- 1Division of Transplant Surgery, Department of Surgery, University of Virginia Health System, Charlottesville, VA, United States
- 2Department of Public Health Sciences, University of Virginia, Charlottesville, VA, United States
Introduction: Following procurement, the liver graft is exposed to an ischemic period that triggers several pathophysiologic changes in response to oxygen deprivation. Therefore, the goal during organ preservation is to attenuate such response and provide an adequate environment that prepares the graft for its metabolic reactivation following implantation. This has been widely achieved via static cold storage preservation, where the maintenance of the graft using cold preservation solutions reduce its metabolic activity and confer cytoprotection until transplantation. However, despite being the gold standard for organ preservation, static cold storage holds several disadvantages. In addition, the ongoing organ shortage has led to the use of unconventional grafts that could benefit from therapies pre-transplant. Organ preservation via machine perfusion systems appears as a promising solution to address both.
Methods: Here, we aim to present a state-of-the-art narrative review regarding liver graft modification options using machine perfusion systems in combination with adjuvant strategies including immunomodulation, gene therapy and pharmacotherapy.
Results: Available reports are scarce and mostly on experimental animal models. Most of the literature reflects the use of normothermic or subnormothermic machine perfusion devices given that these particular type of machine allows for a metabolically active organ, and therefore facilitates its modification. Although limited, promising findings in available reports suggest that organ preservation using machine perfusion system when combined with alternative therapies can be feasible and safe strategies for graft modification.
Discussion: Further research on clinical settings are needed to better elucidate the true effect of graft modification pre-transplant on short- and long-term graft and patient survival. There is a long way ahead to develop guidelines and approve these novel therapies for clinical practice. However, the path looks promising.
Introduction
Despite having a futuristic ring to it, the concept of organ preservation using machine perfusion (MP) systems is far from modern. In fact, Belzer et al. performed the first successful human transplant using a machine-perfused graft more than half a century ago (1). The procedure took place 1 year after successfully achieving organ viability following ex vivo perfusion of canine kidneys in 1967 (2). The team decided to use the model for transplantation of a kidney graft in a 47-year-old man with a history of amyloidosis without a chance of alternative treatment (1). The authors’ promising results were then supported by many other studies, demonstrating MP superiority against static cold storage (SCS) in different areas, including a lower incidence of delayed graft function and improved graft survival (3, 4). Technical and logistics advances over the years have since allowed MP to become one of the key preservation methods for kidney transplantation (3, 4). Moreover, its clinical applications have reached different areas in transplantation, including organ preservation for lung, heart, and liver transplantations (LT).
Regardless of being around for many years, it was only in 2010 that the first clinical trial using MP for human liver grafts was published (5). Over a span of 4 years, 20 liver grafts from donors after brain death (DBD) were preserved from 3–7 h using a hypothermic machine perfusion (HMP) device (5). In the clinical trial, HMP was demonstrated to be a safe and feasible option, with potential superiority to SCS in terms of liver injury and postoperative complications (5). Since then, several experimental studies, along with a handful of clinical trials, have aimed to demonstrate the superiority of dynamic preservation methods—MP systems—in the reduction of ischemia reperfusion injury of the liver graft when compared to SCS (6–9). Thus, under the premise of reducing graft injury before transplantation, research efforts have been toward the evaluation of the potential role of MP as a pathway to lessen the unfavorable shortage of liver organs by increasing the usage of all available organs.
Hence, subsequent randomized and non-randomized clinical trials have included grafts that are often discarded due to poor quality, such as those from donors after cardiac death (DCD) and extended criteria donors (ECD) (Supplementary Table S1). Similarly, research involving the development and implementation of innovative techniques, such as hypothermic oxygenated perfusion (HOPE), dual-HOPE, and normothermic machine perfusion (NMP), have been on the rise (10–20). However, the potential benefits and superiority of MP not only encompass ischemic-related injury, but an umbrella of possibilities, including graft viability assessment and graft modification, which SCS is unable to offer. Here, we aim to present a state-of-the-art review regarding liver graft modification options using MP systems.
Importance of graft preservation and overview of dynamic preservation techniques
After procurement, the liver graft is exposed to an ischemic period known as cold ischemia. During this period, the ischemic insult triggers several pathophysiologic changes in response to oxygen deprivation. Initial changes include the activation of Kupffer cells and sinusoidal endothelial cells, leading to a complex immunologic response that potentiates hepatocellular damage (21). In addition, hepatic ischemia leads to a detrimental metabolic cascade preceded by a decrease in oxidative phosphorylation, leading to ATP depletion, an increase in anaerobic metabolism, hepatocyte acidosis, dysregulation of ATP-dependent ion exchange mechanisms, mitochondrial dysfunction, cellular swelling, and death (21, 22). Moreover, damage to the sinusoidal endothelial cells also leads to damage of hepatic microcirculation—moderated by, among others, the release of thromboxane A2, which plays an important role in liver dysfunction after implantation (23). Although this complex process is still not fully elucidated, it is known that hepatic ischemia is a key determinant in the development of early allograft dysfunction and negative post-transplant outcomes (24).
Therefore, the goal during organ preservation is to attenuate the intense hepatic response to ischemia and to provide an adequate environment that prepares the graft for its metabolic reactivation after implantation (24). This has been widely achieved via SCS to reduce the graft metabolic activity along with the use of different hypothermic solutions to confer cytoprotection (25). However, despite being the gold standard for organ preservation, static cold storage holds several disadvantages, including a prolonged cold ischemia time and the inability to assess graft viability before implantation (24, 25). Hence, in an attempt to tackle the shortcomings of SCS, in summation with the need of more suitable liver grafts for transplantation, dynamic preservation techniques using a machine perfusion device came into sight as a promising option.
Different MP systems are currently under consideration for LT. Most of them are not yet fully approved and are still undergoing experimental and clinical trials. Dynamic preservation techniques aim to provide a more supervised environment to the graft when compared to SCS. It involves perfusion of the graft under specific circumstances, including controlled temperature, perfusion flow, and special perfusate (25–27). Currently, the two types of MP most studied for use in liver transplantation are hypothermic (HMP) and normothermic (NMP). In the former, as the name implies, preservation occurs under temperatures of approximately 0–8°C, while the latter aims to provide a more physiological environment, with preservation under temperatures of approximately 35–38°C. In addition, different types of perfusate are available as preservation solutions. Common perfusates used during machine perfusion usually consist of enriched solutions—with electrolytes, buffer components, antioxidants, and amino acids among others—that facilitate organ preservation and nutrient delivery to the organs. Common solutions used for preservation in hypothermic/subnormothermic devices include the Euro-Collins solution, University of Wisconsin (UW) solution, and Histidine-tryptophan-ketoglutarate (HTK) solution (25). During NMP, solutions that provide a more physiologic environment are needed. Therefore, whole blood or blood-based solutions, such as the Steen solution or Organ Care System perfusate, are commonly used. Detailed components and descriptions of perfusate solutions are vastly described elsewhere (25). The preservation time varies, usually <12 h, with the longest viable time to date recently achieved by Clavien et al., who performed a successful transplantation of a patient whose implanted graft spent >3 days in ex situ preservation using NMP (28). Additional types and important considerations of each are elucidated in Table 1. Although evidence is still scarce, organ preservation under such controlled environments have opened the door for unlimited potential in graft modification and the assessment before LT (Figure 1). Important modalities are discussed below.
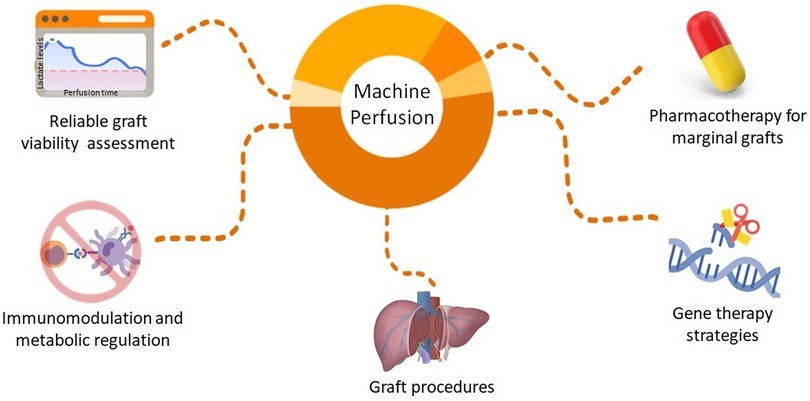
Figure 1. Future directions to improve organ quality by graft modification during machine perfusion of liver grafts.
Graft modification: possibilities beyond the horizon
Almost 10% of the livers recovered for transplant are not transplanted in the United States (29). Evidence shows that livers from DCD are far more likely to be discarded when compared to grafts from DBD (26.6% vs. 7.1%, respectively) (29). This comes as no surprise, taking into account the positive correlation between the prolonged warm ischemia experienced by these grafts and the detrimental effects on the graft leading to poor graft function, biliary complications, ischemic cholangiopathy, and worst graft survival (20, 30). Nevertheless, increasing reports suggest that outcomes after LT with DCD may be comparable to DBD when preservation is aided by machine perfusion (20). Therefore, the discard rate of these grafts could be further reduced, allowing a new resource to supply the donor pool.
Although DCD accounts for an important portion of liver discard, multiple other conditions affect graft usage. With the increasing obesity epidemic, macrosteatosis represents another important factor. In addition, livers with a prolonged cold ischemia time and those from donors with positive hepatitis C or B virus are also commonly discarded (31). Moreover, the organ shortage has forced transplant teams to be more flexible when it comes to selection criteria, risking postoperative outcomes when using these types of grafts. Therefore, research efforts are toward effective methods that provide treatment or amelioration of such aggravates (Table 2).
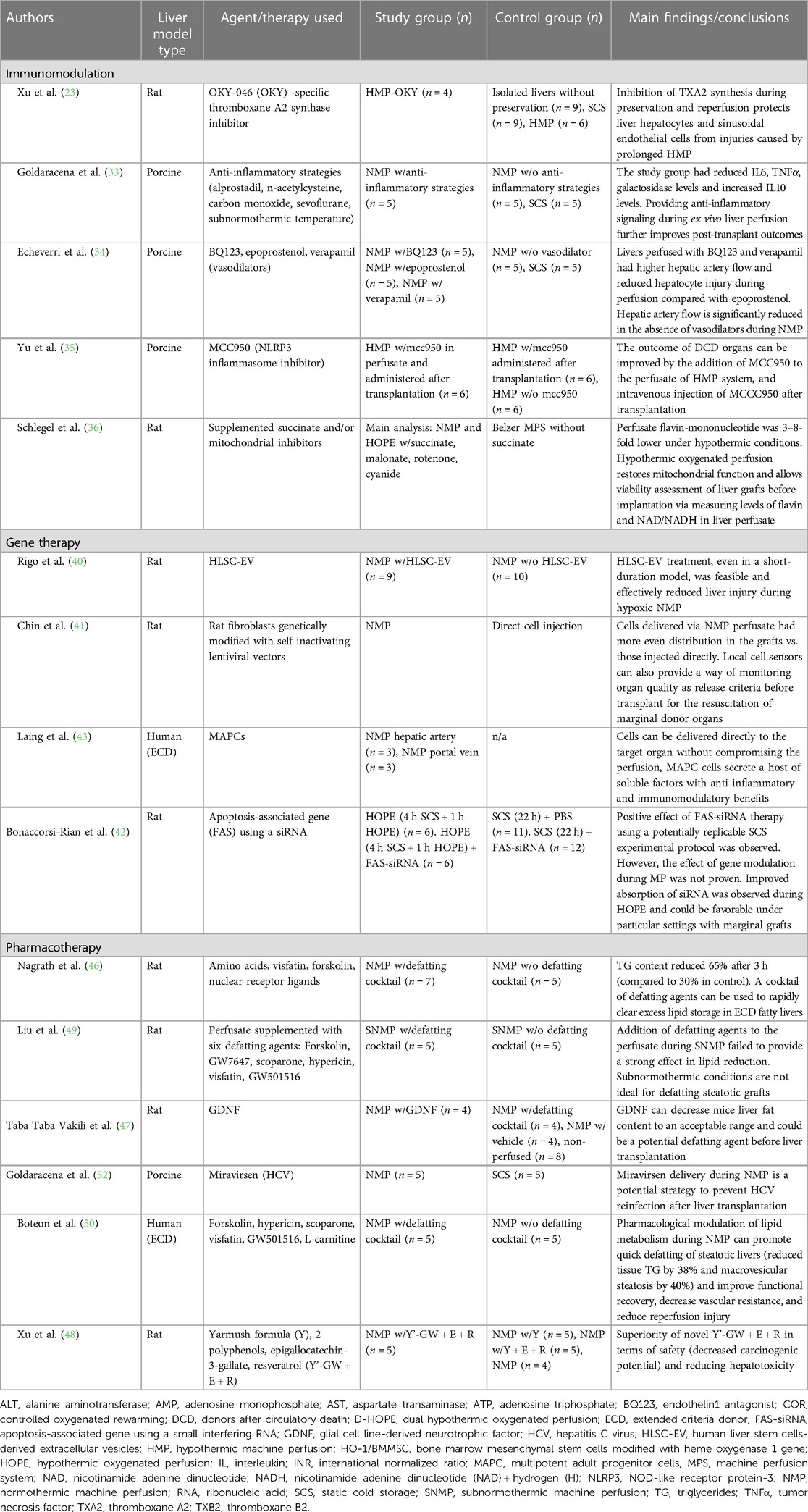
Table 2. Available literature related to liver graft modification using machine perfusion according to graft modification type.
Immunomodulation and metabolic regulation of the liver while on MP
The intense immunologic response triggered by hepatic ischemia denotes a threat for graft viability and transplant success. Therefore, dampening known inflammatory mediators of ischemic injury is a promising strategy (32). In addition, the opposite remains true, and upregulating anti-inflammatory pathways may also play an important role (32). Goldaracena et al. demonstrated improved endothelial cell function and graft viability after the addition of prostaglandin E1, n-acetylcysteine, carbon monoxide, and sevoflurane in an ex vivo subnormothermic model using porcine livers (33). These additives were considered due to their anti-inflammatory, cytoprotective, and vasodilator properties (33). A reduction in hepatocyte injury and inflammatory mediators (IL-6, tumor necrosis factor α) has also been found after treatment with vasodilator agents, particularly with endothelin 1 antagonist BQ123 and verapamil during NMP in porcine models (34). Additional strategies further support the use of MP as a promising tool to deliver immune-specific therapies (35).
In addition to targeting immunologic pathways, metabolic-specific strategies have also demonstrated great potential. Previous experimental studies on animal models support this idea. Xu et al. (23) added a specific thromboxane A2 synthase inhibitor to the perfusate during HMP. The authors found that grafts in the study group demonstrated reduced portal pressure during reperfusion, lower lactate dehydrogenase levels in the perfusate, and significant improvement in tissue edema and hepatocellular necrosis (23). Recently, Schlegel et al. implemented their animal model to a clinical setting and evaluated mitochondrial metabolism (36). The authors added succinate and mitochondrial inhibitors during HOPE and NMP of DCD rat liver. Their findings suggest that the HOPE treatment provides mitochondrial protection from ischemic injury in a higher degree than normothermic condition, and even allows graft viability assessment pre-transplantation (36).
Although there are currently not enough data to determine which particular strategy contributes the most to the beneficial effect of pre-treatment with immunomodulatory agents during machine perfusion, these preliminary results highlight the importance of continuous research toward modulation of biochemical pathways involved in cell injury to improve organ preservation.
Gene therapy strategies
Through the modification of gene expression by supplementation, silencing, or repair, gene therapy can treat, cure, or prevent conditions that would have been otherwise intractable (37, 38). Currently, the research on liver-directed gene therapies focuses on inherited metabolic liver diseases and bleeding disorders (38). However, little is known about the role of gene therapy for liver transplantation using machine perfusion. Increasing research interest have focused on gene silencing techniques with small RNA interference and the use of mesenchymal stem cells (MSCs) added to the perfusate during MP (39). In addition, novel strategies, including stem cell-derived extracellular vesicles, have shown promising results inspiring future research in the field (40).
The current methods employed for gene therapy include ex vivo administration, with cell modification outside the patient, or in vivo, via systemic or local administration using carrier vectors—generally non-viral or viral vectors (38). Hence, specific targeting to the desired organ is limited, jeopardizing the expected effects and exposing it to processes of clearance, filtration, and enzymatic breakdown in the serum and different organs (39, 41). To address this limitation, Chin et al. developed a model using engineered rat fibroblasts infused into the livers of rats while undergoing NMP to simulate in vivo conditions (41). Bioluminescence assays demonstrated the superiority of the continuous flow-based seeding process, by using NMP, to obtain adequate distribution of the engineered fibroblasts within the liver tissue when compared to a simple injection (41). In addition, the authors found no negative impact on graft survival and function after transplant when compared with control grafts. This innovative study laid the foundation for the implementation of MP as a feasible delivery method for targeted therapies. Another recent experimental study using HOPE as a delivery system demonstrated increased absorption of small interfering RNA compounds during perfusion (42). Unfortunately, no strong conclusions were drawn in terms of the benefit of the used compounds themselves (42). Laing et al. further validated the role of MP as a tool to provide direct and targeted organ delivery of cellular therapy in a human model of liver transplantation under normothermic perfusion conditions (43). The available experimental experiences provide proof-of-concept and indicate that merging MP with gene therapy is feasible. However, further studies are needed to determine if there is a true clinical benefit of combining these two complex modalities.
Similarly, the delivery of MSC via MP offers the possibility of taking advantage of its immunomodulatory and regenerative effects, without the burden of conventional MSC therapy associated risks. MSC therapy is associated with prothrombotic activity, leading to microvascular thrombosis and pulmonary embolism, as well as increased lung uptake/entrapment, reducing its delivery to other organ targets (44). Nevertheless, further studies to validate the benefits of MP for this particular use are needed.
Although novel in the liver transplant field, a combination of gene therapy and machine perfusion preservation has the potential to offer therapeutic options for graft reconditioning and rejection prevention. However, further research is needed to determine the true benefits of this novel modality. In addition, the evaluation of cost-effective benefits of these therapies is warranted as, currently, gene therapy alone can represent significant expenses, limiting patient access and increasing healthcare-associated expenses (45).
Pharmacotherapy for marginal grafts
Steatotic grafts
Steatotic grafts continue to increase among the donor pool and constitute one of the major reasons for graft discard. Therefore, the reduction of fat content in the graft before transplantation seems like a long-needed strategy. Nagrath et al. first proved this concept in 2009 (46). Liver grafts from hepatocellular steatotic-induced rats were perfused for 3 h using NMP. Perfusion was carried out with supplemented perfusate with a defatting cocktail composed of multiple agents with an individually known stimulatory effect on triglyceride secretion and/or β-oxidation (46). The authors found that lipid clearance was significantly higher in grafts perfused with the defatting cocktail when compared to the control perfusate. After 3 h of perfusion, the triglyceride content decreased by 65% in the study group, but only by 30% in the control group. Important histologic changes were also observed, particularly in the periportal region, including a reduction of lipid vesicles in hepatocytes, restored hepatocellular volume, and increased diameter in the sinusoid diameter (46). In addition, the expression of regulatory genes involved in lipid oxidation and secretion pathways was also increased. The authors suggested that their results imply a potential option to salvage moderate to severely steatotic grafts and, therefore, increase the donor pool (46). Similar results were later achieved using animal models and different agents including Glial cell line-derived neurotrophic factor and multidrug combinations, achieving good results in the reduction of lipid content and hepatocellular damage (47, 48). Conflicting results using subnormothermic conditions have been reported (49).
The promising results evidenced during animal models were tested in human livers undergoing MP supplemented with defatting agents for the first time in 2019 (50). The group evaluated 10 discarded steatotic human liver grafts, including DCD, and grafts with different degrees of steatosis (ranging from non-to severe) perfused with a defatting cocktail and L-carnitine supplementation (50). In alignment with previous experimental reports (46), lipid enzyme upregulation was observed, along with the increased activity of lipid regulation metabolic pathways (50). Over a perfusion period of 6 h, triglycerides levels were reduced by 38% in the study group, compared with 7% in those undergoing NMP alone. In addition, the authors observed a time-dependent decrease of 40% in the macrosteatosis rate after 6 h and of 50% after 12 h of perfusion in the treatment group, with no changes in the control group. Importantly, a statistically significant difference was found in terms of organ viability. While all the treated organs reached the viability criteria for transplantation, only two in the control group did (50). These available promising results, obtained by lipid metabolic reconditioning of steatotic grafts while undergoing machine perfusion, could reshape the way organ allocation and procurement are performed. Further studies are needed to determine the best defatting agent combination to use, as well as to better elucidate short- and long-term effects of this novel strategy in the clinical setting.
Hepatitis C-positive grafts
Since the approval of direct acting antiviral agents effective against hepatitis C virus (HCV), the acceptance of grafts from viremic donors for transplant into negative recipients have been less stringent (51). This allows more availability of grafts for transplantation for some recipients. However, the treatment regimen after LT with a HCV-positive graft is usually long—approximately 12 weeks—incurring high costs, ongoing surveillance, and possible drug side effects and drug-to-drug interactions (51). Therefore, the reduction or complete eradication of the graft viral load before transplantation would be an ideal scenario.
On the other hand, transplantation of HCV-negative grafts into a positive recipient results in immediate reinfection of the graft, with a more aggressive presentation and important rate of cirrhosis recurrence within 5 years (52). Therefore, pre-treatment of HCV-negative grafts before transplantation into a positive recipient is a promising preventive strategy. Goldaracena et al. first explored this concept in their experimental porcine model (52). The authors measured the impact of Miravirsen on HCV replication in grafts undergoing MP preservation. Miravirsen is an antiviral agent that targets microRNA-122, an important component in the replication of HCV (52). It requires active metabolism for an adequate uptake; therefore, the authors used NMP for its delivery into the graft. Following a series of analyses, it was found that NMP resulted in significantly higher absorption of the agent when compared to SCS, with higher concentrations found at 2 h and 72 h after LT (52). In addition, sequestration of microRNA-122 was significantly higher among the study group. Therefore, the authors suggested that their results indicate temporary gene silencing and, possibly, a reduction in HCV replication after LT with pre-treated grafts in NMP (52). This proof-of-concept supports the necessity of an active metabolism to successfully deliver a drug ex vivo and allow it to achieve its actions on a specific target organ, goals that were facilitated by the environment provided by NMP. Therefore, pharmacotherapy studies—not only for HCV but also for a plethora of conditions—should focus on the use of NMP, as hypothermic conditions assert the opposite effect on metabolism.
Much is still needed to explore pharmacotherapy during MP of marginal grafts in the clinical setting. However, favorable results achieved during animal models support the notion of combining dynamic flow preservation and existing pharmacological agents to improve the quality of marginal organs beyond the benefits of dynamic flow alone. In addition to potentially serving as a drug-delivery platform, pharmacotherapy using MP allows for the detailed pharmacokinetic and pharmacodynamic assessment in human livers (53). Research teams are encouraged to continue expanding the options that these technologies have to offer. Important areas to explore the role of MP include toxicology assessment, oncologic therapies, delivery of nanoparticles, and surgical training (32, 54). Scientific, technologic, and logistic advances make graft modification using MP a tangible treatment possibility in the near future.
Conclusion
Organ preservation using the MP system offers a vast array of possibilities for graft modification when combined with alternative therapies, including immunomodulation, gene therapy, and pharmacologic strategies. The available reports, mostly on experimental phases, are promising. However, further research on clinical settings is needed to better elucidate the true effect of graft modification pre-transplant on short- and long-term grafts and patient survival. To date, there are not enough data to support one MP device over the other for graft modification. However, the selection of hypothermic, normothermic, or subnormothermic conditions should be goal-driven based on the adjuvant therapy to be used. There is a long road ahead to develop guidelines and approve these novel therapies. However, the path looks promising.
Data availability statement
The original contributions presented in the study are included in the article/Supplementary Material, further inquiries can be directed to the corresponding author.
Author contributions
NG and PV participated in the conception and design of the manuscript, literature review, writing of the paper, and drafting of the manuscript. CY participated in the literature review and additional content of the manuscript. All authors contributed to the article and approved the submitted version.
Conflict of interest
The authors declare that the research was conducted in the absence of any commercial or financial relationships that could be construed as a potential conflict of interest.
Publisher's note
All claims expressed in this article are solely those of the authors and do not necessarily represent those of their affiliated organizations, or those of the publisher, the editors and the reviewers. Any product that may be evaluated in this article, or claim that may be made by its manufacturer, is not guaranteed or endorsed by the publisher.
Supplementary material
The Supplementary Material for this article can be found online at: https://www.frontiersin.org/articles/10.3389/frtra.2023.1163539/full#supplementary-material.
References
1. Belzer FO, Ashby BS, Gulyassy PF, Powell M. Successful seventeen-hour preservation and transplantation of human-cadaver kidney. N Engl J Med. (1968) 278(11):608–10. doi: 10.1056/NEJM196803142781108
2. Belzer FO, Ashby BS, Dunphy JE. 24-hour and 72-hour preservation of canine kidneys. Lancet. (1967) 2(7515):536–8. doi: 10.1016/s0140-6736(67)90498-9
3. Peng P, Ding Z, He Y, Zhang J, Wang X, Yang Z. Hypothermic machine perfusion versus static cold storage in deceased donor kidney transplantation: a systematic review and meta-analysis of randomized controlled trials. Artif Organs. (2019) 43(5):478–89. doi: 10.1111/aor.13364
4. Bellini MI, Nozdrin M, Yiu J, Papalois V. Machine perfusion for abdominal organ preservation: a systematic review of kidney and liver human grafts. J Clin Med. (2019) 8(8):1221. doi: 10.3390/jcm8081221
5. Guarrera JV, Henry SD, Samstein B, Odeh-Ramadan R, Kinkhabwala M, Goldstein MJ, et al. Hypothermic machine preservation in human liver transplantation: the first clinical series. Am J Transplant. (2010) 10(2):372–81. doi: 10.1111/j.1600-6143.2009.02932.x
6. Horváth T, Jász DK, Baráth B, Poles MZ, Boros M, Hartmann P. Mitochondrial consequences of organ preservation techniques during liver transplantation. Int J Mol Sci. (2021) 22(6):2816. doi: 10.3390/ijms22062816
7. Jakubauskas M, Jakubauskiene L, Leber B, Strupas K, Stiegler P, Schemmer P. Machine perfusion in liver transplantation: a systematic review and meta-analysis. Visc Med. (2022) 38(4):243–54. doi: 10.1159/000519788
8. Abraham N, Zhang M, Cray P, Gao Q, Samy KP, Neill R, et al. Two compartment evaluation of liver grafts during acellular room temperature machine perfusion (acRTMP) in a rat liver transplant model. Front Med (Lausanne). (2022) 9:804834. doi: 10.3389/fmed.2022.804834
9. Czigany Z, Pratschke J, Froněk J, Guba M, Schöning W, Raptis DA, et al. Hypothermic oxygenated machine perfusion reduces early allograft injury and improves post-transplant outcomes in extended criteria donation liver transplantation from donation after brain death: results from a multicenter randomized controlled trial (HOPE ECD-DBD). Ann Surg. (2021) 274(5):705–12. doi: 10.1097/SLA.0000000000005110
10. Henry SD, Nachber E, Tulipan J, Stone J, Bae C, Reznik L, et al. Hypothermic machine preservation reduces molecular markers of ischemia/reperfusion injury in human liver transplantation. Am J Transplant. (2012) 12(9):2477–86. doi: 10.1111/j.1600-6143.2012.04086.x
11. Guarrera JV, Henry SD, Samstein B, Reznik E, Musat C, Lukose TI, et al. Hypothermic machine preservation facilitates successful transplantation of “orphan” extended criteria donor livers. Am J Transplant. (2015) 15(1):161–9. doi: 10.1111/ajt.12958
12. Dutkowski P, Polak WG, Muiesan P, Schlegel A, Verhoeven CJ, Scalera I, et al. First comparison of hypothermic oxygenated perfusion versus static cold storage of human donation after cardiac death liver transplants: an international-matched case analysis. Ann Surg. (2015) 262(5):764–70, discussion 770–1. doi: 10.1097/SLA.0000000000001473
13. van Rijn R, Karimian N, Matton APM, Burlage LC, Westerkamp AC, van den Berg AP, et al. Dual hypothermic oxygenated machine perfusion in liver transplants donated after circulatory death. Br J Surg. (2017) 104(7):907–17. doi: 10.1002/bjs.10515
14. van Rijn R, van Leeuwen OB, Matton APM, Burlage LC, Wiersema-Buist J, van den Heuvel MC, et al. Hypothermic oxygenated machine perfusion reduces bile duct reperfusion injury after transplantation of donation after circulatory death livers. Liver Transpl. (2018) 24(5):655–64. doi: 10.1002/lt.25023
15. Schlegel A, Muller X, Kalisvaart M, Muellhaupt B, Perera MTPR, Isaac JR, et al. Outcomes of DCD liver transplantation using organs treated by hypothermic oxygenated perfusion before implantation. J Hepatol. (2019) 70(1):50–7. doi: 10.1016/j.jhep.2018.10.005
16. Burlage LC, Hessels L, van Rijn R, Matton APM, Fujiyoshi M, van den Berg AP, et al. Opposite acute potassium and sodium shifts during transplantation of hypothermic machine perfused donor livers. Am J Transplant. (2019) 19(4):1061–71. doi: 10.1111/ajt.15173
17. van Leeuwen OB, de Vries Y, Fujiyoshi M, Nijsten MWN, Ubbink R, Pelgrim GJ, et al. Transplantation of high-risk donor livers after ex situ resuscitation and assessment using combined hypo- and normothermic machine perfusion: a prospective clinical trial. Ann Surg. (2019) 270(5):906–14. doi: 10.1097/SLA.0000000000003540
18. Brüggenwirth IMA, Lantinga VA, Rayar M, van den Berg AP, Blokzijl H, Reyntjens KMEM, et al. Prolonged dual hypothermic oxygenated machine preservation (DHOPE-PRO) in liver transplantation: study protocol for a stage 2, prospective, dual-arm, safety and feasibility clinical trial. BMJ Open Gastroenterol. (2022) 9(1):e000842. doi: 10.1136/bmjgast-2021-000842
19. Patrono D, Cussa D, Sciannameo V, Montanari E, Panconesi R, Berchialla P, et al. Outcome of liver transplantation with grafts from brain-dead donors treated with dual hypothermic oxygenated machine perfusion, with particular reference to elderly donors. Am J Transplant. (2022) 22(5):1382–95. doi: 10.1111/ajt.16996
20. Patrono D, Zanierato M, Vergano M, Magaton C, Diale E, Rizza G, et al. Normothermic regional perfusion and hypothermic oxygenated machine perfusion for livers donated after controlled circulatory death with prolonged warm ischemia time: a matched comparison with livers from brain-dead donors. Transpl Int. (2022) 35:10390. doi: 10.3389/ti.2022.10390
21. Hirao H, Nakamura K, Kupiec-Weglinski JW. Liver ischaemia-reperfusion injury: a new understanding of the role of innate immunity. Nat Rev Gastroenterol Hepatol. (2022) 19(4):239–56. doi: 10.1038/s41575-021-00549-8
22. Rampes S, Ma D. Hepatic ischemia-reperfusion injury in liver transplant setting: mechanisms and protective strategies. J Biomed Res. (2019) 33(4):221–34. doi: 10.7555/JBR.32.20180087
23. Xu H, Lee CY, Clemens MG, Zhang JX. Inhibition of TXA synthesis with OKY-046 improves liver preservation by prolonged hypothermic machine perfusion in rats. J Gastroenterol Hepatol. (2008) 23(7 Pt 2):e212–20. doi: 10.1111/j.1440-1746.2007.05061.x
24. Micó-Carnero M, Zaouali MA, Rojano-Alfonso C, Maroto-Serrat C, Ben Abdennebi H, Peralta C. A potential route to reduce ischemia/reperfusion injury in organ preservation. Cells. (2022) 11(17):2763. doi: 10.3390/cells11172763
25. Jing L, Yao L, Zhao M, Peng LP, Liu M. Organ preservation: from the past to the future. Acta Pharmacol Sin. (2018) 39(5):845–57. doi: 10.1038/aps.2017.182
26. Schlegel A, Muller X, Dutkowski P. Machine perfusion strategies in liver transplantation. Hepatobiliary Surg Nutr. (2019) 8(5):490–501. doi: 10.21037/hbsn.2019.04.04
27. Knaak JM, Spetzler VN, Goldaracena N, Louis KS, Selzner N, Selzner M. Technique of subnormothermic ex vivo liver perfusion for the storage, assessment, and repair of marginal liver grafts. J Vis Exp. (2014) 90:e51419. doi: 10.3791/51419
28. Clavien PA, Dutkowski P, Mueller M, Eshmuminov D, Bautista Borrego L, Weber A, et al. Transplantation of a human liver following 3 days of ex situ normothermic preservation. Nat Biotechnol. (2022) 40(11):1610–6. doi: 10.1038/s41587-022-01354-7
29. Kwong AJ, Ebel NH, Kim WR, Lake JR, Smith JM, Schladt DP, et al. OPTN/SRTR 2020 annual data report: liver. Am J Transplant. (2022) 22(Suppl 2):204–309. doi: 10.1111/ajt.16978
30. Kalisvaart M, Croome KP, Hernandez-Alejandro R, Pirenne J, Cortés-Cerisuelo M, Miñambres E, et al. Donor warm ischemia time in DCD liver transplantation—working group report from the ILTS DCD, liver preservation, and machine perfusion consensus conference. Transplantation. (2021) 105(6):1156–64. doi: 10.1097/TP.0000000000003819
31. Lauterio A, Di Sandro S, De Carlis R, Ferla F, Pinotti E, De Carlis L. Every liver graft should be evaluated for transplantation. Transplantation. (2018) 102(10):e456–7. doi: 10.1097/TP.0000000000002364
32. Carlson K, Barbas A, Goldaracena N, Fernandez L, Al-Adra DP. Immunological organ modification during ex vivo machine perfusion: the future of organ acceptance. Transplant Rev (Orlando). (2021) 35(2):100586. doi: 10.1016/j.trre.2020.100586
33. Goldaracena N, Echeverri J, Spetzler VN, Kaths JM, Barbas AS, Louis KS, et al. Anti-inflammatory signaling during ex vivo liver perfusion improves the preservation of pig liver grafts before transplantation. Liver Transpl. (2016) 22(11):1573–83. doi: 10.1002/lt.24603
34. Echeverri J, Goldaracena N, Kaths JM, Linares I, Roizales R, Kollmann D, et al. Comparison of BQ123, epoprostenol, and verapamil as vasodilators during normothermic ex vivo liver machine perfusion. Transplantation. (2018) 102(4):601–8. doi: 10.1097/TP.0000000000002021
35. Yu Y, Cheng Y, Pan Q, Zhang YJ, Jia DG, Liu YF. Effect of the selective NLRP3 inflammasome inhibitor mcc950 on transplantation outcome in a pig liver transplantation model with organs from donors after circulatory death preserved by hypothermic machine perfusion. Transplantation. (2019) 103(2):353–62. doi: 10.1097/TP.0000000000002461
36. Schlegel A, Muller X, Mueller M, Stepanova A, Kron P, de Rougemont O, et al. Hypothermic oxygenated perfusion protects from mitochondrial injury before liver transplantation. EBioMedicine. (2020) 60:103014. doi: 10.1016/j.ebiom.2020.103014
37. Brianna , Ling APK, Wong YP. Applying stem cell therapy in intractable diseases: a narrative review of decades of progress and challenges. Stem Cell Investig. (2022) 9:4. doi: 10.21037/sci-2022-021
38. Maestro S, Weber ND, Zabaleta N, Aldabe R, Gonzalez-Aseguinolaza G. Novel vectors and approaches for gene therapy in liver diseases. JHEP Rep. (2021) 3(4):100300. doi: 10.1016/j.jhepr.2021.100300
39. Pavan-Guimaraes J, Martins PN. Modifying organs with gene therapy and gene modulation in the age of machine perfusion. Curr Opin Organ Transplant. (2022) 27(5 ):474–80. doi: 10.1097/MOT.0000000000001007
40. Rigo F, De Stefano N, Navarro-Tableros V, David E, Rizza G, Catalano G, et al. Extracellular vesicles from human liver stem cells reduce injury in an ex vivo normothermic hypoxic rat liver perfusion model. Transplantation. (2018) 102(5 ):e205–10. doi: 10.1097/TP.0000000000002123
41. Chin LY, Carroll C, Raigani S, Detelich DM, Tessier SN, Wojtkiewicz GR, et al. Ex vivo perfusion-based engraftment of genetically engineered cell sensors into transplantable organs. PLoS One. (2019) 14(12):e0225222. doi: 10.1371/journal.pone.0225222
42. Bonaccorsi-Riani E, Gillooly AR, Iesari S, Brüggenwirth IMA, Ferguson CM, Komuta M, et al. Delivering siRNA compounds during HOPE to modulate organ function: a proof-of-concept study in a rat liver transplant model. Transplantation. (2022) 106(8):1565–76. doi: 10.1097/TP.0000000000004175
43. Laing RW, Stubblefield S, Wallace L, Roobrouck VD, Bhogal RH, Schlegel A, et al. The delivery of multipotent adult progenitor cells to extended criteria human donor livers using normothermic machine perfusion. Front Immunol. (2020) 11:1226. doi: 10.3389/fimmu.2020.01226
44. Li J, Peng Q, Yang R, Li K, Zhu P, Zhu Y, et al. Application of mesenchymal stem cells during machine perfusion: an emerging novel strategy for organ preservation. Front Immunol. (2021) 12:713920. doi: 10.3389/fimmu.2021.713920
45. Salzman R, Cook F, Hunt T, Malech HL, Reilly P, Foss-Campbell B, et al. Addressing the value of gene therapy and enhancing patient access to transformative treatments. Mol Ther. (2018) 26(12):2717–26. doi: 10.1016/j.ymthe.2018.10.017
46. Nagrath D, Xu H, Tanimura Y, Zuo R, Berthiaume F, Avila M, et al. Metabolic preconditioning of donor organs: defatting fatty livers by normothermic perfusion ex vivo. Metab Eng. (2009) 11(4-5):274–83. doi: 10.1016/j.ymben.2009.05.005
47. Taba Taba Vakili S, Kailar R, Rahman K, Nezami BG, Mwangi SM, Anania FA, et al. Glial cell line-derived neurotrophic factor-induced mice liver defatting: a novel strategy to enable transplantation of steatotic livers. Liver Transpl. (2016) 22(4):459–67. doi: 10.1002/lt.24385
48. Xu M, Zhou F, Ahmed O, Upadhya GA, Jia J, Lee C, et al. A novel multidrug combination mitigates rat liver steatosis through activating AMPK pathway during normothermic machine perfusion. Transplantation. (2021) 105(11):e215–25. doi: 10.1097/TP.0000000000003675
49. Liu Q, Berendsen T, Izamis ML, Uygun B, Yarmush ML, Uygun K. Perfusion defatting at subnormothermic temperatures in steatotic rat livers. Transplant Proc. (2013) 45(9):3209–13. doi: 10.1016/j.transproceed.2013.05.005
50. Boteon YL, Attard J, Boteon APCS, Wallace L, Reynolds G, Hubscher S, et al. Manipulation of lipid metabolism during normothermic machine perfusion: effect of defatting therapies on donor liver functional recovery. Liver Transpl. (2019) 25(7):1007–22. doi: 10.1002/lt.25439
51. Snyder HS, Wiegel JJ, Khalil K, Summers BB, Tan T, Jonchhe S, et al. A systematic review of direct acting antiviral therapies in hepatitis C virus-negative liver transplant recipients of hepatitis C-viremic donors. Pharmacotherapy. (2022) 42(12):905–20. doi: 10.1002/phar.2742
52. Goldaracena N, Spetzler VN, Echeverri J, Kaths JM, Cherepanov V, Persson R, et al. Inducing hepatitis C virus resistance after pig liver transplantation—a proof of concept of liver graft modification using warm ex vivo perfusion. Am J Transplant. (2017) 17(4):970–8. doi: 10.1111/ajt.14100
53. Tingle SJ, Thompson ER, Bates L, Ibrahim IK, Govaere O, Shuttleworth V, et al. Pharmacological testing of therapeutics using normothermic machine perfusion: a pilot study of 2,4-dinitrophenol delivery to steatotic human livers. Artif Organs. (2022) 46(11):2201–14. doi: 10.1111/aor.14309
Keywords: machine perfusion, ex vivo, liver transplantation, marginal organs, donor pool
Citation: Vargas PA, Yu C and Goldaracena N (2023) Comprehensive review of the application of MP and the potential for graft modification. Front. Transplant. 2:1163539. doi: 10.3389/frtra.2023.1163539
Received: 10 February 2023; Accepted: 20 April 2023;
Published: 16 May 2023.
Edited by:
Constanca Figueiredo, Hannover Medical School, GermanyReviewed by:
Reinhard Schwinzer, Hannover Medical School, GermanyMichelle McCarthy, Shriners Hospitals for Children Burns, United States
© 2023 Vargas, Yu and Goldaracena. This is an open-access article distributed under the terms of the Creative Commons Attribution License (CC BY). The use, distribution or reproduction in other forums is permitted, provided the original author(s) and the copyright owner(s) are credited and that the original publication in this journal is cited, in accordance with accepted academic practice. No use, distribution or reproduction is permitted which does not comply with these terms.
*Correspondence: Nicolas Goldaracena n.goldaracena@virginia.edu