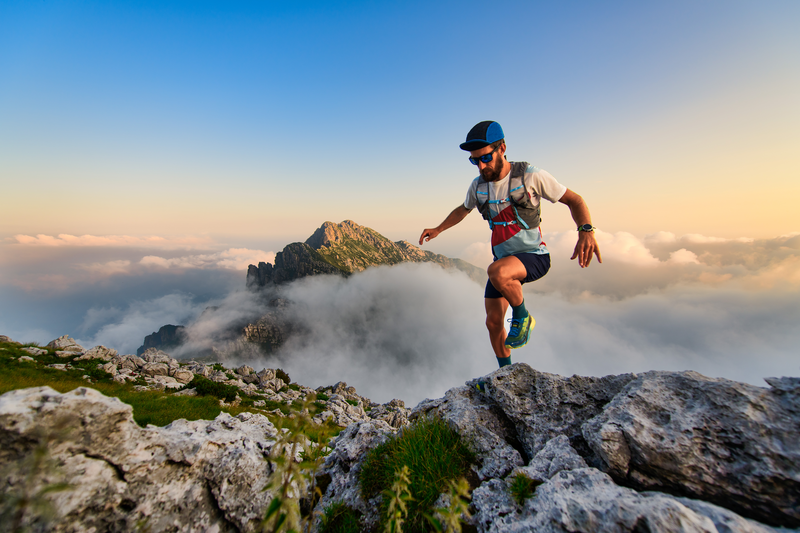
94% of researchers rate our articles as excellent or good
Learn more about the work of our research integrity team to safeguard the quality of each article we publish.
Find out more
REVIEW article
Front. Toxicol.
Sec. Clinical Toxicology
Volume 7 - 2025 | doi: 10.3389/ftox.2025.1558721
This article is part of the Research Topic The toxicology of cannabis and cannabis-based products View all 6 articles
The final, formatted version of the article will be published soon.
You have multiple emails registered with Frontiers:
Please enter your email address:
If you already have an account, please login
You don't have a Frontiers account ? You can register here
This narrative review synthesizes the toxicological, clinical and medico-legal aspects of paediatric cannabis intoxication. By providing a comprehensive overview, it aims to inform future research, guide policymaking, and enhance clinical and toxicological practice in addressing this growing public health concern. The pharmacokinetics of cannabinoid ingestion in children are significantly influenced by the immaturity of their gastrointestinal tract and metabolic enzyme systems, resulting in altered oral bioavailability. Clinical data indicate that Δ9-tetrahydrocannabinol (THC)-related effects in paediatricpaediatric patients typically emerge within two hours of ingestion, with more severe symptoms developing within four hours. The endocannabinoid system (ECS) undergoes significant developmental changes, with marked differences in cannabinoid receptor expression and distribution across fetal, neonatal, and adult brains. During neurodevelopment, CB1 receptors exhibit unique expression patterns, including transient localization in brainstem regions critical for neurovegetative functions. These developmental dynamics likely explain children’s heightened sensitivity to THC’s neurological and neurovegetative effects, often resulting in more severe outcomes compared to adults. The reliable detection of cannabinoids involves integrating screening methods with confirmatory analytical techniques. Urine immunoassay testing is widely considered an helpful toolto assess a previous exposure, becoming positive within 3–4 hours of ingestion. However, this method is prone to false positives. Plasma THC concentration, when measured close to the event, offers valuable insights into the quantity ingested and the correlation between exposure and clinical outcomes in the impairment window. Hair analysis, while useful for distinguishing between acute and chronic use, is susceptible to various biases. The rising incidence of acute cannabis intoxication in children underscores the urgent need for targeted public health interventions and stricter regulatory frameworks. Preventive measures such as child-resistant packaging, public education campaigns, and cannabis use screening during pregnancy are essential to mitigate risks. Clinicians should consider THC exposure in the differential diagnosis of children presenting with unexplained neurological, immune, or metabolic symptoms.
Keywords: Cannabis, thc, intoxication, Paediatric, Children, Toxicology, medico-legal issues, forensic
Received: 10 Jan 2025; Accepted: 14 Mar 2025.
Copyright: © 2025 Malta, Albano, Lavanco, Brancato, Cannizzaro, Argo, Contorno, Plescia and Zerbo. This is an open-access article distributed under the terms of the Creative Commons Attribution License (CC BY). The use, distribution or reproduction in other forums is permitted, provided the original author(s) or licensor are credited and that the original publication in this journal is cited, in accordance with accepted academic practice. No use, distribution or reproduction is permitted which does not comply with these terms.
* Correspondence:
Giuseppe Davide Albano, University of Palermo, Palermo, Italy
Gianluca Lavanco, University of Palermo, Palermo, Italy
Disclaimer: All claims expressed in this article are solely those of the authors and do not necessarily represent those of their affiliated organizations, or those of the publisher, the editors and the reviewers. Any product that may be evaluated in this article or claim that may be made by its manufacturer is not guaranteed or endorsed by the publisher.
Research integrity at Frontiers
Learn more about the work of our research integrity team to safeguard the quality of each article we publish.