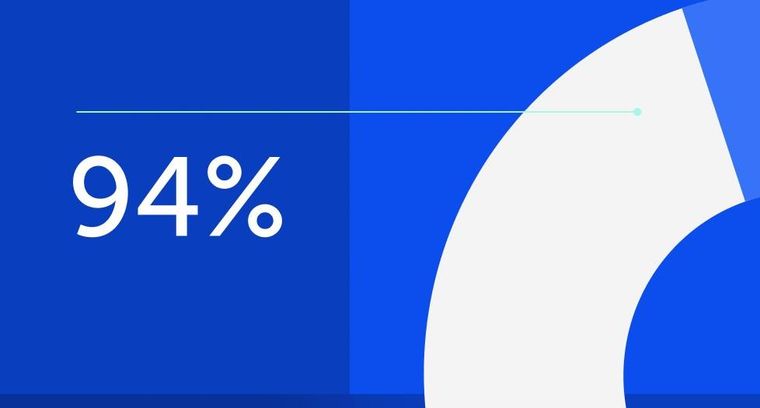
94% of researchers rate our articles as excellent or good
Learn more about the work of our research integrity team to safeguard the quality of each article we publish.
Find out more
MINI REVIEW article
Front. Toxicol., 15 April 2025
Sec. Environmental Toxicology
Volume 7 - 2025 | https://doi.org/10.3389/ftox.2025.1567225
This article is part of the Research TopicThe use of alternative approaches in environmental toxicology research - moving towards the 3Rs principle in animal experimentationView all articles
Micro- and nanoplastics (MNPs) are widespread environmental pollutants that pose significant health risks. They originate from industrial processes, consumer products, and environmental degradation, inducing oxidative stress through cellular dysfunctions such as membrane interaction, internalization, mitochondrial damage, inflammation, metal ion leaching, and impaired antioxidant defense. Despite increasing evidence of their toxicity—particularly developmental neurotoxicity (DNT) and mitochondrial impairment—our understanding remains limited due to the high costs of animal studies, which reduce the overall size of experimental data. This underscores the urgent need for alternative test methods that are cost-effective, rapid, and translational. This review examines new approach methodologies (NAMs) for DNT assessment, addressing the ethical, financial, and translational limitations of animal models. NAMs integrate three complementary non-animal models that enhance conventional testing. First, zebrafish models provide organismal insights into behavioral and neurodevelopmental outcomes at minimal cost. Second, neuronal organoids replicate human-specific neurodevelopmental processes in a 3D system, offering mechanistic insights. Lastly, human cell lines enable high-throughput screening, integrating findings from zebrafish and organoid studies. Establishing a new paradigm for DNT testing is crucial for faster and more efficient toxicity and risk assessments, ultimately protecting public health. Standardizing and gaining regulatory acceptance for NAMs will improve predictive accuracy and broaden their application in environmental toxicology. Advancing these methodologies is essential to addressing the risks of MNP exposure while promoting ethical and sustainable research practices.
Micro- and nanoplastics (MNPs) have emerged as a global public health concern. These small plastic particles, ranging from microplastics (MPs, ≤5 mm) to nanoplastics (NPs, ≤1 µm), are predominantly derived from petroleum-based polymers. They originate from various sources, including the degradation of larger plastic debris, industrial emissions, and consumer products such as cosmetics and textiles (Barboza et al., 2018). MNPs infiltrate aquatic and terrestrial ecosystems, exposing human tissues and organs through ingestion, inhalation, and dermal contact (Horvatits et al., 2022; Hong et al., 2023; Campen et al., 2024; Saha and Saha, 2024; Wang et al., 2024). Their unique physicochemical properties enable them to leach into the environment, carry toxicants on their surfaces, cross biological membrane barriers, and disrupt cellular processes (Li et al., 2024). These findings highlight MNPs contamination as a growing health risk and underscores the urgent need to study MNPs health effects to aid in risk assessment and management.
Recent studies indicate MNPs can impair metabolism, immune function, and neuronal systems while exerting direct toxic effects on mitochondria, redox mechanisms, and signaling pathways (Prust et al., 2020; Yee et al., 2021; Yang et al., 2022; Ali et al., 2024). It was also noted that early-life exposure during critical brain development stages has been linked to neurotoxic effects, including neurotransmitter dysregulation, potentially leading to long-term cognitive and behavioral deficits (Prust et al., 2020).
Rodent models have been the standard for DNT testing but face ethical, logistical, and translational challenges, prompting efforts to reduce animal use (Bal-Price et al., 2010). Additionally, rodent studies are costly and labor intensive, limiting their scalability for testing emerging toxicants like MNPs (Du et al., 2024). Furthermore, physiological and developmental differences between rodents and humans often make it difficult to translate the results to human health (Krewski et al., 2010).
To overcome these limitations, alternative models that are high throughput, scalable and better adapted to human biology, including metabolic processes, are needed. Advances in NAMs provide ethical and predictive tools for DNT testing. NAMs provide critical insights into the developmental neurotoxicity of MNPs without relying on in vivo models and enable more sustainable and human-relevant research practices.
This review highlights how NAMs can transform current practices and promote a shift towards sustainable and accurate methods for assessing MNP-related global health risks.
Mitochondria is vital organelles involved in energy metabolism, ATP production, fatty acid oxidation, calcium homeostasis, macromolecule biosynthesis, and cellular signaling pathways (Casanova et al., 2023; Popov, 2023). Mitochondria are susceptible to damage from chemicals, nanomaterials, and environmental pollutants resulting in dysfunction (Lee et al., 2022; Yao et al., 2024). Mechanisms of mitochondrial toxicity include reduced membrane potential, impaired enzymatic activity, disrupted ATP production, calcium dysregulation, and pro-apoptotic factor release (Tait and Green, 2012; Zong et al., 2024). Exposure to MNPs has been linked to mitochondrial dysfunction, such as impaired membrane potential, reduced respiratory complex activity, disrupted ATP production, increased reactive oxygen species (ROS), and oxidative stress, leading to inflammation, mitophagy, and apoptosis (Hu and Palic, 2020; Das, 2023). These effects contribute to various health issues, including metabolic and developmental disorders (Ali et al., 2024; Jeong et al., 2024). However, in vivo evidence of mitochondrial accumulation of MNPs remains limited (Silva et al., 2023), and further research is necessary to elucidate size- and type-dependent MNP-induced mitochondrial toxicity and its implications for developmental neurotoxicity.
MPs commonly found in our environment are primarily composed of polyethylene, polystyrene, polyvinyl chloride, polypropylene, among others (Gan et al., 2023). In rodent models, polyethylene terephthalate MPs reduced mitochondrial membrane potential, increased Bax protein expression, and decreased PARP, caspase-3, and Bcl-2 expression in H9C2 cells (Lu et al., 2024). MNPs exposure elevated ROS levels, leading to mitochondrial damage, reduced antioxidant enzyme activity (SOD, CAT, GSH-Px), disrupted redox balance, and cardiomyocyte apoptosis (Lu et al., 2024). Studies in zebrafish showed that chronic exposure to PP MNPs caused oxidative stress in the brain and liver, reducing mitochondrial respiratory efficiency and depolarizing mitochondrial membranes (Felix et al., 2023; Liu et al., 2024). These disruptions impaired respiratory complexes (e.g., Complex II, IV), increased ROS production, and triggered inflammatory and apoptotic pathways (Felix et al., 2023). In SH-SY5Y human cell lines, MNP exposure similarly caused increased ROS, mitochondrial damage, and apoptosis, disrupting cellular energy metabolism (Prust et al., 2020).
Despite progress, a standardized classification system for MPs based on size, shape, composition, and charge is unavailable. While organ-level MNP effects are studied, research on their developmental toxicity, especially DNT, is limited, highlighting the need for advanced models.
Zebrafish’s transparency, external fertilization, and rapid development make it ideal for high-throughput, real-time toxicology studies. With over 70% genetic homology to humans, including conserved neural pathways, they have played a critical role for neurotoxicity research (Kalueff et al., 2014). Historically used in environmental toxicology, the use of zebrafish is favored in DNT studies. Their small size, high fecundity, and low cost makes them ideal for fast turnover and scale-up chemical screening, such as MNPs (Planchart et al., 2016). Zebrafish thus emerge as an ethical, efficient, and human-relevant model in advancing toxicological research.
Zebrafish have recently emerged as a valuable model for assessing the developmental effects of MNPs. Research indicates that MNP exposure disrupts early neurodevelopment by inducing oxidative stress (Felix et al., 2023; Liu et al., 2024), apoptosis (Liu et al., 2024), and altering neurotransmitter balance (Prust et al., 2020). MNPs can also induce behavioral changes, such as altered locomotion, serve as sensitive indicators of neurotoxicity (Malhotra et al., 2019; Wu et al., 2024). For example, environmentally relevant MNP concentrations in zebrafish embryos disrupted motor activity and reduced neuronal connectivity, highlighting their potential to impair central nervous system (CNS) development (Barboza et al., 2018). Biomarkers like oxidative stress and apoptotic signaling further clarify the pathways leading to MNP toxicity.
Zebrafish model is also used to explore the size- and type-dependent effects of MNPs. Exposure to polystyrene nanoplastics (20 nm, 0.3–9 mg/L) caused morphological deformities, reduced body length, lower survival and hatching rates, impaired locomotion, and inhibited motor neuron development. These effects were associated with an increase in ROS, decreased superoxide dismutase (SOD) activity, and upregulation of genes related to apoptosis (e.g., bcl2a and caspase-3a). Additionally, nanoplastics altered the expression of neurodevelopmental genes (gli2a, neurog1, syn2a), underscoring ROS-induced apoptosis as a critical mechanism (Cao et al., 2024).
MNPs, particularly MPs, can increase their toxicity potential through adsorption by pollutants such as PCBs, PAHs, pesticides, and heavy metals). These contaminants adhere to MPs' large surface area and their hydrophobic character induces higher concentration in aquatic organisms (Gupta et al., 2024). For example, during zebrafish embryogenesis, polystyrene microplastics (PS-MPs) and perfluorooctane sulfonic acid (PFOS) exhibit synergistic toxicity. PS-MPs adsorb PFOS, cummulating oxidative stress, disrupting antioxidant enzyme activity, and impairing neurotransmission through reduced acetylcholinesterase (ACHE) activity and elevated acetylcholine (ACh) levels. Co-exposure also causes morphological defects, such as pericardial edema and altered yolk sac area, emphasizing the amplified metabolic disruptions initiated by contaminant adsorption (Gupta et al., 2024).
Zebrafish models offer advantages for DNT testing but face challenges, including replicating chronic low-dose exposures and quantifying the extent of MNP bioaccumulation in embryos (Lei et al., 2023). More research is needed on the multigenerational effects of MNPs on neurodevelopment and behavior. Standardized protocols could improve reproducibility and regulatory acceptance, solidifying zebrafish as a key model in neurotoxicity testing. In addition, the use of zebrafish embryos for developmental toxicity assays was well established. It allows multiple endpoints evaluation related to lethal or sub-lethal effects along with developmental defects (Ducharme et al., 2013; Jin et al., 2013; Shen et al., 2020; Elersek et al., 2021). Zebrafish embryos also assess MNP toxicity through lethality, inflammation, oxidative stress, apoptosis, neurodevelopment, and behavior assays, highlighting environmental and health risks.
A neural organoid is a 3D model that captures key aspects of brain development. This biological model includes an appropriate cellular diversity, structural organization, and maturation that make it a valid tool for studying the neurodevelopment and neurotoxicity (Lancaster and Knoblich, 2014). 3D neural organoids are derived from pluripotent stem cells (PSCs) and have transformed the study of DNT (Wu et al., 2025). Comparing to conventional 2D cell cultures, neural organoids mimic human brain development by providing a 3D structure that supports complex cellular interactions and regional organization of the brain. This unique capability makes organoids suitable for investigating brain region-specific effects of environmental toxicants, such as MNPs (Lancaster and Knoblich, 2014).
The emergence of neural organoids as research tools has filled critical gaps in translational studies by replicating essential neurodevelopmental processes, including neurogenesis, synaptogenesis, and circuit formation. Neural organoids provide human-specific insights, overcoming rodent models' limitations in replicating human neural development. This linking of in vitro assays and in vivo systems enhances our ability to investigate the developmental impacts of toxicants like MNPs, providing a more human relevant and ethical approach to studying human DNT (Quadrato et al., 2016).
Recent advances have underscored the utility of neural organoids in assessing the neurotoxicity of MNPs including neuroinflammation, disruption of neurogenesis, and alteration of neural connectivity. RNA sequencing has identified molecular signaling pathways impacted by MNPs exposure, including oxidative stress and inflammatory signaling (Chen et al., 2023). For example, Tao et al. (2024) demonstrated that polystyrene nanoparticle exposure reduced neural progenitor cell proliferation and disrupted cell differentiation in neural organoids (Tao et al., 2024). Advanced techniques including calcium imaging and electrophysiological recordings have further highlighted functional deficits in organoids, revealing the developmental risks posed by MNPs during critical periods of brain growth (Yan et al., 2024).
Neural organoids provide a human-relevant model for studying MNP-induced mitochondrial dysfunction in developmental neurotoxicity. MNPs cause dose-dependent mitochondrial damage in brain organoids, with low doses causing mitochondrial swelling, cristae disruption, and vacuole formation and high doses resulted in severe mitochondrial vacuolation, membrane disappearance, and structural breakdown (Chen et al., 2023). These mitochondrial impairments were accompanied by broader cellular dysfunction, including membrane breaches and intracellular lipid droplet formation, highlighting mitochondria as the central target of MNP-induced developmental neurotoxicity (Chen et al., 2023).
Organoids also enable brain region-specific developmental toxicity studies. PSC-derived cortical organoids exposed to PS-MPs (1 μm, endocytosed; 10 μm, affecting adhesion) at 5–100 μg/mL showed increased ROS, oxidative stress, and redox imbalance. Short-term exposure upregulated SOD2, while long-term exposure depleted antioxidants. Mitochondrial dysfunction impaired cortical differentiation, cell viability, and neural marker expression (Hua et al., 2022). These effects highlight oxidative stress, mitochondrial impairment, and depletion of the antioxidant response as central mechanisms of MNPs-induced neurotoxicity and effects on brain region development.
These studies confirm the relevance of neural organoids in understanding MNPs-induced neurotoxicity and promote sustainable, human-relevant research methods for assessing neural developmental risks.
Neural organoids hold great promise but face challenges due to a lack of vascularization and immune components compared to in vivo conditions (Quadrato et al., 2016). Recent advances such as vascularizing neural organoids via co-culture with vascular cells, vascular organoid integration, and stem cell co-differentiation, are addressing the issue (Nwokoye and Abilez, 2024). Advancements in Organ-on-a-chip now integrate perfusable vasculature into organoids, enhancing physiological modeling in vitro (Li et al., 2024; Zhang et al., 2024). These approaches aim to overcome the constraints on tissue size and achieve in vivo-like functionality by providing essential nutrients and oxygen to the neural organoid core (LaMontagne et al., 2022).
Human cell lines are important tools in DNT testing and they offer ethical and reproducible alternatives to animal models. Neuronal lines such as SH-SY5Y and IMR-32, derived from human neuroblastoma, are commonly used to study neuronal differentiation, signaling pathways, and DNT toxicant responses (Xicoy et al., 2017). Complementary non-neural lines, such as Caco-2, derived from intestinal epithelial cells, help to study the uptake and systemic distribution of toxins. These cell lines are invaluable in their simplicity, reproducibility, and mechanistic insights. They facilitate the research on key processes in toxicant-induced developmental neurotoxicity, including oxidative stress, apoptosis, and mitochondrial dysfunction.
Research on MNPs has consistently shown cytotoxicity, oxidative stress, mitochondrial dysfunction, and inflammation in human cell lines. In SH-SY5Y cells, MNP exposure induces oxidative stress, ROS accumulation, and structural damage of mitochondria and dysfunction, which disrupt energy metabolism and induce apoptosis (Prust et al., 2020). Dose-response studies show that smaller NPs exhibit greater cytotoxicity due to their increased surface area and cellular uptake (Ruan et al., 2023; Mahmud et al., 2024). In addition, MNPs exposure upregulates inflammatory cytokines such as IL-6 and TNF-α, highlighting their potential to induce inflammation (Donisi et al., 2024; Martin-Folgar et al., 2024). All these findings underscore the importance of human cell lines in evaluating MNPs DNT toxicity. The comparative summary is shown in the Table 1.
Human cell lines lack the complexity and 3D structure of tissues, so they cannot replicate the cellular interactions in the body. Important systemic interactions, such as those between cells, immune responses, or the dynamics of the blood-brain barrier, are critical for understanding the overall effects of toxicants but cannot be modeled effectively with cell lines alone. These limitations highlight the need for incorporating cell lines into more complex models, such as organoids or in silico simulations. Co-culture systems and microfluidic “organ-on-a-chip” technologies offer complementary solutions, creating more physiologically relevant environments to bridge these gaps.
A tiered, integrated testing battery combining human cells, neural organoids, and zebrafish models can improve the efficiency and relevance of DNT studies while reducing the use of animals. In this approach, human cell lines such as SH-SY5Y serve as an initial screening platform for the identification of potential neurotoxicants and provide scalable and cost-effective assays for the detection of early molecular signaling. Next, neural organoids enable deeper investigation of region-specific neurodevelopmental toxicity in the 3D human in vitro model, enabling more comprehensive dose-response analysis and pathway discovery. Finally, zebrafish provide an organismal perspective by assessing systemic and behavioral effects, including locomotion and memory, capturing complex outcomes and providing information on long-term effects. This stepwise strategy is consistent with the 3Rs—replacement, reduction, and refinement—by focusing animal-based approaches on the final confirmation stages. Regulatory acceptance of NAMs depends on robust validation, standardization, and ongoing collaboration, as demonstrated by programs such as US Tox21 and the EU Joint Research Center. These efforts lead to shared performance criteria, foster reproducibility, and support the incorporation of omics technologies and machine learning, which refine biomarker detection and improve predictive power. By addressing technical variability and promoting confidence in NAMs, such collaborative efforts accelerate their formalization, ensuring broader acceptance in the regulatory framework.
NAMs are revolutionizing DNT testing by offering human-relevant, scalable, and high-throughput solutions. These methodologies have shown significant potential to reduce reliance on traditional rodent animal models while providing valuable mechanistic insights into the developmental neurotoxic effects of environmental contaminants, including MNPs (Krewski et al., 2010).
The development of in vitro cell-based assays and the integration of new approach methodologies for developmental neurotoxicity screening and risk assessments can be traced back to 2008, when the Tox21 Consortium was established among OECD countries and federal agencies in the U.S. (Tice et al., 2013; OECD, 2023). Recent advances have highlighted the different strengths of zebrafish, neural organoids, and human cell lines in DNT research. Zebrafish models provide cost-effective, organismal insights into the behavior and neurodevelopmental outcomes (Bailey et al., 2013). Neural organoids bridge the gap between in vitro 2D cell models and in vivo testing systems, enabling the study of complex cellular interactions and region-specific neurodevelopment (Lancaster and Knoblich, 2014). Human cell lines are efficient for high-throughput screening and mechanistic studies, revealing cellular responses like oxidative stress, inflammation, and apoptosis (Xicoy et al., 2017).
Collectively, these NAMs establish a robust and adaptable framework to address the challenges of DNT testing in an evolving regulatory landscape, enhancing our understanding of neurotoxicity while reducing animal use. Ongoing efforts to integrate available NAMs into toxicity assessments—led by multiple federal agencies, including the U.S. EPA, NCATS, NIEHS, and FDA—are expected to further accelerate the identification of optimal assays for future toxicology studies.
NAMs still face challenges in DNT testing that necessitate standardization and validation for acceptable reproducibility and for gaining regulatory acceptance. OECD zebrafish guidelines and neural organoid harmonization are efforts in this direction, but more steps need to be taken before NAMs can be embraced by the scientific community as reliable alternative models (Sobanska et al., 2018). Recent studies emphasize acute and high doses MNPs exposure, leaving a gap in understanding MNPs' cumulative effects on neural development. Future research should also focus on long-term, low-dose MNPs exposure, mimicking human relevant inhalation or dietary intake level (Prust et al., 2020). Meanwhile, integrating omics and machine learning with NAMs increases predictability and helps identify novel DNT biomarkers. (Zhou et al., 2024). In addition, fostering collaboration between researchers working on standardized MNPs in research, industry stakeholders and regulators is critical to accelerating the use of NAMs. By addressing these challenges and taking advantage of these opportunities, we can advance DNT testing using NAMs, contributing to more ethical, human-relevant toxicology research.
XW: Writing – original draft, Writing – review and editing. TL: Writing – original draft, Writing – review and editing. DJ: Writing – review and editing, Writing – original draft. MI: Writing – review and editing, Data curation, Methodology. JB: Writing – original draft, Writing – review and editing, Conceptualization.
The author(s) declare that financial support was received for the research and/or publication of this article. This work was supported partly by NSF-EiR (award# 1832134), NIEHS/CHHE Pilot (P30ES025128), Department of Pharmacology and Toxicology at ECU and the College of Health and Sciences at NCCU.
The authors declare that the research was conducted in the absence of any commercial or financial relationships that could be construed as a potential conflict of interest.
The author(s) declare that Generative AI was used in the creation of this manuscript. Generative AI was used to prepare and summarize the scope statement.
All claims expressed in this article are solely those of the authors and do not necessarily represent those of their affiliated organizations, or those of the publisher, the editors and the reviewers. Any product that may be evaluated in this article, or claim that may be made by its manufacturer, is not guaranteed or endorsed by the publisher.
Ali, N., Katsouli, J., Marczylo, E. L., Gant, T. W., Wright, S., and Bernardino de la Serna, J. (2024). The potential impacts of micro-and-nano plastics on various organ systems in humans. EBioMedicine 99, 104901. doi:10.1016/j.ebiom.2023.104901
Bailey, J., Oliveri, A., and Levin, E. D. (2013). Zebrafish model systems for developmental neurobehavioral toxicology. Birth Defects Res. C Embryo Today 99 (1), 14–23. doi:10.1002/bdrc.21027
Bal-Price, A. K., Hogberg, H. T., Buzanska, L., Lenas, P., van Vliet, E., and Hartung, T. (2010). In vitro developmental neurotoxicity (DNT) testing: relevant models and endpoints. Neurotoxicology 31 (5), 545–554. doi:10.1016/j.neuro.2009.11.006
Barboza, L. G. A., Dick Vethaak, A., Lavorante, B., Lundebye, A. K., and Guilhermino, L. (2018). Marine microplastic debris: an emerging issue for food security, food safety and human health. Mar. Pollut. Bull. 133, 336–348. doi:10.1016/j.marpolbul.2018.05.047
Campen, M., Nihart, A., Garcia, M., Liu, R., Olewine, M., Castillo, E., et al. (2024). Bioaccumulation of microplastics in Decedent human brains assessed by Pyrolysis Gas Chromatography-Mass Spectrometry. Res. Sq. doi:10.21203/rs.3.rs-4345687/v1
Cao, X., Xie, W., Feng, M., Chen, J., Zhang, J., Luo, J., et al. (2024). Nanoplastic exposure Mediates neurodevelopmental toxicity by Activating the oxidative stress response in zebrafish (Danio rerio). ACS Omega 9 (14), 16508–16518. doi:10.1021/acsomega.4c00231
Casanova, A., Wevers, A., Navarro-Ledesma, S., and Pruimboom, L. (2023). Mitochondria: it is all about energy. Front. Physiol. 14, 1114231. doi:10.3389/fphys.2023.1114231
Chen, S., Chen, Y., Gao, Y., Han, B., Wang, T., Dong, H., et al. (2023). Toxic effects and mechanisms of nanoplastics on embryonic brain development using brain organoids model. Sci. Total Environ. 904, 166913. doi:10.1016/j.scitotenv.2023.166913
Das, A. (2023). The emerging role of microplastics in systemic toxicity: Involvement of reactive oxygen species (ROS). Sci. Total Environ. 895, 165076. doi:10.1016/j.scitotenv.2023.165076
Donisi, I., Colloca, A., Anastasio, C., Balestrieri, M. L., and D'Onofrio, N. (2024). Micro(nano)plastics: an emerging Burden for human health. Int. J. Biol. Sci. 20 (14), 5779–5792. doi:10.7150/ijbs.99556
Du, L., Liu, H., Song, X., Feng, X., Xu, H., Tang, W., et al. (2024). Developments in the field of intestinal toxicity and signaling pathways associated with rodent exposure to micro(nano)plastics. Toxicology 507, 153883. doi:10.1016/j.tox.2024.153883
Ducharme, N. A., Peterson, L. E., Benfenati, E., Reif, D., McCollum, C. W., Gustafsson, J. A., et al. (2013). Meta-analysis of toxicity and teratogenicity of 133 chemicals from zebrafish developmental toxicity studies. Reprod. Toxicol. 41, 98–108. doi:10.1016/j.reprotox.2013.06.070
Elersek, T., Novak, M., Mlinar, M., Virant, I., Bahor, N., Leben, K., et al. (2021). Lethal and sub-lethal effects and Modulation of gene expression induced by T Kinase Inhibitors in zebrafish (Danio rerio) embryos. Toxics 10 (1), 4. doi:10.3390/toxics10010004
Felix, L., Carreira, P., and Peixoto, F. (2023). Effects of chronic exposure of naturally weathered microplastics on oxidative stress level, behaviour, and mitochondrial function of adult zebrafish (Danio rerio). Chemosphere 310, 136895. doi:10.1016/j.chemosphere.2022.136895
Gan, Q., Cui, J., and Jin, B. (2023). Environmental microplastics: classification, sources, fates, and effects on plants. Chemosphere 313, 137559. doi:10.1016/j.chemosphere.2022.137559
Gupta, P., Mahapatra, A., Manna, B., Suman, A., Ray, S. S., Singhal, N., et al. (2024). Sorption of PFOS onto polystyrene microplastics potentiates synergistic toxic effects during zebrafish embryogenesis and neurodevelopment. Chemosphere 366, 143462. doi:10.1016/j.chemosphere.2024.143462
Hong, Y., Wu, S., and Wei, G. (2023). Adverse effects of microplastics and nanoplastics on the reproductive system: a comprehensive review of fertility and potential harmful interactions. Sci. Total Environ. 903, 166258. doi:10.1016/j.scitotenv.2023.166258
Horvatits, T., Tamminga, M., Liu, B., Sebode, M., Carambia, A., Fischer, L., et al. (2022). Microplastics detected in cirrhotic liver tissue. EBioMedicine 82, 104147. doi:10.1016/j.ebiom.2022.104147
Hu, M., and Palic, D. (2020). Micro- and nano-plastics activation of oxidative and inflammatory adverse outcome pathways. Redox Biol. 37, 101620. doi:10.1016/j.redox.2020.101620
Hua, T., Kiran, S., Li, Y., and Sang, Q. A. (2022). Microplastics exposure affects neural development of human pluripotent stem cell-derived cortical spheroids. J. Hazard Mater 435, 128884. doi:10.1016/j.jhazmat.2022.128884
Jeong, B., Kim, J. S., Kwon, A. R., Lee, J., Park, S., Koo, J., et al. (2024). Maternal nanoplastic ingestion induces an increase in offspring body weight through altered lipid species and microbiota. Environ. Int. 185, 108522. doi:10.1016/j.envint.2024.108522
Jin, S., Sarkar, K. S., Jin, Y. N., Liu, Y., Kokel, D., Van Ham, T. J., et al. (2013). An in vivo zebrafish screen identifies organophosphate antidotes with diverse mechanisms of action. J. Biomol. Screen 18 (1), 108–115. doi:10.1177/1087057112458153
Kalueff, A. V., Stewart, A. M., and Gerlai, R. (2014). Zebrafish as an emerging model for studying complex brain disorders. Trends Pharmacol. Sci. 35 (2), 63–75. doi:10.1016/j.tips.2013.12.002
Krewski, D., Acosta, D., Andersen, M., Anderson, H., Bailar, J. C., Boekelheide, K., et al. (2010). Toxicity testing in the 21st century: a vision and a strategy. J. Toxicol. Environ. Health B Crit. Rev. 13 (2-4), 51–138. doi:10.1080/10937404.2010.483176
LaMontagne, E., Muotri, A. R., and Engler, A. J. (2022). Recent advancements and future requirements in vascularization of cortical organoids. Front. Bioeng. Biotechnol. 10, 1048731. doi:10.3389/fbioe.2022.1048731
Lancaster, M. A., and Knoblich, J. A. (2014). Organogenesis in a dish: modeling development and disease using organoid technologies. Science 345 (6194), 1247125. doi:10.1126/science.1247125
Lee, S. E., Yi, Y., Moon, S., Yoon, H., and Park, Y. S. (2022). Impact of micro- and nanoplastics on mitochondria. Metabolites 12 (10), 897. doi:10.3390/metabo12100897
Lei, P., Zhang, W., Ma, J., Xia, Y., Yu, H., Du, J., et al. (2023). Advances in the Utilization of zebrafish for assessing and understanding the mechanisms of nano-/Microparticles toxicity in Water. Toxics 11 (4), 380. doi:10.3390/toxics11040380
Li, Y., Chen, L., Zhou, N., Chen, Y., Ling, Z., and Xiang, P. (2024). Microplastics in the human body: a comprehensive review of exposure, distribution, migration mechanisms, and toxicity. Sci. Total Environ. 946, 174215. doi:10.1016/j.scitotenv.2024.174215
Liu, W., Zeng, M., Li, Y., Chen, G., and Wang, J. (2024). Polystyrene nanoplastics mediate skeletal toxicity through oxidative stress and the BMP pathway in zebrafish (Danio rerio). Ecotoxicol. Environ. Saf. 285, 117096. doi:10.1016/j.ecoenv.2024.117096
Lu, T., Li, D., Yuan, X., Wang, Z., Shao, Z., Feng, X., et al. (2024). Potential effects of Orally ingesting polyethylene terephthalate microplastics on the Mouse Heart. Cardiovasc Toxicol. 24 (3), 291–301. doi:10.1007/s12012-024-09837-6
Mahmud, F., Sarker, D. B., Jocelyn, J. A., and Sang, Q. A. (2024). Molecular and cellular effects of microplastics and nanoplastics: focus on inflammation and Senescence. Cells 13 (21), 1788. doi:10.3390/cells13211788
Malhotra, N., Chen, J. R., Sarasamma, S., Audira, G., Siregar, P., Liang, S. T., et al. (2019). Ecotoxicity assessment of Fe(3)O(4) Magnetic nanoparticle exposure in adult zebrafish at an environmental Pertinent concentration by behavioral and Biochemical testing. Nanomater. (Basel) 9 (6), 873. doi:10.3390/nano9060873
Martin-Folgar, R., Gonzalez-Caballero, M. C., Torres-Ruiz, M., Canas-Portilla, A. I., de Alba Gonzalez, M., Liste, I., et al. (2024). Molecular effects of polystyrene nanoplastics on human neural stem cells. PLoS One 19 (1), e0295816. doi:10.1371/journal.pone.0295816
Nwokoye, P. N., and Abilez, O. J. (2024). Blood vessels in a dish: the evolution, challenges, and potential of vascularized tissues and organoids. Front. Cardiovasc. Med. 11, 1336910. doi:10.3389/fcvm.2024.1336910
OECD (2023). Initial Recommendations on evaluation of data from the developmental neurotoxicity (DNT) in-vitro testing battery
Planchart, A., Mattingly, C. J., Allen, D., Ceger, P., Casey, W., Hinton, D., et al. (2016). Advancing toxicology research using in vivo high throughput toxicology with small fish models. ALTEX 33 (4), 435–452. doi:10.14573/altex.1601281
Popov, L. D. (2023). Mitochondria as intracellular signalling organelles. An update. Cell Signal 109, 110794. doi:10.1016/j.cellsig.2023.110794
Prust, M., Meijer, J., and Westerink, R. H. S. (2020). The plastic brain: neurotoxicity of micro- and nanoplastics. Part Fibre Toxicol. 17 (1), 24. doi:10.1186/s12989-020-00358-y
Quadrato, G., Brown, J., and Arlotta, P. (2016). The promises and challenges of human brain organoids as models of neuropsychiatric disease. Nat. Med. 22 (11), 1220–1228. doi:10.1038/nm.4214
Ruan, Y., Zhong, Z., Liu, X., Li, Z., Li, J., Sun, L., et al. (2023). Correlation between cellular uptake and cytotoxicity of polystyrene micro/nanoplastics in HeLa cells: a size-dependent matter. PLoS One 18 (8), e0289473. doi:10.1371/journal.pone.0289473
Saha, S. C., and Saha, G. (2024). Effect of microplastics deposition on human lung airways: a review with computational benefits and challenges. Heliyon 10 (2), e24355. doi:10.1016/j.heliyon.2024.e24355
Shen, W., Lou, B., Xu, C., Yang, G., Yu, R., Wang, X., et al. (2020). Lethal toxicity and gene expression changes in embryonic zebrafish upon exposure to individual and mixture of malathion, chlorpyrifos and lambda-cyhalothrin. Chemosphere 239, 124802. doi:10.1016/j.chemosphere.2019.124802
Silva, M. G., Oliveira, M. M., and Peixoto, F. (2023). Assessing micro and nanoplastics toxicity using rodent models: investigating potential mitochondrial implications. Toxicology 499, 153656. doi:10.1016/j.tox.2023.153656
Sobanska, M., Scholz, S., Nyman, A. M., Cesnaitis, R., Gutierrez Alonso, S., Kluver, N., et al. (2018). Applicability of the fish embryo acute toxicity (FET) test (OECD 236) in the regulatory context of Registration, Evaluation, Authorisation, and Restriction of Chemicals (REACH). Environ. Toxicol. Chem. 37 (3), 657–670. doi:10.1002/etc.4055
Tait, S. W., and Green, D. R. (2012). Mitochondria and cell signalling. J. Cell Sci. 125 (Pt 4), 807–815. doi:10.1242/jcs.099234
Tao, M., Wang, C., Zheng, Z., Gao, W., Chen, Q., Xu, M., et al. (2024). Nanoplastics exposure-induced mitochondrial dysfunction contributes to disrupted stem cell differentiation in human cerebral organoids. Ecotoxicol. Environ. Saf. 285, 117063. doi:10.1016/j.ecoenv.2024.117063
Tice, R. R., Austin, C. P., Kavlock, R. J., and Bucher, J. R. (2013). Improving the human hazard characterization of chemicals: a Tox21 update. Environ. Health Perspect. 121 (7), 756–765. doi:10.1289/ehp.1205784
Wang, T., Yi, Z., Liu, X., Cai, Y., Huang, X., Fang, J., et al. (2024). Multimodal detection and analysis of microplastics in human thrombi from multiple anatomically distinct sites. EBioMedicine 103, 105118. doi:10.1016/j.ebiom.2024.105118
Wu, B., Yu, H., Yi, J., Lei, P., He, J., Ruan, J., et al. (2024). Behavioral studies of zebrafish reveal a new perspective on the reproductive toxicity of micro- and nanoplastics. Toxics 12 (3), 178. doi:10.3390/toxics12030178
Wu, X., Kreutz, A., Dixon, D., and Tokar, E. J. (2025). Engineering human cerebral organoids to explore mechanisms of arsenic-induced developmental neurotoxicity. Toxicol. Appl. Pharmacol. 496, 117230. doi:10.1016/j.taap.2025.117230
Xicoy, H., Wieringa, B., and Martens, G. J. (2017). The SH-SY5Y cell line in Parkinson's disease research: a systematic review. Mol. Neurodegener. 12 (1), 10. doi:10.1186/s13024-017-0149-0
Yan, Y., Yang, Z., and Chen, L. (2024). High-quality models for assessing the effects of environmental pollutants on the nervous system: 3D brain organoids. Ecotoxicol. Environ. Saf. 284, 116876. doi:10.1016/j.ecoenv.2024.116876
Yang, W., Jannatun, N., Zeng, Y., Liu, T., Zhang, G., Chen, C., et al. (2022). Impacts of microplastics on immunity. Front. Toxicol. 4, 956885. doi:10.3389/ftox.2022.956885
Yao, Y., Zhang, T., and Tang, M. (2024). Toxicity mechanism of engineered nanomaterials: focus on mitochondria. Environ. Pollut. 343, 123231. doi:10.1016/j.envpol.2023.123231
Yee, M. S., Hii, L. W., Looi, C. K., Lim, W. M., Wong, S. F., Kok, Y. Y., et al. (2021). Impact of microplastics and nanoplastics on human health. Nanomater. (Basel) 11 (2), 496. doi:10.3390/nano11020496
Zhang, S., Wan, Z., and Kamm, R. D. (2024). Vascularized organoids on a chip: strategies for engineering organoids with functional vasculature. Lab Chip 21 (3), 473–488. doi:10.1039/d0lc01186j
Zhou, Y., Wang, Y., Peijnenburg, W., Vijver, M. G., Balraadjsing, S., Dong, Z., et al. (2024). Application of machine learning in Nanotoxicology: a critical review and perspective. Environ. Sci. & Technol. 58 (34), 14973–14993. doi:10.1021/acs.est.4c03328
Keywords: micro-and nanoplastics, developmental neurotoxicity, metabolism, zebrafish, organoid
Citation: Wu X, Leung T, Jima DD, Iyangbe M and Bang J (2025) Developing a feasible fast-track testing method for developmental neurotoxicity studies: alternative model for risk assessment of micro- and nanoplastics. Front. Toxicol. 7:1567225. doi: 10.3389/ftox.2025.1567225
Received: 26 January 2025; Accepted: 02 April 2025;
Published: 15 April 2025.
Edited by:
Omayma Missawi, University of Namur, BelgiumReviewed by:
Lara Nigro, University of Milan, ItalyCopyright © 2025 Wu, Leung, Jima, Iyangbe and Bang. This is an open-access article distributed under the terms of the Creative Commons Attribution License (CC BY). The use, distribution or reproduction in other forums is permitted, provided the original author(s) and the copyright owner(s) are credited and that the original publication in this journal is cited, in accordance with accepted academic practice. No use, distribution or reproduction is permitted which does not comply with these terms.
*Correspondence: Xian Wu, d3V4MjNAZWN1LmVkdQ==; John Bang, ampiYW5nQG5jY3UuZWR1
Disclaimer: All claims expressed in this article are solely those of the authors and do not necessarily represent those of their affiliated organizations, or those of the publisher, the editors and the reviewers. Any product that may be evaluated in this article or claim that may be made by its manufacturer is not guaranteed or endorsed by the publisher.
Research integrity at Frontiers
Learn more about the work of our research integrity team to safeguard the quality of each article we publish.