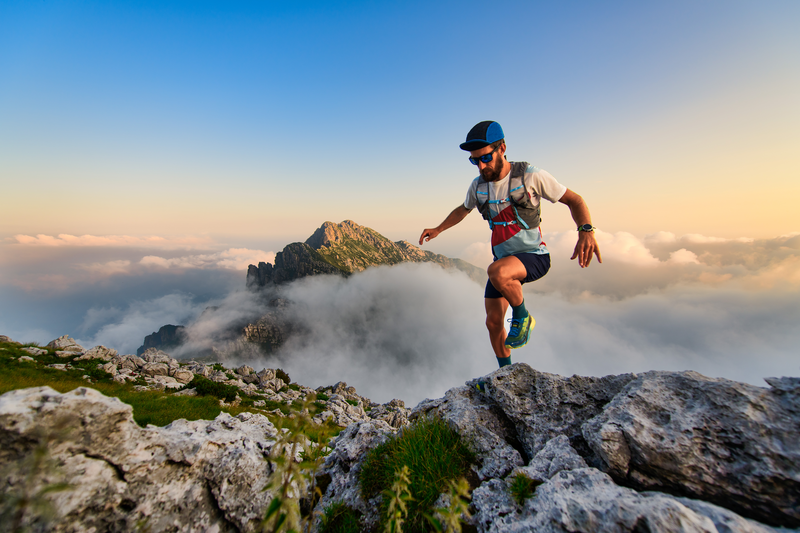
94% of researchers rate our articles as excellent or good
Learn more about the work of our research integrity team to safeguard the quality of each article we publish.
Find out more
ORIGINAL RESEARCH article
Front. Toxicol.
Sec. Neurotoxicology
Volume 7 - 2025 | doi: 10.3389/ftox.2025.1560980
This article is part of the Research Topic Understanding the Link Between Environmental Pollutants, Brain & Behavior View all 8 articles
The final, formatted version of the article will be published soon.
You have multiple emails registered with Frontiers:
Please enter your email address:
If you already have an account, please login
You don't have a Frontiers account ? You can register here
Microplastics are ubiquitous environmental pollutants with potential neurotoxic effects that can impair learning and memory. MicroRNAs are essential regulators of a number of physiological and pathological processes, but detailed information on the impact of miRNAs on the neurotoxic effects of microplastics is lacking. In the present study, polystyrene microplastics (PS-MPs) were administered orally and, miR-103a-3p was injected intraventricularly as a treatment for PS-MPs-induced neurotoxicity. Performance in the novel object discrimination (NOD), Y-maze and Barnes maze tests indicated that miR-103a-3p mitigates the deleterious effects of PS-MPs on learning and memory.Oxidative stress, pyroptosis, apoptosis and inflammation induced by PS-MPs were modulated after miR-103a-3p injection by reducing MDA, protein carbonyl, nitrite, caspase 3, caspase 1, TNFα, and NLRP3 levels in hippocampal tissue. Our results also showed that miR-103a-3p can reverse the impact of PS-MPs on astrocytic reaction and SIRT1 and BDNF levels. MiR-103a-3p alleviated PS-MPs-induced endoplasmic reticulum (ER) stress through reducing the levels of PERK, CHOP and GRP78. These findings imply that miR-103a-3p exerts a neuroprotective influence against cognitive deficits induced by exposure to PS-MPs. This is achieved by reducing inflammation, oxidative stress, apoptosis and ER stress.
Keywords: Polystyrene microplastic, MiR-103a-3p, cognitive deficits, Neurotoxicity, Endoplasmic Reticulum Stress
Received: 15 Jan 2025; Accepted: 19 Mar 2025.
Copyright: © 2025 Baluchnejadmojarad, Mohammadi, Goudarzi, Khodashenas, Khoshravesh and Roghani. This is an open-access article distributed under the terms of the Creative Commons Attribution License (CC BY). The use, distribution or reproduction in other forums is permitted, provided the original author(s) or licensor are credited and that the original publication in this journal is cited, in accordance with accepted academic practice. No use, distribution or reproduction is permitted which does not comply with these terms.
* Correspondence:
Tourandokht Baluchnejadmojarad, Iran University of Medical Sciences, Tehran, Iran
Disclaimer: All claims expressed in this article are solely those of the authors and do not necessarily represent those of their affiliated organizations, or those of the publisher, the editors and the reviewers. Any product that may be evaluated in this article or claim that may be made by its manufacturer is not guaranteed or endorsed by the publisher.
Research integrity at Frontiers
Learn more about the work of our research integrity team to safeguard the quality of each article we publish.