- 1Norton Thoracic Institute, St. Joseph’s Hospital and Medical Center, Phoenix, AZ, United States
- 2HLA Laboratory, Vitalant, Phoenix, AZ, United States
- 3Department of Surgery, Washington University, St. Louis, MO, United States
Identification of recipients with pre-existing antibodies and cross-matching of recipient sera with donor lymphocytes have reduced the incidence of antibody-mediated rejection (AMR) after human lung transplantation. However, AMR is still common and requires not only immediate intervention but also has long-term consequences including an increased risk of chronic lung allograft dysfunction (CLAD). The mechanisms resulting in AMR remain largely unknown due to the variation in clinical and histopathological features among lung transplant recipients; however, several reports have demonstrated a strong association between the development of antibodies against mismatched donor human leucocyte antigens [donor-specific antibodies (DSAs)] and AMR. In addition, the development of antibodies against lung self-antigens (K alpha1 tubulin and collagen V) also plays a vital role in AMR pathogenesis, either alone or in combination with DSAs. In the current article, we will review the existing literature regarding the association of DSAs with AMR, along with clinical diagnostic features and current treatment options for AMR. We will also discuss the role of extracellular vesicles (EVs) in the immune-related pathogenesis of AMR, which can lead to CLAD.
1. Introduction
Lung transplantation (LTx) is the primary treatment for patients with end-stage lung disease. Surgical advancements have improved outcomes, but the long-term function of the transplanted lungs remains disappointing, with a median survival after LTx of 6.2 years (1–4). Antibody-mediated rejection (AMR) of transplanted lungs remains an important problem, which is further complicated by a lack of consensus on the clinical characteristic as well as the immunological profile, histological features, and management strategies (5, 6).
Antibody-mediated rejection is a complex pathological, serological, and clinical process affecting graft function after transplantation. It has been better characterized in kidney and heart transplant recipients than in lung transplant recipients (LTxRs) (7). In LTxRs, specific B-cells and plasma cells producing antibodies directed against donor lung antigens can often be detected even before transplant, implicating these antibodies in the immunopathogenesis of AMR (8). Recent literature provides strong evidence of an important role for antibodies in AMR since antibodies to mismatched donor human leukocyte antigens, known as donor-specific antibodies (DSAs) and to lung self-antigens are often detected in patients with AMR. De novo production of DSAs can be detected within weeks to months after transplantation (6, 9, 10). Further, the presences of DSAs are associated with a poor prognosis and possibly accelerated graft failure, particularly within the first post-transplant year (11–13).
Investigations in the last few decades in solid organ transplants have demonstrated that antibodies, with or without a cellular response, could lead to ligation of major histocompatibility complex molecules, resulting in complement-dependent cell lysis with or without C4d deposition, which can damage the allograft (14–17). Other risk factors for AMR include gender; female recipients have higher risk of AMR post-transplant in cardiac patients (18–20); higher levels of pre-transplant panel reactive antibodies (PRAs) to HLA (21, 22); development of de novo DSAs resulting in positive donor-specific crossmatch (23); and re-transplantation (24). Per the International Society of Heart and Lung Transplantation (ISHLT) consensus, patients with AMR can be symptomatic (hypoxemia, decrease in FEV1, dyspnea, and pulmonary infiltrates) or asymptomatic (5, 25, 26). AMR can be clinical or subclinical with normal allograft function (25, 26), which can be further sub-categorized into definite, probable, and possible. These categories are based on the degree of certainty related to (a) pathologic, (b) serologic, (c) clinical, and (d) immunologic presentations (26).
The diagnosis of AMR in LTxRs is challenging as there is a lack of specific diagnostic criterion as well as tremendous variability in DSAs and allograft damage from patient to patient. There is a definite need to develop new diagnostic tools and techniques to diagnose and describe the clinical presentation of AMR, and ISHLT is currently attempting to come to a consensus on defining AMR (25, 27).
Chronic lung allograft dysfunction (CLAD) is the main barrier to good long-term outcomes the first year after lung transplantation (28, 29). Antibody-mediated rejection after lung transplantation is a progressive process that has been identified as a significant cause of morbidity that can lead to CLAD, eventually resulting in graft failure (5). In the current article, we will discuss recent updates on the understanding of AMR and our ongoing research on extracellular vesicles and their contents.
2. Pathogenies of AMR
Studies in kidney transplant recipients have helped to define the mechanisms of AMR (30, 31). AMR can be (a) hyperacute (occurring within minutes after the vascular anastomosis), (b) acute (occurring days to weeks after transplantation), (c) late acute (occurring within 3 months after transplantation), and (d) chronic (occurring months to years after transplantation) (5, 32).
Recent research has demonstrated that B cells and plasma cells produce DSAs that interact with the endothelium, leading to the activation of signaling pathways (31, 33). Antibody binding leads to the recruitment of immune cells leading to graft dysfunction, which can be either complement dependent or independent (34–36). The antibodies interacting with endothelium and activating different signaling pathways can be specific to HLA or non-HLA molecules (35, 36).
Non-HLA antibodies are further classified as alloantibodies and autoantibodies (37, 38). Unlike antibodies specific to HLA, non-HLA antibodies use alternative pathways to bind to endothelial cells causing injuries that do not involve binding to integrins (37). Fernandez et al. have demonstrated that one-third of lung recipients have pre-existing antibodies against non-HLA self-antigens, which can lead to hyperacute rejection (39). Subramanian et al. confirmed this viewpoint using a murine lung transplant model of rejection by administering antibodies specific to the lung self-antigen K alpha 1 tubulin (40).
3. Diagnosis of AMR and associated challenges
Allograft failure within 12 months of LTx due to AMR is one of the leading causes of early death in LTxRs (41). Combinations of multiple AMR diagnostic criterions are used at different centers. Several different invasive (biopsies, molecular microscopy) and noninvasive approaches (specific antibodies, donor-derived cell-free DNA, chemokine analysis, etc.) are used to diagnose AMR. As per ISHLT consensus on pulmonary AMR (25), a diagnosis of allograft dysfunction requires histologic evidence, complement component 4d (C4d) deposition, circulating DSAs, and the reasonable exclusion of other causes (11, 17). Although histology remains a popular diagnostic approach, new noninvasive methods involving blood (DSAs, donor-derived cell-free DNA, mRNA assays) or urine (chemokines, urinary mRNA, and urinary proteomics) are also becoming popular (5, 27, 42). We have summarized AMR diagnosis with invasive and non-invasive methods and treatments in Table 1.
3.1. Histologic evidence
Lung graft dysfunction is associated with certain histological types. De Nicola et al. analyzed 41 biopsies from LTxRs with or without circulating DSAs. The authors concluded that pathological findings of grade 2+ neutrophilic infiltration is the most closely related to DSAs with graft dysfunction (50). Per ISHLT consensus (25, 27), the histopathologic features of AMR, which can progress to Chronic Lung Allograft Dysfunction (CLAD) include: neutrophilic margination (43), neutrophilic capillaritis, organizing pneumonia, pulmonary capillaritis (44, 45), septal capillary injury syndrome (46), and septal capillary necrosis (47). Immunohistochemistry for C4d, either by immunofluorescence (IF) or immunoperoxidase (IP) assays, may also provide supportive evidence of AMR (47–49).
3.2. C4d deposition
C4d is a degradation product of the complement pathway that binds to endothelium and is one of the markers of endothelial injury mediated by complement deposition (30). C4d deposition in recipients with AMR has been inconsistent and its role in the diagnosis has been controversial. The sensitivity of C4d deposition is always a question due to the emerging evidence of pulmonary AMR in the absence of C4d deposition (6, 51, 52). C4d staining has been difficult to interpret in lung biopsies because of poor reproducibility, high background staining, and poor specificity for AMR. Reports from the AMR studies in kidney and heart transplant recipients led to the recognition of a unique AMR pathogenesis, which is mediated primarily by natural killer cell interactions with DSAs, independent of complement activation and bound to endothelial cells (6, 53, 54). Studies from other organ transplant recipients will provide impactful insights in determining the incidence and clinical presentation of C4d-negative probable AMR and C4d-positive definite AMR after human lung transplantation (54, 55). Mauiyyedi et al. proposed that a correlation between C4d deposition and DSAs could be a diagnostic marker for AMR (56).
3.3. Donor-specific antibodies
Donor-specific antibodies have been strongly associated with acute allograft rejection after solid organ transplant, including kidney, heart, and lung (57–59). DSAs were first identified in 1960 in kidney transplant recipients undergoing AMR, and it was postulated that they may be associated with graft rejection/failure. Further evidence by other groups also supported this concept (59, 60). All nucleated cells within transplanted lungs can express HLA class I antigens (HLA-A, HLA-B, or HLA-C). In addition, antigen-presenting cells (APCs) within the lungs may also express HLA class II antigens (HLA-DQ, HLA-DR, HLA-DP) (61). In addition, the expression of HLA class II molecules can be induced on pulmonary endothelial cells in response to pro-inflammatory cytokines (62, 63).
DSAs have been associated with not only AMR but also the development of CLAD in lung transplant recipients, manifested as bronchiolitis obliterans syndrome (BOS) or, more frequently, restrictive allograft syndrome (RAS) (28, 29, 64).
Although there are number of traditional options to diagnose AMR (histologic evidence, C4d deposition, and DSAs), there are problems with the current identification methods: (a) positive C4d stains without detectable DSAs; (b) positive C4d stains without graft abnormalities; (c) variable levels of DSAs with varying strength; and (d) antibodies to non-HLAs with or without detectable DSAs, etc. Therefore, it is important to devlop modern diagnostic techniques to determine AMR. One emerging possibility, in addition to C4d staining, is non-invasive molecular diagnostics (27, 42).
4. Management and treatment of AMR
Despite the identification of AMR in lung, kidney, and heart transplant recipients, the literature describing the management of AMR after lung transplantation is very limited. Treatment strategies for AMR in LTxRs are based on experience with other solid organ transplants which varies by center. There are multiple treatment options that can be considered to treat AMR. The overall aim of AMR treatment is to deplete the circulating antibodies, plasma cells, and B-cells to decrease antibody-mediated graft injury. Combinations of therapies have also been demonstrated in different centers that include plasmapheresis, intravenous immune globulin (IVIG), plasma cell depleting agents, T- or B-cell specific agents, and targeting of the complement pathway.
4.1. Plasmapheresis and intravenous immune globulins
Plasmapheresis and intravenous immune globulins remove antibodies but also reduce cytokine levels. Multiple studies have shown better graft function with the combination of plasma exchange and intravenous immune globulins (IVIG) (65, 66). Plasmapheresis is used to remove or reduce the level of existing antibodies by replacing patient plasma with plasma from healthy individuals. IVIG therapy decreases HLA sensitivity, which in turn lowers the level of HLA antibodies by blocking their ability to attack the transplanted organ.
4.2. Anti-CD20 antibody
Rituximab, an anti-CD20 monoclonal antibody, has been used for AMR treatment. Rituximab binds to pre-B-cells and mature B-lymphocytes that express CD20 (67).
4.3. Complement inhibition
Eculizumab, a monoclonal antibody, is used to inhibit complement component C5 during the formation of the membrane-attack complex, which is the final common pathway of AMR (68).
Although there are multiple treatment options for AMR, the combination of treatments depends on the patient′s status, and center bias still holds dominance. To date, there is no uniformity or consensus treatment for AMR. Different centers are using different combinations of treatments depending on their results. There is a need for more clinical trials, and studies are needed to address the impact of gender, demographics, and populations in different locations (69).
5. Extracellular vesicles
Extracellular vesicles (EVs) are a group of particles that are encapsulated by a lipid bilayer; they are released from different types of cells in the body and are present in body fluids (70). The origin of EVs can be ectosomal or endosomal. They are divided on the basis of size: (a) microvesicles (100–1,000 nm); (b) apoptotic bodies (1,000–5,000 nm); (c) exosomes/small EVs <200 nm (71, 72) (Figure 1). There are multiple terms associated with the nomenclature of EVs and exosomes as per Minimal Information for Studies of Extracellular Vesicles (MISEV) guidelines. Research to identify biomarkers specific to different EVs is ongoing, but currently there are no specific markers associated with EV subtypes. There are different kinds of classifications: (A) based on origin of EVs. EVs originated from endosomes are called “exosomes” and the EVs which are plasma membrane-derived are referred to as “ectosomes”: (B) Based on size, small EVs (<200 nm) and medium/large EVs (>200 nm); (C) on the basis of density low, medium and high; and (D) on the basis of composition CD9/CD63/CD81 etc. (74). Still newer guidelines are needed for the uniformity of the terms related to EVs. Witwer et al. have explained the different nomenclatures of EVs and what is influencing the choice of authors (75). We will be using the term EVs in the current manuscript. The composition of EVs depends on the cells releasing them. This can vary depending upon on the clinical status of the patient (acute and chronic rejection, respiratory viral infections, cancers, etc.) and many other clinical conditions (28, 76–78). EVs are encapsulated by a lipid bilayer and carry specific surface markers (e.g., CD9, CD81, Flotilin, HSP70, tetraspanins, Alix, CD63) along with major histocompatibility complex (MHC) molecules. EVs not only participate in intercellular communication but also have a role in pathological and physiological processes related to diseases.
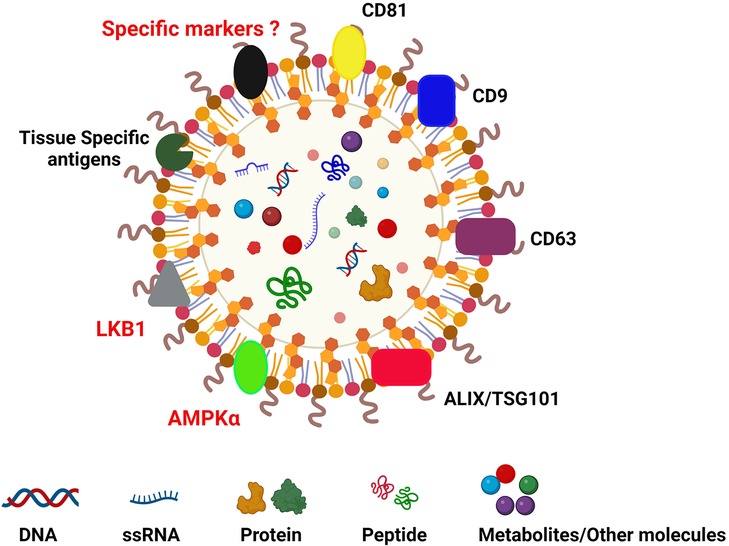
Figure 1. Diagrammatic reprentation of extracellular vesicle carrying CD9, CD63, CD81, TSG101, ALIX, tissue-specific markers (collagen V and K alpha1 tubulin in lung transplant; cardiac myosin and vimentin in cardiac transplant; fibronectin, collagen IV and perlecan in kidney transplant) (73). Images created using BioRENDER.
5.1. EVs in solid organ transplantation
A large number of reports are available on the role of EVs in the activation and regulation of the immune system. It is highly possible that EVs carry intracellular and membrane proteins from the cells of origin, which makes them a potential candidate for biomarkers of various disease states. The ability of EVs to circulate in different bodily fluids (serum/plasma, saliva, urine, bronchial alveolar lavage, cerebrospinal fluid, etc.) makes them a less invasive biomarker compared to tissue biopsies. These biomarkers can be protein/peptide, DNA, RNA, or small metabolites (79–81).
Solid organ transplantation is the last option for patients with end-stage organ failure. Different groups have studied EVs in different solid organ transplant recipients to identify various biomarkers; this has been recently summarized by Garcia et al. (82). The details of EVs as biomarkers in lung transplant recipients are provided in Table 2. Our group is focused on lung transplant, thus we will discuss more about EVs in lung transplant recipients. Failure of normal lung function can be caused by several diseases including cystic fibrosis, idiopathic pulmonary fibrosis, chronic obstructive pulmonary disease, autoimmune disease, and respiratory infections (91). There are many advancements in the last decade in surgical strategies, but the outcomes are still poor (92, 93), and the median survival of LTxRs is limited to ∼5.8 years (41). Immune mechanisms are the driving force behind the development of rejection after transplantation. LTxRs who develop antibody-mediated rejection, have a higher chance of developing CLAD, which depends on the number and severity of early AMR episodes (83, 94).
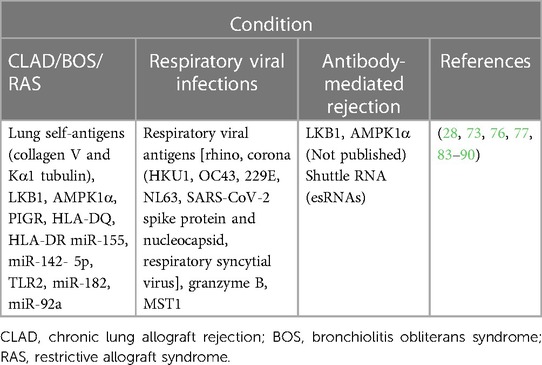
Table 2. Extracellular vesicles as biomarkers in lung transplant recipients with different clinical conditions.
5.2. EVs in chronic lung allograft dysfunction
EVs can be potential biomarkers for LTxRs at risk for the development of CLAD (28, 83, 84). We have also presented evidence that EVs and their contents can differentiate between different phenotypes of CLAD (BOS and RAS) (28, 84). Our results have demonstrated significantly higher levels of lung self-antigens, transcription factors, 20S proteasome, polymeric immunoglobulin receptor (PIGR), and HLA antigens on the EVs from CLAD as compared to stable patients. We further delineated this in different phenotypes of CLAD (BOS/RAS) in a recent study, where we have demonstrated the significantly higher amounts of transcription factor NFkB, 20S Proteasome, PIGR, MHC Class I (W6/32) and II (HLA DQ, DR) in EVs from RAS phenotype of CLAD (28). In addition, mice immunized with EVs isolated from LTxRs with different CLAD phenotypes caused damage to mice lungs with varying severity and type of injury. Previous reports from our laboratory have shown that small EVs play a significant role in development of CLAD (28, 85) but the association of EVs with AMR has not been explored.
5.3. EVs in AMR
Extracellular vesicles are emerging as key biomarkers and mediators in several diseases by carrying specific markers involving both cell-cell interaction and regulation (73, 86, 95, 96). Franzin et al. have shown that EVs can play a pertinent role in tubular senescence and epithelial-to-mesenchymal transition in kidney transplant recipients with AMR (97). In this study authors have characterized the EVs from patients with AMR in Kidney transplants. They have published the data on the difference in presence of pro-inflammatory, pro-aging and profibrotic effects on tubular and endothelial cells in kidney transplant recipients with AMR and controls (No AMR). EVs from AMR patients carried significantly higher amounts of miRNAs which were associated with the renal inflammation, tubular senescence and renal fibrosis as compared to controls. They have also demonstrated that EVs from AMR patients induced Epithelial to mesenchymal transition by significantly decreasing the endothelial markers, such as CD31 and VE-Cadherin and increasing the fibroblast markers Vimentin and collagen I in the endothelial cells treated with EVs from AMR patients. Limited literature is available on EVs and their association with AMR after LTx.
Our preliminary data with LTxRs at the time of development of AMR have shown the presence of lower amounts of LKB1, and AMPK1α in EVs isolated from plasma as compared to stable controls (data not presented). Lower levels of LKB1 and AMPKα in EVs from LTxRs at the time of AMR is in agreement with the in vitro and in vivo studies conducted by Rahman et al. with samples from LTxRs with CLAD (87, 88). On the basis of our published data on CLAD and preliminary investigations with AMR in lung transplant patients (data not shown), there is a possibility that LKB1 and AMPK1α may play a key role in AMR and a biomarker for the development of CLAD. This hypothesis needs further investigation and validation. Rahman et al. have reported the role of the tumor suppressor gene LKB1 in the initiation of epithelial-to-mesenchymal transition resulting in CLAD after lung transplantation in humans and mice (87, 88). Based on our published data on LTxRs with CLAD and unpublished data on those with AMR, we propose LKB1 as a potential EV biomarker for the onset of AMR in LTxRs as shown in Figure 2. Future studies are needed to explore the novel mechanisms of interactions and the role of LKB1 in the pathogenesis of AMR.
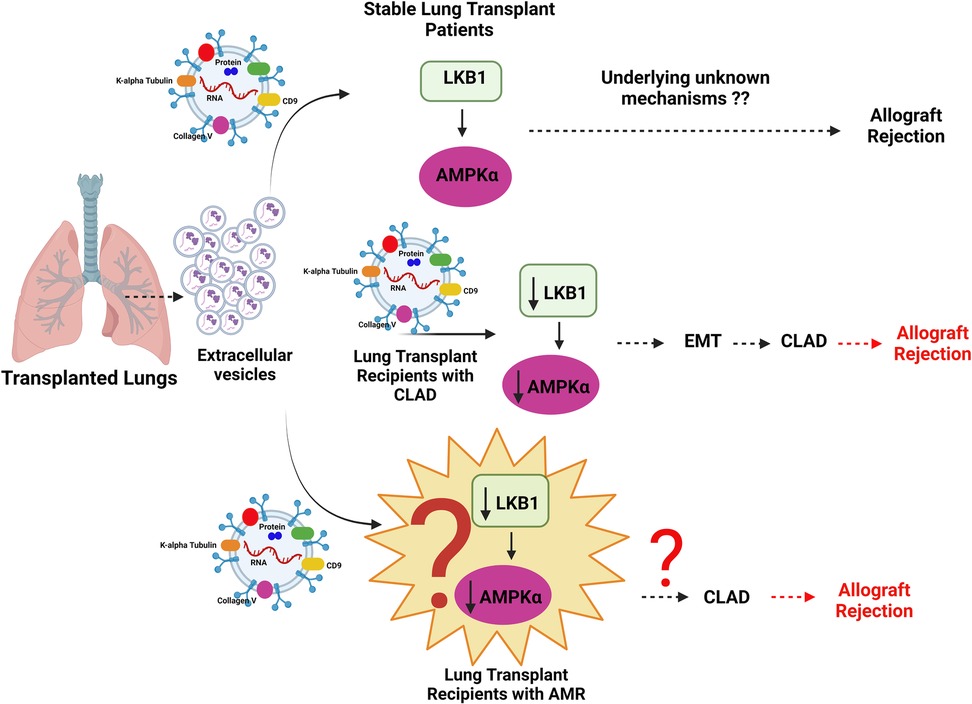
Figure 2. Diagrammatic representation of the mechanism of allograft rejection in lung transplant recipients (LTxRs) with antibody-mediated rejection (AMR). Extracellular vesicles from LTxRs with CLAD carry lower amounts of liver kinase B (LKB1) and AMPKα than those from stable LTxRs. Decreased levels of LKB1 downregulate AMPKα, which can potentially increase epithelial-to-mesenchymal transition followed by chronic lung allograft dysfunction and graft loss. Similarly EVs in AMR can also play a potential role involving LKB1 and AMPKα which can be further associated with development of CLAD followed by graft rejection, this hypothesis needs further investigation. Images created using BioRENDER.
6. Future directions
Due to a lack of experimental data regarding EVs and their underlying mechanisms in LTxRs with AMR, the scope of required EV biomarker research is huge. Comparing the levels of biomarkers in EVs from the plasma isolated from LTxRs before and after the onset of AMR may be predictive of clinical outcomes, i.e., CLAD. Biomarker analysis on EVs in LTx needs further investigation with a larger number of patient samples from multiple centers. In addition, the analysis of EVs needs to be expanded by studying the differences in biomarkers between DSA-positive and DSA-negative samples from LTxRs with AMR. A multi-parametric study considering pre-, current, and post-AMR along with DSA status and following up with CLAD development can help to elucidate the mechanisms of EVs.
Data availability statement
The original contributions presented in the study are included in the article/Supplementary Material, further inquiries can be directed to the corresponding author.
Author contributions
Concept and design: SB and TM. Hypothesis and interpretation: SB, MR. Drafting/writing of the manuscript: SB, TF, RH and TM. Technical support: SB, BF, AA, JC, AG. All authors contributed to the article and approved the submitted version.
Funding
This work was supported by grants from the National Institutes of Health, HL156891 to TM, St. Joseph′s Foundation, and Seed grant from Arizona State University to (TM).
Acknowledgments
We thank AG-A and Kristine Nally for their assistance in preparing this manuscript. Images are created using BioRENDER.
Conflict of interest
The author RH declared that they were an editorial board member of Frontiers, at the time of submission. This had no impact on the peer review process and the final decision.
The remaining authors declare that the research was conducted in the absence of any commercial or financial relationships that could be construed as a potential conflict of interest.
Publisher's note
All claims expressed in this article are solely those of the authors and do not necessarily represent those of their affiliated organizations, or those of the publisher, the editors and the reviewers. Any product that may be evaluated in this article, or claim that may be made by its manufacturer, is not guaranteed or endorsed by the publisher.
References
1. van der Mark SC, Hoek RAS, Hellemons ME. Developments in lung transplantation over the past decade. Eur Respir Rev. (2020) 29(157):1–16. doi: 10.1183/16000617.0132-2019
2. Chambers DC, Cherikh WS, Goldfarb SB, Hayes D Jr, Kucheryavaya AY, Toll AE, et al. The international thoracic organ transplant registry of the international society for heart and lung transplantation: thirty-fifth adult lung and heart-lung transplant report-2018; focus theme: multiorgan transplantation. J Heart Lung Transplant. (2018) 37(10):1169–83. doi: 10.1016/j.healun.2018.07.020
3. Leard LE, Holm AM, Valapour M, Glanville AR, Attawar S, Aversa M, et al. Consensus document for the selection of lung transplant candidates: an update from the international society for heart and lung transplantation. J Heart Lung Transplant. (2021) 40(11):1349–79. doi: 10.1016/j.healun.2021.07.005
4. Khush KK, Cherikh WS, Chambers DC, Goldfarb S, Hayes D Jr, Kucheryavaya AY, et al. The international thoracic organ transplant registry of the international society for heart and lung transplantation: thirty-fifth adult heart transplantation report-2018; focus theme: multiorgan transplantation. J Heart Lung Transplant. (2018) 37(10):1155–68. doi: 10.1016/j.healun.2018.07.022
5. Bery AI, Hachem RR. Antibody-mediated rejection after lung transplantation. Ann Transl Med. (2020) 8(6):411. doi: 10.21037/atm.2019.11.86
6. Witt CA, Gaut JP, Yusen RD, Byers DE, Iuppa JA, Bennett Bain K, et al. Acute antibody-mediated rejection after lung transplantation. J Heart Lung Transplant. (2013) 32(10):1034–40. doi: 10.1016/j.healun.2013.07.004
7. Valenzuela NM, Reed EF. Antibody-mediated rejection across solid organ transplants: manifestations, mechanisms, and therapies. J Clin Invest. (2017) 127(7):2492–504. doi: 10.1172/JCI90597
8. Chong AS, Khiew SH. Transplantation tolerance: don't forget about the B cells. Clin Exp Immunol. (2017) 189(2):171–80. doi: 10.1111/cei.12927
9. Hachem RR. Donor-specific antibodies in lung transplantation. Curr Opin Organ Transplant. (2020) 25(6):563–7. doi: 10.1097/MOT.0000000000000816
10. Roux A, Bendib Le Lan I, Holifanjaniaina S, Thomas KA, Picard C, Grenet D, et al. Characteristics of donor-specific antibodies associated with antibody-mediated rejection in lung transplantation. Front Med. (2017) 4:155. doi: 10.3389/fmed.2017.00155
11. Smith JD, Ibrahim MW, Newell H, Danskine AJ, Soresi S, Burke MM, et al. Pre-transplant donor HLA-specific antibodies: characteristics causing detrimental effects on survival after lung transplantation. J Heart Lung Transplant. (2014) 33(10):1074–82. doi: 10.1016/j.healun.2014.02.033
12. Smith JD, Danskine AJ, Laylor RM, Rose ML, Yacoub MH. The effect of panel reactive antibodies and the donor specific crossmatch on graft survival after heart and heart-lung transplantation. Transpl Immunol. (1993) 1(1):60–5. doi: 10.1016/0966-3274(93)90060-L
13. Courtwright A, Diamond JM, Wood I, Guleria I, Milford E, El-Chemaly S, et al. Detection and clinical impact of human leukocyte antigen antibodies in lung transplantation: a systematic review and meta-analysis. HLA. (2018) 91(2):102–11. doi: 10.1111/tan.13185
14. Valenzuela NM, McNamara JT, Reed EF. Antibody-mediated graft injury: complement-dependent and complement-independent mechanisms. Curr Opin Organ Transplant. (2014) 19(1):33–40. doi: 10.1097/MOT.0000000000000040
15. Miller GG, Destarac L, Zeevi A, Girnita A, McCurry K, Iacono A, et al. Acute humoral rejection of human lung allografts and elevation of C4d in bronchoalveolar lavage fluid. Am J Transplant. (2004) 4(8):1323–30. doi: 10.1111/j.1600-6143.2004.00508.x
16. Wallace WD, Reed EF, Ross D, Lassman CR, Fishbein MC. C4d staining of pulmonary allograft biopsies: an immunoperoxidase study. J Heart Lung Transplant. (2005) 24(10):1565–70. doi: 10.1016/j.healun.2004.11.038
17. Ionescu DN, Girnita AL, Zeevi A, Duquesnoy R, Pilewski J, Johnson B, et al. C4d deposition in lung allografts is associated with circulating anti-HLA alloantibody. Transpl Immunol. (2005) 15(1):63–8. doi: 10.1016/j.trim.2005.05.001
18. Gries CJ, Rue TC, Heagerty PJ, Edelman JD, Mulligan MS, Goss CH. Development of a predictive model for long-term survival after lung transplantation and implications for the lung allocation score. J Heart Lung Transplant. (2010) 29(7):731–8. doi: 10.1016/j.healun.2010.02.007
19. Demir A, Coosemans W, Decaluwe H, De Leyn P, Nafteux P, Van Veer H, et al. Donor-recipient matching in lung transplantation: which variables are important?†. Eur J Cardiothorac Surg. (2015) 47(6):974–83. doi: 10.1093/ejcts/ezu340
20. Nguyen LS, Coutance G, Salem JE, Ouldamar S, Lebreton G, Combes A, et al. Effect of recipient gender and donor-specific antibodies on antibody-mediated rejection after heart transplantation. Am J Transplant. (2019) 19(4):1160–7. doi: 10.1111/ajt.15133
21. Hadjiliadis D, Chaparro C, Reinsmoen NL, Gutierrez C, Singer LG, Steele MP, et al. Pre-transplant panel reactive antibody in lung transplant recipients is associated with significantly worse post-transplant survival in a multicenter study. J Heart Lung Transplant. (2005) 24(7 Suppl):S249–54. doi: 10.1016/j.healun.2004.06.022
22. Kim M, Townsend KR, Wood IG, Boukedes S, Guleria I, Gabardi S, et al. Impact of pretransplant anti-HLA antibodies on outcomes in lung transplant candidates. Am J Respir Crit Care Med. (2014) 189(10):1234–9. doi: 10.1164/rccm.201312-2160OC
23. Courtwright AM, Cao S, Wood I, Mallidi HR, Kawasawa J, Moniodis A, et al. Clinical outcomes of lung transplantation in the presence of donor-specific antibodies. Ann Am Thorac Soc. (2019) 16(9):1131–7. doi: 10.1513/AnnalsATS.201812-869OC
24. Hulbert AL, Pavlisko EN, Palmer SM. Current challenges and opportunities in the management of antibody-mediated rejection in lung transplantation. Curr Opin Organ Transplant. (2018) 23(3):308–15. doi: 10.1097/MOT.0000000000000537
25. Levine DJ, Glanville AR, Aboyoun C, Belperio J, Benden C, Berry GJ, et al. Antibody-mediated rejection of the lung: a consensus report of the international society for heart and lung transplantation. J Heart Lung Transplant. (2016) 35(4):397–406. doi: 10.1016/j.healun.2016.01.1223
26. Charya AV, Ponor IL, Cochrane A, Levine D, Philogene M, Fu YP, et al. Clinical features and allograft failure rates of pulmonary antibody-mediated rejection categories. J Heart Lung Transplant. (2023) 42(2):226–35. doi: 10.1016/j.healun.2022.09.012
27. Berry G, Burke M, Andersen C, Angelini A, Bruneval P, Calabrese F, et al. Pathology of pulmonary antibody-mediated rejection: 2012 update from the pathology council of the ISHLT. J Heart Lung Transplant. (2013) 32(1):14–21. doi: 10.1016/j.healun.2012.11.005
28. Bansal S, Arjuna A, Perincheri S, Poulson C, Bremner RM, Smith MA, et al. Restrictive allograft syndrome vs bronchiolitis obliterans syndrome: immunological and molecular characterization of circulating exosomes. J Heart Lung Transplant. (2022) 41(1):24–33. doi: 10.1016/j.healun.2021.09.001
29. Sato M. Bronchiolitis obliterans syndrome and restrictive allograft syndrome after lung transplantation: why are there two distinct forms of chronic lung allograft dysfunction? Ann Transl Med. (2020) 8(6):418. doi: 10.21037/atm.2020.02.159
30. Djamali A, Kaufman DB, Ellis TM, Zhong W, Matas A, Samaniego M. Diagnosis and management of antibody-mediated rejection: current status and novel approaches. Am J Transplant. (2014) 14(2):255–71. doi: 10.1111/ajt.12589
31. Takemoto SK, Zeevi A, Feng S, Colvin RB, Jordan S, Kobashigawa J, et al. National conference to assess antibody-mediated rejection in solid organ transplantation. Am J Transplant. (2004) 4(7):1033–41. doi: 10.1111/j.1600-6143.2004.00500.x
32. Garces JC, Giusti S, Staffeld-Coit C, Bohorquez H, Cohen AJ, Loss GE. Antibody-mediated rejection: a review. Ochsner J. (2017) 17(1):46–55.28331448
33. Nankivell BJ, Alexander SI. Rejection of the kidney allograft. N Engl J Med. (2010) 363(15):1451–62. doi: 10.1056/NEJMra0902927
34. Farkash EA, Colvin RB. Diagnostic challenges in chronic antibody-mediated rejection. Nat Rev Nephrol. (2012) 8(5):255–7. doi: 10.1038/nrneph.2012.61
35. Sis B, Halloran PF. Endothelial transcripts uncover a previously unknown phenotype: C4d-negative antibody-mediated rejection. Curr Opin Organ Transplant. (2010) 15(1):42–8. doi: 10.1097/MOT.0b013e3283352a50
36. Hidalgo LG, Sis B, Sellares J, Campbell PM, Mengel M, Einecke G, et al. NK cell transcripts and NK cells in kidney biopsies from patients with donor-specific antibodies: evidence for NK cell involvement in antibody-mediated rejection. Am J Transplant. (2010) 10(8):1812–22. doi: 10.1111/j.1600-6143.2010.03201.x
37. Zhang Q, Reed EF. The importance of non-HLA antibodies in transplantation. Nat Rev Nephrol. (2016) 12(8):484–95. doi: 10.1038/nrneph.2016.88
38. Brasile L, Rodman E, Shield CF 3rd, Clarke J, Cerilli J. The association of antivascular endothelial cell antibody with hyperacute rejection: a case report. Surgery. (1986) 99(5):637–40.3518110
39. Fernandez R, Chiu S, Raparia K, Garcha P, Farver C, Budev M, et al. Humoral human lung allograft rejection by tissue-restricted non-HLA antibodies. Ann Thorac Surg. (2016) 102(4):e339–41. doi: 10.1016/j.athoracsur.2016.03.042
40. Subramanian V, Ramachandran S, Banan B, Bharat A, Wang X, Benshoff N, et al. Immune response to tissue-restricted self-antigens induces airway inflammation and fibrosis following murine lung transplantation. Am J Transplant. (2014) 14(10):2359–66. doi: 10.1111/ajt.12908
41. Parulekar AD, Kao CC. Detection, classification, and management of rejection after lung transplantation. J Thorac Dis. (2019) 11(Suppl 14):S1732–S9. doi: 10.21037/jtd.2019.03.83
42. Krishnamoorthy S, Kyeso Y. Challenges of diagnosing antibody-mediated rejection: the role of invasive and non-invasive biomarkers. Medicina. (2021) 57(5):1–9. doi: 10.3390/medicina57050439
43. Girnita AL, Lee TM, McCurry KR, Baldwin WM 3rd, Yousem SA, Detrick B, et al. Anti-human leukocyte antigen antibodies, vascular C4d deposition and increased soluble c4d in broncho-alveolar lavage of lung allografts. Transplantation. (2008) 86(2):342–7. doi: 10.1097/TP.0b013e31817cf2e2
44. Badesch DB, Zamora M, Fullerton D, Weill D, Tuder R, Grover F, et al. Pulmonary capillaritis: a possible histologic form of acute pulmonary allograft rejection. J Heart Lung Transplant. (1998) 17(4):415–22.9588587
45. Cohen D, Colvin RB, Daha MR, Drachenberg CB, Haas M, Nickeleit V, et al. Pros and cons for C4d as a biomarker. Kidney Int. (2012) 81(7):628–39. doi: 10.1038/ki.2011.497
46. Magro CM, Deng A, Pope-Harman A, Waldman WJ, Bernard Collins A, Adams PW, et al. Humorally mediated posttransplantation septal capillary injury syndrome as a common form of pulmonary allograft rejection: a hypothesis. Transplantation. (2002) 74(9):1273–80. doi: 10.1097/00007890-200211150-00013
47. Magro CM, Klinger DM, Adams PW, Orosz CG, Pope-Harman AL, Waldman WJ, et al. Evidence that humoral allograft rejection in lung transplant patients is not histocompatibility antigen-related. Am J Transplant. (2003) 3(10):1264–72. doi: 10.1046/j.1600-6143.2003.00229.x
48. Roden AC, Aisner DL, Allen TC, Aubry MC, Barrios RJ, Beasley MB, et al. Diagnosis of acute cellular rejection and antibody-mediated rejection on lung transplant biopsies: a perspective from members of the pulmonary pathology society. Arch Pathol Lab Med. (2017) 141(3):437–44. doi: 10.5858/arpa.2016-0459-SA
49. Roden AC, Maleszewski JJ, Yi ES, Jenkins SM, Gandhi MJ, Scott JP, et al. Reproducibility of complement 4d deposition by immunofluorescence and immunohistochemistry in lung allograft biopsies. J Heart Lung Transplant. (2014) 33(12):1223–32. doi: 10.1016/j.healun.2014.06.006
50. DeNicola MM, Weigt SS, Belperio JA, Reed EF, Ross DJ, Wallace WD. Pathologic findings in lung allografts with anti-HLA antibodies. J Heart Lung Transplant. (2013) 32(3):326–32. doi: 10.1016/j.healun.2012.11.018
51. Westall GP, Snell GI, McLean C, Kotsimbos T, Williams T, Magro C. C3d and C4d deposition early after lung transplantation. J Heart Lung Transplant. (2008) 27(7):722–8. doi: 10.1016/j.healun.2008.03.018
52. Mengel M, Sis B, Haas M, Colvin RB, Halloran PF, Racusen LC, et al. Banff 2011 meeting report: new concepts in antibody-mediated rejection. Am J Transplant. (2012) 12(3):563–70. doi: 10.1111/j.1600-6143.2011.03926.x
53. Haas M, Sis B, Racusen LC, Solez K, Glotz D, Colvin RB, et al. Banff 2013 meeting report: inclusion of c4d-negative antibody-mediated rejection and antibody-associated arterial lesions. Am J Transplant. (2014) 14(2):272–83. doi: 10.1111/ajt.12590
54. Orandi BJ, Alachkar N, Kraus ES, Naqvi F, Lonze BE, Lees L, et al. Presentation and outcomes of C4d-negative antibody-mediated rejection after kidney transplantation. Am J Transplant. (2016) 16(1):213–20. doi: 10.1111/ajt.13434
55. Aguilar PR, Carpenter D, Ritter J, Yusen RD, Witt CA, Byers DE, et al. The role of C4d deposition in the diagnosis of antibody-mediated rejection after lung transplantation. Am J Transplant. (2018) 18(4):936–44. doi: 10.1111/ajt.14534
56. Mauiyyedi S, Pelle PD, Saidman S, Collins AB, Pascual M, Tolkoff-Rubin NE, et al. Chronic humoral rejection: identification of antibody-mediated chronic renal allograft rejection by C4d deposits in peritubular capillaries. J Am Soc Nephrol. (2001) 12(3):574–82. doi: 10.1681/ASN.V123574
57. Lobo LJ, Aris RM, Schmitz J, Neuringer IP. Donor-specific antibodies are associated with antibody-mediated rejection, acute cellular rejection, bronchiolitis obliterans syndrome, and cystic fibrosis after lung transplantation. J Heart Lung Transplant. (2013) 32(1):70–7. doi: 10.1016/j.healun.2012.10.007
58. Halloran PF, Reeve JP, Pereira AB, Hidalgo LG, Famulski KS. Antibody-mediated rejection, T cell-mediated rejection, and the injury-repair response: new insights from the genome Canada studies of kidney transplant biopsies. Kidney Int. (2014) 85(2):258–64. doi: 10.1038/ki.2013.300
59. Zeevi A, Lunz J, Feingold B, Shullo M, Bermudez C, Teuteberg J, et al. Persistent strong anti-HLA antibody at high titer is complement binding and associated with increased risk of antibody-mediated rejection in heart transplant recipients. J Heart Lung Transplant. (2013) 32(1):98–105. doi: 10.1016/j.healun.2012.09.021
60. Loupy A, Lefaucheur C, Vernerey D, Prugger C, Duong van Huyen JP, Mooney N, et al. Complement-binding anti-HLA antibodies and kidney-allograft survival. N Engl J Med. (2013) 369(13):1215–26. doi: 10.1056/NEJMoa1302506
61. Angaswamy N, Tiriveedhi V, Sarma NJ, Subramanian V, Klein C, Wellen J, et al. Interplay between immune responses to HLA and non-HLA self-antigens in allograft rejection. Hum Immunol. (2013) 74(11):1478–85. doi: 10.1016/j.humimm.2013.07.002
62. Cunningham AC, Zhang JG, Moy JV, Ali S, Kirby JA. A comparison of the antigen-presenting capabilities of class II MHC-expressing human lung epithelial and endothelial cells. Immunology. (1997) 91(3):458–63. doi: 10.1046/j.1365-2567.1997.d01-2249.x
63. Jankowska Gan E, Agashe VV, Lema DA, Zhou Y, Gonzalez Bosc L, Sullivan JA, et al. Donor HLA-DR drives the development of De Novo autoimmunity following lung and heart transplantation. Transplant Direct. (2020) 6(10):e607. doi: 10.1097/TXD.0000000000001062
64. Safavi S, Robinson DR, Soresi S, Carby M, Smith JD. De novo donor HLA-specific antibodies predict development of bronchiolitis obliterans syndrome after lung transplantation. J Heart Lung Transplant. (2014) 33(12):1273–81. doi: 10.1016/j.healun.2014.07.012
65. Montgomery RA, Zachary AA, Racusen LC, Leffell MS, King KE, Burdick J, et al. Plasmapheresis and intravenous immune globulin provides effective rescue therapy for refractory humoral rejection and allows kidneys to be successfully transplanted into cross-match-positive recipients. Transplantation. (2000) 70(6):887–95. doi: 10.1097/00007890-200009270-00006
66. Timofeeva OA, Choe J, Alsammak M, Yoon EJ, Geier SS, Mathew L, et al. Guiding therapeutic plasma exchange for antibody-mediated rejection treatment in lung transplant recipients - a retrospective study. Transpl Int. (2021) 34(4):700–8. doi: 10.1111/tri.13825
67. Golay J, Semenzato G, Rambaldi A, Foa R, Gaidano G, Gamba E, et al. Lessons for the clinic from rituximab pharmacokinetics and pharmacodynamics. MAbs. (2013) 5(6):826–37. doi: 10.4161/mabs.26008
68. Dawson KL, Parulekar A, Seethamraju H. Treatment of hyperacute antibody-mediated lung allograft rejection with eculizumab. J Heart Lung Transplant. (2012) 31(12):1325–6. doi: 10.1016/j.healun.2012.09.016
69. Otani S, Davis AK, Cantwell L, Ivulich S, Pham A, Paraskeva MA, et al. Evolving experience of treating antibody-mediated rejection following lung transplantation. Transpl Immunol. (2014) 31(2):75–80. doi: 10.1016/j.trim.2014.06.004
70. van Niel G, D'Angelo G, Raposo G. Shedding light on the cell biology of extracellular vesicles. Nat Rev Mol Cell Biol. (2018) 19(4):213–28. doi: 10.1038/nrm.2017.125
71. Kalluri R, LeBleu VS. The biology, function, and biomedical applications of exosomes. Science. (2020) 367(6478):1–15. doi: 10.1126/science.aau6977
72. Bansal S, Sharma M, Ranjithkumar R, Mohanakumar T. The role of exosomes in allograft immunity. Cell Immunol. (2018) 331:85–92. doi: 10.1016/j.cellimm.2018.06.003
73. Ravichandran R, Bansal S, Rahman M, Sureshbabu A, Sankpal N, Fleming T, et al. Extracellular vesicles mediate immune responses to tissue-associated self-antigens: role in solid organ transplantations. Front Immunol. (2022) 13:861583. doi: 10.3389/fimmu.2022.861583
74. Thery C, Witwer KW, Aikawa E, Alcaraz MJ, Anderson JD, Andriantsitohaina R, et al. Minimal information for studies of extracellular vesicles 2018 (MISEV2018): a position statement of the international society for extracellular vesicles and update of the MISEV2014 guidelines. J Extracell Vesicles. (2018) 7(1):1535750. doi: 10.1080/20013078.2018.1535750
75. Witwer KW, Thery C. Extracellular vesicles or exosomes? On primacy, precision, and popularity influencing a choice of nomenclature. J Extracell Vesicles. (2019) 8(1):1648167. doi: 10.1080/20013078.2019.1648167
76. Gunasekaran M, Bansal S, Ravichandran R, Sharma M, Perincheri S, Rodriguez F, et al. Respiratory viral infection in lung transplantation induces exosomes that trigger chronic rejection. J Heart Lung Transplant. (2020) 39(4):379–88. doi: 10.1016/j.healun.2019.12.009
77. Bansal S, Limaye AP, Lee J, Fleming T, Poulson C, Omar A, et al. Circulating exosomes induced by respiratory viral infections in lung transplant recipients activate cellular stress, innate immune pathways and epithelial to mesenchymal transition. Transpl Immunol. (2021) 69:101480. doi: 10.1016/j.trim.2021.101480
78. Arcasoy SM, Kotloff RM. Lung transplantation. N Engl J Med. (1999) 340(14):1081–91. doi: 10.1056/NEJM199904083401406
79. Gyorgy B, Szabo TG, Pasztoi M, Pal Z, Misjak P, Aradi B, et al. Membrane vesicles, current state-of-the-art: emerging role of extracellular vesicles. Cell Mol Life Sci. (2011) 68(16):2667–88. doi: 10.1007/s00018-011-0689-3
80. Kowal J, Arras G, Colombo M, Jouve M, Morath JP, Primdal-Bengtson B, et al. Proteomic comparison defines novel markers to characterize heterogeneous populations of extracellular vesicle subtypes. Proc Natl Acad Sci U S A. (2016) 113(8):E968–77. doi: 10.1073/pnas.1521230113
81. Soekmadji C, Li B, Huang Y, Wang H, An T, Liu C, et al. The future of extracellular vesicles as theranostics - an ISEV meeting report. J Extracell Vesicles. (2020) 9(1):1809766. doi: 10.1080/20013078.2020.1809766
82. Romero-Garcia N, Huete-Acevedo J, Mas-Bargues C, Sanz-Ros J, Dromant M, Badenes R, et al. Extracellular vesicles: the future of diagnosis in solid organ transplantation? Int J Mol Sci. (2023) 24(6):1–22. doi: 10.3390/ijms24065102
83. Gunasekaran M, Xu Z, Nayak DK, Sharma M, Hachem R, Walia R, et al. Donor-derived exosomes with lung self-antigens in human lung allograft rejection. Am J Transplant. (2017) 17(2):474–84. doi: 10.1111/ajt.13915
84. Sharma M, Gunasekaran M, Ravichandran R, Fisher CE, Limaye AP, Hu C, et al. Circulating exosomes with lung self-antigens as a biomarker for chronic lung allograft dysfunction: a retrospective analysis. J Heart Lung Transplant. (2020) 39(11):1210–9. doi: 10.1016/j.healun.2020.07.001
85. Bansal S, Tokman S, Fleming T, Maine GN, Sanborn K, Hachem R, et al. SARS-CoV-2 infection in lung transplant recipients induces circulating exosomes with SARS-CoV-2 spike protein S2. Clin Transl Med. (2021) 11(11):e576. doi: 10.1002/ctm2.576
86. Mohanakumar T, Sharma M, Bansal S, Ravichandran R, Smith MA, Bremner RM. A novel mechanism for immune regulation after human lung transplantation. J Thorac Cardiovasc Surg. (2019) 157(5):2096–106. doi: 10.1016/j.jtcvs.2018.12.105
87. Rahman M, Ravichandran R, Sankpal NV, Bansal S, Sureshbabu A, Fleming T, et al. Downregulation of a tumor suppressor gene LKB1 in lung transplantation as a biomarker for chronic murine lung allograft rejection. Cell Immunol. (2023) 386:104690. doi: 10.1016/j.cellimm.2023.104690
88. Rahman M, Ravichandran R, Bansal S, Sanborn K, Bowen S, Eschbacher J, et al. Novel role for tumor suppressor gene, liver kinase B1, in epithelial-mesenchymal transition leading to chronic lung allograft dysfunction. Am J Transplant. (2022) 22(3):843–52. doi: 10.1111/ajt.16903
89. Bansal S, McGilvrey M, Garcia-Mansfield K, Sharma R, Bremner RM, Smith MA, et al. Global proteomics analysis of circulating extracellular vesicles isolated from lung transplant recipients. ACS Omega. (2020) 5(24):14360–9. doi: 10.1021/acsomega.0c00859
90. Gregson AL, Hoji A, Injean P, Poynter ST, Briones C, Palchevskiy V, et al. Altered exosomal RNA profiles in bronchoalveolar lavage from lung transplants with acute rejection. Am J Respir Crit Care Med. (2015) 192(12):1490–503. doi: 10.1164/rccm.201503-0558OC
91. Rana A, Gruessner A, Agopian VG, Khalpey Z, Riaz IB, Kaplan B, et al. Survival benefit of solid-organ transplant in the United States. JAMA Surg. (2015) 150(3):252–9. doi: 10.1001/jamasurg.2014.2038
92. Merlo A, Egan TM. Survival after lung transplant: when is survival long enough? J Heart Lung Transplant. (2022) 41(7):874–6. doi: 10.1016/j.healun.2022.04.004
93. Thabut G, Mal H. Outcomes after lung transplantation. J Thorac Dis. (2017) 9(8):2684–91. doi: 10.21037/jtd.2017.07.85
94. Chambers DC, Yusen RD, Cherikh WS, Goldfarb SB, Kucheryavaya AY, Khusch K, et al. The registry of the international society for heart and lung transplantation: thirty-fourth adult lung and heart-lung transplantation report-2017; focus theme: allograft ischemic time. J Heart Lung Transplant. (2017) 36(10):1047–59. doi: 10.1016/j.healun.2017.07.016
95. Rak J. Extracellular vesicles - biomarkers and effectors of the cellular interactome in cancer. Front Pharmacol. (2013) 4(21):1–14. doi: 10.3389/fphar.2013.00021
96. Yanez-Mo M, Siljander PR, Andreu Z, Zavec AB, Borras FE, Buzas EI, et al. Biological properties of extracellular vesicles and their physiological functions. J Extracell Vesicles. (2015) 4:27066. doi: 10.3402/jev.v4.27066
Keywords: antibody mediated rejection (AMR), extracellular vesicle (EV), donor specific antibodies, lung transplanation, chronic lung allograft dysfunction (CLAD)
Citation: Bansal S, Arjuna A, Franz B, Guerrero-Alba A, Canez J, Fleming T, Rahman M, Hachem R and Mohanakumar T (2023) Extracellular vesicles: a potential new player in antibody-mediated rejection in lung allograft recipients. Front. Transplant. 2:1248987. doi: 10.3389/frtra.2023.1248987
Received: 27 June 2023; Accepted: 22 August 2023;
Published: 4 September 2023.
Edited by:
Madhav C. Menon, Yale University, United StatesReviewed by:
Marilia Cascalho, University of Michigan, United StatesAndrzej Rydzewski, CSK MSWiA, Poland
© 2023 Bansal, Arjuna, Franz, Guerrero-Alba, Canez, Fleming, Rahman, Hachem and Mohanakumar. This is an open-access article distributed under the terms of the Creative Commons Attribution License (CC BY). The use, distribution or reproduction in other forums is permitted, provided the original author(s) and the copyright owner(s) are credited and that the original publication in this journal is cited, in accordance with accepted academic practice. No use, distribution or reproduction is permitted which does not comply with these terms.
*Correspondence: Sandhya Bansal c2FuZGh5YS5iYW5zYWxAZGlnbml0eWhlYWx0aC5vcmc=; c2FuZGh5YWJhbnNhbEBnbWFpbC5jb20=
Abbreviations AMR, antibody-mediated rejection; APC, antigen presenting cell; BOS, bronchiolitis obliterative syndrome; CLAD, chronic lung allograft dysfunction; DSAs, donor-specific antibodies; EVs, extracellular vesicles; ISHLT, International Society of Heart and Lung Transplantation; IVIG, intravenous immune globulin; LTx, lung transplantation; LTxR, lung transplant recipient; PRA, panel reactive antibodies; RAS, restrictive allograft syndrome.