is a major concern leading to high morbidity and mortality in patients undergone allogeneic hematopoietic cell transplantation (HCT) (186). The infused allografts during HCT contain mature CD4+ and CD8+ αβ T cells which establish hematopoietic engraftment, reconstitute T cells immunity, and induce graft-vs.-tumor (GVT) effect that is key for elimination of the malignant cells. However, these donor cells may recognize the host as “foreign” and invade the host tissues causing GVHD manifested as acute damages to the skin, gastrointestinal tract, and liver, termed acute GVHD, or as prolonged inflammation and immune dysregulation that could affect limited organs or be widespread, referred to as chronic GVHD (187–189). Immunosuppressive drugs are primarily employed as preventive or therapeutic measures to manage the complications presented by GVHD. But these are typically associated with severe toxic complications while do not always lead to complete resolution of GVHD manifestations (190–192). Therefore, a better understanding of the underlying mechanisms of immune dysregulation is necessary to overcome GVHD while maintaining appropriate GVT response, and thereby improve the outcome following HCT.
iNKT cells are one of the key immune regulatory cell populations extensively studied for their role in protection from GVHD. Murine studies of allogenic HCT by the group of Strober et al. demonstrated key role of host NKT cells in protection against GVHD following nonmyeloablative conditioning with total lymphoid irradiation (TLI) and anti-thymocyte serum (ATS) (193, 194). Our group employed adoptive transfer of CD4+ iNKT cells to major histocompatibility complex (MHC)-mismatched murine models of GVHD to demonstrate protective effects of donor-type (3, 195) as well as third-party (150) iNKT cells against GVHD. Furthermore, our group and others showed that specific activation of host iNKT cells using invariant T cell receptor (iTCR) stimulator a-galactosylceramide (a-GalCer) leads to mitigation of GVHD (30, 196, 197). Recently, we isolated highly purified murine iNKT sublineages: iNKT1, iNKT2 and iNKT17, and demonstrated antitumor function associated with iNKT1 while GVHD suppression function associated with iNKT2 and iNKT17 (42). These studies suggest iNKT cells enact key roles in the mechanisms underlying immune regulation leading to GVHD suppression. For the GVHD suppressive function of iNKT cells, their interplay with other immune regulatory cell populations is crucial. Multiple studies have provided compelling evidence that iNKT-induced interleukin-4 (IL4) dependent regulatory T (Treg) cell expansion drives inhibition of GVHD response in HCT (3, 30, 150, 198–201). Treg cells have potent immune suppressive function in allogeneic HCT with demonstrated capability to suppress GVHD while preserving the GVT effect (202–204). Further insights into the underlying mechanisms of tolerogenic immune pathways driving anti-GVHD response in HCT are provided by numerous murine studies implicating myeloid-derived suppressor cells (MDSCs) and CD8+ dendritic cells (DCs) in the interplay between Treg and iNKT cells (150, 205–207).
Various clinical studies validate iNKT cells being associated with GVHD suppressive function. Higher number of iNKT cells in the graft for allogeneic HCT is reported to be associated with lower risk of acute GVHD (128, 208, 209). Following allogeneic HCT, iNKT cells have widely been reported to persist and rapidly recover which is correlated with protection against GVHD. Post-HCT recovery analysis of iNKT cells demonstrated correlation between increased number of iNKT cells and protection against GVHD resulting in improved GVHD relapse free survival (129, 210). Likewise, in patients that received allogeneic HCT with total lymphoid irradiation (TLI) and anti-thymocyte globulin (ATG) conditioning, we revealed increased persistence of iNKT compared to CD4+ and CD8+ T cells post-TLI/ATG and protection against acute GVHD in patients with residual iNKT cells than those without detectable iNKT cells (211). To summarize, these studies suggest a key role of iNKT cells in suppression of GVHD in humans, and therefore attempts have been made to expand iNKT cells in HCT patients. A phase 2A study utilized RGI-2001, a liposomal formulation of a-GalCer to activate and expand iNKT cells which induced Treg expansion and reduced the incidence of grade 2 to 4 GVHD in the HCT patients (212). A recent study sheds light on a probable underlying mechanism of GVHD prevention by human iNKT cells where human culture-expanded iNKT cells were shown to induce DC apoptosis, and thereby impair the activation and proliferation of allo-reactive T cells (213). One of the key considerations in this regard could be the heterogeneity of iNKT cells, as evidenced by our own murine studies (42). Heterogeneity in human iNKT cells has been well characterized by a recent study where two iNKT phenotypes have been identified which correlated with a T helper 1 function and enhanced cytotoxic function (214). Identification of human iNKT subsets with Th2 function and ensuring their preservation during in vitro expansion could be crucial in clinical trials using iNKT cells. Despite a multitude of questions, the therapeutic potential of iNKT cells appear promising in overcoming a major limitation of HCT by achieving long-lasting suppression of GVHD.
8 Concluding remarks and future directions
The roles of iNKT cells in the specific immune mediated disease aspects are emerging, and the use of iNKT cells as a platform for cell therapy is beginning to be appreciated, with potential impact and roles in a wide range of diseases such as autoimmune diseases, infection, and especially cancer. CD1d restriction defines this cell population which is ubiquitously expressed and not polymorphic. In addition, CD1d is commonly expressed in hematological malignancies. Hence, iNKT cells are a potential candidate for “off-the-shelf” allogeneic cell therapy for hematological malignancies. iNKT cells have some unique beneficial biological properties which make them the ideal candidate for adoptive cell transfer to treat cancer such as the synergistic mechanism between the inherent anti-tumor activity against CD1d harboring cancer cells and the induction of host CD8+ T cell responses. The isolation, enrichment, and expansion of iNKT cells in vitro are feasible and iNKT cells can be readily transduced with viral approaches to express a CAR and as such are amendable as the “off-the-shelf” therapeutic agents for large-scale clinical application. We anticipate that the improved vector design and other strategies such as combinatorial treatment with small molecules or immune blockade inhibitor could improve CAR iNKT in vivo persistence, and its functionality, leverage its anti-tumor activity along with the abatement of iNKT cell dysfunction or exhaustion. These unique and beneficial biological properties along with several strategies adopted from development of CAR T cell therapy to address shortcomings make iNKT cell therapy an attractive novel therapeutic approach to address immune dysregulation and disease relapse in hematological malignancies.
Author contributions
CB: Conceptualization, Writing – original draft, Writing – review & editing. HY: Writing – original draft, Writing – review & editing. AR: Writing – original draft, Writing – review & editing. BG: Supervision, Writing – original draft, Writing – review & editing. JB: Supervision, Writing – review & editing. RN: Supervision, Writing – review & editing.
Funding
The author(s) declare that financial support was received for the research, authorship, and/or publication of this article.
Postdoctoral research training T32 fellowship with support from Ruth L. Kirschstein Institutional National Research Service Award to CB and PPG CA49605 to RN.
Acknowledgments
All figures were created with BioRender.com
Conflict of interest
The authors declare that the research was conducted in the absence of any commercial or financial relationships that could be construed as a potential conflict of interest.
Publisher's note
All claims expressed in this article are solely those of the authors and do not necessarily represent those of their affiliated organizations, or those of the publisher, the editors and the reviewers. Any product that may be evaluated in this article, or claim that may be made by its manufacturer, is not guaranteed or endorsed by the publisher.
References
1. Brennan PJ, Brigl M, Brenner MB. Invariant natural killer T cells: an innate activation scheme linked to diverse effector functions. Nat Rev Immunol. (2013) 13:101–17. doi: 10.1038/nri3369
2. Delfanti G, Dellabona P, Casorati G, Fedeli M. Adoptive immunotherapy with engineered iNKT cells to target cancer cells and the suppressive microenvironment. Front Med (Lausanne). (2022) 9:1–18. doi: 10.3389/fmed.2022.897750
3. Schneidawind D, Pierini A, Alvarez M, Pan Y, Baker J, Buechele C, et al. CD4 + invariant natural killer T cells protect from murine GVHD lethality through expansion of donor CD4 + CD25 + FoxP3 + regulatory T cells. Blood. (2014) 124:3320–8. doi: 10.1182/blood-2014-05-576017
4. Liu Y, Wang G, Chai D, Dang Y, Zheng J, Li H. iNKT: a new avenue for CAR-based cancer immunotherapy. Transl Oncol. (2022) 17:101342. doi: 10.1016/j.tranon.2022.101342
5. Tian G, Courtney AN, Jena B, Heczey A, Liu D, Marinova E, et al. CD62l+ NKT cells have prolonged persistence and antitumor activity in vivo. J Clin Invest. (2016) 126:2341–55. doi: 10.1172/JCI83476
6. Ngai H, Tian G, Courtney AN, Ravari SB, Guo L, Liu B, et al. IL-21 selectively protects CD62l+ NKT cells and enhances their effector functions for adoptive immunotherapy. J Immunol. (2018) 201:2141–53. doi: 10.4049/jimmunol.1800429
7. Heczey A, Liu D, Tian G, Courtney AN, Wei J, Marinova E, et al. Invariant NKT cells with chimeric antigen receptor provide a novel platform for safe and effective cancer immunotherapy. Blood. (2014) 124:2824–33. doi: 10.1182/blood-2013-11-541235
8. Heczey A, Xu X, Courtney AN, Tian G, Barragan GA, Guo L, et al. Anti-GD2 CAR-NKT cells in relapsed or refractory neuroblastoma: updated phase 1 trial interim results. Nat Med. (2023) 29:1379–88. doi: 10.1038/s41591-023-02363-y
9. Aoki T, Takami M, Takatani T, Motoyoshi K, Ishii A, Hara A, et al. Activated invariant natural killer T cells directly recognize leukemia cells in a CD1d-independent manner. Cancer Sci. (2020) 111:2223–33. doi: 10.1111/cas.14428
10. Simonetta F, Lohmeyer JK, Hirai T, Maas-Bauer K, Alvarez M, Wenokur AS, et al. Allogeneic CAR-invariant natural killer T cells exert potent antitumor effects through host CD8 T cell cross-priming. Clin Cancer Res. (2021) 27:6054–64. doi: 10.1158/1078-0432.CCR-21-1329
11. Wolf BJ, Choi JE, Exley MA. Novel approaches to exploiting invariant NKT cells in cancer immunotherapy. Front Immunol. (2018) 9:384. doi: 10.3389/fimmu.2018.00384
12. Brossay L, Chioda M, Burdin N, Koezuka Y, Casorati G, Dellabona P, et al. CD1d-mediated recognition of an α-galactosylceramide by natural killer T cells is highly conserved through mammalian evolution. J Exp Med. (1998) 188:1521–8. doi: 10.1084/jem.188.8.1521
13. Matsuda JL, Naidenko OV, Gapin L, Nakayama T, Taniguchi M, Wang CR, et al. Tracking the response of natural killer T cells to a glycolipid antigen using CD1d tetramers. J Exp Med. (2000) 192:741–54. doi: 10.1084/jem.192.5.741
14. Benlagha K, Weiss A, Beavis A, Teyton L, Bendelac A. In vivo identification of glycolipid antigen-specific T cells using fluorescent CD1d tetramers. J Exp Med. (2000) 191:1895–903. doi: 10.1084/jem.191.11.1895
15. Dascher CC, Brenner MB. Evolutionary constraints on CD1 structure: insights from comparative genomic analysis. Trends Immunol. (2003) 24:412–8. doi: 10.1016/S1471-4906(03)00179-0
16. Harsha Krovi S, Zhang J, Michaels-Foster MJ, Brunetti T, Loh L, Scott-Browne J, et al. Thymic iNKT single cell analyses unmask the common developmental program of mouse innate T cells. Nat Commun. (2020) 11:6238. doi: 10.1038/s41467-020-20073-8
17. Yuan J, Nguyen CK, Liu X, Kanellopoulou C, Muljo SA. Lin28b reprograms adult bone marrow hematopoietic progenitors to mediate fetal-like lymphopoiesis. Science. (2012) 335:1195–200. doi: 10.1126/science.1216557
18. Doisne JM, Becourt C, Amniai L, Duarte N, Le Luduec JB, Eberl G, et al. Skin and peripheral lymph node invariant NKT cells are mainly retinoic acid receptor-related orphan receptor (gamma)t+ and respond preferentially under inflammatory conditions. J Immunol. (2009) 183:2142–9. doi: 10.4049/jimmunol.0901059
19. Gumperz JE, Miyake S, Yamamura T, Brenner MB. Functionally distinct subsets of CD1d-restricted natural killer T cells revealed by CD1d tetramer staining. J Exp Med. (2002) 195:625–36. doi: 10.1084/jem.20011786
20. Lee PT, Benlagha K, Teyton L, Bendelac A. Distinct functional lineages of human V(alpha)24 natural killer T cells. J Exp Med. (2002) 195:637–41. doi: 10.1084/jem.20011908
21. Montoya CJ, Pollard D, Martinson J, Kumari K, Wasserfall C, Mulder CB, et al. Characterization of human invariant natural killer T subsets in health and disease using a novel invariant natural killer T cell-clonotypic monoclonal antibody, 6B11. Immunology. (2007) 122:1–14. doi: 10.1111/j.1365-2567.2007.02647.x
22. Lynch L, O'Shea D, Winter DC, Geoghegan J, Doherty DG, O'Farrelly C. Invariant NKT cells and CD1d+ cells amass in human omentum and are depleted in patients with cancer and obesity. Eur J Immunol. (2009) 39:1893–901. doi: 10.1002/eji.200939349
23. Kinjo Y, Wu D, Kim G, Xing GW, Poles MA, Ho DD, et al. Recognition of bacterial glycosphingolipids by natural killer T cells. Nature. (2005) 434:520–5. doi: 10.1038/nature03407
24. Kinjo Y, Tupin E, Wu D, Fujio M, Garcia-Navarro R, Benhnia MREI, et al. Natural killer T cells recognize diacylglycerol antigens from pathogenic bacteria. Nat Immunol. (2006) 7:978–86. doi: 10.1038/ni1380
26. Mattner J, DeBord KL, Ismail N, Goff RD, Cantu C, Zhou D, et al. Exogenous and endogenous glycolipid antigens activate NKT cells during microbial infections. Nature. (2005) 434:525–9. doi: 10.1038/nature03408
27. Morita M, Motoki K, Akimoto K, Natori T, Sakai T, Sawa E, et al. Structure-activity relationship of.alpha.-galactosylceramides against B16-bearing mice. J Med Chem. (1995) 38:2176–87. doi: 10.1021/jm00012a018
28. Schneiders FL, Scheper RJ, von Blomberg BME, Woltman AM, Janssen HLA, van den Eertwegh AJM, et al. Clinical experience with α-galactosylceramide (KRN7000) in patients with advanced cancer and chronic hepatitis B/C infection. Clin Immunol. (2011) 140:130–41. doi: 10.1016/j.clim.2010.11.010
29. Giaccone G, Punt CJA, Ando Y, Ruijter R, Nishi N, Peters M, et al. A phase I study of the natural killer T-cell ligand alpha-galactosylceramide (KRN7000) in patients with solid tumors. Clin Cancer Res. (2002) 8:3702–9.12473579
30. Hirai T, Lin PY, Simonetta F, Maas-Bauer K, Turkoz M, Mavers M, et al. Activation of natural killer T cells enhances the function of regulatory T-cell therapy in suppressing murine GVHD. Blood Advances. (2021) 5:2528–38. doi: 10.1182/bloodadvances.2020003272
31. Jukes J, Gileadi U, Ghadbane H, Yu T, Shepherd D, Cox LR, et al. Non-glycosidic compounds can stimulate both human and mouse i NKT cells. Eur J Immunol. (2016) 46:1224–34. doi: 10.1002/eji.201546114
32. Coupe N, Walters IB, Kramer RA, Gileadi U, Middleton MR. A phase 1 first-in-human dose finding/randomized phase 2 study of IMM60 and pembrolizumab (PEM) in advanced melanoma and non–small cell lung cancer (NSCLC; IMP-MEL). JCO. (2022) 40:2582–2582. doi: 10.1200/JCO.2022.40.16_suppl.2582
33. iOx Therapeutics. IMPORT-201: A Phase 1 First-in-Human Dose Finding/Randomized Phase 2 Study of IMM60 and Pembrolizumab for Advanced Melanoma and Metastatic NSCLC (2023). Available online at: https://clinicaltrials.gov/study/NCT05709821 (Accessed March 24, 2024).
34. Chaudhry MS, Karadimitris A. Role and regulation of CD1d in normal and pathological B cells. J Immunol. (2014) 193:4761–8. doi: 10.4049/jimmunol.1401805
35. CD1D Protein Expression Summary—The Human Protein Atlas. Available online at: https://www.proteinatlas.org/ENSG00000158473-CD1D (Accessed March 24, 2024).
36. Exley M, Garcia J, Wilson SB, Spada F, Gerdes D, Tahir SMA, et al. CD1d Structure and regulation on human thymocytes, peripheral blood T cells, B cells and monocytes. Immunology. (2000) 100:37–47. doi: 10.1046/j.1365-2567.2000.00001.x
37. Courtney AN, Tian G, Metelitsa LS. Natural killer T cells and other innate-like T lymphocytes as emerging platforms for allogeneic cancer cell therapy. Blood. (2023) 141:869–76. doi: 10.1182/blood.2022016201
38. Kotsianidis I, Nakou E, Spanoudakis E, Bouchliou I, Moustakidis E, Miltiades P, et al. The diagnostic value of CD1d expression in a large cohort of patients with B-cell chronic lymphoproliferative disorders. Am J Clin Pathol. (2011) 136:400–8. doi: 10.1309/AJCP2F2DOXOTXHZA
39. Metelitsa LS, Weinberg KI, Emanuel PD, Seeger RC. Expression of CD1d by myelomonocytic leukemias provides a target for cytotoxic NKT cells. Leukemia. (2003) 17:1068–77. doi: 10.1038/sj.leu.2402943
40. Lam PY, Nissen MD, Mattarollo SR. Invariant natural killer T cells in immune regulation of blood cancers: harnessing their potential in immunotherapies. Front Immunol. (2017) 8:858–70.28798746
41. Hogquist K, Georgiev H. Recent advances in iNKT cell development. F1000Res. (2020) 9:1–10. doi: 10.12688/f1000research.21378.1
42. Maas-Bauer K, Lohmeyer JK, Hirai T, Ramos TL, Fazal FM, Litzenburger UM, et al. Invariant natural killer T-cell subsets have diverse graft-versus-host-disease–preventing and antitumor effects. Blood. (2021) 138:858–70. doi: 10.1182/blood.2021010887
43. Krovi SH, Gapin L. Invariant natural killer T cell subsets—more than just developmental intermediates. Front Immunol. (2018) 9:1393. doi: 10.3389/fimmu.2018.01393
44. Engel I, Seumois G, Chavez L, Samaniego-Castruita D, White B, Chawla A, et al. Innate-like functions of natural killer T cell subsets result from highly divergent gene programs. Nat Immunol. (2016) 17:728–39. doi: 10.1038/ni.3437
45. Lee YJ, Wang H, Starrett GJ, Phuong V, Jameson SC, Hogquist KA. Tissue specific distribution of iNKT cells impacts their cytokine response. Immunity. (2015) 43:566–78. doi: 10.1016/j.immuni.2015.06.025
46. Crosby CM, Kronenberg M. Tissue-specific functions of invariant natural killer T cells. Nat Rev Immunol. (2018) 18:559–74. doi: 10.1038/s41577-018-0034-2
47. Benlagha K, Wei DG, Veiga J, Teyton L, Bendelac A. Characterization of the early stages of thymic NKT cell development. J Exp Med. (2005) 202:485–92. doi: 10.1084/jem.20050456
48. Gapin L. Development of invariant natural killer T cells. Curr Opin Immunol. (2016) 39:68–74. doi: 10.1016/j.coi.2016.01.001
49. Shissler SC, Webb TJ. The ins and outs of type I iNKT cell development. Mol Immunol. (2019) 105:116–30. doi: 10.1016/j.molimm.2018.09.023
50. Bennstein SB. Unraveling natural killer T-cells development. Front Immunol. (2018) 8:1950. doi: 10.3389/fimmu.2017.01950
51. Kovalovsky D, Uche OU, Eladad S, Hobbs RM, Yi W, Alonzo E, et al. The BTB-zinc finger transcriptional regulator, PLZF, controls the development of iNKT cell effector functions. Nat Immunol. (2008) 9:1055–64. doi: 10.1038/ni.1641
52. Savage AK, Constantinides MG, Han J, Picard D, Martin E, Li B, et al. The transcription factor PLZF (Zbtb16) directs the effector program of the NKT cell lineage. Immunity. (2008) 29:391–403. doi: 10.1016/j.immuni.2008.07.011
53. Watarai H, Sekine-Kondo E, Shigeura T, Motomura Y, Yasuda T, Satoh R, et al. Development and function of invariant natural killer T cells producing TH2- and TH17-cytokines. PLoS Biol. (2012) 10:e1001255. doi: 10.1371/journal.pbio.1001255
54. Taniguchi M, Harada M, Kojo S, Nakayama T, Wakao H. The regulatory role of Vα14 NKT cells in innate and acquired immune response. Annu Rev Immunol. (2003) 21:483–513. doi: 10.1146/annurev.immunol.21.120601.141057
55. Brigl M, Brenner MB. CD1: antigen presentation and T cell function. Annu Rev Immunol. (2004) 22:817–90. doi: 10.1146/annurev.immunol.22.012703.104608
56. Godfrey DI, MacDonald HR, Kronenberg M, Smyth MJ, Kaer LV. NKT Cells: what’s in a name? Nat Rev Immunol. (2004) 4:231–7. doi: 10.1038/nri1309
57. Bendelac A, Savage PB, Teyton L. The biology of NKT cells. Annu Rev Immunol. (2007) 25:297–336. doi: 10.1146/annurev.immunol.25.022106.141711
58. Girardi E, Zajonc DM. Molecular basis of lipid antigen presentation by CD 1d and recognition by natural killer T cells. Immunol Rev. (2012) 250:167–79. doi: 10.1111/j.1600-065X.2012.01166.x
59. Zhou D, Cantu C, Sagiv Y, Schrantz N, Kulkarni AB, Qi X, et al. Editing of CD1d-bound lipid antigens by endosomal lipid transfer proteins. Science. (2004) 303:523–7. doi: 10.1126/science.1092009
60. van den Elzen P, Garg S, León L, Brigl M, Leadbetter EA, Gumperz JE, et al. Apolipoprotein-mediated pathways of lipid antigen presentation. Nature. (2005) 437:906–10. doi: 10.1038/nature04001
61. Freigang S, Zadorozhny V, McKinney MK, Krebs P, Herro R, Pawlak J, et al. Fatty acid amide hydrolase shapes NKT cell responses by influencing the serum transport of lipid antigen in mice. J Clin Invest. (2010) 120:1873–84. doi: 10.1172/JCI40451
62. Prigozy TI, Naidenko O, Qasba P, Elewaut D, Brossay L, Khurana A, et al. Glycolipid antigen processing for presentation by CD1d molecules. Science. (2001) 291:664–7. doi: 10.1126/science.291.5504.664
63. de la Salle H, Mariotti S, Angenieux C, Gilleron M, Garcia-Alles LF, Malm D, et al. Assistance of microbial glycolipid antigen processing by CD1e. Science. (2005) 310:1321–4. doi: 10.1126/science.1115301
64. Natori T, Morita M, Akimoto K, Koezuka Y. Agelasphins, novel antitumor and immunostimulatory cerebrosides from the marine sponge Agelas mauritianus. Tetrahedron. (1994) 50:2771–84. doi: 10.1016/S0040-4020(01)86991-X
65. Kawano T, Cui J, Koezuka Y, Toura I, Kaneko Y, Motoki K, et al. CD1d-restricted And TCR-mediated activation of valpha14 NKT cells by glycosylceramides. Science. (1997) 278:1626–9. doi: 10.1126/science.278.5343.1626
66. Kawano T, Cui J, Koezuka Y, Toura I, Kaneko Y, Sato H, et al. Natural killer-like nonspecific tumor cell lysis mediated by specific ligand-activated Vα14 NKT cells. Proc Natl Acad Sci U.S.A. (1998) 95:5690–3. doi: 10.1073/pnas.95.10.5690
67. Stenström M, Sköld M, Andersson Å, Cardell SL. Natural killer T-cell populations in C57BL/6 and NK1.1 congenic BALB.NK mice—a novel thymic subset defined in BALBNK mice. Immunology. (2005) 114:336–45. doi: 10.1111/j.1365-2567.2004.02111.x
68. Mujal AM, Delconte RB, Sun JC. Natural killer cells: from innate to adaptive features. Annu Rev Immunol. (2021) 39:417–47. doi: 10.1146/annurev-immunol-101819-074948
69. Matsuda JL, Gapin L, Baron JL, Sidobre S, Stetson DB, Mohrs M, et al. Mouse V alpha 14i natural killer T cells are resistant to cytokine polarization in vivo. Proc Natl Acad Sci USA. (2003) 100:8395–400. doi: 10.1073/pnas.1332805100
70. Wesley JD, Robbins SH, Sidobre S, Kronenberg M, Terrizzi S, Brossay L. Cutting edge: IFN-gamma signaling to macrophages is required for optimal Valpha14i NK T/NK cell cross-talk. J Immunol. (2005) 174:3864–8. doi: 10.4049/jimmunol.174.7.3864
71. Kronenberg M. Toward an understanding of NKT cell biology: progress and paradoxes. Annu Rev Immunol. (2005) 23:877–900. doi: 10.1146/annurev.immunol.23.021704.115742
72. Sriram V, Du W, Gervay-Hague J, Brutkiewicz RR. Cell wall glycosphingolipids of Sphingomonas paucimobilis are CD1d-specific ligands for NKT cells. Eur J Immunol. (2005) 35:1692–701. doi: 10.1002/eji.200526157
73. Fujii S, Shimizu K, Kronenberg M, Steinman RM. Prolonged IFN-γ–producing NKT response induced with α-galactosylceramide–loaded DCs. Nat Immunol. (2002) 3:867–74. doi: 10.1038/ni827
74. Fujii S-I, Shimizu K. Immune networks and therapeutic targeting of iNKT cells in cancer. Trends Immunol. (2019) 40:984–97. doi: 10.1016/j.it.2019.09.008
75. Parekh VV, Wilson MT, Olivares-Villagómez D, Singh AK, Wu L, Wang CR, et al. Glycolipid antigen induces long-term natural killer T cell anergy in mice. J Clin Invest. (2005) 115:2572–83. doi: 10.1172/JCI24762
76. Salio M, Silk JD, Yvonne Jones E, Cerundolo V. Biology of CD1- and MR1-restricted T cells. Annu Rev Immunol. (2014) 32:323–66. doi: 10.1146/annurev-immunol-032713-120243
77. Stetson DB, Mohrs M, Reinhardt RL, Baron JL, Wang ZE, Gapin L, et al. Constitutive cytokine mRNAs mark natural killer (NK) and NK T cells poised for rapid effector function. J Exp Med. (2003) 198:1069–76. doi: 10.1084/jem.20030630
78. Leite-De-Moraes MC, Hameg A, Arnould A, Machavoine F, Koezuka Y, Schneider E, et al. A distinct IL-18-induced pathway to fully activate NK T lymphocytes independently from TCR engagement. J Immunol. (1999) 163:5871–6. doi: 10.4049/jimmunol.163.11.5871
79. Anderson CK, Reilly SP, Brossay L. The invariant NKT cell response has differential signaling requirements during antigen-dependent and antigen-independent activation. J Immunol. (2021) 206:132–40. doi: 10.4049/jimmunol.2000870
80. Wesley JD, Tessmer MS, Chaukos D, Brossay L. NK cell-like behavior of valpha14i NK T cells during MCMV infection. PLoS Pathog. (2008) 4:e1000106. doi: 10.1371/journal.ppat.1000106
81. Tyznik AJ, Tupin E, Nagarajan NA, Her MJ, Benedict CA, Kronenberg M. Cutting edge: the mechanism of invariant NKT cell responses to viral danger signals. J Immunol. (2008) 181:4452–6. doi: 10.4049/jimmunol.181.7.4452
82. Exley MA, Lynch L, Varghese B, Nowak M, Alatrakchi N, Balk SP. Developing understanding of the roles of CD1d-restricted T cell subsets in cancer: reversing tumor-induced defects. Clin Immunol. (2011) 140:184–95. doi: 10.1016/j.clim.2011.04.017
83. Paget C, Bialecki E, Fontaine J, Vendeville C, Mallevaey T, Faveeuw C, et al. Role of invariant NK T lymphocytes in immune responses to CpG oligodeoxynucleotides. J Immunol. (2009) 182:1846–53. doi: 10.4049/jimmunol.0802492
84. Nagarajan NA, Kronenberg M. Invariant NKT cells amplify the innate immune response to lipopolysaccharide. J Immunol. (2007) 178:2706–13. doi: 10.4049/jimmunol.178.5.2706
85. Bourgeois E, Van LP, Samson M, Diem S, Barra A, Roga S, et al. The pro-Th2 cytokine IL-33 directly interacts with invariant NKT and NK cells to induce IFN-gamma production. Eur J Immunol. (2009) 39:1046–55. doi: 10.1002/eji.200838575
86. Smithgall MD, Comeau MR, Yoon BRP, Kaufman D, Armitage R, Smith DE. IL-33 amplifies both Th1- and Th2-type responses through its activity on human basophils, allergen-reactive Th2 cells, iNKT and NK cells. Int Immunol. (2008) 20:1019–30. doi: 10.1093/intimm/dxn060
87. Robin A, Mackowiak C, Bost R, Dujardin F, Barbarin A, Thierry A, et al. Early activation and recruitment of invariant natural killer T cells during liver ischemia-reperfusion: the major role of the alarmin interleukin-33. Front Immunol. (2023) 14:1099529. doi: 10.3389/fimmu.2023.1099529
88. Keller CW, Freigang S, Lünemann JD. Reciprocal crosstalk between dendritic cells and natural killer T cells: mechanisms and therapeutic potential. Front Immunol. (2017) 8:570. doi: 10.3389/fimmu.2017.00570
89. Barral P, Sánchez-Niño MD, Van Rooijen N, Cerundolo V, Batista FD. The location of splenic NKT cells favours their rapid activation by blood-borne antigen: NKT cells are activated outside splenic white pulp. EMBO J. (2012) 31:2378–90. doi: 10.1038/emboj.2012.87
90. Arora P, Baena A, Yu KOA, Saini NK, Kharkwal SS, Goldberg MF, et al. A single subset of dendritic cells controls the cytokine bias of natural killer T cell responses to diverse glycolipid antigens. Immunity. (2014) 40:105–16. doi: 10.1016/j.immuni.2013.12.004
91. Paget C, Mallevaey T, Speak AO, Torres D, Fontaine J, Sheehan KCF, et al. Activation of invariant NKT cells by toll-like receptor 9-stimulated dendritic cells requires type I interferon and charged glycosphingolipids. Immunity. (2007) 27:597–609. doi: 10.1016/j.immuni.2007.08.017
92. Salio M, Speak AO, Shepherd D, Polzella P, Illarionov PA, Veerapen N, et al. Modulation of human natural killer T cell ligands on TLR-mediated antigen-presenting cell activation. Proc Natl Acad Sci USA. (2007) 104:20490–5. doi: 10.1073/pnas.0710145104
93. Brigl M, Tatituri RVV, Watts GFM, Bhowruth V, Leadbetter EA, Barton N, et al. Innate and cytokine-driven signals, rather than microbial antigens, dominate in natural killer T cell activation during microbial infection. J Exp Med. (2011) 208:1163–77. doi: 10.1084/jem.20102555
94. Fujii S, Liu K, Smith C, Bonito AJ, Steinman RM. The linkage of innate to adaptive immunity via maturing dendritic cells in vivo requires CD40 ligation in addition to antigen presentation and CD80/86 costimulation. J Exp Med. (2004) 199:1607–18. doi: 10.1084/jem.20040317
95. Carnaud C, Lee D, Donnars O, Park SH, Beavis A, Koezuka Y, et al. Cutting edge: cross-talk between cells of the innate immune system: NKT cells rapidly activate NK cells. J Immunol. (1999) 163:4647–50. doi: 10.4049/jimmunol.163.9.4647
96. Fujii S, Shimizu K, Smith C, Bonifaz L, Steinman RM. Activation of natural killer T cells by α-galactosylceramide rapidly induces the full maturation of dendritic cells in vivo and thereby acts as an adjuvant for combined CD4 and CD8 T cell immunity to a coadministered protein. J Exp Med. (2003) 198:267–79. doi: 10.1084/jem.20030324
97. Semmling V, Lukacs-Kornek V, Thaiss CA, Quast T, Hochheiser K, Panzer U, et al. Alternative cross-priming through CCL17-CCR4-mediated attraction of CTLs toward NKT cell–licensed DCs. Nat Immunol. (2010) 11:313–20. doi: 10.1038/ni.1848
98. Cortesi F, Delfanti G, Casorati G, Dellabona P. The pathophysiological relevance of the iNKT cell/mononuclear phagocyte crosstalk in tissues. Front Immunol. (2018) 9:2375. doi: 10.3389/fimmu.2018.02375
99. Cortesi F, Delfanti G, Grilli A, Calcinotto A, Gorini F, Pucci F, et al. Bimodal CD40/fas-dependent crosstalk between iNKT cells and tumor-associated macrophages impairs prostate cancer progression. Cell Rep. (2018) 22:3006–20. doi: 10.1016/j.celrep.2018.02.058
100. Schmieg J, Yang G, Franck RW, Van Rooijen N, Tsuji M. Glycolipid presentation to natural killer T cells differs in an organ-dependent fashion. Proc Natl Acad Sci U.S.A. (2005) 102:1127–32. doi: 10.1073/pnas.0408288102
101. Barral P, Polzella P, Bruckbauer A, van Rooijen N, Besra GS, Cerundolo V, et al. CD169(+) macrophages Present lipid antigens to mediate early activation of iNKT cells in lymph nodes. Nat Immunol. (2010) 11:303–12. doi: 10.1038/ni.1853
102. Varol C, Mildner A, Jung S. CD1d-Restricted and TCR-mediated activation of Vα14 NKT cells by glycosylceramides. Annu Rev Immunol. (2015) 33:643–75. doi: 10.1146/annurev-immunol-032414-112220
103. Wang H, Breed ER, Lee YJ, Qian LJ, Jameson SC, Hogquist KA. Myeloid cells activate iNKT cells to produce IL-4 in the thymic medulla. Proc Natl Acad Sci U.S.A. (2019) 116:22262–8. doi: 10.1073/pnas.1910412116
104. Gensollen T, Lin X, Zhang T, Pyzik M, See P, Glickman JN, et al. Embryonic macrophages function during early life to determine invariant natural killer T cell levels at barrier surfaces. Nat Immunol. (2021) 22:699–710. doi: 10.1038/s41590-021-00934-0
105. Ji Y, Sun S, Xia S, Yang L, Li X, Qi L. Short term high fat diet challenge promotes alternative macrophage polarization in adipose tissue via natural killer T cells and interleukin-4. J Biol Chem. (2012) 287:24378–86. doi: 10.1074/jbc.M112.371807
106. Lynch L, Nowak M, Varghese B, Clark J, Hogan AE, Toxavidis V, et al. Adipose tissue invariant NKT cells protect against diet-induced obesity and metabolic disorder through regulatory cytokine production. Immunity. (2012) 37:574–87. doi: 10.1016/j.immuni.2012.06.016
107. Metelitsa LS. Anti-tumor potential of type-I NKT cells against CD1d-positive and CD1d-negative tumors in humans. Clin Immunol. (2011) 140:119–29. doi: 10.1016/j.clim.2010.10.005
108. Vivier E, Ugolini S, Blaise D, Chabannon C, Brossay L. Targeting natural killer cells and natural killer T cells in cancer. Nat Rev Immunol. (2012) 12:239–52. doi: 10.1038/nri3174
109. Coquet JM, Chakravarti S, Kyparissoudis K, McNab FW, Pitt LA, McKenzie BS, et al. Diverse cytokine production by NKT cell subsets and identification of an IL-17–producing CD4−NK1.1−NKT cell population. Proc Natl Acad Sci USA. (2008) 105:11287–92. doi: 10.1073/pnas.0801631105
110. Roark JH, Park SH, Jayawardena J, Kavita U, Shannon M, Bendelac A. CD1.1 Expression by mouse antigen-presenting cells and marginal zone B cells. J Immunol. (1998) 160:3121–7. doi: 10.4049/jimmunol.160.7.3121
111. Leadbetter EA, Brigl M, Illarionov P, Cohen N, Luteran MC, Pillai S, et al. NK T cells provide lipid antigen-specific cognate help for B cells. Proc Natl Acad Sci USA. (2008) 105:8339–44. doi: 10.1073/pnas.0801375105
112. Tonti E, Galli G, Malzone C, Abrignani S, Casorati G, Dellabona P. NKT-cell help to B lymphocytes can occur independently of cognate interaction. Blood. (2009) 113:370–6. doi: 10.1182/blood-2008-06-166249
113. Vomhof-DeKrey EE, Yates J, Hägglöf T, Lanthier P, Amiel E, Veerapen N, et al. Cognate interaction with iNKT cells expands IL-10-producing B regulatory cells. Proc Natl Acad Sci USA. (2015) 112:12474–9. doi: 10.1073/pnas.1504790112
114. Mauri C, Gray D, Mushtaq N, Londei M. Prevention of arthritis by interleukin 10-producing B cells. J Exp Med. (2003) 197:489–501. doi: 10.1084/jem.20021293
115. Oleinika K, Rosser EC, Matei DE, Nistala K, Bosma A, Drozdov I, et al. CD1d-dependent Immune suppression mediated by regulatory B cells through modulations of iNKT cells. Nat Commun. (2018) 9:684. doi: 10.1038/s41467-018-02911-y
116. Cho YN, Kee SJ, Lee SJ, Seo SR, Kim TJ, Lee SS, et al. Numerical and functional deficiencies of natural killer T cells in systemic lupus erythematosus: their deficiency related to disease activity. Rheumatology (Oxford). (2011) 50:1054–63. doi: 10.1093/rheumatology/keq457
117. Nieuwenhuis EES, Matsumoto T, Exley M, Schleipman RA, Glickman J, Bailey DT, et al. CD1d-dependent macrophage-mediated clearance of Pseudomonas aeruginosa from lung. Nat Med. (2002) 8:588–93. doi: 10.1038/nm0602-588
118. Kawakami K, Yamamoto N, Kinjo Y, Miyagi K, Nakasone C, Uezu K, et al. Critical role of Vα14+ natural killer T cells in the innate phase of host protection against Streptococcus pneumoniae infection. Eur J Immunol. (2003) 33:3322–30. doi: 10.1002/eji.200324254
119. Lappas CM, Day Y-J, Marshall MA, Engelhard VH, Linden J. Adenosine A2A receptor activation reduces hepatic ischemia reperfusion injury by inhibiting CD1d-dependent NKT cell activation. J Exp Med. (2006) 203:2639–48. doi: 10.1084/jem.20061097
120. Wang H, Feng D, Park O, Yin S, Gao B. Invariant NKT cell activation induces neutrophil accumulation and hepatitis: opposite regulation by IL-4 and IFN-γ: hepatology. Hepatology. (2013) 58:1474–85. doi: 10.1002/hep.26471
121. Wingender G, Hiss M, Engel I, Peukert K, Ley K, Haller H, et al. Neutrophilic granulocytes modulate invariant NKT cell function in mice and humans. Journal Immunol. (2012) 188:3000–8. doi: 10.4049/jimmunol.1101273
122. Reilly EC, Wands JR, Brossay L. Cytokine dependent and independent iNKT cell activation. Cytokine. (2010) 51:227–31. doi: 10.1016/j.cyto.2010.04.016
123. Young JS, Heffernan DS, Chung CS, Kettenmann ML, Young WA, Guillen VS, et al. Effect of PD-1: PD-L1 in invariant natural killer T-cell emigration and chemotaxis following sepsis. Shock. (2016) 45:534–9. doi: 10.1097/SHK.0000000000000553
124. Liu TY, Uemura Y, Suzuki M, Narita Y, Hirata S, Ohyama H, et al. Distinct subsets of human invariant NKT cells differentially regulate T helper responses via dendritic cells. Eur J Immunol. (2008) 38:1012–23. doi: 10.1002/eji.200737838
125. Wallace KL, Marshall MA, Ramos SI, Lannigan JA, Field JJ, Strieter RM, et al. NKT cells mediate pulmonary inflammation and dysfunction in murine sickle cell disease through production of IFN-γ and CXCR3 chemokines. Blood. (2009) 114:667–76. doi: 10.1182/blood-2009-02-205492
126. Field JJ, Nathan DG, Linden J. Targeting iNKT cells for the treatment of sickle cell disease. Clin Immunol. (2011) 140:177–83. doi: 10.1016/j.clim.2011.03.002
127. Berzins SP. Presumed guilty: natural killer T cell defects and human disease. Nat Rev Immunol. (2011) 12:131–42.
128. Chaidos A, Patterson S, Szydlo R, Chaudhry MS, Dazzi F, Kanfer E, et al. Graft invariant natural killer T-cell dose predicts risk of acute graft-versus-host disease in allogeneic hematopoietic stem cell transplantation. Blood. (2012) 119:5030–6. doi: 10.1182/blood-2011-11-389304
129. Haraguchi K, Takahashi T, Hiruma K, Kanda Y, Tanaka Y, Ogawa S, et al. Recovery of Vα24+ NKT cells after hematopoietic stem cell transplantation. Bone Marrow Transplant. (2004) 34:595–602. doi: 10.1038/sj.bmt.1704582
130. Guan P, Bassiri H, Patel NP, Nichols KE, Das R. Invariant natural killer T cells in hematopoietic stem cell transplantation: killer choice for natural suppression. Bone Marrow Transplant. (2016) 51:629–37. doi: 10.1038/bmt.2015.335
131. de Lalla C, Rinaldi A, Montagna D, Azzimonti L, Bernardo ME, Sangalli LM, et al. Invariant NKT cell reconstitution in pediatric leukemia patients given HLA-haploidentical stem cell transplantation defines distinct CD4+ and CD4− subset dynamics and correlates with remission state. J Immunol. (2011) 186:4490–9. doi: 10.4049/jimmunol.1003748
132. Bassiri H, Das R, Nichols KE. Invariant NKT cells: killers and conspirators against cancer. Oncoimmunology. (2013) 2:e27440. doi: 10.4161/onci.27440
133. Wingender G, Krebs P, Beutler B, Kronenberg M. Antigen-specific cytotoxicity by invariant NKT cells in vivo is CD95/CD178-dependent and is correlated with antigenic potency. J Immunol. (2010) 185:2721–9. doi: 10.4049/jimmunol.1001018
134. Matsuda JL, Zhang Q, Ndonye R, Richardson SK, Howell AR, Gapin L. T-bet concomitantly controls migration, survival, and effector functions during the development of Vα14i NKT cells. Blood. (2006) 107:2797–805. doi: 10.1182/blood-2005-08-3103
135. Dao T, Mehal WZ, Crispe IN. IL-18 augments perforin-dependent cytotoxicity of liver NK-T cells1. J Immunol. (1998) 161:2217–22. doi: 10.4049/jimmunol.161.5.2217
136. Nieda M, Nicol A, Koezuka Y, Kikuchi A, Lapteva N, Tanaka Y, et al. TRAIL expression by activated human CD4+ Vα24NKT cells induces in vitro and in vivo apoptosis of human acute myeloid leukemia cells. Blood. (2001) 97:2067–74. doi: 10.1182/blood.V97.7.2067
137. Expression of CD1D in Cancer—Summary—The Human Protein Atlas. Available online at: https://www.proteinatlas.org/ENSG00000158473-CD1D/pathology (Accessed March 24, 2024).
138. Nur H, Fostier K, Aspeslagh S, Renmans W, Bertrand E, Leleu X, et al. Preclinical evaluation of invariant natural killer T cells in the 5T33 multiple myeloma model. PloS One. (2013) 8:e65075. doi: 10.1371/journal.pone.0065075
139. Hix LM, Shi YH, Brutkiewicz RR, Stein PL, Wang CR, Zhang M. CD1d-expressing breast cancer cells modulate NKT cell-mediated antitumor immunity in a murine model of breast cancer metastasis. PloS One. (2011) 6:e20702. doi: 10.1371/journal.pone.0020702
140. Delfanti G, Cortesi F, Perini A, Antonini G, Azzimonti L, de Lalla C, et al. TCR-engineered iNKT cells induce robust antitumor response by dual targeting cancer and suppressive myeloid cells. Sci Immunol. (2022) 7:eabn6563. doi: 10.1126/sciimmunol.abn6563
141. Gorini F, Azzimonti L, Delfanti G, Scarfò L, Scielzo C, Bertilaccio MT, et al. Invariant NKT cells contribute to chronic lymphocytic leukemia surveillance and prognosis. Blood. (2017) 129:3440–51. doi: 10.1182/blood-2016-11-751065
142. Scott EN, Gocher AM, Workman CJ, Vignali DAA. Regulatory T cells: barriers of immune infiltration into the tumor microenvironment. Front Immunol. (2021) 12:1–10.
143. Solinas G, Germano G, Mantovani A, Allavena P. Tumor-associated macrophages (TAM) as major players of the cancer-related inflammation. J Leukocyte Biol. (2009) 86:1065–73. doi: 10.1189/jlb.0609385
144. Tsukada N, Burger JA, Zvaifler NJ, Kipps TJ. Distinctive features of “nurselike” cells that differentiate in the context of chronic lymphocytic leukemia. Blood. (2002) 99:1030–7. doi: 10.1182/blood.V99.3.1030
145. Nowak M, Arredouani MS, Tun-Kyi A, Schmidt-Wolf I, Sanda MG, Balk SP, et al. Defective NKT cell activation by CD1d+ TRAMP prostate tumor cells is corrected by interleukin-12 with alpha-galactosylceramide. PloS One. (2010) 5:e11311. doi: 10.1371/journal.pone.0011311
146. Liu X, Ranganathan R, Jiang S, Fang C, Sun J, Kim S, et al. A chimeric switch-receptor targeting PD1 augments the efficacy of second-generation CAR T cells in advanced solid tumors. Cancer Res. (2016) 76:1578–90. doi: 10.1158/0008-5472.CAN-15-2524
147. Murray J, Stringer J, Hutt D. Graft-Versus-Host disease (GvHD). In: Kenyon M, Babic A, editors. The European blood and marrow transplantation textbook for nurses: Under the auspices of EBMT. Cham (CH): Springer (2018). p. 221–51.
148. Teshima T, Hill GR. The pathophysiology and treatment of graft-versus-host disease: lessons learnt from animal models. Front Immunol. (2021) 12:715424. doi: 10.3389/fimmu.2021.715424
149. Kumar S, Mohammadpour H, Cao X. Targeting cytokines in GVHD therapy. J Immunol Res Therapy. (2017) 2:90.
150. Schneidawind D, Pierini A, Alvarez M, Pan Y, Baker J, Buechele C, et al. Third-party CD4 + invariant natural killer T cells protect from murine GVHD lethality. Blood. (2015) 125:3491–500. doi: 10.1182/blood-2014-11-612762
151. Mavers M, Maas-Bauer K, Negrin RS. Invariant natural killer T cells as suppressors of graft-versus-host disease in allogeneic hematopoietic stem cell transplantation. Front Immunol. (2017) 8:900. doi: 10.3389/fimmu.2017.00900
152. Negrin RS. Immune regulation in hematopoietic cell transplantation. Bone Marrow Transplant. (2019) 54:765–8. doi: 10.1038/s41409-019-0600-7
153. Chang DH, Osman K, Connolly J, Kukreja A, Krasovsky J, Pack M, et al. Sustained expansion of NKT cells and antigen-specific T cells after injection of α-galactosyl-ceramide loaded mature dendritic cells in cancer patients. J Exp Med. (2005) 201:1503–17. doi: 10.1084/jem.20042592
154. Motohashi S, Okamoto Y, Yoshino I, Nakayama T. Anti-tumor immune responses induced by iNKT cell-based immunotherapy for lung cancer and head and neck cancer. Clin Immunol. (2011) 140:167–76. doi: 10.1016/j.clim.2011.01.009
155. Tatsumi T, Takehara T, Yamaguchi S, Sasakawa A, Sakamori R, Ohkawa K, et al. Intrahepatic delivery of α-galactosylceramide-pulsed dendritic cells suppresses liver tumor. Hepatology. (2007) 45:22–30. doi: 10.1002/hep.21447
156. Escribà-Garcia L, Alvarez-Fernández C, Tellez-Gabriel M, Sierra J, Briones J. Dendritic cells combined with tumor cells and α-galactosylceramide induce a potent, therapeutic and NK-cell dependent antitumor immunity in B cell lymphoma. J Transl Med. (2017) 15:115. doi: 10.1186/s12967-017-1219-3
157. Shimizu K, Kurosawa Y, Taniguchi M, Steinman RM, Fujii S. Cross-presentation of glycolipid from tumor cells loaded with α-galactosylceramide leads to potent and long-lived T cell–mediated immunity via dendritic cells. J Exp Med. (2007) 204:2641–53. doi: 10.1084/jem.20070458
158. Fujii S-I, Shimizu K, Hemmi H, Fukui M, Bonito AJ, Chen G, et al. Glycolipid α-C-galactosylceramide is a distinct inducer of dendritic cell function during innate and adaptive immune responses of mice. Proc Natl Acad Sci USA. (2006) 103:11252–7. doi: 10.1073/pnas.0604812103
159. Kobayashi T, Doff BL, Rearden RC, Leggatt GR, Mattarollo SR. NKT cell-targeted vaccination plus anti-4–1BB antibody generates persistent CD8 T cell immunity against B cell lymphoma. OncoImmunology. (2015) 4:e990793. doi: 10.4161/2162402X.2014.990793
160. Mattarollo SR, West AC, Steegh K, Duret H, Paget C, Martin B, et al. NKT Cell adjuvant-based tumor vaccine for treatment of myc oncogene-driven mouse B-cell lymphoma. Blood. (2012) 120:3019–29. doi: 10.1182/blood-2012-04-426643
161. Gibbins JD, Ancelet LR, Weinkove R, Compton BJ, Painter GF, Petersen TR, et al. An autologous leukemia cell vaccine prevents murine acute leukemia relapse after cytarabine treatment. Blood. (2014) 124:2953–63. doi: 10.1182/blood-2014-04-568956
162. Myers JA, Miller JS. Exploring the NK cell platform for cancer immunotherapy. Nat Rev Clin Oncol. (2021) 18:85–100. doi: 10.1038/s41571-020-0426-7
163. Ruella M, Korell F, Porazzi P, Maus MV. Mechanisms of resistance to chimeric antigen receptor-T cells in haematological malignancies. Nat Rev Drug Discov. (2023):1–20. doi: 10.1038/s41573-023-00807-1
164. Benjamin R, Jain N, Maus MV, Boissel N, Graham C, Jozwik A, et al. UCART19, A first-in-class allogeneic anti-CD19 chimeric antigen receptor T-cell therapy for adults with relapsed or refractory B-cell acute lymphoblastic leukaemia (CALM): a phase 1, dose-escalation trial. The Lancet Haematology. (2022) 9:e833–43. doi: 10.1016/S2352-3026(22)00245-9
165. Xu KL, Zheng JN. Clinical Study of CAR-iNKT Cells in the Treatment of Relapsed/Refractory/High-Risk B-Cell Tumors (2021). Available online at: https://clinicaltrials.gov/study/NCT04814004 (Accessed March 24, 2024).
166. M.D. Anderson Cancer Center. Dose Escalation Study Phase I/II of Umbilical Cord Blood-Derived CAR-Engineered NK Cells in Conjunction with Lymphodepleting Chemotherapy in Patients With Relapsed/Refractory B-Lymphoid Malignancies (2023). Available online at: https://clinicaltrials.gov/study/NCT03056339 (Accessed March 24, 2024).
167. Fate Therapeutics. FT500 as Monotherapy and in Combination with Immune Checkpoint Inhibitors in Subjects With Advanced Solid Tumors (Phase 1) (2023). Available online at: https://clinicaltrials.gov/study/NCT03841110 (Accessed March 24, 2024).
168. Central Hospital, Nancy, France. Expansion of Invariant NKT Cells for a Cell Immunotherapeutic Approach Allowing the Control of Graft Versus Host-Disease and Preserving the Graft Versus Leukemia Effect After Allogeneic Hematopoietic Stem Cell Transplantation (2018). Available online at: https://clinicaltrials.gov/study/NCT03605953 (Accessed March 24, 2024).
169. Athenex, Inc. Allogeneic Natural Killer T-Cells Expressing CD19 Specific Chimeric Antigen Receptor and Interleukin-15 in Relapsed or Refractory B-Cell Malignancies 2 (2023). Available online at: https://clinicaltrials.gov/study/NCT05487651 (Accessed March 24, 2024).
170. Ramos C. Allogeneic Natural Killer T-Cells Expressing CD19 Specific Chimeric Antigen Receptor and Interleukin-15 in Relapsed or Refractory B-Cell Malignancies (2023). Available online at: https://clinicaltrials.gov/study/NCT03774654 (Accessed March 24, 2024).
171. Watarai H, Fujii S, ichiro Yamada D, Rybouchkin A, Sakata S, Nagata Y, et al. Murine induced pluripotent stem cells can be derived from and differentiate into natural killer T cells. J Clin Invest. (2010) 120:2610–8. doi: 10.1172/JCI42027
172. Li Y-R, Dunn ZS, Zhou Y, Lee D, Yang L. Development of stem cell-derived immune cells for off-the-shelf cancer immunotherapies. Cells. (2021) 10:3497. doi: 10.3390/cells10123497
173. Rotolo A, Caputo VS, Holubova M, Baxan N, Dubois O, Chaudhry MS, et al. Enhanced anti-lymphoma activity of CAR19-iNKT cells underpinned by dual CD19 and CD1d targeting. Cancer Cell. (2018) 34:596–610.e11. doi: 10.1016/j.ccell.2018.08.017
174. Poels R, Drent E, Lameris R, Katsarou A, Themeli M, van der Vliet HJ, et al. Preclinical evaluation of invariant natural killer T cells modified with CD38 or BCMA chimeric antigen receptors for multiple myeloma. IJMS. (2021) 22:1096. doi: 10.3390/ijms22031096
175. Parrish-Novak J, Dillon SR, Nelson A, Hammond A, Sprecher C, Gross JA, et al. Interleukin 21 and its receptor are involved in NK cell expansion and regulation of lymphocyte function. Nature. (2000) 408:57–63. doi: 10.1038/35040504
176. Coquet JM, Kyparissoudis K, Pellicci DG, Besra G, Berzins SP, Smyth MJ, et al. IL-21 is produced by NKT cells and modulates NKT cell activation and cytokine production1. J Immunol. (2007) 178:2827–34. doi: 10.4049/jimmunol.178.5.2827
177. Habib T, Senadheera S, Weinberg K, Kaushansky K. The common γ chain (γc) is a required signaling component of the IL-21 receptor and supports IL-21-induced cell proliferation via JAK3. Biochemistry. (2002) 41:8725–31. doi: 10.1021/bi0202023
178. O'Neal J, Cooper ML, Ritchey JK, Gladney SW, Niswonger J, Gonzalez LS, et al. Anti-myeloma efficacy of CAR-iNKT is enhanced with a long-acting IL-7, rhIL-7hyFc. Blood Advances. (2023) 7(20):6009–22. doi: 10.1182/bloodadvances.2023010032
179. Landoni E, Smith CC, Fucá G, Chen Y, Sun C, Vincent BG, et al. A high-avidity T-cell receptor redirects natural killer T-cell specificity and outcompetes the endogenous invariant T-cell receptor. Cancer Immunol Res. (2020) 8:57–69. doi: 10.1158/2326-6066.CIR-19-0134
180. Lin H, Cheng J, Mu W, Zhou J, Zhu L. Advances in universal CAR-T cell therapy. Front Immunol. (2021) 12:744823. doi: 10.3389/fimmu.2021.744823
182. Verma M, Obergfell K, Topp S, Panier V, Wu J. The next-generation CAR-T therapy landscape. Nat Rev Drug Discovery. (2023) 22:776–7. doi: 10.1038/d41573-023-00140-7
183. Myburgh R, Kiefer JD, Russkamp NF, Magnani CF, Nuñez N, Simonis A, et al. Anti-human CD117 CAR T-cells efficiently eliminate healthy and malignant CD117-expressing hematopoietic cells. Leukemia. (2020) 34:2688–703. doi: 10.1038/s41375-020-0818-9
184. Li AM, Hucks GE, Dinofia AM, Seif AE, Teachey DT, Baniewicz D, et al. Checkpoint inhibitors augment CD19-directed chimeric antigen receptor (CAR) T cell therapy in relapsed B-cell acute lymphoblastic leukemia. Blood. (2018) 132:556. doi: 10.1182/blood-2018-99-112572
185. Fraietta JA, Beckwith KA, Patel PR, Ruella M, Zheng Z, Barrett DM, et al. Ibrutinib enhances chimeric antigen receptor T-cell engraftment and efficacy in leukemia. Blood. (2016) 127:1117–27. doi: 10.1182/blood-2015-11-679134
186. Malard F, Holler E, Sandmaier BM, Huang H, Mohty M. Acute graft-versus-host disease. Nat Rev Dis Primers. (2023) 9:1–18. doi: 10.1038/s41572-023-00438-1
187. Shlomchik WD. Graft-versus-host disease. Nat Rev Immunol. (2007) 7:340–52. doi: 10.1038/nri2000
188. Filipovich AH, Weisdorf D, Pavletic S, Socie G, Wingard JR, Lee SJ, et al. National institutes of health consensus development project on criteria for clinical trials in chronic graft-versus-host disease: i. Diagnosis and staging working group report. Biol Blood Marrow Transplant. (2005) 11:945–56. doi: 10.1016/j.bbmt.2005.09.004
189. Kadri N, Amu S, Iacobaeus E, Boberg E, Le Blanc K. Current perspectives on mesenchymal stromal cell therapy for graft versus host disease. Cell Mol Immunol. (2023) 20:613–25. doi: 10.1038/s41423-023-01022-z
190. Zeiser R, Blazar BR. Acute graft-versus-host disease biology, prevention and therapy. N Engl J Med. (2017) 377:2167–79. doi: 10.1056/NEJMra1609337
191. Wolff D, Fatobene G, Rocha V, Kröger N, Flowers ME. Steroid-refractory chronic graft-versus-host disease: treatment options and patient management. Bone Marrow Transplant. (2021) 56:2079–87. doi: 10.1038/s41409-021-01389-5
192. Moiseev I, Ambron P, Badoglio M, Peczynski C, Basak G, Koenecke C, et al. Steroid-free first line treatment of moderate and severe chronic GVHD: a survey from the transplant complications working party of the EBMT. Bone Marrow Transplant. (2023) 58:325–7. doi: 10.1038/s41409-022-01881-6
193. Lan F, Zeng D, Higuchi M, Higgins JP, Strober S. Host conditioning with total lymphoid irradiation and antithymocyte globulin prevents graft-versus-host disease: the role of CD1-reactive natural killer T cells. Biol Blood Marrow Transplant. (2003) 9:355–63. doi: 10.1016/S1083-8791(03)00108-3
194. Pillai AB, George TI, Dutt S, Teo P, Strober S. Host NKT cells can prevent graft-versus-host disease and permit graft antitumor activity after bone marrow Transplantation1. J Immunol. (2007) 178:6242–51. doi: 10.4049/jimmunol.178.10.6242
195. Leveson-Gower DB, Olson JA, Sega EI, Luong RH, Baker J, Zeiser R, et al. Low doses of natural killer T cells provide protection from acute graft-versus-host disease via an IL-4–dependent mechanism. Blood. (2011) 117:3220–9. doi: 10.1182/blood-2010-08-303008
196. Hashimoto D, Asakura S, Miyake S, Yamamura T, Van Kaer L, Liu C, et al. Stimulation of host NKT cells by synthetic glycolipid regulates acute graft-versus-host disease by inducing Th2 polarization of donor T Cells1. J Immunol. (2005) 174:551–6. doi: 10.4049/jimmunol.174.1.551
197. Duramad O, Laysang A, Li J, Ishii Y, Namikawa R. Pharmacologic expansion of donor-derived, naturally occurring CD4 + Foxp3+ regulatory T cells reduces acute graft-versus-host disease lethality without abrogating the graft-versus-leukemia effect in murine models. Biol Blood Marrow Transplant. (2011) 17:1154–68. doi: 10.1016/j.bbmt.2010.11.022
198. Pillai AB, George TI, Dutt S, Strober S. Host natural killer T cells induce an interleukin-4–dependent expansion of donor CD4 + CD25 + Foxp3+ T regulatory cells that protects against graft-versus-host disease. Blood. (2009) 113:4458–67. doi: 10.1182/blood-2008-06-165506
199. Hongo D, Tang X, Dutt S, Nador RG, Strober S. Interactions between NKT cells and Tregs are required for tolerance to combined bone marrow and organ transplants. Blood. (2012) 119:1581–9. doi: 10.1182/blood-2011-08-371948
200. Hirai T, Ishii Y, Ikemiyagi M, Fukuda E, Omoto K, Namiki M, et al. A novel approach inducing transplant tolerance by activated invariant natural killer T cells with costimulatory blockade. Am J Transplant. (2014) 14:554–67. doi: 10.1111/ajt.12606
201. Du J, Paz K, Thangavelu G, Schneidawind D, Baker J, Flynn R, et al. Invariant natural killer T cells ameliorate murine chronic GVHD by expanding donor regulatory T cells. Blood. (2017) 129:3121–5. doi: 10.1182/blood-2016-11-752444
202. Hoffmann P, Ermann J, Edinger M, Fathman CG, Strober S. Donor-type CD4+ CD25+ regulatory T cells suppress lethal acute graft-versus-host disease after allogeneic bone marrow transplantation. J Exp Med. (2002) 196:389–99. doi: 10.1084/jem.20020399
203. Edinger M, Hoffmann P, Ermann J, Drago K, Fathman CG, Strober S, et al. CD4+ CD25+ regulatory T cells preserve graft-versus-tumor activity while inhibiting graft-versus-host disease after bone marrow transplantation. Nat Med. (2003) 9:1144–50. doi: 10.1038/nm915
204. Guo WW, Su XH, Wang MY, Han MZ, Feng XM, Jiang EL. Regulatory T cells in GVHD therapy. Front Immunol. (2021) 12:1–12.
205. Hongo D, Tang X, Baker J, Engleman EG, Strober S. Requirement for interactions of natural killer T cells and myeloid-derived suppressor cells for transplantation tolerance. Am J Transplant. (2014) 14:2467–77. doi: 10.1111/ajt.12914
206. Hongo D, Tang X, Zhang X, Engleman EG, Strober S. Tolerogenic interactions between CD8 + dendritic cells and NKT cells prevent rejection of bone marrow and organ grafts. Blood. (2017) 129:1718–28. doi: 10.1182/blood-2016-07-723015
207. Yamazaki S, Dudziak D, Heidkamp GF, Fiorese C, Bonito AJ, Inaba K, et al. CD8+ CD205+ splenic dendritic cells are specialized to induce Foxp3+ regulatory T cells. J Immunol. (2008) 181:6923. doi: 10.4049/jimmunol.181.10.6923
208. Malard F, Labopin M, Chevallier P, Guillaume T, Duquesne A, Rialland F, et al. Larger number of invariant natural killer T cells in PBSC allografts correlates with improved GVHD-free and progression-free survival. Blood. (2016) 127:1828–35. doi: 10.1182/blood-2015-12-688739
209. Rubio MT, Bouillié M, Bouazza N, Coman T, Trebeden-Nègre H, Gomez A, et al. Pre-transplant donor CD4− invariant NKT cell expansion capacity predicts the occurrence of acute graft-versus-host disease. Leukemia. (2017) 31:903–12. doi: 10.1038/leu.2016.281
210. Rubio MT, Moreira-Teixeira L, Bachy E, Bouillié M, Milpied P, Coman T, et al. Early posttransplantation donor-derived invariant natural killer T-cell recovery predicts the occurrence of acute graft-versus-host disease and overall survival. Blood. (2012) 120:2144–54. doi: 10.1182/blood-2012-01-404673
211. Kohrt HE, Turnbull BB, Heydari K, Shizuru JA, Laport GG, Miklos DB, et al. TLI and ATG conditioning with low risk of graft-versus-host disease retains antitumor reactions after allogeneic hematopoietic cell transplantation from related and unrelated donors. Blood. (2009) 114:1099–109. doi: 10.1182/blood-2009-03-211441
212. Chen YB, Efebera YA, Johnston L, Ball ED, Avigan D, Lekakis LJ, et al. Increased Foxp3+ Helios+ regulatory T cells and decreased acute graft-versus-host disease after allogeneic bone marrow transplantation in patients receiving sirolimus and RGI-2001, an activator of invariant natural killer T cells. Biol Blood Marrow Transplant. (2017) 23:625–34. doi: 10.1016/j.bbmt.2017.01.069
213. Schmid H, Ribeiro EM, Secker KA, Duerr-Stoerzer S, Keppeler H, Dong R, et al. Human invariant natural killer T cells promote tolerance by preferential apoptosis induction of conventional dendritic cells. Haematologica. (2022) 107:427–36. doi: 10.3324/haematol.2020.267583
Keywords: invariant natural killer T cell, graft vs. host disease, acute myeloid leukemia, chimeric antigen receptor, microenvironment, immune therapy, hematopoietic stem cell transplant (HSCT)
Citation: Boonchalermvichian C, Yan H, Gupta B, Rubin A, Baker J and Negrin RS (2024) invariant Natural Killer T cell therapy as a novel therapeutic approach in hematological malignancies. Front. Transplant. 3:1353803. doi: 10.3389/frtra.2024.1353803
Received: 11 December 2023; Accepted: 4 March 2024;
Published: 6 May 2024.
Edited by:
Oriol de Barrios, Josep Carreras Leukaemia Research Institute (IJC), Spain© 2024 Boonchalermvichian, Yan, Gupta, Rubin, Baker and Negrin. This is an open-access article distributed under the terms of the Creative Commons Attribution License (CC BY). The use, distribution or reproduction in other forums is permitted, provided the original author(s) and the copyright owner(s) are credited and that the original publication in this journal is cited, in accordance with accepted academic practice. No use, distribution or reproduction is permitted which does not comply with these terms.
*Correspondence: Chaiyaporn Boonchalermvichian YmNoYWl5YXBAc3RhbmZvcmQuZWR1
†These authors have contributed equally to this work